Estimates of abundance and apparent survival of coastal dolphins in Port Essington harbour, Northern Territory, Australia
Carol Palmer A B H , Lyndon Brooks C , Guido J. Parra D E , Tracey Rogers F , Debra Glasgow G and John C. Z. Woinarski BA Marine Ecosystems, Department of Land Resource Management, PO Box 496, Palmerston NT, 0831, Australia.
B Research Institute for the Environment and Livelihoods, Charles Darwin University, NT 0909, Australia.
C Southern Cross University, Marine Ecology Research Centre, Southern Cross University, Lismore, NSW 2480, Australia.
D Cetacean Ecology, Behaviour and Evolution Lab, School of Biological Sciences, Flinders University, GPO Box 2100, Adelaide, SA 5001, Australia.
E South Australian Research and Development Institute (Aquatic Sciences) PO Box 120, Henley Beach, SA 5022, Australia.
F Evolution & Ecology Research Centre, School of Biological, Earth & Environmental Sciences, University of New South Wales, Sydney, NSW 2052, Australia.
G PO Box 98, Paekakariki 5034, New Zealand.
H Corresponding author. Email: carolL.palmer@nt.gov.au
Submitted: 7 August 2013 Accepted: 18 February 2014 Published: 7 May 2014
Abstract
Context: Three dolphin species occur in coastal waters of monsoonal northern Australia: the Australian snubfin (Orcaella heinsohni), humpback (Sousa sp.) and the bottlenose (Tursiops sp.). Their overall population size and trends are poorly known, and their conservation status has been difficult to resolve, but can be expected to deteriorate with likely increased development pressures.
Aims: We sought to provide an estimate of abundance, and apparent survival, of the three dolphin species at the largely undeveloped harbour of Port Essington (325 km2), Northern Territory, with repeated sampling over a 2.9-year period. Given increasing obligations to undertake population assessments for impact studies at proposed development sites, we assess the strengths and limitations of a systematic sampling program.
Methods: We used photo-identification data collected during systematic boat-based transect surveys undertaken from 2008 to 2010 and Pollock’s robust capture–recapture design model.
Key results: Total abundance estimates for the three species were variable across different sampling periods. The estimated number of individuals in the sampled area varied per sampling episode from 136 (s.e. 62) to 222 (s.e. 48) for snubfin, from 48 (s.e. 7) to 207 (s.e. 14) for humpbacks and from 34 (s.e. 6) to 75 (s.e. 9) for bottlenose dolphins. Apparent survival was estimated for snubfin at 0.81 (s.e. 0.11), humpbacks at 0.59 (s.e. 0.12) and bottlenose at 0.51 (s.e. 0.17) per annum.
Key conclusions: (1) The values derived here provide some of the only estimates of local population size for these species across monsoonal northern Australia; (2) population-size estimates varied considerably among seasons or sampling episodes; (3) the low apparent survival probabilities indicated that many individuals may move at scales larger than the study area; (4) density of snubfin and humpback dolphins in the present study area exceeded the few other estimates available for these species elsewhere in Australia.
Implications: The present study provided the first baseline estimates of abundance and apparent survival for three coastal dolphin species in monsoonal northern Australia. Such information is becoming increasingly important as development pressures intensify in coastal areas. Sampling protocols for future monitoring and impact assessment need an enhanced consideration of seasonality and scale issues.
Additional keywords: Australian snubfin, bottlenose, capture–recapture, Indo-Pacific humpback, Orcaella heinsohni, photo identification, robust design, Sousa chinensis, Tursiops sp.
Introduction
Coastal and river dolphins are threatened by a range of human activities (Borobia et al. 1991; Thompson et al. 2000; Kreb and Budiono 2005; Cantor et al. 2012), and several species (i.e. vaquita, Phocoena sinus; Hector’s dolphin, Cephalorhynchus hectori) are now among the world’s most threatened mammals (Rojas-Bracho et al. 2006; Reeves et al. 2013). By global standards, the coastal waters of Australia are relatively undisturbed (Halpern et al. 2008; Edyvane and Dethmers 2010). There are now increasing prospects and proposals for development in coastal waters of northern Australia, and, consequently, there are concerns about the conservation of the following three species of coastal dolphins occurring in these waters (Parra et al. 2006; Allen et al. 2012; Bejder et al. 2012; Cagnazzi et al. 2013; Palmer et al. 2014): the Australian snubfin, Orcaella heinsohni (hereafter snubfin), a new as-yet-unnamed species of humpback dolphin, Sousa sp. (Frère et al. 2011; Mendez et al. 2013), and the bottlenose dolphins, Tursiops sp. (hereafter bottlenose). Tursiops taxonomy is unresolved and at present T. truncatus (Montagu, 1821) and T. aduncus (Ehrenberg, 1833) are recognised in the Indo-Pacific region (Rice 1998; Kemper 2004). In Australian waters, until recently, all Tursiops were recorded as T. truncatus (Ross and Cockcroft 1990; Charlton-Robb et al. 2011); however, T. aduncus is now known to occur in estuarine and coastal waters of eastern Australia (Möller and Beheregaray 2001; Fury and Harrison 2008). In Western Australia, both aduncus- and truncatus-type haplotypes are present in coastal waters (Krützen et al. 2004). In the present study, we treat all records of Tursiops as T. sp.
Populations for snubfin and humpback dolphins are thought to be small, and some subpopulations may be geographically and genetically isolated (Cagnazzi 2010); however, there have been very few quantitative estimates of population size in any areas across their range in northern Australia (Corkeron et al. 1997; Parra et al. 2006; Fury and Harrison 2008; Cagnazzi et al. 2011, 2013). In contrast, bottlenose dolphins including both T. aduncus and T. sp. appear to be relatively abundant in subtropical and temperate Australian waters (Table 1).
In the Northern Territory (and across monsoonal northern Australia), baseline population estimates for coastal dolphins are lacking, and the conservation status of all three species is unknown (Parra et al. 2002, 2004; Palmer et al. 2014). The lack of sufficient baseline information on the status of coastal dolphin populations potentially hinders adequate environmental impact assessment, such that pre-impact assessment is likely to be based on very sparse opportunistic sighting data (Bejder et al. 2012; Palmer et al. 2014).
Assessment of the conservation status of these species at a state and national level requires the development and implementation of an appropriate systematic survey design and appropriate models for population size, survival and other demographic parameters. Assessing the population dynamics of free-ranging cetaceans can be difficult, time consuming and expensive (Taylor and Gerrodette 1993), particularly for species such as the snubfin and humpback dolphins, which are boat shy and have unpredictable surfacing patterns (Parra and Arnold 2008; Parra and Ross 2009; Palmer et al. 2011). Furthermore, they may have very low detectability in surveys of turbid in-shore waters and these conditions characterise much of their range (Dhandapani 1992; Parra et al. 2002, 2006; Parra 2006; Palmer et al. 2011).
Here, we use and evaluate a systematic sampling protocol that aims to estimate abundance at one site. Capture–recapture methods are commonly used to estimate demographic parameters for several dolphin species, including snubfin, humpback and bottlenose dolphins (Würsig and Jefferson 1990; Parra et al. 2006; Nicholson et al. 2012). Many cetaceans bear nicks and marks that allow identification of individuals from photographs, and such identifiers provide a mechanism for population estimation based on capture–recapture methods, where resightings of distinctively marked individuals constitute recaptures (Hammond and Thompson 1991).
There are two general types of models (closed- and open-population models) used in capture–recapture studies, to calculate abundance estimates over multiple sampling periods (Pollock et al. 1990; Williams et al. 2002). Closed-population models assume that the population remains unchanged for the duration of the study (i.e. no gains through births or immigration, nor losses through deaths or emigration). Closed-populations models are applied to short-term studies and can accommodate variation in capture probabilities by sampling occasion (time), individual animal response (heterogeneity) and behavioural response to first capture (‘behaviour’ – ‘trap happy’ and ‘trap shy’ responses) (Otis et al. 1978). Open-population models allow for demographic changes in the population over time, including gains (births, immigration) and losses (mortality, emigration) (Pollock et al. 1990; Williams et al. 2002). Such models can be used to estimate abundance at each sampling occasion, and the probability of apparent survival (alive and remaining in the sampling area) (Lebreton et al. 1992) and apparent births (born or immigrated) between sampling occasions (Jolly 1965; Seber 1965; Crosbie and Manly 1985; Schwarz and Arnason 1996). Open models cannot accommodate variation in capture probabilities, except by time, and may produce biased estimates in the presence of individual heterogeneity (downward bias) or behavioural response to first capture (trap happy = downward bias; trap shy = upward bias) (Pollock et al. 1990; Williams et al. 2002).
Pollock (1982) proposed a sampling regime (the robust design) of primary samples separated by time scales that would allow gains and losses from the population, with each primary sample composed of a set of sufficiently closely spaced secondary samples for population closure to be assumed. The combination of both open- and closed-population models within the robust design allows abundance to be estimated for each primary sampling period and apparent survival to be estimated between primary sampling periods. Kendall et al. (1995, 1997) and Kendall and Nichols (1995) further developed the robust design model and incorporated estimation of temporary emigration between primary samples. This is an advance on standard open-population models in which all immigration and emigration are assumed permanent. Examples of robust-design studies on coastal dolphins include Balmer et al. (2008), Rosel et al. (2011) and Smith et al. (2013).
Here, we use photo-identification data collected in Port Essington harbour, in the Northern Territory, from 2008–2010 and Pollock’s robust capture–recapture design model to provide baseline estimates of abundance and apparent survival of three species of tropical coastal dolphins (snubfin, humpback and bottlenose). These estimates are the first robust population estimates for monsoonal northern Australia and provide a baseline for future comparisons, which will become increasingly important as development pressures intensify in this region. We evaluate the strengths and limitations of this protocol, with particular reference to the increasing need for population assessments for environmental impact studies, and with reference to the developing need (e.g. Beasley et al. 2012a, 2012b) for national programs for monitoring coastal dolphins.
Materials and methods
Study site
Port Essington harbour (11.23°S, 132.15°E) is located within the Garig Gunak Barlu National Park, ~220 km north-east of Darwin (Fig. 1) and is the only National Park in the Northern Territory that contains adjoining land and marine areas. Port Essington harbour comprises a semi-enclosed 325 km2 former river valley that was drowned during periods of sea-level rise and now forms a largely sheltered and deeply incised harbour. The harbour provides varied environments that are distinct from the open water areas of the adjacent Van Diemen’s Gulf and the Arafura Sea. There are no major creeks or rivers flowing into Port Essington harbour and, consequently, there is restricted freshwater input. Within the harbour, tides range between 2 and 2.5 m, and turbidity is relatively low compared with many other parts of the Northern Territory. Within the Marine Park and surrounding waters, there is a relative lack of commercial shipping, almost no onshore development and limited aquaculture.
![]() |
Field methods
Boat-based photo-identification surveys were conducted in Port Essington harbour from March 2008 to November 2010 (Fig. 2). Seasonal patterns of habitat use and dispersal are very poorly known for these species, so sampling was deliberately timed to attempt to encompass and represent the extent of seasonal variation (i.e. late wet season (March), mid-dry season (June), late dry season (September), and early wet and build-up (November). Surveys followed a pre-determined set of fixed transect lines (total distance = 45 km) parallel to the shore and ~300–1000 m from the shore, depending on tide state, but depth remained approximately the same (Fig. 2). Transects were located in areas with expected higher rates of sighting detectability, and were sited to focus particularly on areas least exposed to rough seas and, therefore, reasonable sea-state conditions.
Most studies have indicated that these animals occur mainly in shallow coastal waters (Parra 2006; Parra et al. 2006; Cagnazzi et al. 2013). Given the above and taking into consideration that these are highly mobile animals that are likely to visit most of the space at some time, our estimates should not include substantial biases as a result of sampling design. A survey consisted of completing all transects, or as many transects as possible, in one day when weather and sea-state conditions permitted (Beaufort Sea states <3; swell less than 1 m and in daylight hours). Four survey sessions were conducted each year in March, June, September and November (primary sampling periods) and in which six consecutive survey days (secondary sampling periods) of sampling were attempted but not always achieved, because of weather constraints per session (Table 2). Sampling across this range of seasonal conditions was also designed to provide some context for the typically limited sampling undertaken for development impact assessments (which may often include only a single sampling event at one time of year).
Surveys were conducted from a 5.5-m aluminium vessel with a 100-hp four-stroke outboard engine, with the boat driver and one or two observers. Surveys followed transect lines at 15–20 km h–1 until a dolphin or dolphin group was sighted. When dolphins were sighted, the observer(s) attempted to take good-quality images of every dolphin dorsal fin in the group. A dolphin group was defined as all dolphins within a 100-m radius of any other dolphin that was involved in similar behavioural activities (Irvine et al. 1981; Wells et al. 1987; Parra et al. 2006; Fury and Harrison 2008). Data for each group on species identification, location and time of day, group size and behaviour were recorded on a hand-held Personal Digital Assistant using Cybertracker software (www.cybertracker.org, verified 11 March 2014). Sampling could not reliably distinguish the sex of individuals, and hence this factor is not considered in subsequent analyses here.
Image and data analysis
Dolphins of each species were identified by the location and number of nicks and scars on their dorsal fins (Würsig and Jefferson 1990; Hammond and Thompson 1991; Parra and Corkeron 2001). Calves were excluded from analysis because they are generally unmarked. Pigmentation patterns were used as secondary characteristics to confirm identification for humpback dolphins. Individuals were photographed independently of their distinctiveness. All images were classified into three grades (excellent, good and poor) on the basis on focus, relative angle to the dolphin and contrast. Only images graded as excellent and good were used to identify animals, develop identification catalogues for each species and individual capture histories for population analysis (Würsig and Jefferson 1990; Parra et al. 2006).
Estimating marked proportion and total abundance
Not all individual dolphins have sufficiently distinctive marks to support unambiguous identification. To include the unmarked portions of the populations in our estimates, we estimated the proportion of distinctively marked dolphins in the population of each species (Wilson et al. 1999). The (distinctively) marked proportion (Mp) of each population was estimated by dividing the number of excellent- and good-quality photographs of marked dolphins (Pm) by the total number of excellent- and good-quality photographs (Pt), as follows:

The total abundance (Ntotal) of each population for any sampling period was then estimated by dividing the estimated abundance of marked dolphins () by the estimated marked proportion, as follows:

Log-normal confidence intervals for abundance estimates were calculated following Burnham et al. (1987), as follows:
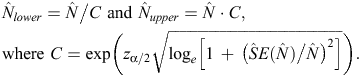
Statistical analysis
The robust-design model (closed-captures type) was fitted to the mark–recapture data to estimate abundance and survival parameters by using program MARK (V 6.1; White and Burnham 1999). The intervals between primary samples were specified in decimal years between their mid-dates to obtain consistent, per annum estimates of apparent survival. The relationships between the probabilities of apparent survival (φ), true (biological) survival (S), fidelity (F) and permanent emigration (E) may be expressed as

The parameters estimated by the robust design model (closed captures) are:
-
Sj apparent survival to primary sample j for j > 1,
-
δ″ probability of temporarily emigrating before sample j given presence at j–1 for j > 1,
-
δ′ probability of temporarily emigrating before sample j given absence at j–1 for j > 2,
-
pij probability of first capture in sample i of primary sample j for i ≥ 1,
-
cij probability of recapture in sample i of primary sample j for i > 1,
-
Nj population size during primary sample j.
These models may estimate temporary emigration as random or Markovian. When the two temporary emigration parameters, δ″ and δ′, are set equal at all times j, the temporary emigration estimated is random (the probability that an animal temporarily emigrates is independent of its state on the previous occasion); when δ″ = δ′, the temporary emigration estimated is Markovian (the probability that an animal temporarily emigrates is dependent on its state on the previous occasion; i.e. there is a temporally structured process underlying the movements (e.g. breeding; Kendall and Bjorkland 2001). When apparent survival is estimated as time varying, it is necessary to constrain each of the last δ″ and δ′ to equal their values at some earlier occasion to attain parameter identification in Markovian models. The number of parameters for each model was set at the number nominally estimated by the model independently of whether some were at the boundaries of possible values and not counted by MARK (see e.g. Jolly–Seber models in MARK, Schwarz and Arnason in White and Burnham 1999). Models were compared with the Akaike information criterion corrected for small sample sizes (AICc; Burnham and Anderson 2002), with smaller values of AICc indicating the better-fitting models, and with AICc weights, which measure the relative likelihoods of the models in the set.
Goodness-of-fit tests
There is no goodness-of-fit test for the robust-design model as a whole; however, separate tests for the open and closed parts of the model can be applied. Each set of secondary samples was tested for closure using CloseTest (Stanley and Burnham 1999) and the data were collapsed to primary samples and goodness of fit was tested in the Cormack–Jolly–Seber (CJS) framework using program RELEASE (as implemented in MARK (Cooch and White 2012) and by means of the median goodness-of-fit test (Cooch and White 2012).
Results
Survey effort
In total, 65 days of surveys were completed between March 2008 and November 2010, covering 2279 km on transect. During this period, 485 snubfin, 274 humpback and 165 bottlenose dolphins were recorded. Among these groups, 179 distinctively marked snubfin, 113 humpback and 53 bottlenose were identified in total. The proportion of marked individuals was high for each species (), namely, 0.61 ± 0.033 for snubfin, 0.61 ± 0.043 for humpback and 0.54 ± 0.058 for bottlenose. During the last primary sample for the project (November 2010), there was an influx of 26 marked snubfin and 15 humpback dolphins not previously photographed. Densities in Port Essington are higher than other reported values for snubfin and humpback and lower than in other reported studies for bottlenose (Table 1).
Data
Primary samples included in the robust-design model analysis were selected if they yielded reasonable estimates from closed models (Table 2). This was assessed by attempting to fit closed models to the within-session data and rejecting sessions that yielded estimates with unacceptably large standard errors. The resulting data included 124 snubfins captured 165 times over five primary samples, 74 humpbacks captured 102 times over eight primary samples and 38 bottlenose captured 52 times over six primary samples. The primary samples taken during September and November of each year rendered the most captures (Table 2).
Goodness-of-fit tests
CloseTest did not reject the assumption of closure for any primary sample of any species (P ≥ 0.05 in all cases). Goodness-of-fit tests from program RELEASE found no evidence of over-dispersion for snubfin (χ22 = 0.39, P = 0.821) and humpback (χ132 = 11.47, P = 0.571), whereas there were insufficient data for the test for bottlenose dolphins. Estimates of median, , were 0.99 for snubfin, 0.91 for humpback and 0.58 for bottlenose. No adjustments were made to
for the models for any species.
Robust-design analyses
Snubfin dolphins
Eight Pollock’s robust-design models were fitted to the snubfin capture–recapture data (Table 3). Preliminary analyses found that no useful parameter estimate could be obtained from models including heterogeneity (latent mixtures type), and that models with simplified temporal structures for capture probabilities fitted very poorly. Therefore, all models reported have capture probabilities varying by both primary and secondary sample, i.e. p(S*t), and none estimates heterogeneity (see Table 3 legend for symbols used to specify models).
The best-fitting (lowest AICc) model {phi(.) g (FIXED = 0) p(S*t) c(= p)N} with constant probability of apparent survival, no temporary emigration, fully time-varying capture probabilities and no behavioural response attracted 73% of the AICc weight. The resulting estimated abundance of marked snubfin from this model varied over the five primary samples between 82 (s.e. 37, 95% CI = 10–155) and 134 (s.e. 28, 95% CI = 80–189); and the total abundance varied over primary samples between 136 (s.e. 61, 95% CI = 58–317) and 222 (s.e. 48, 95% CI = 146–336) (Table 4). The average apparent survival probability was 0.80 (s.e. 0.11, 95% CI = 0.50–0.94) per annum.
Humpback dolphins
Five Pollock’s robust-design models were fitted to the humpback capture–recapture data (Table 3).The best-fitting model {phi(.) g(FIXED = 0) p(.) c(=p)N} with constant probability of apparent survival, no temporary emigration, constant capture probability and no behavioural response attracted 99% of the AICc weight. The estimated abundance of marked humpbacks varied across eight primary samples, between 29 (s.e. 10, 95% CI = 10–49) and 126 (s.e. 39, 95% CI = 49–202), and the estimated total abundance varied across primary samples between 48 (s.e. 7, 95% CI = 24–95) and 207 (s.e. 14, 95% CI = 113–379) (Table 4). The estimated average apparent annual survival probability was 0.59 (s.e. 0.12, 95% CI = 0.35–0.79).
Bottlenose dolphins
Nine Pollock’s robust-design models were fitted to the bottlenose capture–recapture data (Table 3). The best-fitting model {phi(.) g(FIXED = 0) p(.) c(= p)N} with constant probability of apparent survival, no temporary emigration, constant capture probability and no behavioural response attracted 99% of the AICc weight. The estimated abundance of marked bottlenose varied across six primary samples between 21 (s.e. 7, 95% CI = 7–35) and 41 (s.e.13, 95% CI = 15–66), and the estimated total abundance varied between 34 (s.e. 6, 95% CI = 14–83) and 75 (s.e. 9, 95% = CI 39–145) (Table 4). The estimated average apparent annual survival probability was 0.51 (s.e. = 0.17, 95% CI = 0.21–0.80).
Discussion
The present study has provided the first population estimates of three coastal dolphin species for a site in monsoonal northern Australia. The population estimates derived here indicated that ~136–222 snubfin, 48–207 humpback and 34–75 bottlenose dolphins were using Port Essington harbour at different sampling periods. These population-size estimates are broadly comparable to local populations of snubfin and humpback dolphins from the few previous studies reported elsewhere in Australia, but the densities are somewhat higher than reported in those studies (Parra et al. 2006; Cagnazzi et al. 2011) (Table 1). The estimated population size and density for bottlenose dolphins in Port Essington were smaller than those from studies elsewhere in Australia (Connor et al. 2000; Möller et al. 2002; Chilvers and Corkeron 2003; Lukoschek and Chilvers 2008; Nicholson et al. 2012), except for the estuarine populations reported by Fury and Harrison (2008). The present study has reinforced that (1) overall, there are indeed few comparative studies, (2) the densities in Port Essington are higher than the very few other reported values for snubfin and humpback dolphins, but (3) lower than those from other reported studies for bottlenose (Table 1).
Some of the population estimates had relatively large standard errors and confidence intervals (particularly so for snubfin, but far less so for the other two species) and the population estimates showed substantial variation across sampling periods (particularly for humpback dolphins). In this situation, turbid waters and inconspicuous and boat-shy behaviours (particularly for the snubfin), and perhaps movements from the sampled area within a sampling period, may have led to substantial constraints on detectability and the precision of population estimation for any primary sampling period. More intensive and extensive sampling within primary sampling periods would be likely to narrow confidence intervals, albeit with substantial additional costs.
Variation in abundance estimates among sampling periods may reflect the actual movement patterns to and from the sampled area, temporal changes in behaviour, or temporal changes in detectability (e.g. through seasonally varying levels of turbidity). Although there was no marked temporal patterning in estimates of abundance for any of the three species, sampling in September and November was more likely to provide usable data than sampling in March and June (Table 2). We interpret this result to be related to seasonal variation in detectability, with this period providing optimal sea-state conditions (Beaufort <3).
Estimates of apparent annual survival were low, particularly for the humpback and bottlenose. This is likely to be due to the occurrence of some transient animals and movements of marked animals to and from the sampling area, with this area probably smaller than the annual home ranges of at least some of the study individuals.
In the present study, the relatively wide CIs, combined with other limitations of relatively small sample sizes, had an impact on the potential of the robust-design model to deal with heterogeneity, time-varying survival probabilities, and to provide an informative analysis of temporary emigration. Studies of dolphins often ignore the possibility that some members of the populations are wide-ranging and may emigrate temporarily from the study area (Silva et al. 2010). Study areas rarely encompass the whole range of the animals and, even though we could not model it here, estimates of temporary emigration are very useful in accounting for movements between the overall range of the population and the area under study.
Movements could be due to local-scale shifts in prey distribution (Irvine et al. 1981; Fazioli et al. 2006; Silva et al. 2010; Speakman et al. 2010), seasonality (Smith 2012) or phases in reproductive activity. Further investigations into individual movement patterns by the three species are required and might be addressed by multi-state models (Williams et al. 2002; Nicholson et al. 2012), whereby data are collected simultaneously in several close-by study sites. However, a useful first step would be to increase capture probabilities within the harbour to a point where temporary emigration and its seasonal pattern could be modelled.
Application of the robust-design model in the present study has highlighted the value of estimating heterogeneity and temporary emigration for these wide-ranging species that occur in small populations, although data limitations in the study did not allow their estimation. Our inability to estimate heterogeneity would have biased our abundance estimates low if it were present; however, there is no strong a-priori reason to expect it; and our inability to estimate temporary emigration means that we were not able to provide an informative description of the processes that may underlie movements into and out of the harbour, although it is clear that such movements occur. If there were movement into and out of the sampling area within primary samples, and if this were random, then the abundance estimates are for the entire population using the sampling area during the primary sample and not just those that were consistently present throughout it (Kendall et al. 1997).
Although the population estimates derived here were generally similar within species across sampling sessions, the confidence intervals were large, highlighting that more intense effort (i.e. longer secondary samples or more boats) would produce more informative results. This would allow for assessment of the heterogeneity assumption, provide a basis for a meaningful description of temporary emigration processes and improvement of the precision of the estimates.
Recommendations for sampling design in future studies
The analyses here highlighted some constraints in the study design and effort. Although we were unable to provide useful estimates of temporary emigration, we do not claim that there was no temporary emigration. There is reason to expect it in any study area that is likely to be smaller than the home range of a local population of these highly mobile animals. For example, the snubfin model with random temporary emigration varying by primary sample had the second-lowest AICc and a deviance that was ~5.1 lower than for the best-fitting model (calculated from their AICc values), and it was inclusion of the additional four parameters in the AICc for the temporary emigration model that led to it being ranked lower than a model with no temporary emigration. In the study area of 325 km2, some signal for temporary emigration was detected and the estimated emigration rates were high. Given that the size of the home range of a local population was not known before survey, it is important that the study design adequately allows for consideration of the extent of temporary emigration.
The analysis of the results from the present study indicated that future study design may be more effective if sampling is concentrated in fewer primary samples per year, with longer periods being allocated within each primary sample period, to allow for days in which full sampling could not be completed because of extraneous factors (notably inclement weather), so as to ensure that primary samples included about five or six ‘good’ secondary samples. The use of more than one boat could usefully contribute to better coverage of the study area and limiting the time crews may be required to spend under difficult conditions in remote areas.
Sampling these species is expensive and challenging in remote areas subject to highly variable weather conditions and careful planning in the allocation of resources is necessary to maximise the chance of obtaining the quality of data required to provide informative results. The present study has highlighted that focussing surveys at the time of year when sea-state conditions are optimal (September–November) for sightings would improve the cost effectiveness of survey program in these remote areas and provide a basis for estimating change in abundance over time.
Management implications
The aim of the study was to derive baseline population estimates for snubfin, humpback and bottlenose dolphins in the coastal waters of monsoonal northern Australia, which will allow for future comparisons as development activities in this remote region increase. The information from the study can assist future efforts to develop a robust-design model with good precision and the capacity to estimate temporary emigration rates. Following recent guidelines for a coordinated research strategy to collect information required to assess the national conservation status of Australian tropical inshore dolphins (Parra et al. 2012), we recommend that comparable, but more intensive, studies across a set of key sites (with varying environmental settings, including degree of anthropogenic impact) be undertaken across northern Australian coastal waters, to provide robust estimates of population sizes as baseline for ongoing monitoring of population trends. This is a necessary foundation for establishing sound management actions, and measuring their efficacy.
Acknowledgements
The project would never have been possible without the tremendous support of rangers from Parks and Wildlife of the Northern Territory, Peter Fitzgerald, Andy Wood and Adrian McKenzie. CP warmly thanks all the volunteers who assisted in the boat-based surveys and friends and colleagues from the Flora and Fauna Division (NT Government) and Chris Austin (Charles Darwin University). Special thanks go to Felicity Watt and Matthew Fegan for their constant support with ArcGIS and mapping. Funding was received from the Natural Resource Management Board of the NT (Project no. CF2007/135). Research was carried out under permits from the Parks and Wildlife Service of the Northern Territory (33840) and Charles Darwin University Animal Ethics Committee (A06018).
References
Allen, S. J., Cagnazzi, D. D. B., Hodgson, A. J., Loneragan, N. R., and Bejder, L. (2012). Tropical inshore dolphins of north-western Australia: unknown populations in a rapidly changing region. Pacific Conservation Biology 18, 56–66.Ansmann, I. C., Lanyon, J. M., Seddon, J. M., and Parra, G. J. (2013). Monitoring dolphins in an urban marine system: total and effective population size estimates of Indo-Pacific bottlenose dolphins in Moreton Bay, Australia. PLoS ONE 8, e65239.
| Monitoring dolphins in an urban marine system: total and effective population size estimates of Indo-Pacific bottlenose dolphins in Moreton Bay, Australia.Crossref | GoogleScholarGoogle Scholar | 1:CAS:528:DC%2BC3sXpvVKhtb8%3D&md5=9c4db160ddc437d6660dcdd1ffe13710CAS | 23755197PubMed |
Balmer, B. C., Wells, R. S., Nowacek, D. P., Schwacke, L. H., Mclellan, W. A., Scharf, F. S., Rowles, T. K., Hansen, L. J., Spradlin, T. R., and Pabst, D. A. (2008). Seasonal abundance and distribution patterns of common bottlenose dolphins (Tursiops truncatus) near St Joseph Bay, Florida, USA. The Journal of Cetacean Research and Management 10, 157–167.
Beasley, I., Allen, S., and Parra, G. J. (2012a). Review of Australian snubfin dolphin nomination for listing as threatened species under the EPBC Act. Australian Government Department of Sustainability, Environment, Water, Population and Communities, Canberra.
Beasley, I., Allen, S., and Parra, G. J. (2012b). Current status of inshore dolphins in northern Australia. Australian Government Department of Sustainability, Environment, Water, Population and Communities, Canberra.
Bejder, L., Hodgson, A. J., Loneragan, N. R., and Allen, S. J. (2012). Coastal dolphins in north-western Australia: the need for re-evaluation of species listings and short-comings in the environmental impact assessment process. Pacific Conservation Biology 18, 22–25.
Borobia, M., Siciliano, S., Lodi, L., and Hoek, W. (1991). Distribution of the South American dolphin Sotalia fluviatilis. Canadian Journal of Zoology 69, 1025–1039.
| Distribution of the South American dolphin Sotalia fluviatilis.Crossref | GoogleScholarGoogle Scholar |
Burnham, K. P., and Anderson, D. R. (2002). ‘Model Selection and Multimodel Inference: a Practical Information-theoretic Approach.’ 2nd edn. (Springer-Verlag: New York.)
Burnham, K. P., Anderson, D. R., White, G. C., Brownie, C., and Pollock, K. P. (1987). ‘Design and Analysis Methods for Fish Survival Experiments based on Release–Recapture.’ Monograph 5. (American Fisheries Society: Bethesda, MD.)
Cagnazzi, D. (2010). Conservation Status of Australian snubfin dolphin, Orcaella heinsohni, and Indo-Pacific humpback dolphin, Sousa chinensis, in the Capricorn Coast, central Queensland, Australia. Ph.D. Thesis, Southern Cross University, Lismore, NSW.
Cagnazzi, D. D. B., Harrison, P. L., Ross, G. J. B., and Lynch, P. (2011). Abundance and site fidelity of Indo-Pacific Humpback dolphins in the Great Sandy Strait, Queensland, Australia. Marine Mammal Science 27, 255–281.
| Abundance and site fidelity of Indo-Pacific Humpback dolphins in the Great Sandy Strait, Queensland, Australia.Crossref | GoogleScholarGoogle Scholar |
Cagnazzi, D., Parra, G. J., Westley, S., and Harrison, P. L. (2013). At the heart of the industrial boom: Australian snubfin dolphins in the Capricorn Coast, Queensland, need urgent conservation action. PLoS ONE 8, e56729.
| At the heart of the industrial boom: Australian snubfin dolphins in the Capricorn Coast, Queensland, need urgent conservation action.Crossref | GoogleScholarGoogle Scholar | 1:CAS:528:DC%2BC3sXjsF2itr8%3D&md5=3e28e217ef18344e0ca25eb0fd1f6af2CAS | 23437225PubMed |
Cantor, M., Wedekin, L. L., Daura-Jorge, F. G., Rossi-Santos, M. R., and Simões-Lopes, P. C. (2012). Assessing population parameters and trends of Guiana dolphins (Sotalia guianensis): an eight year mark–recapture study. Marine Mammal Science 28, 63–83.
| Assessing population parameters and trends of Guiana dolphins (Sotalia guianensis): an eight year mark–recapture study.Crossref | GoogleScholarGoogle Scholar |
Charlton-Robb, K., Gershwin, L-a., Thompson, R., Austin, J., Owen, K., and McKenchnie, S. (2011). A new dolphin species, the Burrunan Dolphin Tursiops australis sp. nov., endemic to southern Australian coastal waters. PLoS ONE 6, 1–17.
Chilvers, B. L., and Corkeron, P. J. (2003). Abundance of Indo-Pacific bottlenose dolphins, Tursiops aduncus, off Point Lookout, Queensland, Australia. Marine Mammal Science 19, 85–95.
| Abundance of Indo-Pacific bottlenose dolphins, Tursiops aduncus, off Point Lookout, Queensland, Australia.Crossref | GoogleScholarGoogle Scholar |
Connor, R. C., Wells, R. S., Mann, J., and Read, A. J. (2000). The bottlenose dolphin: social relationships in a fission–fusion society. In ‘Cetacean Societies’. (Eds J. Mann, R. C. Connor, P. L. Tyack and H. Whitehead.) pp. 91–126. (University of Chicago Press: Chicago, IL.)
Cooch, E., and White, G. (2012). ‘Program MARK. ‘A Gentle Introduction’.’ 11th edn. Available at http://www.phidot.org/software/mark/docs/book/ [verified 11 March 2014].
Corkeron, P. J., Morissette, N. M., Porter, L., and Marsh, H. (1997). Distribution and status of humpbacked dolphins Sousa chinensis, in Australian waters. Asian Marine Biology 14, 49–57.
Crosbie, S. F., and Manly, B. F. J. (1985). Parsimonious modelling of capture–mark–recapture studies. Biometrics 41, 385–398.
| Parsimonious modelling of capture–mark–recapture studies.Crossref | GoogleScholarGoogle Scholar |
Dhandapani, P. (1992). Status of the Irrawaddy River dolphin Orcaella brevirostris in Chilka Lake. Journal of the Marine Biological Association of India 34, 90–93.
Edyvane, K., and Dethmers, K. (2010). Identifying potential areas for marine protected areas in the Northern Territory. Final project report CF2007/135. Department of Natural Resources, Environment the Arts and Sport, Darwin.
Fazioli, K. L., Hofmann, S., and Wells, R. S. (2006). Use of Gulf of Mexico coastal waters by distinct assemblages of bottlenose dolphins (Tursiops truncatus). Aquatic Mammals 32, 212–222.
| Use of Gulf of Mexico coastal waters by distinct assemblages of bottlenose dolphins (Tursiops truncatus).Crossref | GoogleScholarGoogle Scholar |
Frère, C. H., Seddon, J., Palmer, C., Porter, L., and Parra, G. J. (2011). Multiple lines of evidence for an Australasian geographic boundary in the Indo-Pacific humpback dolphin (Sousa chinensis): population or species divergence? Conservation Genetics 12, 1633–1638.
| Multiple lines of evidence for an Australasian geographic boundary in the Indo-Pacific humpback dolphin (Sousa chinensis): population or species divergence?Crossref | GoogleScholarGoogle Scholar |
Fury, C. A., and Harrison, P. L. (2008). Abundance, site fidelity and range patterns of Indo-Pacific bottlenose dolphins (Tursiops aduncus) in two Australian subtropical estuaries. Marine and Freshwater Research 59, 1015–1027.
| Abundance, site fidelity and range patterns of Indo-Pacific bottlenose dolphins (Tursiops aduncus) in two Australian subtropical estuaries.Crossref | GoogleScholarGoogle Scholar |
Halpern, B. S., Walbridge, S., Selkoe, K. A., Kappel, C. V., Micheli, F., D’Agrosa, C., Bruno, J. F., Kenneth, S., Casey, K. S., Ebert, C., Fox, H. E., Fujita, R., Heinemann, D., Lenihan, H. S., Madin, E. M. P., Matthew, T., Perry, M. T., Selig, E. R., Spalding, M., Robert Steneck, R., and Watson, R. (2008). A global map of human impact on marine ecosystems. Science 319, 948–952.
| A global map of human impact on marine ecosystems.Crossref | GoogleScholarGoogle Scholar | 1:CAS:528:DC%2BD1cXhslOmtrk%3D&md5=da37b1149cd8dc551172209ab0638f06CAS | 18276889PubMed |
Hammond, P. S., and Thompson, P. M. (1991). Minimum estimate of the number of bottlenose dolphins (Tursiops truncatus) in the Moray Firth, NE Scotland. Biological Conservation 56, 79–87.
| Minimum estimate of the number of bottlenose dolphins (Tursiops truncatus) in the Moray Firth, NE Scotland.Crossref | GoogleScholarGoogle Scholar |
Irvine, A. B., Scott, M. D., Wells, R. S., and Kaufmann, J. H. (1981). Movements and activities of the Atlantic bottlenose dolphin, Tursiops truncatus, near Sarasota, Florida. Fish Bulletin 79, 671–688.
Jolly, G. M. (1965). Explicit estimates from capture–recapture data with both death and immigration-Stochastic model. Biometrika 52, 225–247.
| 1:STN:280:DyaF2M7ks1WktQ%3D%3D&md5=88ad844f9a6079c7ea057e0d0dc0b47dCAS | 14341276PubMed |
Kemper, C. (2004). Osteological variation and taxonomic affinities of bottlenose dolphins Tursiops spp., from South Australia. Australian Journal of Zoology 52, 29–48.
| Osteological variation and taxonomic affinities of bottlenose dolphins Tursiops spp., from South Australia.Crossref | GoogleScholarGoogle Scholar |
Kendall, W. L., and Bjorkland, R. (2001). Using open robust design models to estimate temporary emigration from capture–recapture data. Biometrics 57, 1113–1122.
| Using open robust design models to estimate temporary emigration from capture–recapture data.Crossref | GoogleScholarGoogle Scholar | 1:STN:280:DC%2BD38%2Fjs1yqug%3D%3D&md5=4ead789415a996f25c27c380d5b0bfc4CAS | 11764251PubMed |
Kendall, W. L., and Nichols, J. D. (1995). On the use of secondary capture–recapture samples to estimate temporary emigration and breeding proportions. Journal of Applied Statistics 22, 751–762.
| On the use of secondary capture–recapture samples to estimate temporary emigration and breeding proportions.Crossref | GoogleScholarGoogle Scholar |
Kendall, W. L., Pollock, K. H., and Brownie, C. (1995). A likelihood-based approach to capture–recapture estimation of demographic parameters under the robust design. Biometrics 51, 293–308.
| A likelihood-based approach to capture–recapture estimation of demographic parameters under the robust design.Crossref | GoogleScholarGoogle Scholar | 1:STN:280:DyaK2M3osVansQ%3D%3D&md5=baebf3753bae512b5424feb12947c4b4CAS | 7766783PubMed |
Kendall, W. L., Nichols, J. D., and Hines, J. E. (1997). Estimating temporary emigration using capture–recapture data with Pollock’s robust design. Ecology 78, 563–578.
Kreb, D., and Budiono, (2005). Conservation management of small core areas: key to survival of a critically endangered population of Irrawaddy river dolphins Orcaella brevirostris in Indonesia. Oryx 39, 178–188.
| Conservation management of small core areas: key to survival of a critically endangered population of Irrawaddy river dolphins Orcaella brevirostris in Indonesia.Crossref | GoogleScholarGoogle Scholar |
Krützen, M., Sherwin, W. B., Berggren, P., and Gales, N. (2004). Population structure in an inshore cetacean revealed by microsatellite and mtDNA analysis: Bottlenose dolphins (Tursiops sp.) in Shark Bay, Western Australia. Marine Mammal Science 20, 28–47.
Lebreton, J. D., Burnham, K. P., Clobert, J., and Anderson, D. R. (1992). Modelling survival and testing biological hypotheses using marked animals: a unified approach with case studies. Ecological Monographs 62, 67–118.
| Modelling survival and testing biological hypotheses using marked animals: a unified approach with case studies.Crossref | GoogleScholarGoogle Scholar |
Lukoschek, V., and Chilvers, B. L. (2008). A robust baseline for bottlenose dolphin abundance in coastal Moreton Bay: a large carnivore living in a region of escalating anthropogenic impacts. Wildlife Research 35, 593–605.
| A robust baseline for bottlenose dolphin abundance in coastal Moreton Bay: a large carnivore living in a region of escalating anthropogenic impacts.Crossref | GoogleScholarGoogle Scholar |
Mendez, M., Jefferson, T. A., Kolokotronis, S.-O., Krützen, M., Parra, G. J., Collins, T., Minton, G., Baldwin, R., Berggren, P., Särnblad, A., Amir, O. A., Peddemors, V. M., Karczmarski, L., Guissamulo, A., Smith, B., Sutaria, D., Amato, G., and Rosenbaum, H. C. (2013). Integrating multiple lines of evidence to better understand the evolutionary divergence of humpback dolphins along their entire distribution range: a new dolphin species in Australian waters? Molecular Ecology 22, 5936–5948.
| Integrating multiple lines of evidence to better understand the evolutionary divergence of humpback dolphins along their entire distribution range: a new dolphin species in Australian waters?Crossref | GoogleScholarGoogle Scholar | 24268046PubMed |
Möller, L. M., and Beheregaray, L. B. (2001). Coastal bottlenose dolphins from southeastern Australia are Tursiops aduncus according to sequences of the mitochondrial DNA control region. Marine Mammal Science 17, 249–263.
Möller, L. M., Allen, S. J., and Harcourt, R. G. (2002). Group characteristics, site fidelity and seasonal abundance of bottlenose dolphins Tursiops aduncus in Jervis Bay and Port Stephens, south-eastern Australia. Australian Mammalogy 24, 11–22.
| Group characteristics, site fidelity and seasonal abundance of bottlenose dolphins Tursiops aduncus in Jervis Bay and Port Stephens, south-eastern Australia.Crossref | GoogleScholarGoogle Scholar |
Nicholson, K., Bejder, L., Allen, S., Krützen, K., and Pollock, K. (2012). Abundance, survival and temporary emigration of bottlenose dolphins (Tursiops sp.) off Useless Loop in the western gulf of Shark Bay, Western Australia. Marine and Freshwater Research 63, 1059–1068.
| Abundance, survival and temporary emigration of bottlenose dolphins (Tursiops sp.) off Useless Loop in the western gulf of Shark Bay, Western Australia.Crossref | GoogleScholarGoogle Scholar |
Otis, D. L., Burnham, K. P., White, G. C., and Anderson, D. R. (1978). Statistical inference from capture data on closed animal populations. Wildlife Monographs 62, 1–135.
Palmer, C., Murphy, S. A., Thiele, D., Parra, J. G., Robertson, K. M., Beasley, I., and Austin, C. M. (2011). Analysis of mitochondrial DNA clarifies the taxonomy and distribution of the Australian snubfin dolphin (Orcaella heinsohni) in northern Australian waters. Marine and Freshwater Research 62, 1303–1307.
| Analysis of mitochondrial DNA clarifies the taxonomy and distribution of the Australian snubfin dolphin (Orcaella heinsohni) in northern Australian waters.Crossref | GoogleScholarGoogle Scholar | 1:CAS:528:DC%2BC3MXhsVaqu77N&md5=68927b87609be8df4b15c4fbce5db5f6CAS |
Palmer, C., Parra, G. J., Rogers, T., and Woinarski, J. C. Z. (2014). Collation and review of sightings and distribution of three coastal dolphin species in waters of the Northern Territory, Australia. Pacific Conservation Biology , in press.
Parra, G. J. (2006). Resource partitioning in sympatric delphinids: space use and habitat preferences of Australian snubfin and Indo-Pacific humpback dolphins. Journal of Animal Ecology 75, 862–874.
| Resource partitioning in sympatric delphinids: space use and habitat preferences of Australian snubfin and Indo-Pacific humpback dolphins.Crossref | GoogleScholarGoogle Scholar | 17009750PubMed |
Parra, G. J., and Arnold, P. W. (2008). Australian snubfin dolphin: Orcaella heinsohni. In ‘The Mammals of Australia’. (Eds S. M. Van Dyck and R. Strahan.) pp. 865–866. (New Holland: Sydney.)
Parra, G. J., and Corkeron, P. J. (2001). Feasibility of using photo-identification techniques to study the Irrawaddy dolphin, Orcaella brevirostris (Owen in Gray 1866). Aquatic Mammals 27, 45–49.
Parra, G. J., and Ross, G. J. B. (2009). Humpback dolphins: S. chinensis and S. teuszii. In ‘Encyclopedia of Marine Mammals’. 2nd edn. (Eds W. F. Perrin, W. Bernd and J. G. M. Thewissen.) pp. 576–582. (Academic Press: London.)
Parra, G. J., Azuma, C., Preen, A. R., Corkeron, P. J., and Marsh, H. (2002). Distribution of Irrawaddy dolphins, Orcaella brevirostris, in Australian waters. The Raffles Bulletin of Zoology 10, 141–154.
Parra, G. J., Corkeron, P. J., and Marsh, H. (2004). The Indo-Pacific humpback dolphin, Sousa chinensis (Osbeck, 1765), in Australian waters: a summary of current knowledge. Aquatic Mammals 30, 197–206.
Parra, G. J., Corkeron, P. J., and Marsh, H. (2006). Population sizes, site fidelity and residence patterns of Australian snubfin and Indo-Pacific humpback dolphins: implications for conservation. Biological Conservation 129, 167–180.
| Population sizes, site fidelity and residence patterns of Australian snubfin and Indo-Pacific humpback dolphins: implications for conservation.Crossref | GoogleScholarGoogle Scholar |
Parra, G. J., Beasley, I., Allen, S., Brooks, L., and Pollock, H. (2012). Coordinated research strategy to collect information required to assess the national conservation status of Australian tropical inshore dolphins. Final report to the Department of Sustainability, Environment, Water, Population and Communities, Canberra, ACT.
Pollock, K. H. (1982). A capture–recapture design robust to unequal probability of capture. The Journal of Wildlife Management 46, 752–757.
| A capture–recapture design robust to unequal probability of capture.Crossref | GoogleScholarGoogle Scholar |
Pollock, K. H., Nichols, J. D., Brownie, C., and Hines, J. E. (1990). Statistical inference for capture–recapture experiments. Wildlife Monographs 107, 1–98.
Preen, A. R., Marsh, H., Lawler, I. R., Prince, R. I. T., and Shepherd, R. (1997). Distribution and abundance of dugongs, turtles, dolphins and other megafauna in Shark Bay, Ningaloo Reef and Exmouth Gulf, Western Australia. Wildlife Research 24, 185–208.
| Distribution and abundance of dugongs, turtles, dolphins and other megafauna in Shark Bay, Ningaloo Reef and Exmouth Gulf, Western Australia.Crossref | GoogleScholarGoogle Scholar |
Reeves, R. R., Dawson, S. M., Jefferson, T. A., Karczmarski, L., Laidre, K., O’Corry-Crowe, G., Rojas-Bracho, L., Secchi, E. R., Slooten, E., Smith, B. D., Wang, J. Y., and Zhou, K. (2013). Cephalorhynchus hectori. In: IUCN 2013. IUCN Red List of Threatened Species. Version 2013.2. Available at www.iucnredlist.org [verified 9 March 2014].
Rice, D. W. (1998). Marine mammals of the world: systematics and distribution. Society for Marine Mammalogy 4, 102–104.
Rojas-Bracho, L., Reeves, R. R., and Jaramillo-Legorreta, A. (2006). Conservation of the vaquita Phocoena sinus. Mammal Review 36, 179–216.
Rosel, P. E., Mullin, K. D., Garrison, L. P., Schwacke, L., Adams, J., Balmer, B. C., Conn, P., Conroy, M. J., Eguchi, T., Gorgone, A., Hohn, A., Mazzoil, M., Schwarz, C., Sinclair, C., Speakman, T., Urian, K., Vollmer, N., Wade, P., Wells, R., and Zolman, E. (2011). Photo-identification capture–mark–recapture techniques for estimating abundance of Bay, Sound and Estuary Populations of Bottlenose dolphins along the US East Coast and Gulf of Mexico: a workshop report NOAA Technical Memorandum NMFS–SEFSC-621. National Oceanic and Atmospheric Administration, National Marine Fisheries Service, Lafayette, LA.
Ross, G. J. B., and Cockcroft, V. G. (1990). Comments on Australian Bottlenose Dolphins and the taxonomic status of Tursiops aduncus (Ehrenberg, 1832). In: ‘The Bottlenose Dolphin’. (Eds S. Leatherwood, R. R. Randall) pp 101–128. (Academic Press: London)
Schwarz, C. J., and Arnason, A. N. (1996). A general methodology for the analysis of capture–recapture experiments in open populations. Biometrics 52, 860–873.
| A general methodology for the analysis of capture–recapture experiments in open populations.Crossref | GoogleScholarGoogle Scholar |
Seber, G. A. F. (1965). A note on the multiple-recapture census. Biometrika 52, 249–259.
| 1:STN:280:DyaF2M7ks1Wkug%3D%3D&md5=dc0c961918eee6a971d8af08530c3512CAS |
Silva, M. A., Magalhães, S., Prieto, R., Santos, R. S., and Hammond, P. S. (2010). Estimating survival and abundance in a bottlenose dolphin population taking into account transience and temporary emigration. Marine Ecology Progress Series 392, 263–276.
| Estimating survival and abundance in a bottlenose dolphin population taking into account transience and temporary emigration.Crossref | GoogleScholarGoogle Scholar |
Smith, H. (2012) Population dynamics and habitat use of bottlenose dolphins (Tursiops aduncus), Bunbury, Western Australia. Ph.D. Thesis, Murdoch University, Perth.
Smith, H. C., Pollock, K., Waples, K., Bradley, S., and Bejder, L. (2013). Use of the robust design to estimate seasonal abundance and demographic parameters of a coastal bottlenose dolphin (Tursiops aduncus) population. PLoS ONE 8, e76574.
| Use of the robust design to estimate seasonal abundance and demographic parameters of a coastal bottlenose dolphin (Tursiops aduncus) population.Crossref | GoogleScholarGoogle Scholar | 1:CAS:528:DC%2BC3sXhs1egs7%2FO&md5=fa1f43f5e53e2975043d3f2343303b8eCAS | 24130781PubMed |
Speakman, T. R., Lane, S. M., Schwacke, L. H., Fair, P. A., and Zolman, E. S. (2010). Mark–recapture estimates of seasonal abundance and survivorship for bottlenose dolphins (Tursiops truncatus) near Charleston, South Carolina, USA. The Journal of Cetacean Research and Management 11, 153–162.
Stanley, T. R., and Burnham, K. P. (1999). A closure test for time specific capture–recapture data. Environmental and Ecological Statistics 6, 197–209.
| A closure test for time specific capture–recapture data.Crossref | GoogleScholarGoogle Scholar |
Taylor, B. L., and Gerrodette, T. (1993). The uses of statistical power in conservation biology: the vaquita and northern spotted owl. Conservation Biology 7, 489–500.
| The uses of statistical power in conservation biology: the vaquita and northern spotted owl.Crossref | GoogleScholarGoogle Scholar |
Thompson, P. M., Wilson, B., Grellier, K., and Hammond, P. S. (2000). Combining power analysis and population viability analysis to compare traditional and precautionary approaches to conservation of coastal cetaceans. Conservation Biology 14, 1253–1263.
| Combining power analysis and population viability analysis to compare traditional and precautionary approaches to conservation of coastal cetaceans.Crossref | GoogleScholarGoogle Scholar |
Wells, R. S., Scott, M. D., and Irvine, A. B. (1987). The social structure of free-ranging bottlenose dolphins. In ‘Current Mammalogy’. (Ed. H. Genoways.) pp. 247–306. (Plenum Press: New York.)
White, G. C., and Burnham, K. P. (1999). Program MARK: survival estimation from populations of marked animals. Bird Study 46, S120–S139.
| Program MARK: survival estimation from populations of marked animals.Crossref | GoogleScholarGoogle Scholar |
Williams, B. K., Nichols, J. D., and Conroy, M. J. (2002). ‘Analysis and Management of Animal Populations: Modelling, Estimation, and Decision Making.’ (Academic Press: San Diego, CA.)
Wilson, B. K., Hammond, D., and Thompson, P. M. (1999). Estimating size and assessing trends in a coastal bottlenose dolphin population. Ecological Applications 9, 288–300.
| Estimating size and assessing trends in a coastal bottlenose dolphin population.Crossref | GoogleScholarGoogle Scholar |
Würsig, B., and Jefferson, T. A. (1990). Methods of photo-identification for small cetaceans. In ‘Individual Recognition of Cetaceans: Use of Photo-Identification and Other Techniques to Estimate Population Parameters’. (Eds P. S. Hammond, S. A. Mizroch and G. P. Donovan.) pp. 43–52. (International Whaling Commission: Cambridge, UK.)