Short-term response of research activities on white shark behaviour
Yuri Niella
A School of Natural Sciences, Faculty of Science and Engineering, Macquarie University, North Ryde, Sydney, NSW 2113, Australia.
B Southern Shark Ecology Group, College of Science and Engineering, Flinders University, Adelaide, SA 5042, Australia.
C South Australian Department for Environment and Water, Adelaide, SA 5000, Australia.
D Rodney Fox Shark Expeditions, Adelaide, SA 5031, Australia.
E Calypso Star Charters, Port Lincoln, SA 5606, Australia.
F Adventure Bay Chartres, Port Lincoln, SA 5606, Australia.
G South Australian Research and Development Institute – Aquatic Sciences, Adelaide, SA 5024, Australia.
H Innovasea, Bedford, NS B4B 0L9, Canada.
Wildlife Research 50(4) 260-271 https://doi.org/10.1071/WR22004
Submitted: 27 January 2022 Accepted: 27 September 2022 Published: 4 November 2022
© 2023 The Author(s) (or their employer(s)). Published by CSIRO Publishing. This is an open access article distributed under the Creative Commons Attribution-NonCommercial-NoDerivatives 4.0 International License (CC BY-NC-ND)
Abstract
Context: Researchers studying animals need to ensure that sampling procedures and the methods they use are as harmless and non-disruptive as possible, particularly when their focal species are threatened or protected. White sharks (Carcharodon carcharias) are Vulnerable under the IUCN Red List, protected globally, and are frequently studied by marine ecologists.
Aims: To assess white shark responses to research activities (i.e. tagging and biopsy procedures, and electric deterrent trials) conducted at the Neptune Islands Group Marine Park (South Australia, Australia).
Methods: Trends in shark residency following research activities were assessed by comparing shark abundance (number of sharks detected by acoustic receivers and sighted by cage-diving operators) before, during, and after scientific expeditions, and to natural fluctuations in the absence of research activities using 8 years (2013–2021) of acoustic tracking and daily sighting reports from a wildlife tourism industry.
Key results: Number of white sharks and residency decreased after sampling. However, changes observed following research activities were similar to natural fluctuations, suggesting that these changes reflected natural variations rather than being due to sharks responding negatively to the research activities.
Conclusions: Our study showed that external tagging, biopsies, or deterrent trials do not affect short- and long-term residency or abundance of white sharks, probably owing to the research activities being minimally intrusive and to sharks having efficient immune systems and remarkable ability to heal from injuries.
Implications: Re-evaluating study methods forms part of the researcher’s responsibilities to ensure best practice and to abide by national and international codes for the care and use of animals for scientific purposes.
Keywords: acoustic telemetry, animal welfare, biopsy sampling, cage-diving, research impacts, shark deterrents, white sharks, wildlife tourism.
Introduction
Considerations of animal welfare is increasingly gaining attention across social and political agendas (Dawkins 2006). In the realm of scientific investigations, researchers that study animals (e.g. biologists, ecologists) are faced with the ethical responsibility and legal obligations of reducing the impacts of their studies on the animal they study and surrounding ecosystems. Globally, various legislative acts and related codes of conduct dictate how scientists should be using animals (Beauchamp and DeGrazia 2019; Palmer and Greenhough 2021). The concept of replacement, reduction, and refinement, also known as the three Rs (Russell and Burch 1959), often forms the basis of animal use for scientific purposes. The idea of the three Rs is based on the premise that the use of animals for research is acceptable only when non-animal alternatives are impossible, and the most benignant methods are conducted, with the smallest number of animals necessary to record data on sufficient individuals for the study to be scientifically robust (Fenwick et al. 2009). In many cases, replacement strategies that use non-animal systems such as computer programs and simulations (Fenwick et al. 2009; Crozier and Schulte-Hostedde 2015) are often inadequate, leading to refinement and reduction being the techniques mostly used to minimise the impacts of research activities.
Refinement aims to reduce pain and distress experienced by the animals involved in scientific activities. The principle of ‘no unnecessary harm’ postulates that scientists must, to the best of their abilities, inflict only the minimum necessary pain when handling their study animals (DeGrazia and Beauchamp 2019). Advances in less invasive methods, such as non-lethal approaches and the development of increasingly small tags, allow researchers to re-use the same individuals and deploy several devices simultaneously. However, this reduction in the number of individuals used may lead to cumulative effects on the individuals that are indeed sampled, thus potentially exacerbating impacts, and must therefore be considered with caution (Fenwick et al. 2009). As part of the refinement process, there is a need to regularly re-assess the impacts scientists might have on the individuals they study. This is even more important when the study site and studied animals are shared among stakeholders (Palmer and Greenhough 2021), such as, for example, when research activities are conducted at the same places where wildlife tourism industries operate, or when the focus species is threatened or protected.
The white shark (Carcharodon carcharias) is listed as globally Vulnerable by the IUCN Red List (Rigby et al. 2019) and is protected both internationally and within Australian waters (Department of Sustainability Environment Water Population and Communities 2013). Commercial white shark cage-diving started in Australia during the late 1970s off the Eyre Peninsula, South Australia. It then expanded globally across the species’ range and currently also takes place in South Africa, the United States of America, Mexico (Guadalupe Island), and New Zealand (Huveneers et al. 2018a). In Australian waters, these activities are now limited to the Neptune Islands Group (Ron and Valerie Taylor) Marine Park (hereafter referred to as the Neptune Islands) located 60–70 km south of Port Lincoln, South Australia. Previous studies have shown changes in white shark spatio-temporal distribution and residency at the Neptune Islands resulting from the cage-diving industry (Bruce and Bradford 2013; Huveneers et al. 2013a). This led to the South Australian government developing and implementing a new policy, limiting the number of commercial tour operators and maximum number of weekly days of activity in 2012, along with a monitoring program to assess whether the new policy successfully returns white shark residency to baseline levels pre-tourism expansion in 2007. As a part of this monitoring program, ~20 white sharks are externally tagged with acoustic tags throughout the year (Huveneers and Niella 2022). While external tagging has not been shown to detrimentally affect the health or condition of tagged fish or sharks (Jepsen et al. 2015), we should also ensure that it does not lead to tagged sharks temporarily leaving the area as a result of the tagging event.
A key factor in assessing the sustainability and acceptability of wildlife tourism industries is determining whether operator activities disrupt the diet, natural foraging, or nutritional condition of the animals (Meyer et al. 2022). Despite not directly feeding white sharks, concerns that occasional incidental feeding occurs when bait handlers are caught unaware and bait (southern bluefin tuna, Thunnus maccoyii, heads and gills) was consumed (Huveneers et al. 2015; Meyer et al. 2021), warranted an assessment of the diet of white sharks at the Neptune Islands, using fatty acid analysis of muscle samples (Meyer et al. 2019). This necessitated the use of a modified speargun to take biopsies from free-swimming white sharks (Meyer et al. 2018). Although this minimally invasive approach has eliminated the need to capture sharks, reducing the stress associated with sample collection, it is imperative that we ensure that this method does not lead to sharks leaving the area, or discourage interactions with the cage-diving industry.
White sharks are one of the main species involved in shark–human interactions worldwide (Curtis et al. 2012). Government initiatives to manage the risk of shark bites have historically relied on the use of lethal approaches such as shark nets (Krogh and Reid 1996). More recently, there has been strong public appeal to replace such strategies with non-lethal approaches (Meeuwig and Ferreira 2014; Simmons and Mehmet 2018). For example, drones can pre-emptively detect potentially dangerous sharks in proximity to bathers and warn them (Butcher et al. 2019; Gorkin et al. 2020) or shark–management–alert–in–real-time (SMART) drumlines can catch shark and enable release further offshore from popular beaches, with minimal detrimental effects for the captured animals (Gallagher et al. 2019; Tate et al. 2019). When these methods aiming to reduce the overlap between sharks and humans are not sufficient, personal electric deterrents can reduce the risk of sharks biting humans (Huveneers et al. 2013b, 2018b; Kempster et al. 2016). With the Neptune Islands providing a predictable and easily accessible white shark aggregation, it becomes a sought-after site to test shark bite-mitigation measures such as personal electric deterrents (e.g. Huveneers et al. 2013b, 2018b). Although the efficacy of electric deterrents decreases rapidly with distance and it is not expected that it would repel sharks further than a few metres (Huveneers et al. 2018b), concerns were raised by the cage-diving industry about the potential impact of sharks interacting with the deterrents and possibly leaving the area as a result of the deterrent testing (A. Wright, pers. comm.).
Because of the ethical considerations required in any scientific activities, and concerns raised about the potential for sampling activities or deterrent testing to affect white shark residency at the Neptune Islands and temporarily lead to sharks leaving the Neptune Islands where the cage-diving industry relies on shark sighting, we aimed to assess the short-term responses of white sharks to external tagging with acoustic transmitters and biopsies (hereafter referred to as sampling), and trials testing the efficacy of electric deterrents. Eight years of acoustic tracking and shark sighting were used to investigate shark response prior to, during, and after each scientific expedition. We hypothesise that our research activities are minimally invasive to such large-bodied marine predators and do not alter their natural behaviour. Consequently, white shark responses (i.e. length of residency, number of sharks detected, and number of sharks sighted) following scientific expeditions were expected to be similar to natural conditions (i.e. when no research activities are being conducted). Understanding how marine megafauna respond to research activities is of outmost importance, particularly in the case of threatened species such as the white shark, to help us determine whether new methods or techniques are required to further minimise the impacts of scientific research.
Materials and methods
Study area and cage-diving sighting data
In Australia, cage-diving with white sharks is allowed only at the Neptune Islands (35.2°S, 136.1°E), located ~70 km south of Port Lincoln, South Australia. The industry is managed by the South Australian Department for Environment and Water, which limits the number of commercial tour operators, maximum number of days they can operate, and the amount of food-based attractant that can be used. White sharks were attracted using a mixture of fish oil and minced southern bluefin tuna (T. maccoyii) dispersed on the water (i.e. berley or chum), and with a piece of tuna attached to a float deployed at ~15 m from the stern of the cage-diving vessels. As part of the new policy implemented in 2012, a monitoring program was initiated requiring cage-diving operators to record their location and number of sharks sighted using a mobile phone app (Nazimi et al. 2018).
Shark sampling (tagging and biopsies) and electric deterrent trial expeditions
Because white sharks occur at the Neptune Islands year-round (Bruce and Bradford 2015; Schilds et al. 2019), research activity also occurred throughout the year and was typically timed when the cage-diving operators reported more than approximately five sharks for a few days to optimise research opportunities. Tagging, biopsies, and electric deterrent trials were conducted on board cage-diving vessels at the Neptune Islands. White sharks were tagged with V16-6H acoustic transmitters (Innovasea), tethered to a Domeier umbrella dart-tag head by using a 10- to 15-cm-long stainless wire trace (1.6 mm in diameter). Tags were implanted in the dorsal musculature of free-swimming sharks by using a modified spear-gun applicator. In total, 133 white sharks (mean ± s.d. = 2 ± 2 sharks tagged per expedition) were tagged during a total of 50 scientific expeditions spanning from 14 September 2013 to 5 July 2021. Biopsies were also taken from the dorsal musculature of free-swimming sharks by using the same modified speargun used to implant tags, but with a hollow 1-cm-diameter stainless steel biopsy probe affixed to the end of the modified spear (Meyer et al. 2018), in place of a dart-tag head attachment. Biopsies were taken over a total of 14 expeditions between 24 September 2013 and 4 July 2020, totalling 54 biopsies (mean ± s.d. = 4 ± 3 sharks sampled per expedition).
After an individual white shark was observed to show interest in the tuna section, electric deterrent trials commenced by replacing it with the electric deterrent set-up. This consisted of a 120 cm × 30 cm custom-built surfboard model to which four commercially available personal electric deterrents (Shark Shield Pty Ltd, Freedom+ Surf, Rpela and SharkBanz bracelet) were attached at a time, together with a tuna gill ~30 cm to attract sharks towards the device. Trials were run for 15 min or until a white shark touched the bait/board. Since it was not the purpose of this study to evaluate how different electric deterrents affect white sharks, the different models were pooled for analyses. For more details on the electric deterrent trials set-up refer to Huveneers et al. (2018b). In total, 458 electric deterrent trials were conducted during 10 scientific expeditions between 8 September 2017 and 22 May 2020 (mean ± s.d. = 46 ± 50 trials per expedition).
Changes in shark residency
White sharks were monitored using three acoustic receivers (Innovasea, VR2W) deployed at the Neptune Islands between September 2013 and July 2021 (Fig. 1). We estimated a detection range of ~300 m (80% detection) by using a Vemco Positioning System at the study site (C. Huveneers, unpubl. data). The three receivers, therefore, covered all three main berleying locations. Most sharks (90%) were first detected within 12 h (Supplementary material Fig. S1). Visit events were identified for each tracked shark and consisted of an individual not being absent from the study area for more than five consecutive days (Bruce and Bradford 2013). Residency times were calculated for each shark and visit event, and corresponded to the respective number of days elapsed between the first and last acoustic detection. We used an ANOVA to compare shark residency following tagging and biopsy activities and found no statistical differences between the two activities (P-value = 0.843). Therefore, we combined tagging and biopsy expeditions.
Changes in shark residency time (response variable) were investigated with a generalised additive mixed model (GAMM) by using a Poisson distribution according to the following formula:

in which the candidate fixed predictor included an interaction between the variables visit event (Visit) and Month. The variables Year (continuous) and shark identification (Shark ID; categorical) were also included as random-factor covariates to account for any possible interannual or intra-individual variations respectively. A thin-plate regression spline was applied to the fixed variable with an interaction, and the dimension basis of its smooth was term kept (k) at a fixed value of 10 to avoid model overfitting.
Changes in the proportion of individuals detected on acoustic receivers
A sensitivity analysis was conducted to identify the best window size (i.e. number of days) to investigate changes in the number of individuals present at the Neptune Islands following each scientific expedition (mean time between expeditions = 57 ± 54 days). This consisted of a linear regression to assess how different window sizes (ranging from 5 to 15 days) influenced the daily maximum number of white sharks detected at the Neptune Islands. Because values ≥10 days led to significantly higher maximum numbers of individuals detected (Fig. S2), a window size of 10 days was selected. The daily maximum numbers of white shark detected by the acoustic receivers and sighted by the cage-diving operators (Fig. S3) were compared during the total monitoring period by using a Pearson’s correlation. Because the two metrics were significantly correlated (Pearson’s correlation = 0.3; P < 0.001), we also used the same window size for the analysis with the number of sharks sighted by cage-diving operators as the response variable (see section ‘Changes in the number of individuals sighted by cage-diving operators’).
Changes in the proportions of currently (i.e. sharks tagged in the most recent expedition) and previously (i.e. sharks tagged in previous expeditions that were detected within the 10 days prior) tagged sharks were assessed independently and compared. This approach enabled the use of previously tagged sharks to investigate the immediate impacts of tagging, i.e. by assessing whether currently tagged sharks would leave the study area quicker than sharks tagged previously. For currently tagged sharks, the number of individuals tagged in the last expedition was considered the baseline value (i.e. 100% frequency). The number of different sharks (i.e. from individuals tagged in previous expeditions) detected in the 10 days prior to the latest sampling expedition was considered as the baseline frequency for previously tagged sharks. Starting from the last day of each sampling expedition, the daily proportions of currently and previously tagged sharks detected at the Neptune Islands were calculated for each of the following 30 days. The daily proportions of currently tagged sharks were calculated using the formula
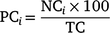
in which the proportion of currently tagged sharks (PC) detected in each day (i) corresponded to the number of individuals (NC) detected on that day in relation to the total number of sharks tagged in the last expedition (TC). For the previously tagged sharks, the daily proportions of individuals detected were calculated using the formula
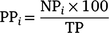
in which the proportion of previously tagged sharks (PP) detected in each day (i) corresponded to the number of individuals (NP) detected on that day in relation to the total number of previously tagged sharks detected during the 10 days prior to the last expedition (TP).
Changes in the proportions of currently and previously tagged sharks (response variables) as a function of days since the latest sampling expedition (Days; candidate fixed predictor) were assessed with independent GAMMs by using binomial distributions according to the following formula:

Because the effects of Month were found to be not significant in these models, this variable was not included as a fixed term. Models included the variables Month (continuous), Year (continuous), and sampling expedition identification (Expedition ID; categorical) as random-factor covariates to account for any possible seasonal, interannual, and between-expedition variations respectively. A thin-plate regression spline was applied to the fixed variable by using a fixed value of k = 5 to avoid model overfitting.
Changes in the number of individuals sighted by cage-diving operators
To obtain a baseline value that would represent natural variations in the number of sharks sighted irrespective of scientific activities, the research expeditions were first excluded from the shark-sighting dataset. Because research expeditions were organised when the number of white sharks at the Neptune Islands was consistently high (i.e. ≳5 sharks), we identified periods of similarly high white shark presence without research activities to enable adequate comparisons. Research expeditions were first excluded from the shark-sighting dataset, after which it was split into 10-day moving windows and assessed for maximum daily number of sharks sighted. Only those windows with an average number of sharks sighted equal or higher than five (46.6%) were kept in the final natural variation dataset and are hereafter referred to as natural events.
The total shark-sighting dataset spanning from September 2013 to July 2021 was then divided into 30-day intervals, starting on the last day of each event (i.e. natural events, and both sampling and electric deterrent trial expeditions). For each event type, the daily frequencies of sharks sighted were calculated using the following formula:

where the daily frequency of sharks sighted (F) on that day (i, varying from one to 30) corresponded to the number of sharks sighted on that day (N) in relation to the maximum number of sharks sighted during the previous 10 days prior to each event end (T).
The variations in the frequency of sharks sighted (response variable) were calculated using binomial distributions with independent GAMMs for each of the three event types according to the following formula:

where the interaction between the variables days since last event (Day) and Month were included as candidate fixed predictors, and the effects of Year (continuous) and event identification (Event ID; categorical) were included as random-factor covariates to account for any possible interannual and between-event variations respectively. Again, a thin-plate regression spline was applied to the fixed variable with an interaction, and model overfitting was avoided by using a fixed value of k = 10. All statistical analyses were conducted in R (ver. 4.2.0), and the GAMMs were conducted with the mgcv package (Wood 2017).
Ethics approval
This project was undertaken under the Department for Environment and Water permit number Q26292. Tagging and biopsies were undertaken under Flinders University ethics approval numbers E398 and E464-17.
Results
In total, 126 white sharks were detected by acoustic receivers at the Neptune Islands between 14 September 2013 and 7 July 2021, with <0.1% of the tags known to have shed (tag being detected continuously by only one receiver or shark returning without a tag). Tagged sharks had a mean number of approximately four visits to the study area (Fig. 2a), including the first visit when tagged, and a mean residency time of ~12 days (Fig. 2b). Whereas most individuals tracked had less than four visit events to the study area, three sharks had more than 10 visits, one of which returned to the Neptune Islands for 16 events (Fig. 2a). White shark residency times were usually shorter than 60 days, but one individual remained at the Neptune Islands for 180 days during its third visit (Fig. 2b).
![]() |
Variation in residency
Changes in white shark residency were significantly influenced by the interaction between Visit and Month, and varied across Years and the different individuals tracked, with Shark ID explaining most of the variation (Table 1; Fig. S4). Shark residency times tended to decrease as a function of visit events, with most individuals returning up to seven times to the Neptune Islands (Fig. 3a). Seasonality in residency was virtually absent for most of the tagged sharks, but individuals with more than seven visits had higher residency times between July and December (Fig. 3b).
Variation in the frequency of individuals detected
Changes in the frequency of white sharks detected, for both currently and previously tagged individuals, were significantly influenced by the number of days since the last scientific expedition ended and Expedition ID, but did not have any significant monthly or yearly variations (Table 2, Figs S5, S6). Changes in the proportion of currently tagged sharks were similarly related to the number of days elapsed since the last scientific expedition and Expedition ID, with non-significant seasonal and interannual effects (Table 2). Proportions of previously tagged sharks were mostly influenced by Expedition ID, followed by the number of days since the last scientific expeditions, with a minimal seasonal effect (Table 2). The proportions of both currently and previously tagged sharks gradually decreased during the 18 days following the end of a sampling event and became significantly lower after 26 days (Fig. 4).
Variations in the frequency of individuals sighted
Changes in the frequency of white sharks sighted at the Neptune Islands in the absence of scientific activities (Natural; Fig. S7) and following sampling events (Sampling; Fig. S8) varied significantly as a function of the interaction between number of days elapsed and Month (Table 3). This effect was consistent across Year but was affected by Event ID (Table 3). Changes in the frequency of sharks sighted following electric deterrent trial expeditions (Deterrent; Fig. S9) were also significantly influenced by the interaction between elapsed number of days and Month, which was the most influential variable (Table 3). The interannual variation was significant for this event type and explained more of the variations than Event ID (Table 3).
The number of white sharks sighted in the Neptune Islands following natural events and both types of scientific sampling had similar trends. Shark frequency was significantly higher during the first 8 days, particularly between January and June, in the absence of research activities (Fig. 5a) and regardless of whether sampling (Fig. 5b) or electric deterrent trial (Fig. 5c) took place. We observed an overall bimodal trend between July and December, with shark frequency decreasing during the first 5 days and increasing after ~15 days (Fig. 5).
Discussion
Scientists need to assess the impacts of their research activities on the animals they study, particularly when studying threatened or protected species or when sharing study sites with other industries and stakeholders that rely on consistent animal encounters, e.g. tourism or fishing industries. Our study shows that while the number of sharks detected with acoustic tracking and sighted by cage-diving operators decreased following research activities, it is unlikely to be a result of the research activities but might instead be inherent to natural variations in the number of sharks at the study site.
Data on species occurrence and abundance collected by tourism operators can provide valuable information on the effects of such activities on animal behaviour and show their seasonal and migratory cycles (Macolm et al. 2001; Theberge and Dearden 2006; Meyer et al. 2009; Legaspi et al. 2020). Acoustic tracking showed that, despite significant interannual and intra-individual variations, white shark residency at the Neptune Islands was consistent year-round. However, the cage-diving sighting data suggested that trends in the number of sharks varied between seasons. From January to June, the number of sharks sighted increased during the first 8 days following natural event, sampling, or deterrent testing. However, from July to December, shark frequency decreased during the first 5 days and increased after ~15 days. The reason for these opposite trends is unknown. The large influences of Expedition ID and Event ID highlight the high variability in the number of white sharks at the Neptune Islands, especially compared with the effect of months, which was much smaller. Seasonal variation has been documented at the Neptune Islands (Bruce and Bradford 2015; Nazimi et al. 2018) and other sites (Ryklief et al. 2014; Francis et al. 2015; Becerril-García et al. 2019), but the number of white sharks can also vary extensively within a month across expeditions or trips.
Although several studies have previously assessed the effects of tagging on fishes, many studies focus on surgically implanted tags (e.g. Klinard et al. 2018) or use captive fishes (e.g. Neves et al. 2018). Understanding the effects of tagging is further complicated by the various measures that can be used to assess impact (e.g. physical, physiological, behavioural, survival) and the different components of the tagging process that could be affecting tagged individuals, such as, for example, capture, handling, tagging, taking biopsies and transporting (Drenner et al. 2012; Reisinger et al. 2014; Jepsen et al. 2015). In our study, white sharks were not captured nor handled, with tagging and biopsying consisting of the tagging tip or biopsy probe penetrating the dorsal muscle for a fraction of a second, minimising shark discomfort as much as possible. However, such disturbance could still lead white sharks to leave the study site. If this occurred, the first residency period of sampled sharks would be expected to be shorter than subsequent visits (during which sharks are not tagged), but this was not observed. Instead, white shark residency declined following sampling and continued to decrease across subsequent visits. Although this could be associated to the effects of sampling, it is unexpected to see such a long-term effect (i.e. over several months and even years) from the deployment of an external acoustic tag, or taking a biopsy of ~1 cm in diameter. The consistent declines in residency throughout subsequent visits to the Neptune Islands suggest that other unknown factors might be affecting trends in white shark residency through time.
The comparison between the number of currently and previously tagged sharks also does not support that tagging affected white sharks and led them to prematurely leave the Neptune Islands. If tagging had an impact, a faster reduction in the number of currently tagged sharks than that of the group of previously tagged sharks would be expected. Instead, the number of currently and previously tagged sharks decreased at a similar rate. It is possible that although previously tagged sharks were not directly affected by the most recent research expeditions, these sharks might follow the currently tagged sharks and also left the Neptune Islands. Such process where ‘naïve’ individuals learn from watching ‘informed’ individuals, consequently altering behaviour, is referred to as social learning and has been reported in a variety of groups including mammals, birds, insects, teleosts, and sharks (Hoppitt and Laland 2008). For example, elasmobranchs will learn a task to access food rewards faster by observing trained individuals than do naïve individuals who did not have the opportunity to observe trained individuals (Guttridge et al. 2013; Thonhauser et al. 2013; Vila Pouca et al. 2020). Therefore, it is possible that sampling caused the departure of currently tagged sharks and that previously tagged sharks followed the currently tagged sharks away from the Neptune Islands through social learning.
The greatest evidence that our research activities did not cause sharks to leave the study site comes from our comparison of the number of sharks sighted following research activities versus natural fluctuations. Our analysis showed that similar reductions were observed in the number of white sharks sighted following periods of high abundance, regardless of whether research activity had taken place. Research activity typically targets periods of high shark abundance to maximise research opportunities. As a result of the operators reporting the daily number of sharks sighted (Nazimi et al. 2018), we can target research expeditions when shark abundance is highest and most likely to result in productive trips. Because we plan our research activities when shark abundance is high, shark numbers are unlikely to increase further or remain at that level for prolonged periods, and the most likely trend is for the number of sharks present at the Neptune Islands to decrease. The increase prior to the research activity and ensuing decrease was most pronounced in relation to the shark deterrent trial events. This was expected, because high shark abundance is particularly important when undertaking shark deterrent testing to maximise the chances of interactions with the deterrents and ensure testing across individuals of different motivation or personalities.
The use of food-based attractant by the cage-diving industry could have masked the effects of research activities by incentivising sharks to remain at the Neptune Islands. This is supported by a previous study showing higher residency linked to an increase in cage-diving activities (Bruce and Bradford 2013). However, regulations managing the activity of cage-diving operators have been in place since 2012, with a monitoring program suggesting a return to baseline residency (Huveneers and Niella 2022). In addition, white shark residency did not change during a 6-week hiatus in berleying and baiting because of COVID restrictions (Huveneers et al. 2021). Although it is difficult to quantify the effect of food-based attractant on white shark residency, research activity was not sufficient to reduce shark residency or lead to shark departure, regardless of whether these are affected by wildlife tourism activities.
Sharks have strong immune systems (Criscitiello 2014) and a remarkable ability to heal and regenerate from serious injuries such as gill destruction or body wall penetration (Towner et al. 2012; Chin et al. 2015; Kessel et al. 2017). They are also known to bite each other during mating, sometimes inflicting serious injuries (Pratt and Carrier 2001). Therefore, a ~15-cm-long tagging tip, or 7-cm-long biopsy tip, quickly penetrating a large shark (>3 m in total length) musculature is unlikely to be considered a serious injury, at least not sufficiently enough to affect shark behaviour. Tag deployment was also external (vs internal tagging) and tagging and taking biopsies was undertaken while sharks were free-swimming, without needing to catch and restrain individuals, further minimising handling and stress. In addition, external tagging with acoustic transmitters has been shown to have limited impacts on the welfare of sharks and rays (Speed et al. 2013). White sharks are also large-bodied, so a small acoustic transmitter (<0.0001% of the shark’s body weight) or a 2-g biopsy is unlikely to have an impact on them. Nevertheless, biofouling on the transmitters could create additional drag, especially in small species, and injuries at the tag insertion and biopsy site are potential sites for infection or inflammation that may consequently affect shark health or microbial communities (Heupel et al. 1998; Black et al. 2021; Perry et al. 2021). These potential effects were not investigated here. These animals are considerably resilient to anthropogenic-induced stressors; for example, capture of up to 75 min on SMART drumlines, followed by acoustic tagging and muscle biopsies and blood draws does not lead to physiological impairment or mortality (Gallagher et al. 2019; Tate et al. 2019). With regards to the deterrent trials, the devices tested have either had no effects (Huveneers et al. 2018b) or have a very small range of less than 2 m (Gauthier et al. 2020; Thiele et al. 2020). Therefore, we would not have expected sampling or deterrent testing to lead white sharks to leave the Neptune Islands. However, concerns from the cage-diving industry and ethical considerations to minimise the impacts of scientific research on animals warrant the need for our study, and the continued investigation of ways to further minimise the impacts of scientific investigations on animal welfare and health (Wilson and Mcmahon 2006; Soulsbury et al. 2020). Although our findings suggesting that research activities are minimally invasive could be extrapolated to other large-bodied sharks, physiological and behavioural response to disturbance and stress varies across species and should be considered to ensure best practice in animal welfare.
Supplementary material
Supplementary material is available online.
Data availability
The white shark acoustic-tracking data are available on the Integrated Marine Observing System Animal Tracking Facility online database (https://animaltracking.aodn.org.au/).
Conflicts of interest
The authors declare no conflicts of interest. Charlie Huveneers is an Associate Editor of Wildlife Research but was blinded from the peer-review process for this paper.
Declaration of funding
This project was funded by the Department for Environment and Water, Flinders University, and the Neiser Foundation.
Acknowledgements
The authors thank Adventure Bay Charters, Calypso Star, and Rodney Fox Shark Expeditions for providing logbook data and logistical support during the deployment of acoustic tags and biopsies and the deployment and servicing of acoustic receivers. We also thank the following people for helping with the fieldwork during this study: Thomas Clarke, Joshua Dennis, Sasha Whitmarsh, Chloe Roberts, Joshua Davey, Christine Barry, Adrienne Gooden, Taryn-Lee Perrior, Will Mingorance, Leila Nazimi, Adam Schilds, James van den Broek, Phil McDowall, and staff of the cage-diving industry. Data were sourced from Australia’s Integrated Marine Observing System (IMOS); IMOS is enabled by the National Collaborative Research Infrastructure Strategy (NCRIS).
References
Beauchamp TL, DeGrazia D (2019) ‘Principles of animal research ethics.’ (Oxford University Press)Becerril-García, EE, Hoyos-Padilla, EM, Micarelli, P, Galván-Magaña, F, and Sperone, E (2019). The surface behaviour of white sharks during ecotourism: a baseline for monitoring this threatened species around Guadalupe Island, Mexico. Aquatic Conservation: Marine and Freshwater Ecosystems 29, 773–782.
| The surface behaviour of white sharks during ecotourism: a baseline for monitoring this threatened species around Guadalupe Island, Mexico.Crossref | GoogleScholarGoogle Scholar |
Black, C, Merly, L, and Hammerschlag, N (2021). Bacterial communities in multiple tissues across the body surface of three coastal shark species. Zoological Studies 60, e69.
| Bacterial communities in multiple tissues across the body surface of three coastal shark species.Crossref | GoogleScholarGoogle Scholar |
Bruce, B, and Bradford, R (2015). Segregation or aggregation? Sex-specific patterns in the seasonal occurrence of white sharks Carcharodon carcharias at the Neptune Islands, South Australia. Journal of Fish Biology 87, 1355–1370.
| Segregation or aggregation? Sex-specific patterns in the seasonal occurrence of white sharks Carcharodon carcharias at the Neptune Islands, South Australia.Crossref | GoogleScholarGoogle Scholar |
Bruce, BD, and Bradford, RW (2013). The effects of shark cage-diving operations on the behaviour and movements of white sharks, Carcharodon carcharias, at the Neptune Islands, South Australia. Marine Biology 160, 889–907.
| The effects of shark cage-diving operations on the behaviour and movements of white sharks, Carcharodon carcharias, at the Neptune Islands, South Australia.Crossref | GoogleScholarGoogle Scholar |
Butcher, PA, Piddocke, TP, Colefax, AP, Hoade, B, Peddemors, VM, Borg, L, and Cullis, BR (2019). Beach safety: can drones provide a platform for sighting sharks? Wildlife Research 46, 701–712.
| Beach safety: can drones provide a platform for sighting sharks?Crossref | GoogleScholarGoogle Scholar |
Chin, A, Mourier, J, and Rummer, JL (2015). Blacktip reef sharks (Carcharhinus melanopterus) show high capacity for wound healing and recovery following injury. Conservation Physiology 3, cov062.
| Blacktip reef sharks (Carcharhinus melanopterus) show high capacity for wound healing and recovery following injury.Crossref | GoogleScholarGoogle Scholar |
Criscitiello, MF (2014). What the shark immune system can and cannot provide for the expanding design landscape of immunotherapy. Expert Opinion on Drug Discovery 9, 725–739.
| What the shark immune system can and cannot provide for the expanding design landscape of immunotherapy.Crossref | GoogleScholarGoogle Scholar |
Crozier, GKD, and Schulte-Hostedde, AI (2015). Towards improving the ethics of ecological research. Science and Engineering Ethics 21, 577–594.
| Towards improving the ethics of ecological research.Crossref | GoogleScholarGoogle Scholar |
Curtis TH, Bruce BD, Cliff G, Dudley SFJ, Klimley AP, Kock A, Lea RN, Lowe CG, McCosker JE, Skomal GB, Werry JM, West JG (2012) Responding to the risk of white whark attack. Updated statistics, prevention, control Methods, and recommendations. In ‘Global perspectives on the biology and life history of the white shark’. (Ed. ML Domeier) pp. 477–510. (CRC Press)
Dawkins, MS (2006). A user’s guide to animal welfare science. Trends in Ecology & Evolution 21, 77–82.
| A user’s guide to animal welfare science.Crossref | GoogleScholarGoogle Scholar |
DeGrazia, D, and Beauchamp, TL (2019). Beyond the 3 Rs to a more comprehensive framework of principles for animal research ethics. ILAR Journal 60, 308–317.
| Beyond the 3 Rs to a more comprehensive framework of principles for animal research ethics.Crossref | GoogleScholarGoogle Scholar |
Department of Sustainability Environment Water Population and Communities (2013) ‘Recovery plan for the white shark (Carcharodon carcharias). (Department of Sustainability Environment Water Population and Communities)
Drenner, SM, Clark, TD, Whitney, CK, Martins, EG, Cooke, SJ, and Hinch, SG (2012). A synthesis of tagging studies examining the behaviour and survival of anadromous salmonids in marine environments. PLoS ONE 7, e31311.
| A synthesis of tagging studies examining the behaviour and survival of anadromous salmonids in marine environments.Crossref | GoogleScholarGoogle Scholar |
Fenwick, N, Griffin, G, and Gauthier, C (2009). The welfare of animals used in science: how the ‘Three Rs’ ethic guides improvements. The Canadian Veterinary Journal 50, 523–530.
Francis, MP, Duffy, C, and Lyon, W (2015). Spatial and temporal habitat use by white sharks (Carcharodon carcharias) at an aggregation site in southern New Zealand. Marine and Freshwater Research 66, 900–918.
| Spatial and temporal habitat use by white sharks (Carcharodon carcharias) at an aggregation site in southern New Zealand.Crossref | GoogleScholarGoogle Scholar |
Gallagher, AJ, Meyer, L, Pethybridge, HR, Huveneers, C, and Butcher, PA (2019). Effects of short-term capture on the physiology of white sharks Carcharodon carcharias: amino acids and fatty acids. Endangered Species Research 40, 297–308.
| Effects of short-term capture on the physiology of white sharks Carcharodon carcharias: amino acids and fatty acids.Crossref | GoogleScholarGoogle Scholar |
Gauthier, ARG, Chateauminois, E, Hoarau, MG, Gadenne, J, Hoarau, E, Jaquemet, S, Whitmarsh, SK, and Huveneers, C (2020). Variable response to electric shark deterrents in bull sharks, Carcharhinus leucas. Scientific Reports 10, 17869.
| Variable response to electric shark deterrents in bull sharks, Carcharhinus leucas.Crossref | GoogleScholarGoogle Scholar |
Gorkin, R, Adams, K, Berryman, MJ, Aubin, S, Li, W, Davis, AR, and Barthelemy, J (2020). Sharkeye: real-time autonomous personal shark alerting via aerial surveillance. Drones 4, 18.
| Sharkeye: real-time autonomous personal shark alerting via aerial surveillance.Crossref | GoogleScholarGoogle Scholar |
Guttridge, TL, van Dijk, S, Stamhuis, EJ, Krause, J, Gruber, SH, and Brown, C (2013). Social learning in juvenile lemon sharks, Negaprion brevirostris. Animal Cognition 16, 55–64.
| Social learning in juvenile lemon sharks, Negaprion brevirostris.Crossref | GoogleScholarGoogle Scholar |
Heupel, MR, Simpfendorfer, CA, and Bennett, MB (1998). Analysis of tissue responses to fin tagging in Australian carcharhinids. Journal of Fish Biology 52, 610–620.
| Analysis of tissue responses to fin tagging in Australian carcharhinids.Crossref | GoogleScholarGoogle Scholar |
Hoppitt, W, and Laland, KN (2008). Social processes influencing learning in animals: a review of the evidence. Advances in the Study of Behavior 38, 105–165.
| Social processes influencing learning in animals: a review of the evidence.Crossref | GoogleScholarGoogle Scholar |
Huveneers C, Niella Y (2022) Residency of white sharks, Carcharodon carcharias, at the Neptune Islands Group Marine Park (2021-2022). Report to the Department of the Environment, Water and Natural Resources.
Huveneers, C, Rogers, PJ, Beckmann, C, Semmens, JM, Bruce, BD, and Seuront, L (2013a). The effects of cage-diving activities on the fine-scale swimming behaviour and space use of white sharks. Marine Biology 160, 2863–2875.
| The effects of cage-diving activities on the fine-scale swimming behaviour and space use of white sharks.Crossref | GoogleScholarGoogle Scholar |
Huveneers, C, Rogers, PJ, Semmens, JM, Beckmann, C, Kock, AA, Page, B, and Goldsworthy, SD (2013b). Effects of an electric field on white sharks: in situ testing of an electric deterrent. PLoS ONE 8, e62730.
| Effects of an electric field on white sharks: in situ testing of an electric deterrent.Crossref | GoogleScholarGoogle Scholar |
Huveneers, C, Holman, D, Robbins, R, Fox, A, Endler, JA, and Taylor, AH (2015). White sharks exploit the sun during predatory approaches. The American Naturalist 185, 562–570.
| White sharks exploit the sun during predatory approaches.Crossref | GoogleScholarGoogle Scholar |
Huveneers, C, Watanabe, YY, Payne, NL, and Semmens, JM (2018a). Interacting with wildlife tourism increases activity of white sharks. Conservation Physiology 6, coy019.
| Interacting with wildlife tourism increases activity of white sharks.Crossref | GoogleScholarGoogle Scholar |
Huveneers, C, Whitmarsh, S, Thiele, M, Meyer, L, Fox, A, and Bradshaw, CJA (2018b). Effectiveness of five personal shark-bite deterrents for surfers. PeerJ 6, e5554.
| Effectiveness of five personal shark-bite deterrents for surfers.Crossref | GoogleScholarGoogle Scholar |
Huveneers, C, Jaine, FRA, Barnett, A, et al. (2021). The power of national acoustic tracking networks to assess the impacts of human activity on marine organisms during the COVID-19 pandemic. Biological Conservation 256, 108995.
| The power of national acoustic tracking networks to assess the impacts of human activity on marine organisms during the COVID-19 pandemic.Crossref | GoogleScholarGoogle Scholar |
Jepsen, N, Thorstad, EB, Havn, T, and Lucas, MC (2015). The use of external electronic tags on fish: an evaluation of tag retention and tagging effects. Animal Biotelemetry 3, 49.
| The use of external electronic tags on fish: an evaluation of tag retention and tagging effects.Crossref | GoogleScholarGoogle Scholar |
Kempster, RM, Egeberg, CA, Hart, NS, Ryan, L, Chapuis, L, Kerr, CC, Schmidt, C, Huveneers, C, Gennari, E, Yopak, KE, Meeuwig, JJ, and Collin, SP (2016). How close is too close? The effect of a non-lethal electric shark deterrent on white shark behaviour. PLoS ONE 11, e0157717.
| How close is too close? The effect of a non-lethal electric shark deterrent on white shark behaviour.Crossref | GoogleScholarGoogle Scholar |
Kessel, ST, Fraser, J, van Bonn, WG, Brooks, JL, Guttridge, TL, Hussey, NE, and Gruber, SH (2017). Transcoelomic expulsion of an ingested foreign object by a carcharhinid shark. Marine and Freshwater Research 68, 2173–2177.
| Transcoelomic expulsion of an ingested foreign object by a carcharhinid shark.Crossref | GoogleScholarGoogle Scholar |
Klinard, NV, Halfyard, EA, Fisk, AT, Stewart, TJ, and Johnson, TB (2018). Effects of surgically implanted acoustic tags on body condition, growth, and survival in a small, laterally compressed forage fish. Transactions of the American Fisheries Society 147, 749–757.
| Effects of surgically implanted acoustic tags on body condition, growth, and survival in a small, laterally compressed forage fish.Crossref | GoogleScholarGoogle Scholar |
Krogh, M, and Reid, D (1996). Bycatch in the protective shark meshing programme off south-eastern New South Wales, Australia. Biological Conservation 77, 219–226.
| Bycatch in the protective shark meshing programme off south-eastern New South Wales, Australia.Crossref | GoogleScholarGoogle Scholar |
Legaspi, C, Miranda, J, Labaja, J, Snow, S, Ponzo, A, and Araujo, G (2020). In-water observations highlight the effects of provisioning on whale shark behaviour at the world’s largest whale shark tourism destination. Royal Society Open Science 7, 200392.
| In-water observations highlight the effects of provisioning on whale shark behaviour at the world’s largest whale shark tourism destination.Crossref | GoogleScholarGoogle Scholar |
Macolm H, Bruce BD, Stevens JD (2001) ‘A review of the biology and status of white shark in Australian waters.’ (CSIRO Marine Research)
Meeuwig, JJ, and Ferreira, LC (2014). Moving beyond lethal programs for shark hazard mitigation. Animal Conservation 17, 297–298.
| Moving beyond lethal programs for shark hazard mitigation.Crossref | GoogleScholarGoogle Scholar |
Meyer, CG, Dale, JJ, Papastamatiou, YP, Whitney, NM, and Holland, KN (2009). Seasonal cycles and long-term trends in abundance and species composition of sharks associated with cage diving ecotourism activities in Hawaii. Environmental Conservation 36, 104–111.
| Seasonal cycles and long-term trends in abundance and species composition of sharks associated with cage diving ecotourism activities in Hawaii.Crossref | GoogleScholarGoogle Scholar |
Meyer, L, Fox, A, and Huveneers, C (2018). Simple biopsy modification to collect muscle samples from free-swimming sharks. Biological Conservation 228, 142–147.
| Simple biopsy modification to collect muscle samples from free-swimming sharks.Crossref | GoogleScholarGoogle Scholar |
Meyer, L, Pethybridge, H, Beckmann, C, Bruce, B, and Huveneers, C (2019). The impact of wildlife tourism on the foraging ecology and nutritional condition of an apex predator. Tourism Management 75, 206–215.
| The impact of wildlife tourism on the foraging ecology and nutritional condition of an apex predator.Crossref | GoogleScholarGoogle Scholar |
Meyer, L, Apps, K, Bryars, S, Clarke, T, Hayden, B, Pelton, G, Simes, B, Vaughan, LM, Whitmarsh, SK, and Huveneers, C (2021). A multidisciplinary framework to assess the sustainability and acceptability of wildlife tourism operations. Conservation Letters 14, e12788.
| A multidisciplinary framework to assess the sustainability and acceptability of wildlife tourism operations.Crossref | GoogleScholarGoogle Scholar |
Meyer, L, Barry, C, Araujo, G, Barnett, A, Brunnschweiler, JM, Chin, A, Gallagher, A, Healy, T, Kock, A, Newsome, D, Ponzo, A, and Huveneers, C (2022). Redefining provisioning in marine wildlife tourism. Journal of Ecotourism 21, 210–229.
| Redefining provisioning in marine wildlife tourism.Crossref | GoogleScholarGoogle Scholar |
Nazimi, L, Robbins, WD, Schilds, A, and Huveneers, C (2018). Comparison of industry-based data to monitor white shark cage-dive tourism. Tourism Management 66, 263–273.
| Comparison of industry-based data to monitor white shark cage-dive tourism.Crossref | GoogleScholarGoogle Scholar |
Neves, V, Silva, D, Martinho, F, Antunes, C, Ramos, S, and Freitas, V (2018). Assessing the effects of internal and external acoustic tagging methods on European flounder Platichthys flesus. Fisheries Research 206, 202–208.
| Assessing the effects of internal and external acoustic tagging methods on European flounder Platichthys flesus.Crossref | GoogleScholarGoogle Scholar |
Palmer, A, and Greenhough, B (2021). Out of the laboratory, into the field: perspectives on social, ethical and regulatory challenges in UK wildlife research. Philosophical Transactions of the Royal Society B: Biological Sciences 376, 20200226.
| Out of the laboratory, into the field: perspectives on social, ethical and regulatory challenges in UK wildlife research.Crossref | GoogleScholarGoogle Scholar |
Perry, CT, Pratte, ZA, Clavere-Graciette, A, Ritchie, KB, Hueter, RE, Newton, AL, Fischer, GC, Dinsdale, EA, Doane, MP, Wilkinson, KA, Bassos-Hull, K, Lyons, K, Dove, ADM, Hoopes, LA, and Stewart, FJ (2021). Elasmobranch microbiomes: emerging patterns and implications for host health and ecology. Animal Microbiome 3, 61.
| Elasmobranch microbiomes: emerging patterns and implications for host health and ecology.Crossref | GoogleScholarGoogle Scholar |
Pratt, H, and Carrier, J (2001). A review of elasmobranch reproductive behaviour with a case study on the nurse shark, Ginglymostoma cirratum. Environmental Biology of Fishes 60, 157–188.
| A review of elasmobranch reproductive behaviour with a case study on the nurse shark, Ginglymostoma cirratum.Crossref | GoogleScholarGoogle Scholar |
Reisinger, RR, Oosthuizen, WC, Péron, G, Cory Toussaint, D, Andrews, RD, and de Bruyn, PJN (2014). Satellite tagging and biopsy sampling of killer whales at subantarctic Marion Island: effectiveness, immediate reactions and long-term responses. PLoS ONE 9, e111835.
| Satellite tagging and biopsy sampling of killer whales at subantarctic Marion Island: effectiveness, immediate reactions and long-term responses.Crossref | GoogleScholarGoogle Scholar |
Rigby CL, Barreto R, Carlson J, Fernando D, Fordham S, Francis MP, Herman K, Jabado RW, Liu KM, Lowe CG, Marshall A, Pacoureau N, Romanov E, Sherley RB, Winker H (2019) Carcharodon carcharias. The IUCN Red List of Threatened Species, e.T3855A2878674. Accessed on 17 October 2022 https://doi.org/
| Crossref |
Russell WMS, Burch RL (1959) ‘The principles of humane experimental technique.’ (Universities Federation for Animal Welfare)
Ryklief, R, Pistorius, PA, and Johnson, R (2014). Spatial and seasonal patterns in sighting rate and life-history composition of the white shark Carcharodon carcharias at Mossel Bay, South Africa. African Journal of Marine Science 36, 449–453.
| Spatial and seasonal patterns in sighting rate and life-history composition of the white shark Carcharodon carcharias at Mossel Bay, South Africa.Crossref | GoogleScholarGoogle Scholar |
Schilds, A, Mourier, J, Huveneers, C, Nazimi, L, Fox, A, and Leu, ST (2019). Evidence for non-random co-occurrences in a white shark aggregation. Behavioral Ecology and Sociobiology 73, 138.
| Evidence for non-random co-occurrences in a white shark aggregation.Crossref | GoogleScholarGoogle Scholar |
Simmons, P, and Mehmet, MI (2018). Shark management strategy policy considerations: community preferences, reasoning and speculations. Marine Policy 96, 111–119.
| Shark management strategy policy considerations: community preferences, reasoning and speculations.Crossref | GoogleScholarGoogle Scholar |
Soulsbury, CD, Gray, HE, Smith, LM, Braithwaite, V, Cotter, SC, Elwood, RW, Wilkinson, A, and Collins, LM (2020). The welfare and ethics of research involving wild animals: a primer. Methods in Ecology and Evolution 11, 1164–1181.
| The welfare and ethics of research involving wild animals: a primer.Crossref | GoogleScholarGoogle Scholar |
Speed, CW, O’Shea, OR, and Meekan, MG (2013). Transmitter attachment and release methods for short-term shark and stingray tracking on coral reefs. Marine Biology 160, 1041–1050.
| Transmitter attachment and release methods for short-term shark and stingray tracking on coral reefs.Crossref | GoogleScholarGoogle Scholar |
Tate, RD, Cullis, BR, Smith, SDA, Kelaher, BP, Brand, CP, Gallen, CR, Mandelman, JW, and Butcher, PA (2019). Corrigendum to: The acute physiological status of white sharks (Carcharodon carcharias) exhibits minimal variation after capture on SMART drumlines. Conservation Physiology 7, coz090.
| Corrigendum to: The acute physiological status of white sharks (Carcharodon carcharias) exhibits minimal variation after capture on SMART drumlines.Crossref | GoogleScholarGoogle Scholar |
Theberge, MM, and Dearden, P (2006). Detecting a decline in whale shark Rhincodon typus sightings in the Andaman Sea, Thailand, using ecotourist operator-collected data. Oryx 40, 337–342.
| Detecting a decline in whale shark Rhincodon typus sightings in the Andaman Sea, Thailand, using ecotourist operator-collected data.Crossref | GoogleScholarGoogle Scholar |
Thiele, M, Mourier, J, Papastamatiou, Y, Ballesta, L, Chateauminois, E, and Huveneers, C (2020). Response of blacktip reef sharks Carcharhinus melanopterus to shark bite mitigation products. Scientific Reports 10, 3563.
| Response of blacktip reef sharks Carcharhinus melanopterus to shark bite mitigation products.Crossref | GoogleScholarGoogle Scholar |
Thonhauser, KE, Gutnick, T, Byrne, RA, Kral, K, Burghardt, GM, and Kuba, MJ (2013). Social learning in Cartilaginous fish (stingrays Potamotrygon falkneri). Animal Cognition 16, 927–932.
| Social learning in Cartilaginous fish (stingrays Potamotrygon falkneri).Crossref | GoogleScholarGoogle Scholar |
Towner A, Smale MJ, Jewell O (2012) Boat strike wound healing in Carcharodon carcharias. In ‘Global perspectives on the biology and life history of the white shark’. (Ed. ML Domeier) pp. 77–84. (CRC Press)
Vila Pouca, C, Heinrich, D, Huveneers, C, and Brown, C (2020). Social learning in solitary juvenile sharks. Animal Behaviour 159, 21–27.
| Social learning in solitary juvenile sharks.Crossref | GoogleScholarGoogle Scholar |
Wilson, RP, and Mcmahon, CR (2006). Measuring devices on wild animals: what constitutes acceptable practice? Frontiers in Ecology and the Environment 4, 147–154.
| Measuring devices on wild animals: what constitutes acceptable practice?Crossref | GoogleScholarGoogle Scholar |
Wood SN (2017) ‘Generalized additive models: an introduction with R.’ 2nd edn. (Chapman and Hall/CRC)