Operational field trialling of Felixer™ grooming traps for the control of feral cats in the Strzelecki Desert, Australia
R. D. Pedler


A
B
C
Abstract
Feral cats (Felis catus) have an impact on native wildlife populations around the world but are difficult to control because of their neophobic behaviours and preference for live prey over scavenging poisoned baits. Felixers™ address these challenges by squirting poison-gel directly onto feral cats, exploiting their fastidious oral grooming tendencies to facilitate poison ingestion.
This study trialled Felixers in a landscape-scale arid-ecosystem site to assess their capacity to sustainably manage cat populations in a semi-bounded in situ predator training area and to eradicate cat incursions into a feral-free safe haven. Specifically, the aims were to determine target specificity and firing rates; optimise installation sites to exploit cat behaviour and landscape features; assess the fate of individuals following Felixer interaction; and assess the overall cost, performance and efficacy of Felixers compared with conventional cat control methods.
Up to nine Felixers were simultaneously deployed across three trial periods, spanning 30 months (4,642 trap nights) in Sturt National Park in the Strzelecki Desert dunefields of Australia. Felixers were deployed within and outside of feral-free safe havens, at varying cat densities, with populations monitored through camera-trap activity indices and individual behaviour monitored through satellite tracking.
Felixers fired at 20.3–43.9% of cats that passed in front (292 of 1,144 cats), with the remainder failing to satisfy the discriminatory algorithm’s target criteria. The devices had 99.93% target specificity from 17,425 interactions with moving animals and objects. Overall cat activity was lower in a semi-bounded 10,400 ha landscape-scale area where Felixers were intensively used, relative to an adjacent unbounded area where no cat control was occurring, over a 12-month subset of the 30-month trial. Felixers also resolved one of two incursions by cats into a 2,000 ha safe haven. Optimisation of Felixer placement and upgrading of software drove improvements in technical reliability and target identification during the trial. Shortcomings included cost and high incidence of technical faults, causing significant downtime and requiring regular investment of field staff time for monitoring and resolution.
Felixers provide a safe tool for cat management and eradication at intensively managed conservation sites. Efficacy could be improved through reductions in cost and improvements in reliability.
Overall Felixers appear to be an effective tool for cat management, when used in an integrated approach.
Keywords: 1080, arid zone, feral cat, invasive species, pest control, reintroduced mammals, sodium fluoroacetate, trap.
Introduction
Feral cats (Felis catus) cause considerable impact to wildlife populations both globally (Medina et al. 2011; Nogales et al. 2013) and in Australia (Woinarski et al. 2014) where they are the focus for pest-control programs (Doherty et al. 2017; Comer et al. 2020; Legge et al. 2020; Lohr and Algar 2020; Moseby et al. 2021). There is a critical need to control cats within areas managed for threatened species and to drive innovation in such methods to maximise effectiveness and impact (Woinarski et al. 2019).
Cats prefer to hunt live prey, rendering conventional invasive pest-control methods such as baiting effective only during food shortages when prey is scarce (Algar et al. 2007; Moseby et al. 2011). Felixer grooming traps (Thylation, www.thylation.com) provide a target-specific lethal control tool for cats that is not restricted to times of food shortage. Felixers are automated stationary devices, usually deployed on predator thoroughfares, such as tracks, fencelines or watercourses. They use an array of sensors to automatically detect and distinguish feral cats and foxes from other moving objects and animals (Read et al. 2014). Once detected by the Felixer sensors and classified as a target by a discriminatory algorithm or artificial intelligence processor, target animals are squirted with a measured dose of toxin-laden gel, subsequently ingested through instinctive oral grooming (Read et al. 2019). The Felixer also takes a time and location-stamped photograph and logs the rate of movement, angle and other metrics when the animal or object is classified (Read et al. 2019). Trials of Felixers have included pen trials to determine proof of concept and target accuracy (Read et al. 2014); photo-only field trials to determine potential impacts on non-target species (Chambers et al. 2020; Rickards et al. 2023); or intensive field trials over a short timeframe of several months and/or limited geographic extent (Hodgens 2019, unpubl. data; Moseby et al. 2020a).
This study trialled Felixers over a time-frame of 30 months to determine their capacity to manage cat populations in a semi-bounded landscape-scale area, set up for trials of in situ predator training (Moseby et al. 2016; West et al. 2018) and to manage incursions into smaller fenced feral-free exclosures. Specifically, the aims were to determine (1) Felixer target accuracy rates for cats and non-target species and comparison between LiDAR-algorithm and artificial intelligence discriminator models, (2) optimisation of Felixer siting within the landscape in relation to cat behaviour and landscape features, particularly predator-proof exclusion fences, (3) the fate of tagged cats following Felixer firing to determine mortality rate, and time and distance moved before death, (4) efficacy of ongoing Felixer usage for controlling cats at a landscape-scale, particularly in a semi-bounded and bounded safe-haven incursion scenarios, (5) Felixer technical performance to recommend innovation and improvements and, (6) the investment needed in relation to time inputs, cost and efficacy compared with conventional cat control methods.
Materials and methods
Study site
The study was conducted over 30 months, from March 2021 to August 2023, at the Wild Deserts partnership site in Sturt National Park in far north-western New South Wales. The site features four main management treatments, including two separate feral predator-free exclosures, Thipa (2,000 ha) and Mingku (2,300 ha), the wild training zone (10,400 ha) and an unbounded outside area (park 20,300 ha) to the east of the wild training zone and exclosures (Fig. 1) (Pedler et al. 2018). An explicit strategic adaptive management approach identified control of feral cats as a key objective, including innovating effective tools, particularly in relation to establishing populations of threatened mammal species (greater bilbies, burrowing bettongs, western quolls) beyond safe havens (Kingsford et al. 2021). Felixers were identified as a potential tool to manage cat incursions into the feral-free safe havens and to suppress populations in the 10,400 ha wild training zone (WTZ). This irregularly shaped triangle area is bounded on the western and northern perimeters (total 28 km) by the dingo barrier fence (Newsome et al. 2001) and on the eastern perimeter (25 km) by the 1.8 m high predator-exclusion fencing (Fig. 1). Given the design of the dingo barrier fence, the WTZ does not exclude all cats or foxes but is designed to be ‘leaky’ to expose native reintroduced species to low levels of cat predation to accelerate natural selection for anti-predator traits (Moseby et al. 2016; West et al. 2018).
Map of the study site in Sturt National Park showing management treatments, including the semi-bounded wild training zone (WTZ), unbounded outside park area and Thipa feral-free safe haven exclosure, where Felixer trials A, B and C were conducted (Table 1). The locations of cameras in the camera trapping arrays were used to calculate cat activity indices and identify individual cats to estimate density.
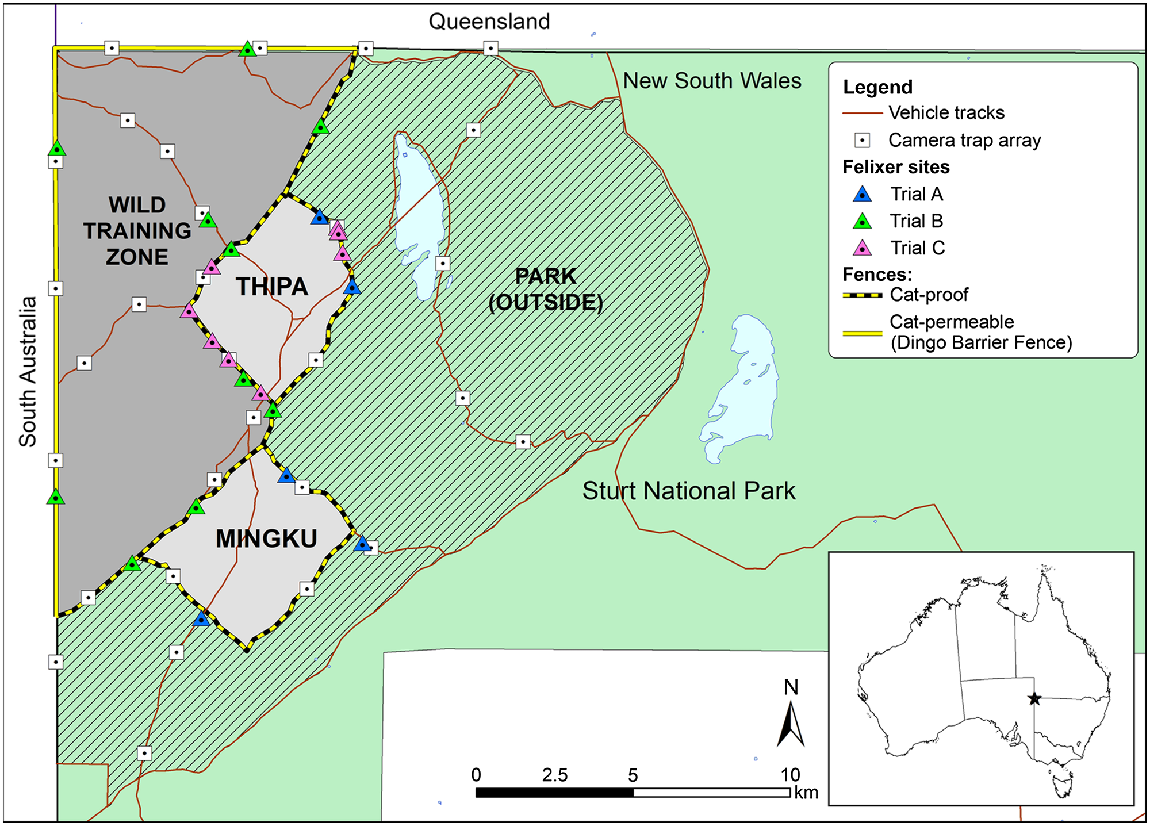
Trial periods and Felixer specifications
The study was divided into three key trial periods (Table 1). Trial period A (12 months, March 2021–March 2022) involved the first usage of Felixers in toxic mode in New South Wales and followed an extended photo only trial, with the devices stationed at up to five sites around the external perimeter of the feral-free exclosures, bordering the large unbounded park treatment where no other cat control was occurring. Trial B began in April 2022, when up to nine Felixers were simultaneously deployed in the WTZ to examine the ability of Felixers to manage cat populations inside this semi-bounded area. During Trial B, cat activity was compared with that in the unbounded park treatment where no cat control was occurring. Trials C1 and C2 involved testing the devices in the Thipa feral-free safe haven, with the aim of removing two recently detected feral cat incursions (C1: 18 September−8 November 2022; C2: 1 June−20 July 2023, Table 1).
Trial period and management treatment | Dates | Models and discrimination algorithms | Description | Aims | Trap-nights | |
---|---|---|---|---|---|---|
A: park (initial trials) | Mar. 2021–Mar. 2022 | 3.0, 3.1 Conservative | First use of Felixers in toxic mode in New South Wales, in which up to five units were stationed around the external perimeter fences of the two feral-free exclosures. | Initial data on firing rates for cats and non-targets | 1241 | |
B: WTZ | Apr. 2022–Aug. 2023 | 3.0, 3.1, 3.2 Conservative, standard, AI | Up to nine Felixers were simultaneously deployed around the internal perimeter of the WTZ. | Examine impact of Felixers on cat populations in large semi-bounded area, compared to unbounded outside area (park) | 3017 | |
C: Thipa exclosure | C1: 18 September − 8 November 2022 C2: 1 June − 20 July 2023 | 3.0, 3.1, 3.2 Standard, aggressive, AI | Two consecutive cat incursions (C1 and C2) were detected inside the Thipa feral-free exclosure and up to five Felixers were temporarily removed from the WTZ and redeployed inside there, each time targeting one individual cat. | Removal of cat incursions into safe haven. | C1: 199 C2: 185 | |
Total | 4642 |
Much of this study was conducted before registration of Felixers by the Australian Pesticides and Veterinary Medicine Association in late 2022 under a certified trial, governed and sanctioned by a NPWS Conservation Risk Assessment Approval. Prior to and throughout this trial, Felixer design and particularly firmware underwent continuous adaptive change (11 firmware upgrades during the trial). The changes by the manufacturer were in response to performance data from Felixer trial sites across Australia and, in some cases, forced deliberate changes in components available for manufacture. The incorporation of an artificial intelligence camera in the model 3.2 Felixer also included several upgraded identification algorithms, as data from these test deployments were integrated. During the study period, Felixer Models 3.0 and 3.1 had two possible LiDAR target discrimination algorithms, based on the rate of movement, angle, and size profile of moving objects, namely, conservative and standard modes. These modes respectively ranged from most stringent to least discriminatory, on the basis of the decision criteria. Conservative mode was designed for when there was a high incidence of off-target fauna, reducing firing at both target and non-target animals. NPWS imposed the trial condition that only conservative mode could be used during the first 24 months of the trial. This condition was lifted for the last 6 months (Table 1), allowing the use of standard mode.
Felixer data were collated by on-board USB flash drives, then uploaded to the cloud-based Felixer Management System, which curates and co-ordinates Felixer data Australia-wide. In total, 17,425 photos were taken by Felixers during the study; most could be positively identified as either moving objects (e.g. vehicles, wind-blown debris) or animals, to species or genus level.
Optimisation of Felixer deployment strategies
Nineteen feral cats were caught and fitted with satellite-tracking collars in two phases. Ten cats were caught within the WTZ during May–October 2021 and fitted with a Lotek Litetrack RF150 GPS transmitting collars. This phase aimed to determine movement patterns relevant to improving monitoring and control, such as identifying high activity zones or thoroughfares used by cats that could be exploited to optimise Felixer placement and determine the frequency of movement in and out of the WTZ. Thus, it was deliberately conducted in a zone where no Felixers were deployed. Three of the 10 cats with satellite collars left the WTZ, before any data could be downloaded, but seven remaining individuals were tracked for 72.6 ± s.d. 68.4 days (range: 10–176 days). In the second phase, a further nine cats were captured during May–July 2023 along fencelines immediately outside the WTZ, fitted with a Lotek Litetrack Iridium Satellite collar (with hourly fixes sent to website for download) and released into the WTZ, where they were tracked for 35.14 ± s.e. 8.7 days (range: 4–71 days). This phase was specifically aimed at determining the fate of tagged cats, post interaction with Felixers. Thus, cats were released within a kilometre of the fenceline bordering the area where they had been captured, with Felixers being stationed at 2–3 km intervals along the inside of that fenceline.
Determining fate of cats following Felixer interactions
Cat satellite-tracking collars used in phase two sent a mortality notification with co-ordinates via email if the cat had remained motionless for >12 h. On receiving this notification, the mortality notification location was visited promptly to retrieve and inspect the cat carcass and to compare this with firing and photograph records from nearby in-field Felixer devices. Examination of carcasses focussed on the location and situation of the carcass and the presence of any remnant Felixer 1080 gel or staining of fur from the pink Rhodamine dye within. In cases where no remnant gel was visible, the stomach and liver were sampled for fluoracetate (1080) laboratory assay, performed by Department of Agriculture and Fisheries, Biosecurity Queensland, to determine whether the cat had died from 1080 poisoning.
Measuring Felixer impact on cat populations
Cat and fox activity was monitored in the WTZ and park treatments, using a remote camera array (Reconyx Hyperfire 550 and 600 cameras). The 30-camera array, stationed along vehicle tracks and fence easements, collected 3.5 million photos. Cameras were permanently mounted on steel posts on the edge of vehicle tracks (half of the cameras on tracks adjacent to a fence, the other half on tracks with no fence) at 400 mm above ground height and angled at 22° to the direction of the track. Photos were downloaded and manually tagged every 8 weeks, using RECONYX Mapview Professional ver. 3.7.2.2 (Holmen, USA). Processing of remote camera trap images excluded multiple camera activations of the same individual animal, within 10 min of the first detection. A pilot study identified that a higher density of cameras would generate an unmanageable volume of images (>100,000 per month) for processing, mostly from high macropod movements, particularly along fencelines. This unavoidable image volume would have prevented timely manual processing, which was incompatible with the need to track cat and macropod activity frequently enough to enable timely adaptive management of their populations. A further 22 Reconyx camera traps were stationed at one-way gates on the eastern boundary of the WTZ that facilitated directional exits of macropods (Pedler et al. 2021), to quantify cat movements through these fence apertures. Further details on activity indices generated through camera traps and conversion to a density estimate by using individual identification and a spatially explicit capture–mark-resight model are provided in the Supplementary material.
A linear model was used to compare the mean cat activity between the WTZ and park treatments for the last 12 months of Trial B, August 2022–August 2023. Using the base R stats package (R Core Team 2023), mean cat activity (cat detections per 100 camera trap-nights) was used as the response variable, log-transformed to meet assumptions of normality, and included treatment (WTZ, park) and month as fixed effects and an interaction between the fixed effects. The first 4 months of Trial B (April 2022–July 2022) were excluded from the analysis, to allow the cat activity in the park treatment to stabilise following removal of the five Felixers from the western boundary during the preceding Trial A. An ANOVA from the ‘car’ package CRAN: Package car see: https://cran.r-project.org/web/packages/car/index.html (Fox and Wesiberg 2019) was used to check for significance of the fixed effects and post hoc testing, using the ‘emmeans’ package (Lenth et al. 2019) to make pairwise comparisons between treatments.
Nine extra cats were satellite-collared and released into the WTZ in May–July 2023, to examine their fate following Felixer interaction. These collared cats were identifiable in camera trap images and constituted 3.9% of cat activity in the WTZ. Additionally, some collared cats climbed back out of the WTZ and were detected in the park treatment camera data, constituting 2.1%. Cat activity used in the linear model was inclusive of these collared individuals. Trial B was also punctuated with the removal of some of the Felixers to deal with cat incursions in the Thipa exclosure (Trial C1: 18 September − 8 November 2022; and C2: 1 June − 20 July 2023; Table 1).
Technical performance, efficacy and cost comparison
Throughout the study, detailed records were kept on Felixer faults, downtime, and expenses, including for unscheduled repairs or maintenance and freight. Records of staff time spent maintaining Felixers were also kept. During Trial periods B and C (Table 1), a professional shooter was engaged to assist in cat control. The shooter spent five nights inside the Thipa exclosure (Fig. 1) with a spotlight, thermal drone and thermal scope in October 2022, as well as five nights in the WTZ in each of August and October 2023. This allowed estimation of relative cost and return for effort at the given cat densities, comparing Felixers to conventional cat control methods such as shooting and trapping.
Permits and ethical approvals
This project was covered by Australian Pesticides and Veterinary Medicine Association Permit 80926v4, UNSW Animal Care and Ethics Committee Approval 21/30B, New South Wales Scientific Licence 101862 and a National Parks and Wildlife Service NSW Conservation Risk Assessment and Research Trial Approval.
Results
Firing rates for cats and non-targets
During the three trial periods, 292 cats (from 1144 passes) and one fox (from four passes) were fired at by Felixers, from total of 17,425 events in which an animal or moving object was detected and photographed by a Felixer (Fig. 2, Table 2). Overall, Felixers correctly identified 99.93% of animal passes as non-target species, including 5,519 small rodents, 1,134 birds (>35 species), 321 reptiles (5 species), and 1,369 macropods. There were no Felixer firings during 273 passes by humans or 2,389 passes by vehicles. Of the 11 total non-target firing events (Table 2), nine involved kangaroos (8 from 1,191 passes of red kangaroos and 1 from 39 passes of euros). All were moving slowly on all fours, triggering both lateral LiDAR sensors. Two further non-target firing events involved a rolling tumbleweed during a dust storm (1 from 3,527 inanimate object passes) and one greater bilby (from 163 bilby passes), during Trial C, when the Felixer was set in standard mode and the ground surface in front of the unit had been altered through track dragging to monitor for cat spoor. The incidence of non-target firing events was higher when the standard algorithm was operating, with 2.25% of kangaroo passes resulting in a fire event (5 from 222 passes), compared to 0.41% (4 from 979 passes) under the conservative algorithm. Non-target firing events were restricted to Models 3.0 and 3.1 Felixers, which used the LiDAR discriminatory algorithms. The Felixer 3.2 Model with artificial-intelligence discriminator did not produce any non-target firing events during the relatively shorter period that this model was trialled (601 trap nights, vs Model 3.0: 3,665 trap-nights, seven non-target firings; Model 3.1: 1,058 trap-nights, four non-target firings).
From top left to bottom right: a Felixer grooming trap under shade shelter used in the trial; images taken by onboard Felixer cameras, including images of cats (middle and right top), showing LiDAR sensors (white dots); non-target images or moving objects (16,280) included >50 species with the following examples: dusky hopping-mouse (middle left), greater bilby (middle), Australian raven (middle right), golden bandicoot (bottom left), red kangaroo (bottom middle), central bearded dragon (bottom right).
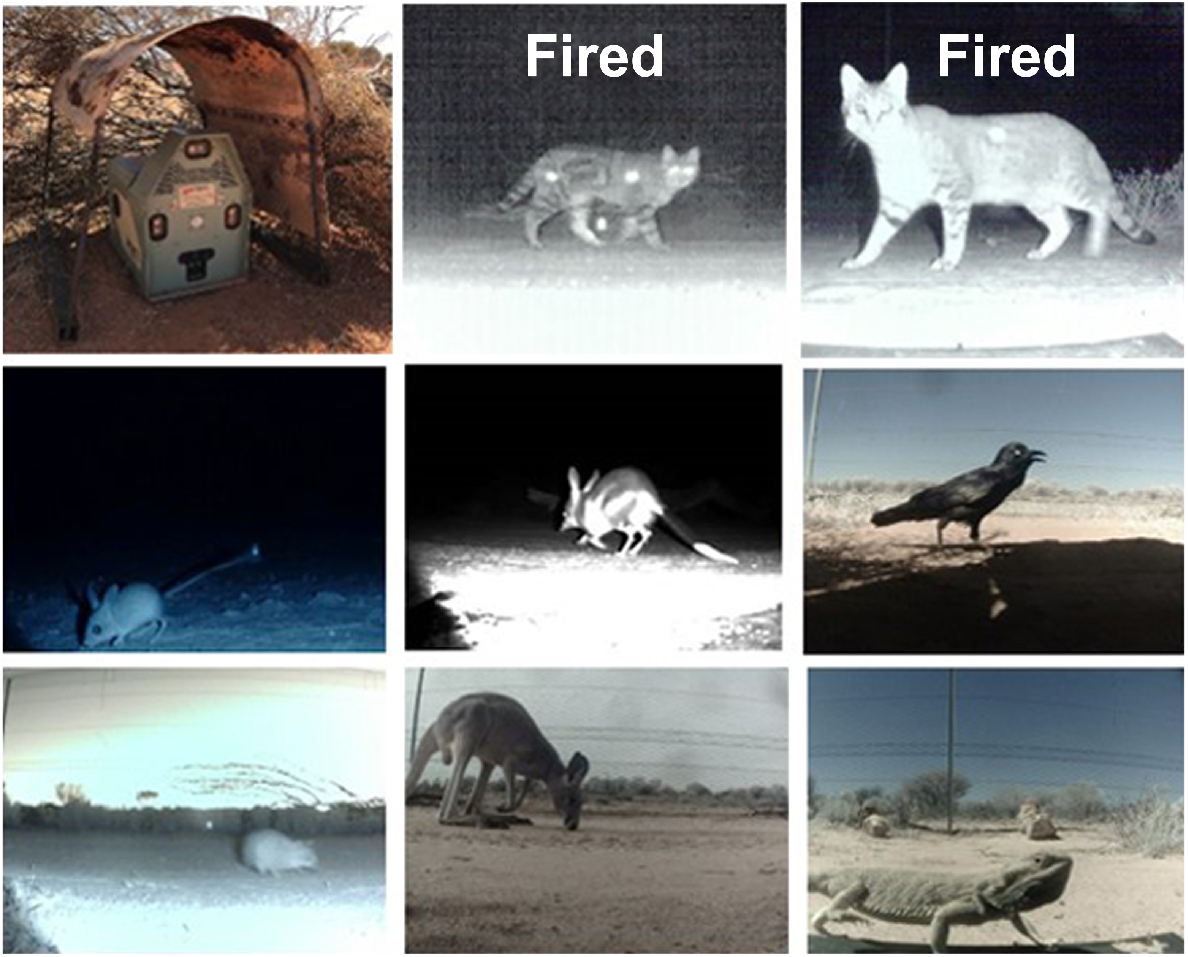
Order/family | Species/order | Number of detections (fire events) | ||||
---|---|---|---|---|---|---|
Trial A | Trial B | Trial C | Total | |||
Mammals | Feral cat (Felis catus) | 364 (101) | 773 (190) | 7 (1) | 1,144 (292) | |
Red fox (Vulpes vulpes) | 2 (1) | 2 | 0 | 4 (1) | ||
Dingo (Canus lupus dingo) | 35 | 17 | 0 | 52 | ||
Short-beaked echidna (Tachyglossus aculeatus) | 3 | 2 | 0 | 5 | ||
Euro (Osphranter robustus) | 12(1) | 27 | 0 | 39 (1) | ||
Red kangaroo (Osphranter rufus) | 384 (4) | 807 (4) | 0 | 1,191 (8) | ||
Western grey kangaroo (Macropus fuliginosus) | 8 | 29 | 0 | 37 | ||
Kangaroo (Macropodidae) species | 48 | 63 | 0 | 111 | ||
Crest-tailed mulgara (Dasycercus cristicauda) | 15 | 1 | 4 | 20 | ||
Greater bilby (Macrotis lagotis) | 0 | 1 | 163 (1) | 164 (1) | ||
Golden bandicoot (Isoodon auratus) | 0 | 91 | 91 | |||
Bat (Chiroptera) species | 2 | 17 | 0 | 19 | ||
Dusky Hopping-Mouse (Notomys fuscus) | 1,455 | 475 | 92 | 2,022 | ||
European Rabbit (Oryctolagus cuniculus) | 442 | 203 | 0 | 645 | ||
Mouse (Muridae) species | 1,584 | 1,448 | 465 | 3,497 | ||
Feral pig (Sus scrofa) | 6 | 0 | 6 | |||
Feral goat (Capra hircus) | 2 | 0 | 2 | |||
Birds | Bird (Aves) 35+ species | 524 | 536 | 74 | 1,134 | |
Reptiles | Central bearded dragon (Pogona vitticeps) | 37 | 178 | 44 | 259 | |
Dragon (Agamidae) species | 1 | 1 | 1 | 3 | ||
Gould’s goanna (Varanus gouldii) | 17 | 27 | 12 | 56 | ||
Snake (Serpentes) species | 1 | 1 | 2 | |||
Woma python (Aspidites ramsayi) | 1 | 0 | 1 | |||
Arthropods | Insect species | 25 | 26 | 6 | 57 | |
Human | Human | 70 | 129 | 74 | 273 | |
Vehicle | Vehicle | 1,020 | 1,025 | 344 | 2,389 | |
No obvious animal | e.g. wind-blown debris, moving vegetation | 1,620 | 1,435 (1) | 472 | 3,527 (1) | |
Overexposed image | Overexposed image | 23 | 12 | 35 | ||
Unknown animal | Unknown animal | 462 | 139 | 39 | 640 | |
Total | 8,140 | 7,384 | 1,901 | 17,425 (304) |
The number of animals fired at is given in parentheses.
Optimisation of Felixer deployment strategies
Cat activity on Felixer images and camera-traps in the WTZ and park treatments during Trial A indicated that cat activity along access tracks along predator-proof fencelines was higher than cat activity along tracks with no adjacent fenceline. Felixers on management tracks adjacent to fencelines in the park treatment achieved 10.5 cat fire events per 100 trap nights, compared with 2.0 fire events per 100 trap nights for units on management tracks, away from fencelines. Camera-array data from remote camera traps adjacent to fences in the WTZ recorded a mean of 25.2 (range 3.9–62.1, s.e. 1.9) detections per month versus 11.3 (range 0–29.6, s.e. 1.4) detections per month for cameras on management tracks away from fencelines. In contrast, there was no evidence that movements of the seven satellite-collared cats in the WTZ were biased towards fencelines. The area within 100 m of fencelines constituted approximately 9.5% of the total area of the WTZ, closely matching proportional night-time satellite fixes of 11.2% for the tagged cats.
Fate of cats following Felixer interactions
There were nine instances in which satellite-tagged cats in the WTZ were fired at by Felixers during Trial B, with six dying post-firing and the remaining three surviving. Tracking collar and website malfunctions led to issues with mortality notifications or loss of data for two individuals. However, satellite tracking records and location of carcasses suggested that these cats that died were motionless at a mean of 3.36 h (n = 4, range 1.5–7.0 h) post-firing, with a mean distance of 425 m (n = 6 range, 230–700 m) from the Felixer (Table S2). All cat carcasses were on the surface, with some under vegetation, and all accessible to vertebrate scavengers. Three of the six collared cats that died post-firing did not have Felixer gel (or associated pink staining) visible on their fur at retrieval (Fig. 3), but laboratory assays of stomach and liver samples detected 1080. Of the three instances where cats did not die post-firing, there were two identified causes. First, there was a Felixer mechanical fault stopping the solenoid/trigger latch from immediately releasing the firing spring, causing slow release of the gel, which failed to reach the cat. Second, a software fault caused a 60-ms delay in firing, resulting in gel not reaching the cat. For the third, an unknown fault was responsible. Two of the three cats not killed by their first interaction were ultimately killed by a second interaction when a Felixer successfully fired. Three additional uncollared dead cats were found with diagnostic traces of pink Felixer gel on their fur during Trials A and B (Supplementary Fig. S1). Identification through matching of pelage patterns and Felixer records suggested that they had died within 250–1,300 m of the Felixer that fired at them.
Photographs of one of nine cats satellite collared in the wild training zone and then fired at by a Felixer: (a) the cat under anaesthesia during satellite collar fitting; (b) the image taken by the Felixer on firing, 2 days post-release and; (c) the body of the cat about 250 m from the Felixer, with diagnostic pink Rhodamine gel remnants on right torso.
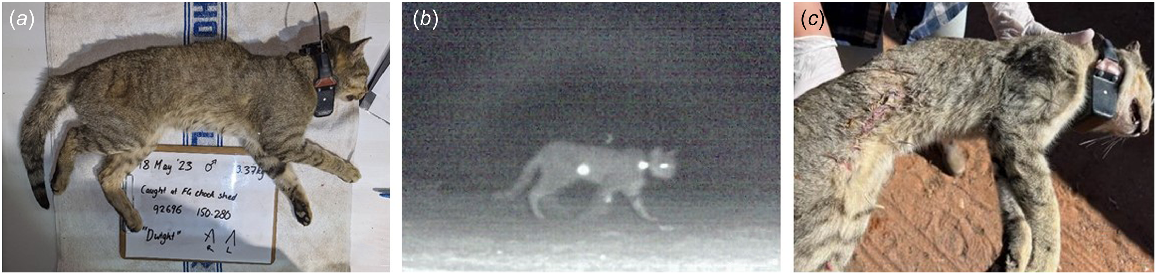
Of the 190 cats fired at during Trial B, there were 24 known instances where the cat did not die (including data from collared cats above). Eighteen of these instances were due to user error rather than a Felixer fault, where insufficient 1080 capsules were loaded in the magazine slots, producing a loud noise from the firing mechanism but no expulsion of gel. A satellite collared male cat (ID 33134) was exposed to such an event and, subsequently, radio tracked for another 9 months but never photographed or fired at by another Felixer, despite the satellite points indicating his proximity to Felixer units. Another satellite collared cat (adult male, ID 33142) was wearing a functional satellite collar when photographed and fired at by a Felixer (v 3.0) with a trigger latch fault in May and July 2022, but was subsequently observed alive following these events. After the satellite collar had expired, this cat was identified in remote camera trap and Felixer images before it was fired at by another Felixer (v 3.1) in August 2022. It was then not seen again on camera for over a year, before it was again detected alive on remote camera in February 2024. This cat was fired at by a Felixer for a fourth time (v 3.2 AI) in May 2024, after which its carcass and expired tracking collar were discovered 510 m from the Felixer, 3 weeks post firing.
Felixer impact on cat populations
During Trial B, cat activity from camera traps and track counts was consistently lower inside the WTZ than in unbounded park area outside (Fig. 4), with the exception of July 2023, when some Felixers were removed for Trial C and also nine satellite-collared cats had been added to the WTZ to assess their fate following Felixer interaction. A linear model for the period August 2022 – August 2023 showed a significant overall effect of ‘treatment’ on cat activity during the Trial B period (F = 23.920, P < 0.001) (Table S3). Cat activity outside the fence was significantly higher than in the WTZ during the Trial B period (T-ratio = 6.315, P < 0.001) (Table S3), averaging 45.7 ± s.d. 16.8 detections/100 trap nights compared with 19.1 ± s.d. 6.21 in the WTZ. There was also a significant effect of ‘month’ on cat activity (F = 5.077, P < 0.035), but no significant interaction between ‘treatment’ and ‘month’ (Table S3). Spatially explicit capture–mark–resight models suggested that mean density of cats in the WTZ was 0.35 (95% CI 0.26–0.47) cats/km2 (Table S4) during the 4-week period in late 2021, for a given cat detection rate of 15–26 detections per 100 trap nights.
Monthly cat activity (from camera array of 30 cameras), in the wild training zone (orange) and unbounded outside park (green) treatments (see Fig. 1) and monthly rainfall (blue bars) during three trial periods, in relation to small mammal prey activity from track counts across all treatments (grey).
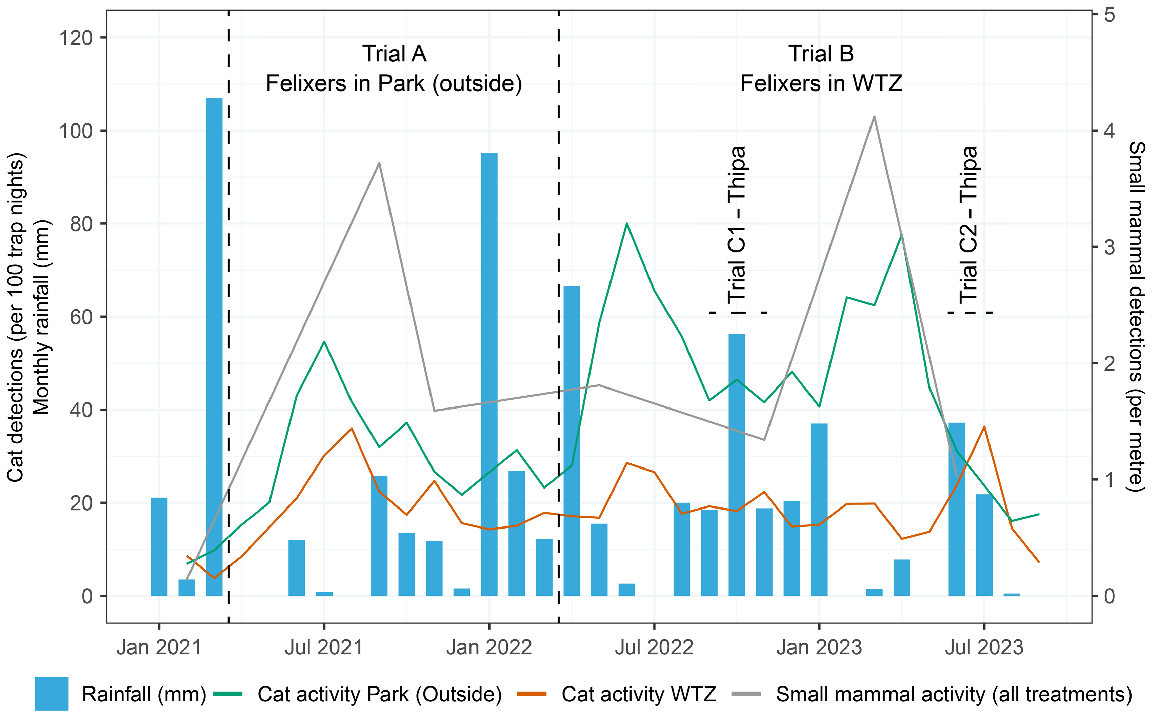
During Trial C, Felixers were twice moved into the cat- and fox-free safe havens to remove a cat that had breached the fence. Trial C1 targeted this small feral cat in the Thipa exclosure, with five Felixers along with 210 cage trap nights, 540 leghold trap-nights, and 15 nights of spotlighting with the aid of thermal scope and drone. Daily tracking over a 5-week period showed that the cat actively avoided the Felixers on several occasions. The cat was additionally detected and photographed by a Felixer six times but not successfully fired at until the seventh pass. Absence of firing may have been due to the young cat’s small size, requiring height adjustment of the sensors for better targeting. The cat was killed by the Felixer 30 days after it was detected within the exclosure. During Trial C2, a second cat entered the Thipa safe haven, requiring deployment of five Felixers over 7 weeks. This cat was not detected again inside the exclosure after a close encounter with a conservation detector dog. The cat’s tracks from the day after this encounter suggested it probably climbed back out of the exclosure. Although Felixers were left in situ for 7 weeks as a precaution, there was no cat interaction or detections.
Technical performance
A progression of Felixer models was used during the trials with successive models designed to attempt to rectify previous faults. Three different Felixer models were compared in relation to the percentage of cats that they fired at, under the different target discrimination algorithms. While set to the conservative algorithm based on LiDAR sensors, Felixer Models 3.0 and 3.1 fired at 20.2% (n = 728) and 22.6% (n = 155) of cat passes respectively. This firing rate increased to 40.0% (n = 155) and 42.6% (n = 68) with the use of the standard LiDAR algorithm. The new generation Model 3.2 Felixers, with the initial artificial intelligence discrimination algorithm, fired at 43.9% (n = 123) of cats.
Felixers incurred a range of hardware and software technical faults across all three trial periods (Table 2), resulting in manufacturer improvements in design or firmware upgrades. Devices often had to be returned to the manufacturer for diagnosis and repair (all units used in the trial returned at least once, some multiple times), compounding downtime, given the remote location of the study site. Overall, the three Felixer models trialled had similar mean downtime, 14.77–17.75% (Table 3). Model 3.0 units had ongoing trigger latch and solenoid seizures and faults. These improved with software upgrades, which allowed the device to manually agitate these components on start-up to lubricate them. The substitution of graphite for oil-based lubricants also reduced such faults in the arid dusty environment. Models 3.1 and 3.2 did not suffer trigger latch issues, but had high incidences of charging errors, some being related to faulty Anderson plug connectors, internal charging chip components, and solar panels. These issues were improved during the study, with iterative adaptive management in collaboration with the manufacturer.
Model | Serial ID | Available trap nights | Trap nights lost | % trap nights lost | Non-target fire events | Mean % downtime per model (s.e.) | Faults causing downtime | |
---|---|---|---|---|---|---|---|---|
3.0 | SP030013 | 917 | 255 | 27.8 | 3 | 17.75 (4.9) | – Trigger latch and trigger solenoid seizures/faults – Charging and hibernation issues – Premature battery failure | |
SP030014 | 821 | 187 | 22.8 | 2 | ||||
SP030019 | 917 | 219 | 23.9 | 1 | ||||
SP030044 | 176 | 0 | 0 | 1 | ||||
SP030048 | 834 | 119 | 14.3 | |||||
Totals | 3,665 | 7 | ||||||
3.1 | SP030125 | 505 | 64 | 12.7 | 1 | 16.91 (4.2) | – Faulty charging connectors/internal charging chip/solar panels | |
SP030185 | 553 | 117 | 21.2 | 3 | ||||
Totals | 1,058 | 4 | ||||||
3.2 | SP030213 | 92 | 0 | 0 | 14.77 (12.8) | – Charging issues related to faulty solar panels – Faulty charging connectors/internal charging chip | ||
SP030217 | 92 | 0 | 0 | |||||
SP030279 | 139 | 7 | 5.0 | |||||
SP030280 | 139 | 7 | 5.0 | |||||
SP030281 | 112 | 88 | 78.6 | |||||
SP030306 | 27 | 0 | 0 | |||||
Totals | 601 | 0 |
Efficacy and cost comparison
Cat removal using Felixers and shooting had similar costs. The cash and staff time costs of Felixer usage during Trial B was estimated at AUD112,997 or AUD219 per day. The estimated cost per cat removed for Felixers in July 2023 (24 cats fired at) was AUD274 and in August (11 cats fired at) was AUD597. In comparison, at a similar cat population density, an intensive five-night shooting campaign in August 2023 by a professional shooter (aided by thermal scope and drone) totalled AUD11,400, representing a cost of AUD299 per cat. A second five-night shooting operation, at lower cat density in October 2023, cost AUD571 per cat. During Trial C1 when a cat incursion occurred in the Thipa exclosure an estimated AUD30,000 was invested in staff time in setting and checking traps (including cage, leghold and Felixers), and shooting with the aid of a thermal scope and drone compared with AUD8,210 for the Felixer deployments which, despite not firing until the seventh interaction, were ultimately successful.
Discussion
This study represents the most comprehensive field trial and evaluation of Felixer grooming traps, in terms of the geographic scale, timespan, management context and number of cats encountered. Importantly, Felixers had extremely low non-target rates and fired at 20–44% of the cats they encountered, boosted by optimising their placement and upgrading discriminatory mechanisms during the life of the trial. Overall, Felixers performed well as a management tool in the semi-bounded 10,400 ha landscape-scale area over a 12-month period. Felixers also resolved one of two incursions by single cats into a 2,000 ha feral-free safe-haven area. Challengingly, the trial mainly used prototype or early commercial model of Felixers and, thus, there was a high incidence of technical faults and failures resulting in significant downtime, which required additional investment of staff time for fault diagnosis and repairs.
Firing rates for cats and non-targets
With >99.9% target accuracy in both conservative and standard LiDAR discrimination algorithms and 100% for artificial intelligence units, there were only 10 incidences (0.03%) of firings at non-target animals (eight red kangaroo, one euro, one bilby). These animals are unlikely to have suffered any negative effects, given their high tolerance to 1080. An average 25 kg female and 55 kg male red kangaroo (Strahan 2023) would need to consume the contents of 10 and 22 × 8 mg cartridges respectively to reach LD50 (APVMA 2008). Similarly, an average 1.1 kg adult bilby would need to consume the contents of 1.5 × 8 mg cartridges to reach the LD50 (APVMA 2008). Any non-target exposure to 1080 is undesirable but Felixers represent a safe and targeted feral-predator control tool in this environment, given the extremely low rates of, and effects on, non-target species.
Target specificity of Felixers exceeded other 1080 delivery methods used at the study site and across Australia. These include 1080 meat baits for foxes and wild dogs/dingoes, distributed through ground and aerial baiting (Fleming et al. 2014). In a recent study at the same site in Sturt National Park, none of the 1080 meat baits laid for foxes and wild dogs/dingoes was consumed by or interacted with by these target species, with birds removing 57.5% of baits, transporting them across management boundaries and into unbaited zones (Rudder 2023). Feral cat baiting also has low target specificity. Across Queensland sites, feral cats consumed just 3.1% of Eradicat™ baits laid, with seven non-target mammals and four non-target bird species consuming enough 1080 bait for a lethal dose (Fancourt et al. 2022). Bait uptake by native non-target species averaged between 14% and 57% of Eradicat baits at Arid Recovery, South Australia (Moseby et al. 2011). Similarly, several studies have demonstrated that only a small percentage of meat baits laid for dingoes/wild dogs or foxes are actually consumed by them, with most being removed or consumed by native non-target species (Dundas et al. 2014; Kreplins et al. 2018; Augusteyn and Nolan 2022).
Optimisation of Felixer deployment strategies
The tendency of cats to follow netting fencelines was likely to be a major factor boosting cat encounter rates with Felixers. This was reflected in detection rates from Felixers and camera traps and may be the result of the tendency for cats (Read et al. 2015), like other mammalian predators, to follow landscape features or barriers (Whittington et al. 2005; McKenzie et al. 2012; Moseby and McGregor 2022). Concentrated movement along fencelines probably also reflected feral cats targeting native rodents moving from the safe havens into adjacent areas (McGregor et al. 2020; Moseby et al. 2020b). Many observations were recorded during spotlighting and camera trap analysis of cats stalking dusky hopping mice and other small mammals along fencelines, a behaviour not observed elsewhere within the study site. This behaviour was most pronounced during March–June 2023, when small mammal populations had increased dramatically inside the safe havens. However, concentration along fencelines was not evident from cat satellite-tracking data, possibly owing to the coarse analysis, rather than detailed activity data. This lack of activity bias of tracked cats towards fencelines in late 2021 was before small mammals had increased inside and, hence, were less likely to attract cats to the boundaries of the exclosure.
The proportion of cats fired at by Felixers varied among models and discrimination algorithms, with a major improvement by using standard LiDAR algorithm and then later, the artificial intelligence processor. The latter is likely to improve with continued retraining of the identification algorithm with more data. Cat identification rates for Model 3.0 and 3.1 LiDAR algorithm units in both conservative (20–23%) and standard (40–43%) algorithms were lower in this study than most published data from Felixer trials at other Australian sites, with 47% in Rickards et al. (2023), 77% in Moseby et al. (2020a), 59% in Hodgens (2019, unpubl. data), and 82% in Read et al. (2019). This may be explained by changes to the Felixer software enacted following these studies, making the discrimination software more conservative. Firmware for the early Felixer models, using the LiDAR discrimination algorithms, was necessarily a trade-off between optimising the proportion of cats fired at, and minimising non-target firings. Some cats of a size and speed that resembled non-targets were not fired at. Others that were moving at an angle or too fast to reliably deposit the toxin on their flank were also blocked from firing. Had these previous studies used the same software version(s) as used in these trials, it is likely that the percentage of cats fired at would have been similarly lower. Given the extremely low rates of non-target firing, there is considerable opportunity to operate Felixers in standard LiDAR algorithm or with artificial intelligence identifier in arid habitats where cat-sized non-target species are absent.
Felixer impact on cat populations
Not every cat fired at by Felixers was killed during the study, on the basis of opportunistic observations of individually identifiable cats and satellite-collared individuals. Many of the known causative technical errors were resolved by hardware or software improvements from feedback provided to the Felixer manufacturer, but it is anticipated that a further unknown proportion of cats survive Felixer firing either through the gel failing to reach the cat or the cat failing to ingest it. Previous Felixer pen trials showed that six of seven cats died from 1080 poisoning following firing by a Felixer (Read et al. 2014; Read et al. 2019) and an unpublished field trial also demonstrated the death of all eight satellite-collared cats fired at by Felixers (Hodgens 2019, unpubl. data). Thus results from this field trial are important in providing alternative data from an arid zone control site, using later Felixer models and software.
Despite not all cats being killed by their interaction with Felixers, the devices appear to have reduced cat activity in the extensive WTZ relative to the unbounded area outside and effectively resolved one of the two cat incursions into the large feral-free safe haven. However, it should be noted that it was difficult to confidently assess the suppressive effect of Felixers on cat activity in the semi-bounded WTZ, given the inherent real-world challenges with trials at such scale. Given the logistical and financial limitations of replication, just one semi-bounded area was compared with an adjacent unbounded area and the cat activity and other intrinsic site characteristics could not be controlled for. The two areas had unknowable cat incursion rates and were not strictly independent of one another, particularly given the initial trials of Felixers in the unbounded area during Trial A, which potentially affected its suitability as a null treatment area during Trial B.
The use of a cat activity index from remote-camera traps as a proxy for cat density is imperfect, given effects of weather, food availability, social interactions, and camera presence on cat behaviour (Meek et al. 2015). However, the density estimates derived from individual identification of cats and spatially explicit capture mark resighting model also had several disadvantages. Most cat photos were indistinguishable from one another, given the high proportion of indistinct grey tabbies, making individual identification challenging and time consuming (Sparkes and Fleming 2023). This issue is presumably more prominent in wild environments, with natural selection drivers in morphology, than in urban environments where cat coat colour, coat length, breed and other features are more diverse (Cove et al. 2023). Capture mark resight models also did not adequately deal with the semi-bounded nature of the WTZ and the unknown extent of habitat use outside it, given that an assumption of such models was that the animals did not move outside this area. In addition to such technical limitations, a logistical and management drawback was that these density estimates could not be calculated in real time, rendering them inadequate for expedient adaptive management of the cat population (i.e. intensifying cat control in response to population or activity increases).
Satellite-collared cats that died from Felixer interaction were motionless within 1.5–7 h and moved 230–700 m from the Felixer. Some uncollared cats found dead with remnant traces of Felixer gel are thought to have travelled 250–1300 m. Thus caution needs to be used when deploying any 1080 control methods near dwellings or public areas. There is also a need to conduct testing of the residual 1080 in and on cat carcasses following death from Felixer firing.
Some cats in this study displayed aversion to Felixers, including examples of individuals that had either been scared by Felixers that either misfired or fired without a cartridge loaded. Another example included the incursion cat in the Thipa treatment that was intensively targeted by a range of control techniques. Such observations are consistent with trials specifically testing cat aversion to Felixers, which recorded initial aversion to Felixers by up to 40% of cats that had been captured and satellite collared, being roughly double the initial aversion rates of non-collared cats (Ryan-Schofield et al. 2024). This highlights the need for concealing traps and Felixers where possible, minimising the risk of scaring cats with misfires. Given that individual feral cats are likely to demonstrate aversion to spotlight shooting, trapping or baiting, these results demonstrate the value of integrating Felixers with a suite of other cat-control tools.
Technical faults and efficacy of Felixers
Due to the trial occurring during a research and development phase, a high incidence of technical faults and failures at times made Felixers time and resource intensive. Although Felixers are designed as autonomous devices, they were under continuous refinement and required frequent checking and troubleshooting by trained personnel to ensure reliable function. As such, although they are still being refined and optimised, Felixers are currently best suited to high-value conservation sites where sufficient personnel and resources are in place for their regular management. A reduction in the incidence of technical faults leading to downtime, and improvement in the image quality of photographs captured by Felixers, are key improvements that would make Felixers more effective field tools. Indeed, improvements over the life of this trial led to 11 successive software upgrades to resolve technical issues and improve cat firing rates, reducing non-target firings. Bespoke products, manufactured in small production runs without tooling, are inherently expensive (Tuck and Hague 2006). Potential future mass production associated with increased demand and improved manufacturing of Felixers or similar products, could potentially lower the cost per unit.
The cost per cat removed by Felixers was similar to that of a professional shooting contractor, at the same site and with similar activity index and population age structure. Felixers had the advantage of continuous deployment year-round, in all weather conditions. One of the two single cat incursions within the feral-free safe haven was ultimately resolved by a Felixer, demonstrating their potential utility for such situations as part of an integrated approach. However this information should be interpreted with caution. Given the simultaneous and interactive effects from multiple methods to remove the incursion cat, it is difficult to assess the efficacy of Felixers in comparison to other methods. Similarly, an acknowledged limitation of comparisons between shooting and Felixers during Trial B, is that they were used at the same site on the same cat population simultaneously and thus their non-independent, interactive effects are impossible to untangle. Cat control methods vary in their effectiveness and cost per individual depending on cat population size, seasonal conditions and food availability, with cats being easier to trap or bait during food resource shortages (Moseby et al. 2011). Moreover, the skill and experience of field practitioners substantially influences effectiveness. Thus, calculations of cost per cat killed are somewhat useful, but remain a simplistic measure of the efficacy of tools used separately or synergistically to reduce feral cat predation, given variability in behaviours of individual cats through time.
Conclusions
Successful pest management and eradication campaigns need a suite of tools to target individuals with different behaviours or learned aversions and to operate effectively in different conditions and circumstances. During this study, Felixers were a highly target-specific and safe poison delivery mechanism for feral cats. Reduction in cost and increased reliability will improve their utility for practitioners, focused on conservation outcomes in the field. Their use will need to be supported by an integrated pest management approach, given the challenges of controlling cats.
Conflicts of interest
John Read declares that he is the founder, voluntary CEO and a shareholder of Thylation Operations Pty Ltd, the developers of Felixers. Katherine Moseby declares that she is a shareholder of Thylation Operations Pty Ltd, the developers of Felixers, through her role as a director of Ecological Horizons. These issues were proactively managed during this study through those with a declared COI being involved in study design, monitoring and assisting in manuscript preparation, but not involved in data collection or analysis associated directly with Felixers. In particular, they did not have final editorial control. Felixers used in this project were either purchased or commercially leased from Thylation. All other authors declare no conflicts of interest.
Declaration of funding
Funding for this study was provided by the New South Wales Environmental Trust, the Centre for Ecosystem Science, UNSW Sydney, donations to UNSW Philanthropy and the New South Wales Department of Climate Change, Energy, the Environment and Water through the Wild Deserts Feral Predator Free Project Partnership.
Acknowledgements
We acknowledge the Wadigali, Wongkumara and Maljangapa traditional owners of the land on which this study took place. We thank Sharon Ryall for significant logistical and administrative support and NSW National Parks and Wildlife Service staff Jaymie Norris, Dan Hough, David Rudder, Tanya Leary and Ryan Duffy for facilitating and supporting this work in Sturt National Park. Thylation staff and contractors Andrew Burnard, Millicent Russell and Conor Barry are thanked for technical and administrative assistance relating to Felixers.
References
Algar D, Angus GJ, Williams MR, Mellican AE (2007) Influence of bait type, weather and prey abundance on bait uptake by feral cats (Felis catus) on Peron Peninsula, Western Australia. Conservation Science Western Australia 6(1), 109-149.
| Google Scholar |
Augusteyn J, Nolan B (2022) Evaluating methods for controlling feral cats that minimise non-target impacts at Taunton National Park (Scientific). Ecological Management & Restoration 23(1), 43-52.
| Crossref | Google Scholar |
Chambers B, Dunlop J, Wayne A (2020) Felixer™ grooming trap non-target safety trial: Numbats. Unpublished report. Department of Biodiversity, Conservation and Attractions, Perth, WA, Australia. Available at https://library.dbca.wa.gov.au/#record/153154
Comer S, Clausen L, Cowen S, Pinder J, Thomas A, Burbidge AH, Tiller C, Algar D, Speldewinde P (2020) Integrating feral cat (Felis catus) control into landscape-scale introduced predator management to improve conservation prospects for threatened fauna: a case study from the south coast of Western Australia. Wildlife Research 47(8), 762-778.
| Crossref | Google Scholar |
Cove MV, Herrmann V, Herrera DJ, Augustine BC, Flockhart DTT, McShea WJ (2023) Counting the capital’s cats: estimating drivers of abundance of free-roaming cats with a novel hierarchical model. Ecological Applications 33(2), e2790.
| Crossref | Google Scholar | PubMed |
Doherty TS, Dickman CR, Johnson CN, Legge SM, Ritchie EG, Woinarski JCZ (2017) Impacts and management of feral cats Felis catus in Australia. Mammal Review 47(2), 83-97.
| Crossref | Google Scholar |
Dundas SJ, Adams PJ, Fleming PA (2014) First in, first served: uptake of 1080 poison fox baits in south-west Western Australia. Wildlife Research 41(2), 117-126.
| Crossref | Google Scholar |
Fancourt BA, Harry G, Speed J, Gentle MN (2022) Efficacy and safety of Eradicat® feral cat baits in eastern Australia: population impacts of baiting programmes on feral cats and non-target mammals and birds. Journal of Pest Science 95(1), 505-522.
| Crossref | Google Scholar |
Fleming PJS, Allen BL, Allen LR, Ballard GA, Bengsen AJ, Gentle MN, McLeod LJ, Meek PD, Saunders GR (2014) Management of wild canids in Australia: free-ranging dogs and red foxes. In ‘Carnivores of Australia: past, present and future’. (Eds AS Glen, CR Dickman) pp. 105–149. (CSIRO Publishing: Melbourne, Vic, Australia)
Kingsford RT, West RS, Pedler RD, Keith DA, Moseby KE, Read JL, Letnic M, Leggett KEA, Ryall SR (2021) Strategic adaptive management planning—restoring a desert ecosystem by managing introduced species and native herbivores and reintroducing mammals. Conservation Science and Practice 3(2), e268.
| Crossref | Google Scholar |
Kreplins TL, Kennedy MS, Adams PJ, Bateman PW, Dundas SD, Fleming PA (2018) Fate of dried meat baits aimed at wild dog (Canis familiaris) control. Wildlife Research 45(6), 528-538.
| Crossref | Google Scholar |
Legge S, Woinarski JCZ, Dickman CR, Doherty TS, McGregor H, Murphy BP (2020) Cat ecology, impacts and management in Australia. Wildlife Research 47(8), i-vi.
| Crossref | Google Scholar |
Lenth R, Singmann H, Love J, Buerkner P, Herve M (2019) Emmeans: estimated marginal means, aka least squares means. (R-package 1.4.2). Available at https://cran.r-project.org/web/packages/emmeans/index
Lohr CA, Algar D (2020) Managing feral cats through an adaptive framework in an arid landscape. Science of The Total Environment 720, 137631.
| Crossref | Google Scholar | PubMed |
McGregor H, Read J, Johnson CN, Legge S, Hill B, Moseby K (2020) Edge effects created by fenced conservation reserves benefit an invasive mesopredator. Wildlife Research 47(8), 677-685.
| Crossref | Google Scholar |
McKenzie HW, Merrill EH, Spiteri RJ, Lewis MA (2012) How linear features alter predator movement and the functional response. Interface Focus 2(2), 205-216.
| Crossref | Google Scholar | PubMed |
Medina FM, Bonnaud E, Vidal E, Tershy BR, Zavaleta ES, Josh Donlan C, Keitt BS, Le Corre M, Horwath SV, Nogales M (2011) A global review of the impacts of invasive cats on island endangered vertebrates. Global Change Biology 17(11), 3503-3510.
| Crossref | Google Scholar |
Meek PD, Ballard G-A, Fleming PJS (2015) The pitfalls of wildlife camera trapping as a survey tool in Australia. Australian Mammalogy 37(1), 13-22.
| Crossref | Google Scholar |
Moseby KE, McGregor HM (2022) Feral cats use fine scale prey cues and microhabitat patches of dense vegetation when hunting prey in arid Australia. Global Ecology and Conservation 35, e02093.
| Crossref | Google Scholar |
Moseby KE, Read JL, Galbraith B, Munro N, Newport J, Hill BM (2011) The use of poison baits to control feral cats and red foxes in arid South Australia II. Bait type, placement, lures and non-target uptake. Wildlife Research 38(4), 350-358.
| Crossref | Google Scholar |
Moseby KE, Blumstein DT, Letnic M (2016) Harnessing natural selection to tackle the problem of prey naïveté. Evolutionary Applications 9(2), 334-343.
| Crossref | Google Scholar | PubMed |
Moseby KE, McGregor H, Read JL (2020a) Effectiveness of the Felixer grooming trap for the control of feral cats: a field trial in arid South Australia. Wildlife Research 47(8), 599-609.
| Crossref | Google Scholar |
Moseby KE, McGregor H, Hill BM, Read JL (2020b) Exploring the internal and external wildlife gradients created by conservation fences. Conservation Biology 34(1), 220-231.
| Crossref | Google Scholar | PubMed |
Moseby K, Hodgens P, Bannister H, Mooney P, Brandle R, Lynch C, Young C, Jansen J, Jensen M (2021) The ecological costs and benefits of a feral cat poison-baiting programme for protection of reintroduced populations of the western quoll and brushtail possum. Austral Ecology 46(8), 1366-1382.
| Crossref | Google Scholar |
Newsome AE, Catling PC, Cooke BD, Smyth R (2001) Two ecological universes separated by the Dingo Barrier Fence in semi-arid Australia: interactions between landscapes, herbivory and carnivory, with and without dingoes. The Rangeland Journal 23(1), 71-98.
| Crossref | Google Scholar |
Nogales M, Vidal E, Medina FM, Bonnaud E, Tershy BR, Campbell KJ, Zavaleta ES (2013) Feral cats and biodiversity conservation: the urgent prioritization of island management. BioScience 63(10), 804-810.
| Crossref | Google Scholar |
Pedler RD, West RS, Read JL, Moseby KE, Letnic M, Keith DA, Leggett KD, Ryall SR, Kingsford RT (2018) Conservation challenges and benefits of multispecies reintroductions to a national park – a case study from New South Wales, Australia. Pacific Conservation Biology 24(4), 397-408.
| Crossref | Google Scholar |
Pedler R, Read J, Moseby K, Kingsford R, West R (2021) Proactive management of kangaroos for conservation and ecosystem restoration – Wild Deserts, Sturt National Park, NSW. Ecological Management & Restoration 22(S1), 90-98.
| Crossref | Google Scholar |
Read J, Gigliotti F, Darby S, Lapidge S (2014) Dying to be clean: pen trials of novel cat and fox control devices. International Journal of Pest Management 60(3), 166-172.
| Crossref | Google Scholar |
Read JL, Bengsen AJ, Meek PD, Moseby KE (2015) How to snap your cat: optimum lures and their placement for attracting mammalian predators in arid Australia. Wildlife Research 42(1), 1-12.
| Crossref | Google Scholar |
Read JL, Bowden T, Hodgens P, Hess M, McGregor H, Moseby K (2019) Target specificity of the felixer grooming “trap”. Wildlife Society Bulletin 43(1), 112-120.
| Crossref | Google Scholar |
Rickards H, Read JL, Johnson CN, Jones ME, Pauza MD, Bentley J, Sculthorpe A, Humphrey M, Hamer R (2023) Is the Felixer cat control device safe for marsupial carnivores? Wildlife Research 50(5), 356-365.
| Crossref | Google Scholar |
Ryan-Schofield NL, Read JL, McGregor HW, Mcwhorter TJ, Moseby KE (2024) Evasive invasives? Implications of neophobia for feral cat (Felis catus) control. Wildlife Society Bulletin 48(3), e1536.
| Crossref | Google Scholar |
Sparkes J, Fleming PJS (2023) Observer differences in individual identification of feral cats from camera trap images. Australian Mammalogy 45(1), 32-40.
| Crossref | Google Scholar |
Tuck C, Hague R (2006) The pivotal role of rapid manufacturing in the production of cost-effective customised products. International Journal of Mass Customisation 1(2/3), 360-373.
| Crossref | Google Scholar |
West R, Letnic M, Blumstein DT, Moseby KE (2018) Predator exposure improves anti-predator responses in a threatened mammal. Journal of Applied Ecology 55(1), 147-156.
| Crossref | Google Scholar |
Whittington J, St. Clair CC, Mercer G (2005) Spatial responses of wolves to roads and trails in Mountain Valleys. Ecological Applications 15(2), 543-553.
| Crossref | Google Scholar |