Insuring woylies (Bettongia penicillata ogilbyi) against extinction: establishment of Perup Sanctuary
Natasha D. Harrison


A
B
C
Abstract
Predator free havens are increasingly relied upon to preserve populations of imperilled species, yet despite their substantial cost, the success of these ventures is rarely critically evaluated.
Based on 12 years of population monitoring data, we report on the translocation of woylies (Bettongia penicillata ogilbyi) to Perup Sanctuary, a 423 ha predator-free haven in the south west of Western Australia.
We built spatially explicit capture–recapture models to estimate population density, population growth rates, and survivorship of woylies inside the sanctuary. Using these estimates, and additional demographic information, we aimed to show key drivers of population density, evaluate the establishment of the sanctuary population against predetermined translocation success criteria, and run simulations of different sampling designs to determine a robust sampling design for future monitoring of this population.
The population rapidly increased in the first 3 years (2010–2013), and then fluctuated around a density of ~0.9 woylies ha−1 before declining slightly in recent years to ~0.6 woylies ha−1. All translocation success criteria evaluated were met. The previous 3 months’ rainfall was a key driver of population density and body weight declined over time, indicating that the population may be regulated by food resources.
Woylies have established and persisted in Perup Sanctuary, and against the criteria, the translocation of woylies into Perup sanctuary is a success. Harvests from this population appear to have been sustainable. We discuss these findings in the context of the Perup Sanctuary, and recommend ongoing monitoring continue to ensure that the population remains viable and well managed.
We describe important considerations for the supplementation and harvest of fenced populations, including: the source of animals (selecting free-living individuals over captive ones); the timing of release (releasing more individuals early on may improve establishment probabilities); and rates of harvest (<30% of adults harvested per generation seemeded to be sustainable for woylies in this case). The results from this study can inform the ongoing management of this and other havened populations, to ensure they continue to benefit mammal conservation.
Keywords: brush-tailed bettong, conservation management, density, monitoring, population growth, predator-free haven, survivorship, translocation.
Introduction
To preserve species, conservation managers are increasingly turning to havens, offshore islands or fenced areas where populations can thrive in isolation from threatening processes such as predation, competition, hunting and habitat loss (Hayward and Kerley 2009). This strategy is especially valuable in Australia, where mammals are facing unprecedented rates of extinction, worse than those of any other continent (Woinarski et al. 2015; Legge et al. 2018). The primary driver of many of these extinctions is predation by invasive species such as feral cats (Felis catus) and red foxes (Vulpes vulpes) (Woinarski et al. 2015; Wayne et al. 2017), who are implicated in the large majority of Australia’s 40 mammal extinctions (Murphy et al. 2019; Burbidge 2024). Across the country, havens have been developed in response to the threat of invasive predators (Hayward and Kerley 2009; Legge et al. 2023), capturing 32 threatened species and spanning approximately 2500 km2 in area (Legge et al. 2018). Havens have proved extremely effective at mitigating the immediate threat of predation, and are thought to have secured close to 200 populations of threatened mammals (Legge et al. 2018).
These successes do not come cheaply – the construction and maintenance of predator-free fences is costly in terms of both money (e.g. AUD$12,432 per km−1 construction costs; Moseby and Read 2006) and resources (i.e. human effort). To ensure this cost is not in vain, it is important to set success criteria and to regularly monitor havened populations over time (Fischer and Lindenmayer 2000). Parameters that are essential to effective monitoring regimes include survival, especially of translocated founding individuals, and population growth, as these can be informative descriptors of early population establishment. Density is also important to monitor as havened populations of some species can quickly reach high densities and approach carrying capacity (Moseby et al. 2018; Smith et al. 2020; Short 2023). Such population monitoring facilitates best practice management approaches that can evolve with the needs of a growing population. Here, we use 12 years of population monitoring data from cage-trapping to evaluate the establishment of a 423 ha haven in Tone-Perup Nature Reserve, Western Australia, housing an insurance population of the Endangered (Threatened Species Scientific Committee 2018) woylie (Bettongia penicillata ogilbyi).
Woylies are a medium-sized, primarily mycophagous macropod, considered ecosystem engineers for their role in soil turnover and seed dispersal (Garkaklis et al. 2004; Murphy et al. 2005). The species has become extinct across nearly its entire range, which once spanned much of the southern and semi-arid zones of Australia (Threatened Species Scientific Committee 2018). Remnant indigenous populations remain in two regions, both in southern forested parts of Western Australia: the Upper Warren and the Wheatbelt (Wayne et al. 2015).
Managing the threats from introduced predators (foxes and feral cats) and translocations have been critical to the conservation and recovery of the woylie (Groom 2010; Yeatman and Groom 2012). More woylies have been translocated for conservation than any other native mammal in Australia (>3000 individuals to more than 75 sites); however, only 30% of these translocations have been considered successful (Short 2009; Finlayson et al. 2010; Clayton et al. 2014; Morris et al. 2015; Palmer et al. 2020; Frick et al. 2023). This highlights the importance of understanding the factors associated with success and failure to improve species conservation outcomes in the future. However, there are relatively few peer-reviewed publications providing details of the demographics of translocated populations in the early stages of establishment (but see Priddel and Wheeler 2004; Ostendorf et al. 2016; Page et al. 2019; Smith et al. 2020; Short 2023). With an increased understanding of the factors that facilitate population establishment and persistence, conservation managers can better manipulate such variables to improve the outcomes of future translocations.
Following substantial region-wide woylie population declines (Wayne et al. 2013a, 2015), the Perup Sanctuary was constructed in 2010 as a rapid response to preserve the species, and to allow further investigation into the causes of the decline. Such a haven serves many purposes. First and foremost it insures this population against their primary threat, invasive predators (Pacioni et al. 2017) and preserves genetic diversity (Wayne et al. 2013b). The haven also facilitates research on population demography, information crucial for effective predictive modelling (Pacioni et al. 2017) and can provide valuable insights to inform further monitoring and management practices. To realise these goals, and to measure the success of the Perup Sanctuary against predetermined translocation success criteria, the havened population has undergone extensive monitoring since the initial release of founding woylies.
In this study, we aim to evaluate the translocation and establishment of woylies into Perup Sanctuary. More specifically, we will use capture–recapture modelling to: (1) describe the establishment of the woylie population by determining population growth rates and density estimates; (2) evaluate survival in the initial founding cohort of woylies (and subsequent sanctuary-born and supplementation cohorts), and determine differences based on their source or timing of release; and (3) investigate demographic parameters such as sex ratios, proportion of adult females breeding, and mean body weight. We will then use these parameters to: (4) assess the overall success of the translocation into the sanctuary using pre-defined success criteria; and finally (5) incorporate these population attributes into design modelling to inform best practice monitoring for the sanctuary going forward. The results from this study will show a clear demographic picture of the havened population, providing future direction for the strategic management of this and other havened populations.
Methods
Perup Sanctuary
Perup Sanctuary is largely characterised by Yerraminnup and Bevan habitat classes (Mattiske and Havel 1998) and receives ~680 mm of annual rainfall on average (Bureau of Meteorology 2022). The haven is 423 ha and is surrounded by a predator-proof fence. The fence is built from 30 mm hexagonal mesh creating a vertical barrier of 190 cm, a 200 cm fixed apron at the base (to resist digging under the fence), a 70 cm outward-facing floppy top, and outrigged electrical wires (to resist animals climbing over) (Wayne et al. 2013b). Foxes, cats, emus (Dromaius novaehollandiae), and the native predator chuditch (Dasyurus geoffroii) were removed or confirmed absent from the Perup Sanctuary at the time of establishment. While most were herded out, a few yongka (western grey kangaroo, Macropus fuliginosus) and one kwara (western brush wallaby, Notamacropus irma) are known to remain within (Yeatman and Wayne 2015). There have been ad hoc opportunistic attempts to control yongka from 2010 to 2023. The sanctuary also contains populations of quenda (Isoodon fusciventer), koomal (Trichosurus vulpecula), ngwayir (Pseudocheirus occidentalis), numbat (Myrmecobius fasciatus), mandarda (Cercartetus concinnus), wambenger (Phascogale tapoatafa), dunnart (Sminthopsis sp.), and mardo (Antechinus flavipes). Originally the objective was to establish an insurance population representing the Kingston and Perup woylie populations in the Upper Warren, which were genetically distinct from the other remnant indigenous population at Dryandra and Tutanning (Pacioni et al. 2011). The objective was later revised in 2013 to have the woylie population in the Perup Sanctuary representative of the genetics across all four remnant indigenous populations (Dryandra, Tutanning, Perup, and Kingston). This was in response to new evidence that demonstrated that contemporary differences between the populations was a modern artefact of genetic isolation resulting from habitat fragmentation, and that historically these fragments were previously part of the same expansive woylie population found across much of southwestern Australia (Pacioni et al. 2015). Based on this, in 2010, 41 founding woylies from surrounding indigenous populations (Upper Warren: Perup and Kingston; Fig. 1) were released into the Perup Sanctuary, with 36 additional individuals later introduced from the Dryandra indigenous population (Fig. 1) in 2013, followed by 23 individuals raised in captivity (orphans from the Upper Warren or Tutanning individuals bred in captivity) introduced between 2013 and 2015 (Table 1). Criteria for the success of the translocation of these founding individuals into the sanctuary determined before the release of animals were as follows:
better than 80% survival at 6 months post release
the appearance of new, unmarked woylies in the population
the establishment of a successful and sustainable population of woylies within the Perup Sanctuary after 3–5 years (>300 individuals)
retention of >90% of the genetic diversity of the founder population of woylies within the sanctuary after 5 years.
Map of Kingston National Park (Kingston population), Tone-Perup Nature Reserve (Perup population), and Perup Sanctuary within part of the Upper Warren region, Western Australia. State forest and other tenure managed by the Western Australian Government Department of Biodiversity, Conservation and Attractions (DBCA) is also shown.
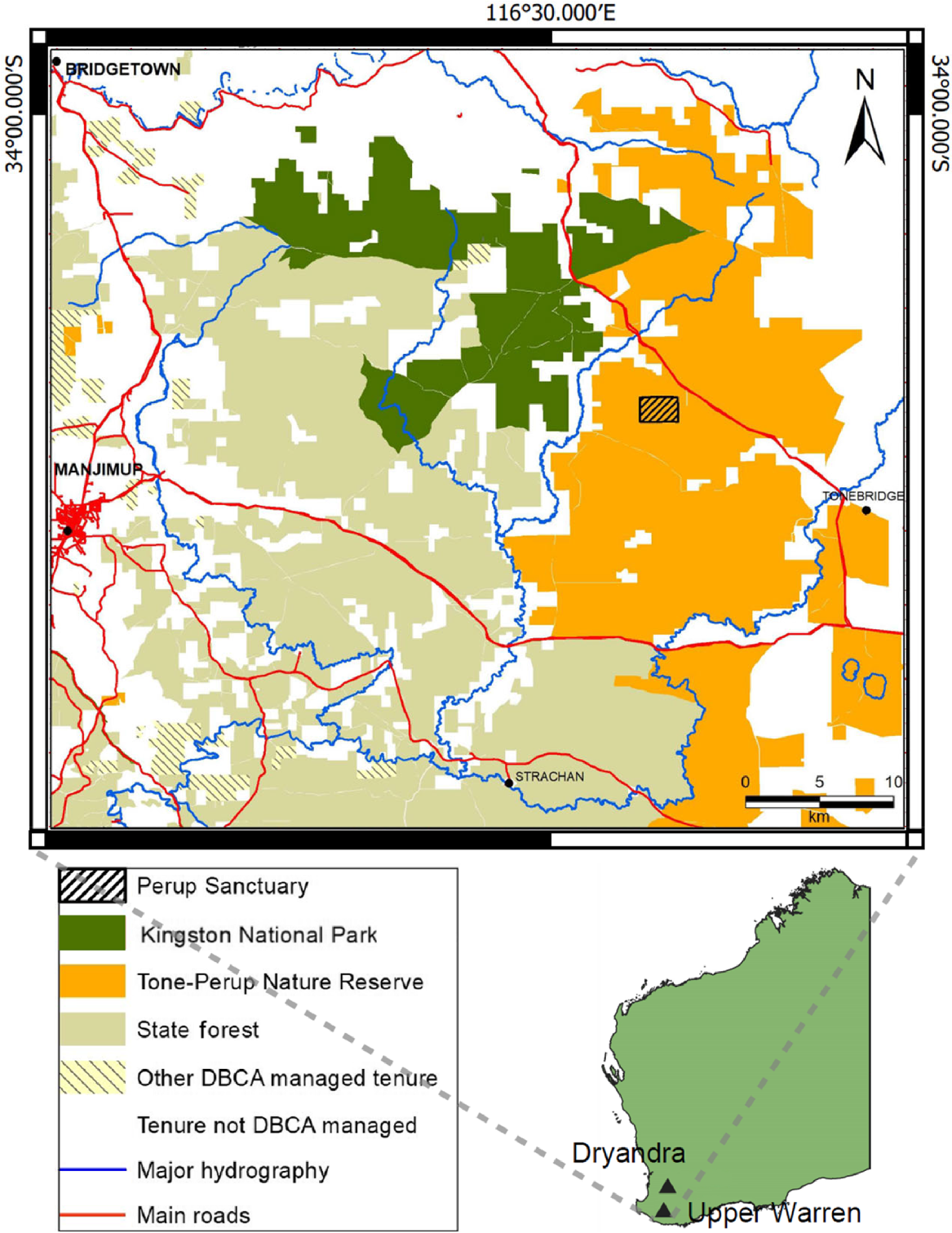
Year | Source population | Destination | Number of woylies | Sex ratios | |
---|---|---|---|---|---|
2010 | Perup | Perup Sanctuary | +28 | 15M:13F | |
2010 | Kingston | Perup Sanctuary | +13 | 6M:7F | |
2013 | Perup Sanctuary | Yendicup (Perup) | −871 | 51M:36F | |
2013 | Dryandra | Perup Sanctuary | +371 | 24M:13F | |
2014 | Perup Sanctuary | Walcott (Kingston) | −92 | 46M:46F | |
2014 | Perup Sanctuary | Warrup East (Kingston) | −90 | 44M:46F | |
2013–2014 | Perup Sanctuary | Whiteman Park | −3 | 3F | |
2013–2015 | Kanyana Wildlife Rehabilitation (Tutanning animals) | Perup Sanctuary | +21 | 14M:7F | |
2014–2015 | Upper Warren (orphans in care) | Perup Sanctuary | +2 | 2M | |
2016 | Perup Sanctuary | Mt Gibson | −31 | 16M:15F | |
2016 | Perup Sanctuary | Dryandra | −28 | 17M:11F | |
2016 | Perup Sanctuary | Karakamia | −5 | 5M | |
2018 | Perup Sanctuary | Mt Gibson | −48 | 32M:16F | |
2022 | Perup Sanctuary | Yorke Peninsula, SA | −16 | 6M:10F | |
2022 | Perup Sanctuary | Pilliga, NSW | −302 | 18M:12F | |
2022 | Kingston | Perup Sanctuary | +102 | 6M:4F | |
Total | Reintroduced: 111 | Harvested: 430 |
Superscript numbers indicate instances where woylies were supplemented into Perup Sanctuary immediately after some were removed.
Since its establishment, the sanctuary has acted as a source for woylie reintroductions and supplementations elsewhere (Table 1). Such harvests have routinely selected individuals that were independent and healthy (preferentially young adults), and only females with pouch young less than 50 mm crown rump length and no evidence of a young at heel (i.e. no large, elongated lactating teat). Harvests also aimed to have parity in sex ratios. Founders or earlier generation individuals and individuals with very high parasite loads (a subjective assessment) were not candidates for translocation. One of the initial Perup founders was inadvertently harvested from the sanctuary in 2013, and one Dryandra individual was found to have escaped in 2014 – these animals have been excluded from the analysis. In some years, harvests of woylies were shortly followed with supplementary introductions, to take advantage of the recently vacated territories (to attempt to improve the prospects of the new individuals).
Population monitoring
Cage trapping to monitor the population has been conducted inside Perup Sanctuary at least annually since 2010 (for dates please see Supplementary Table S1). Over time, the sanctuary has experienced three different configurations for annual routine cage trapping (Table 2; Additional File 1).
Year | Traps | Spacing (m) | Effective sampling area (from JSSAsecrl model described below) | |
---|---|---|---|---|
2010–2012 | Transect of 80 traps | 200 | 401 ha | |
2013 | Transect of 144 traps | 100 | 401 ha | |
2014–2021 | Three 9 × 9 grids (243 traps) | 25 | 180 ha (60 ha per grid) | |
2022–2023 | One 10 × 10 grid (100 traps) | 100 | 210 ha |
Between 2010 and 2013, track-based transects around the internal perimeter and through the middle of the sanctuary were used, but as the population increased, the density of traps was increased in 2013 to avoid trap saturation. From 2014 to 2021, we used three 9 × 9 grids (with reduced spacing in a further attempt to avoid trap saturation) and in 2022 and 2023, a single 10 × 10 grid was trialled. At each of these sessions, traps were baited with peanut butter and oats and opened for four nights (opened in the afternoon and cleared shortly after sunrise the following morning). The sampling design during trapping to harvest individuals for translocation to other populations varied considerably depending on the number of animals required. Although in this study these capture records were only used to supplement our information on demography and the proportion of individuals alive. All captured animals were tagged with uniquely numbered titanium ear tags (Monel 1005-1 ear tags, National Tag and Brand Co., Newport, KY, USA), weighed, sexed, aged (based on the presence of fully formed testes or an active pouch) and released. Each animal was given two ear tags to attempt to ensure animals remained identifiable in the case that one tag was lost.
Extensive surveillance for terrestrial vertebrate predators (including 14 permanently deployed remote sensor camera traps checked fortnightly, opportunistic radio-telemetry of woylies, sign surveys, annual spotlighting, and weekly fence integrity checks) confirmed that there had been no incursions since establishment.
Statistical analysis
All statistical analyses were conducted in R v4.0.3 (R Core Team 2020). With mark–recapture data from between 2010 and 2023, we built an open population Cormack Jolly Seber capture–recapture model (JSSAsecrl) from the package openCR (Efford 2021a) to estimate woylie population density and growth rates (lambda) at each session. Such models are spatially explicit, allowing all of the different sampling designs to be combined into one robust model. We allowed lambda (λ; finite rate of increase for the population), sigma (σ; detection function), and phi (ϕ; apparent survival) to vary among sessions. We incorporated a buffer of 800 m (based on an estimate of 4σ, ensuring that it encompassed a home range ~65 ha; Yeatman and Wayne 2015). The fenced area was added as a closed survey mask and primary trapping sessions were analysed in blocks of three consecutive sessions with the moving.fit function (Treloar et al. 2021). Because this model type is unable to give estimates on final session (population growth in T1 is calculated based on the outcome of T2), we ran a maximum likelihood spatially explicit closed population capture–recapture model from the package SECR (Efford 2023b) to provide a density estimate for 2023. For this model we incorporated a buffer of 800 m, used the half-hazard normal detection function, and added the fenced area as a closed survey mask. Density, detection probability (g0) and sigma (σ; space use) were allowed to vary among sessions.
Survivorship among founders from different source populations was calculated in two ways. Firstly, it was inferred based on all available capture data (including routine monitoring and trapping conducted to harvest woylies for translocation out of the sanctuary) – those individuals that were recaptured were confirmed to be alive, and we retrospectively assigned their survival to all sessions before such time point. This allowed us to calculate the proportion of individuals from each source known to be alive at each session and the minimum number of individuals known to be alive (Krebs 1966). We also calculated survivorship rates with the package openCR (Efford 2021a). We first built a conditional likelihood spatially explicit open population capture–recapture model (JSSAsecrlCL), using the closed survey mask and consecutive session analysis as above, allowing λ0 (detection function intercept), σ (detection function scale), and ϕ (apparent survival) to vary among sessions. From this model, we were able to derive survivorship rates based on source populations and timing of release (as these parameters are derived from the model, and not estimated, we were unable to calculate error margins for these values).
Descriptive statistics were calculated to describe the sex ratio (% female) and proportion of adult females breeding. To explore trends in weight over time we built a linear mixed effects model using the ‘lme4’ package (Bates et al. 2015) to investigate the effects of time (trapping session) and sex on weight, including individual identity as a random effect to account for repeat measures of individuals over time. To investigate potential environmental drivers of population density, we built a linear model using the ‘stats’ package (R Core Team 2020) where the response variable was population density estimates (n = 10). The first 2 years were excluded from this model to reduce any confounding effects from the initial low density as the population established. We included cumulative rainfall from the previous three months, and rainfall from the Nyoongar seasons Djeran (April–May) and Makuru (June–July) in the previous year as predictor variables calculated using Bureau of Meteorology monthly rainfall measurements made at the closest weather station, Westbourne 009616 (Bureau of Meteorology 2022). To account for the harvest from and supplementation of woylies to this population, we included the net harvest number (supplemented individuals minus harvested individuals, calculated monthly) as a predictor variable. Each harvest or supplementation was counted for the subsequent 6-month period after each event as the effects of these may sustain over several months while individuals establish home ranges. In both models, we assessed model assumptions by visually comparing standardised residuals to fitted values (Ferrari and Cribari-Neto 2004). Using the package ‘performance’ (Ldecke et al. 2019) we calculated a variance inflation factor for each covariate to test for collinearity and considered all values below 1.5 to have acceptably low collinearity (Zuur 2011). The model with the lowest AIC value was considered the best model, and to evaluate the significance of each variable, we performed likelihood ratio tests for the best model with and without each respective parameter.
We used the ‘secrdesign’ package (Efford 2023a) to undertake a simulation-based assessment of four survey designs, based around approximately 100 traps, the number of traps used during the 2022/2023 sampling seasons. We compared four grid designs: (1) four grids of 25 traps (5 × 5, n = 100); (2) three grids of 36 traps (6 × 6, n = 108); (3) two grids of 49 traps (7 × 7, n = 98); and (4) one grid of 100 traps (10 × 10, n = 100) (Table 3). The appropriate distance between traps was examined using the optimal spacing function for each grid design assuming that multiple captures per trap were possible (detector = “multi”), as single capture detectors are not compatible with this function. The ‘make.grid’ function was used to create a list of trapping arrays by assigning each optimal distance result to the respective grid design and the ‘make.scenarios’ function was used to assign the scenario parameters (Table 3). Grid arrangements were modelled using the ‘make.systematic’ function, which can be used to divide the sampling area into sections and a grid of traps deployed in each section. All simulations were replicated 500 times and the simulated relative standard error (RSE), relative bias (RB) and root mean square error (RMSE) were extracted for comparison (Clark 2019; Efford and Boulanger 2019; Ash et al. 2020). We considered simulations with an RSE value of <20% and an RB of <15% to be well performing models, with lower values indicating better performance (Kristensen and Kovach 2018). RMSE offers a combined measure of RSE and RB, with lower RMSE values considered to be better performing models. We then ran simulations for the sampling designs deployed in 2010–2012 and 2022–2023 (Table 2) using the same parameters (Table 3) and compared these against the best performing grid design.
Parameter | Value | Source or justification | |
---|---|---|---|
Density | 0.95 ha−1 | Result from the 2022 sampling session above | |
Sigma (index of movement) | 166.5 | Result from the 2022 sampling session above | |
Detection probability | 0.11 | Result from the 2022 sampling session above | |
Occasions | 4 | The standard number of trapping nights per session | |
Spacing (m) | 5 × 5: 70 6 × 6: 101 7 × 7: 116 10 × 10: 140 | From the optimal spacing function |
Results
Population growth and density
In the first years after the initial release in 2010, the woylie population inside Perup Sanctuary experienced high growth rates and a sharp increase in population density (Fig. 2a). Population density peaked at ~1.2 ha−1 in 2016 and appeared to generally fluctuate around a density of 0.9 ha−1, approximately 380 woylies. There has been a steady downward trend of woylies from 2021 to 2023. The growth rate (λ) in the first 3 years (before supplementation began) was 2.57 (95% confidence interval: 1.86–3.55), 2.12 (1.73–2.61), and 2.09 (1.78–2.46) respectively, before slowing to around 1.0 from 2014 to 2022 (0.67, CI: 0.58–0.76 in 2017; 1.05, CI: 0.95–1.16 in 2018).
(a) Density (left axis, solid line) and minimum number of individuals alive (right axis; dashed line). Colours along the dashed lines indicate sampling design: red = 80 trap transect 200 m spacing (2010–2012), orange = 144 trap transect 100 m spacing (2013), green = three 9 × 9 grids 25 m spacing (2014–2021), blue = 10 × 10 grid 100 m spacing (2022–2023). (b) Population growth rate (finite rate of increase, 1 = stable population) of the Perup Sanctuary woylie population. Shading indicates confidence intervals. Vertical dotted lines represent the harvest (−) or supplementation (+) of individuals.
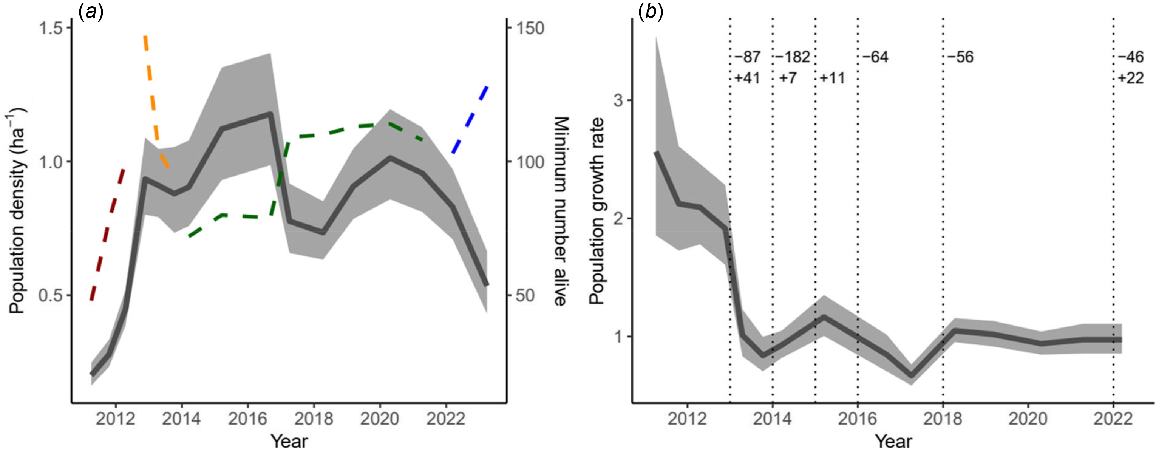
Survivorship
Survival in the founding cohorts varied substantially depending on source population and timing of release. Survivorship was high in individuals sourced locally from the Upper Warren (Kingston and Perup). In the first 2 years, survival was 100% for individuals from both sources, and this gradually declined over time, with the last individuals from these cohorts being recorded alive in 2020, a decade after their initial release (Fig. 3). Individuals from Dryandra, an indigenous remnant population 150 km from Perup, experienced around 50% mortality in the first year after their release in 2013, although after this initial loss, the remaining individuals shared similar survival probabilities with the Perup and Kingston individuals. Survival rates for each of these cohorts dramatically dropped in 2020 and 2021, likely as the remaining founders died from old age. Survival in individuals from each of these sources seemed to fluctuate in line with the survival of the sanctuary born animals (Fig. 3b). None of the individuals raised in captivity (released 2013–2015) were ever recaptured or sighted again, and all are assumed to have died within the first year of their release. The effective sampling area after the release of these individuals was 401 ha (in 2013) and between 180 and 270 ha (between 2014 and 2021). This accounts for between 43% and 95% of the area of the sanctuary, and assuming that the probability of new individuals establishing is equal across the entire sanctuary, we would have expected to detect 43–95% (between 9 and 21 woylies) of these released individuals if they had survived. The proportion of Kingston animals known to be alive following the second release in 2022 was 36% 6 months after release.
(a) Proportion of founding individuals known to be alive and (b) estimated survival rate per trapping session of woylies inside Perup Sanctuary from various founding cohorts (Perup = green; Kingston (one cohort released in 2011 and one released in 2022) = orange; Dryandra = blue; captive raised = yellow; sanctuary born = black dashed).
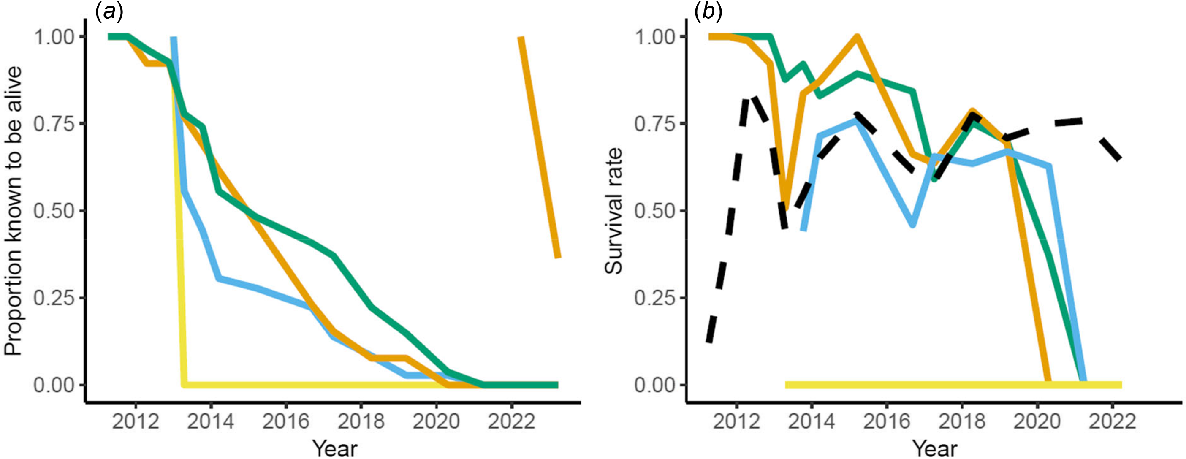
Demographic parameters
The Perup sanctuary woylie population has been male biased except in 2012 (parity; 1:1), and 2021 (female biased) (Fig. 4a). The proportion of adult females breeding was lowest in the first 5 years and has remained high (above 95%) since 2015 (Fig. 4b). The mean weight of both adult males and females has slowly declined since the release of the founders (Fig. 4c, Table 4), dropping from an average of 1450 g to 1180 g in males and 1410 g to 1160 g in females between 2010 and 2022. There was a weak, positive relationship between rainfall in the previous 3 months and population density (Fig. 4d). Rainfall in Djeran and Makuru (from the previous year), and net harvest numbers did not affect population density estimates (Table 5).
Demographic parameters of Perup Sanctuary Woylie population. (a) Sex ratio (percentage female); (b) percentage of adult females breeding; (c) weight of adult males (blue dashed line) and females (red solid line); and (d) effect of previous 3 months rainfall on population density. Shading in (c) and (d) indicates 95% confidence intervals.
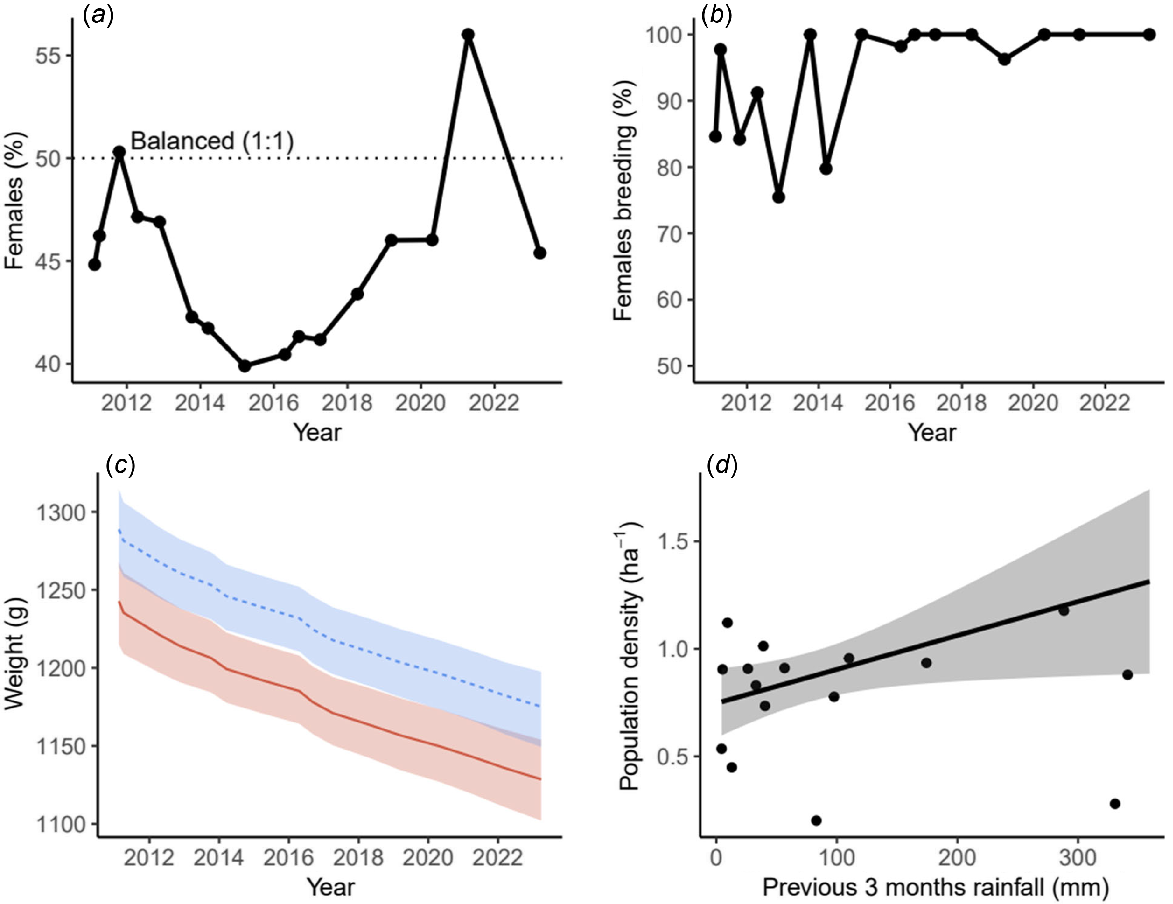
Assessment of translocation success criteria
All three of the demography-based translocation success criteria were met (Table 6).
Criteria | Outcome | Justification | |
---|---|---|---|
1. Better than 80% survival at 6 months post-release. | Satisfied | 100% survival in both Kingston and Perup founders in the first 12 months (Fig. 3). | |
2. The appearance of new, unmarked woylies in the population. | Satisfied | New, unmarked individuals were detected six months post-release. | |
3. The establishment of a successful and sustainable population of woylies within the Perup Sanctuary after 3–5 years (>300 individuals). | Satisfied | Population of ~380 individuals reached after 3–4 years (Fig. 2) | |
4. Retention of >90% of the genetic diversity of the founder population of woylies within the sanctuary after 5 years. | Not formally assessed here | Assessment underway. Other related genetic information also available (e.g., Pacioni et al. 2020; Farquharson et al. 2021) |
Population monitoring sampling design
The optimal spacing for all grid types fell between 70 and 140 m. The larger grid sizes were more sensitive to a change in spacing between traps than the smaller grids, which maintained a smaller RSE value across a larger range of trap spacing (Fig. 5).
Expected number of new captures (En, light green line) and recaptures (Er, dark green line) compared to relative standard error (RSE, black dashed line) for four grid formations: four 5 × 5 grids, three 6 × 6 grids, two 7 × 7 grids and one 10 × 10 grid.
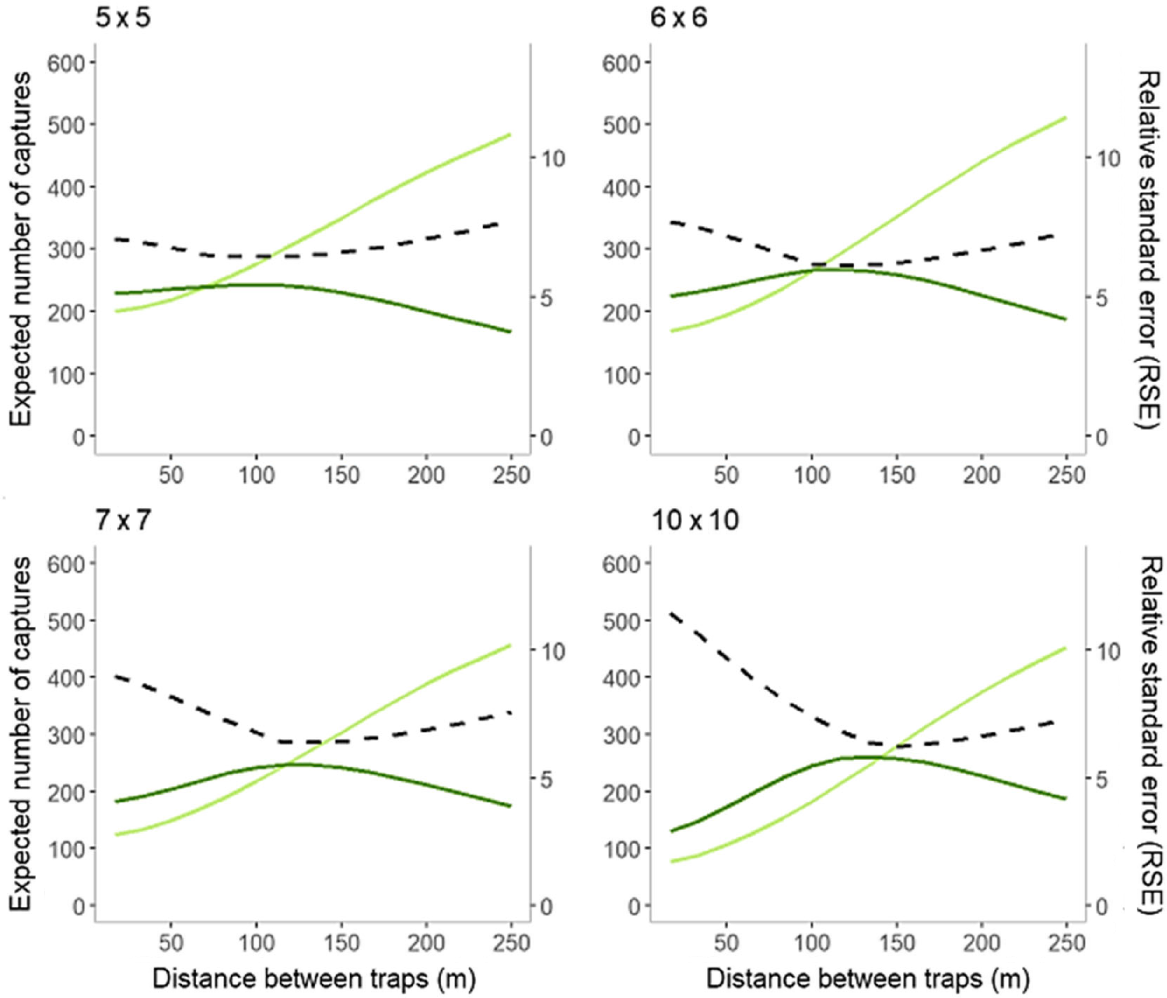
Based on RMSE, the larger grid sizes performed better than smaller grid sizes, with the 10 × 10 grid (RMSE: 0.0614) being the best performing overall. The 6 × 6 grids had the lowest RB, after which it increased at a slow rate with grid size but this increase was minimal (Fig. 6). The 5 × 5s performed poorly compared to all other grids, with an RSE value almost 3% higher than the best performing, and 2% higher than the next best grid formation (Fig. 6). Though the expected number of captures predicted by the simulations might be slightly inflated (as we assumed multiple captures per trap were possible), this bias is likely to be minimal, and isn’t expected to alter the interpretation of the outcome (Efford 2023b).
The relative standard error (RSE; dashed line), relative bias (RB; solid line) and root mean square error (RMSE; dotted line) for each grid formation of traps.
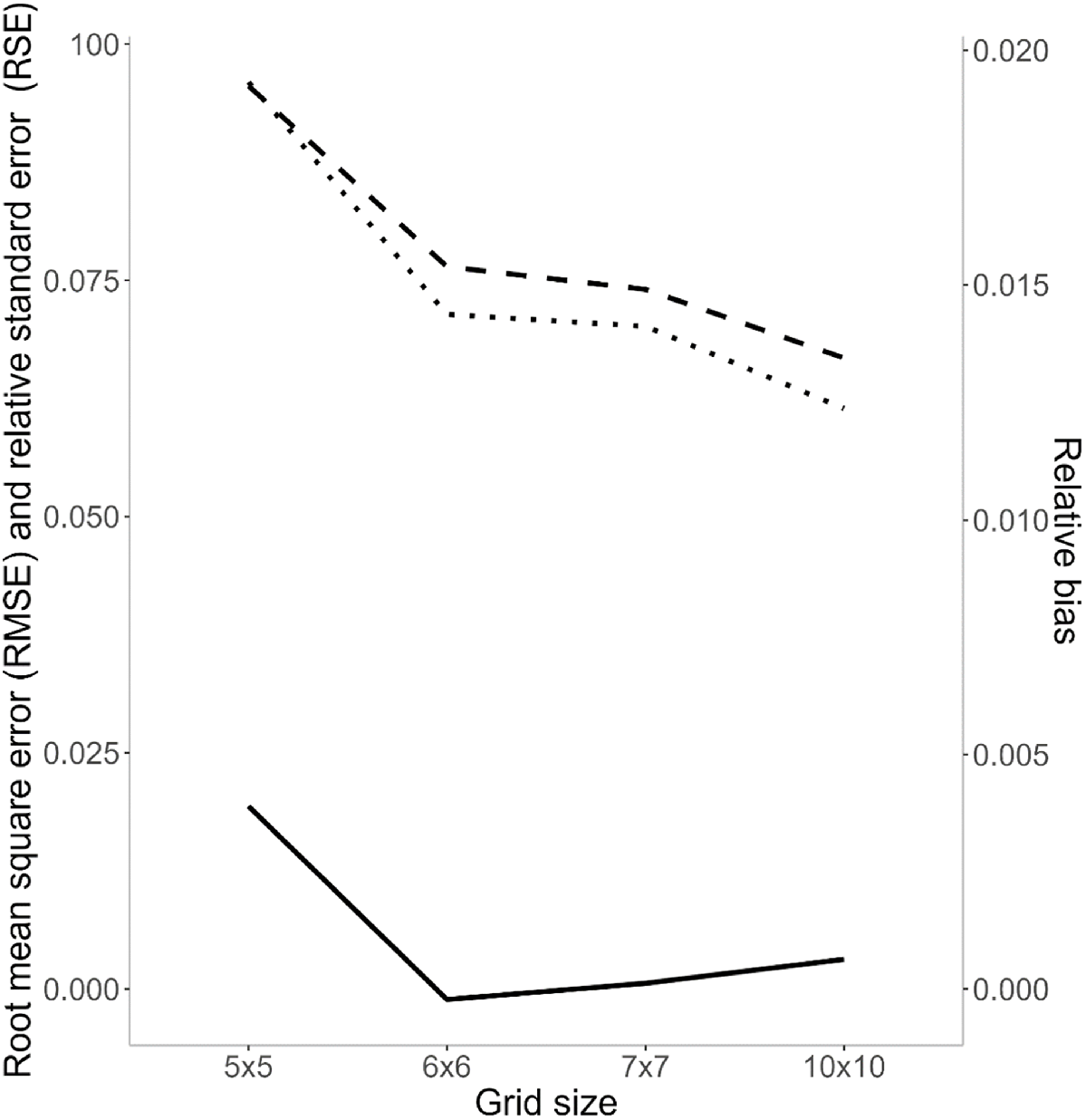
The best performing simulated grid design (single 10 × 10 grid at 150 m spacing) had an RSE of 6.2, performing slightly better than the sampling designs deployed in 2010–2012 (transect of 80 traps at 200 m; RSE = 7.3) and in 2022–2023 (single 10 × 10 grid at 100 m spacing; RSE = 7.4).
Discussion
We have a clear demographic picture of the woylie population establishment inside Perup Sanctuary. Against the translocation success criteria, the translocation of woylies into the sanctuary is a success, and a viable population persists. As with other havened woylie populations, the sanctuary population grew rapidly (Smith et al. 2020; Short 2023), and reached 400 individuals within the first three years. Over the 12-year period, population density generally appeared to fluctuate around a density of 0.9 ha−1, approximately 380 individuals. Understanding the dynamics and size of this population can be used to inform its ongoing management, including ensuring sustainable harvest rates for translocations elsewhere in an effort to continue the conservation and recovery of this species. We outline further insights and future directions below.
Population growth rates and density
Measures of population growth rates are useful for population viability analyses (PVA; e.g. Pacioni et al. 2017, 2020) and other applications relevant to species conservation and recovery (e.g. identifying population response to threats; Duncan et al. 2020). The population growth rates in the initial years after establishment were expected to approximate the intrinsic rate of increase for the woylie, given that the Perup Sanctuary had no terrestrial mammalian predators, and no woylies present at the time of establishment (i.e. not expected to be resource limited at the time of establishment). The finite annual rate of increase in the Perup Sanctuary in the second and third years (before additional supplementation occurred; 2.56, 1.86–3.55 95% CI; 1.91, 1.61–2.28 95% CI), were comparable to other reported maximum values including in the greater Kingston area when the low woylie densities initially responded to the commencement of regular and broadscale fox baiting (Warrup = 1.49 ± 0.4 s.d.; Winnejup = 2.29 ± 0.44 s.d.; Pacioni et al. 2017), and in Wadderin Sanctuary (~1.2; Short 2023). The annual growth rate inside the predator-free haven at Mount Gibson was higher, approximately 3.1 2–3 years after translocations to the site began, although ongoing supplementation in the second and third year (n = 41, n = 15 respectively) more than doubled the number of founders introduced in the first year (n = 50; Smith et al. 2020). It is important to note that growth at Perup Sanctuary may be somewhat inflated due to the demographic skews in the founding population (i.e. all individuals were of reproductive age and most females carried small pouch young).
Woylie densities observed in the Perup Sanctuary peaked in 2016 at around 1.18 woylies ha−1 (95% CI 0.98–1.40) before averaging around 0.9 woylies ha−1. These are comparable to peak woylie density estimates observed across the Upper Warren region in 1999 (1.57 woylie ha−1; Wayne et al. 2013a), prior to their catastrophic declines. These peak estimates provide an indication of the maximum likely densities for this species when it is not limited by predation in this habitat. Such estimates here are higher than estimates from Perup in the 1970s before extensive fox control, 0.45 woylies ha−1 (Christensen 1980), but similar to those of other predator-free populations including Wadderin Sanctuary (427 ha fenced reserve; around 0.7 woylies ha−1; Short 2023) and Karakamia Sanctuary (275 ha fenced reserve; average 0.9 woylie ha−1 2015–2021; Palmer et al. 2022). The reduction of woylie densities below 0.7 woylies ha−1 in the last 2 years (2022 and 2023) represent the lowest densities observed since the initial years of the establishment of the colony. This may be due to a reduction in rainfall – in 2023, the Sanctuary received only 60% of the long-term mean rainfall for the three months preceding the survey (Bureau of Meteorology 2022). Regular monitoring should continue to determine whether this trend persists, and to identify the drivers of population change.
Drivers of population change
The woylie population in the Perup Sanctuary underwent an approximate 34% decline about 7 years after the population was established in 2010. The timing of this decline is consistent with the observations at Wadderin Sanctuary (peaked at 7.5 years; Short 2023) and predictions of eruptive dynamics (boom-bust cycles) by Duncan et al. (2020), based on a species’ intrinsic rate of population growth and adult body mass. Duncan et al. (2020) also revealed that this phenomenon has occurred in other woylie populations, and that it tends to occur in species with higher maximum rates of population increase, and in populations that increase rapidly and substantially, which was the case here. Where this occurs, it is consistent with some form of density-dependent feedback, such as overconsumption of resources or the build-up of diseases or predators (not the case here), but it does not negate the possibility that population declines could be driven by external factors such as abrupt environmental change or the arrival of new predators or diseases. The population here seems to largely have avoided the ‘bust’ that other havened bettong populations may have faced (Moseby et al. 2018; Short 2023), perhaps owing to the steady number of animals harvested out of the Perup Sanctuary. Nonetheless, this highlights the importance of adequately monitoring populations as well as the factors that may explain population changes including predators and predation rates, body condition and food resource availability, and animal health and signs of disease.
The positive correlation between rainfall and woylie population density in the Perup Sanctuary may also be helpful for predicting population change and likely indicates that the population is driven by the availability of food resources. The diversity, abundance, and biomass of fungi sporocarps, the staple diet of the woylie, have been related to climate and rainfall during the preceding one or more months (Bateman et al. 2012; Danks et al. 2013; Zosky et al. 2017; Buscardo et al. 2021). The decreased average body mass of woylies over time in the Perup Sanctuary is also consistent with food resources increasingly being a limiting factor for the population. Short (2023) also related observed declines in woylie densities, condition, and reproduction at Wadderin Sanctuary with resource limitation and years of low rainfall. The confirmed ongoing absence of terrestrial predators and the results from health and disease monitoring (Skogvold et al. 2017) further strengthen the case that the woylie population in the Perup Sanctuary has been regulated by food. Ongoing monitoring of population size is valuable to allow managers to intervene if the population exceeds carrying capacity and fails to self-regulate to prevent the loss of individual conditions that can result from over-population (Moseby et al. 2018).
Harvest rates of woylies from Perup Sanctuary appear to have been sustainable as there was no significant effect on the population density, and despite the considerable disparities in the rate of introductions compared to harvests (111:430), population growth trends remained positive or neutral. The maximum net harvest rate of approximately 45% of the adult population in 2014, when 182 woylies were removed from the population, was unintended and occurred because of an inaccurate overestimate of the population at the time. While this demonstrates the remarkable breeding capabilities of the woylie, this was greater than the advised safe harvest rates for the species of no more than 30% of the adult (based on the presence of fully formed testes or active pouch) population within a generation (about 2.5 years for the woylie; Pacioni et al. 2019). This threshold is recommended to avoid a significant reduction in the population size and the risks of reducing the genetic diversity of the population. While the large harvest in 2014 did not reduce the population size it is not known what effects this may have had on the genetic characteristics of the population. However, the systematic avoidance of harvesting founders for translocation, should have reduced the risks of losing unique alleles or reducing the genetic diversity in the woylie population within the Perup Sanctuary. Nonetheless, we support using the harvest recommendations of Pacioni et al. (2019) as a conservative measure to ensure that harvest rates from source populations remain sustainable.
Survivorship
The striking differences in the survivorship between the founder cohorts (100%, 50% and 0% of the initial release, 3-year release, and captive born animals released after 3–5 years respectively) could have important implications for the establishment and supplementation of new populations. It appears that after the initial release of founders in 2010, subsequent released cohorts had lower survival probabilities. This may indicate that as the population grew closer to carrying capacity, there were less available territories, and new individuals were less likely to establish and persist. This was the case even when existing individuals were removed prior to the release of new animals, suggesting that the existing population has an advantage in competing for resources. When looking to maximise genetic diversity and population sizes, these findings would suggest that introducing all individuals at once, and giving them equal opportunity to establish territories, may lead to more individuals establishing, compared to staggered releases through time. It is worth noting that despite considerable survey effort, no captive-reared individuals were ever captured after release, which raises the question, whether raising orphaned joeys and releasing them into sanctuaries is the most humane option given their apparent survival was 0. Captive-bred woylies have fared better elsewhere (e.g. Short 2023) and as the release of each cohort into Perup Sanctuary was not completely identical, the possibility cannot be ruled out that additional individual or environmental factors led to the disparity in survival rates between cohorts here. A study of individual survival probabilities (e.g. with radio collars) would be better suited to address this question. Other factors that may affect the survival of translocated animals include population source (e.g. wild, havened [fenced or island], captive, hand-reared); habitat similarity between the source and recipient site; timing in relation to seasonal variation in the availability of important and potentially limited resources; sex, age, condition, health, medical intervention or therapy (e.g. Northover et al. 2019a, 2019b); and the manner in which the animals are released into the recipient population (e.g. staged or ‘soft’ release vs immediate; Tetzlaff et al. 2019; Resende et al. 2021). An improved understanding of the factors that affect the survivorship of founders and subsequent supplementations will substantially improve the existing PVA models (Pacioni et al. 2020) used to inform how best to manage the genetics of existing and future translocated woylie populations.
Demographics
The factors that may be driving the observed changes in sex ratio over time are not clear; however, they provide some indication of the growth potential for the population. A female biased population can grow at a faster rate than a male biased one, though this is unlikely to be the main driver of population fluctuation in Perup Sanctuary as sex ratio does not appear to correlate with population growth (population growth remained relatively stable between 2014 and 2022 despite substantial fluctuations in sex ratio). Periods of female bias are also beneficial for achieving usual harvest objectives of sex ratio parity or female bias for translocations elsewhere. Historically, sourcing enough females for translocation has been particularly challenging owing to the unsuitability of a large proportion of captured females because of their continuous breeding habits and the associated welfare risks to larger pouch young and dependent young ‘at heel’. With the apparent reduction in male bias within Perup Sanctuary in recent years, this may become less of an issue. In other marsupials, such as brush tailed possums, biasing offspring in favour of males occurs in times of limited resources to reduce resource competition with kin (philopatric females are more likely to remain in their mothers territory and compete with her, whereas males disperse) (Johnson et al. 2001). If the same exists in woylies (a similar system), the male bias sex ratio in Perup Sanctuary may be indicative of periods of resource competition, though to support this hypothesis, sex-dependent offspring survival should also be examined. While the change in sex ratio may instead be an artefact of sampling variation, adult body mass has declined over time which is also likely to indicate resource competition within this population. A previous study has further suggested that this may be a response to both resource competition and an absence of selection pressure for larger size without predators present (Harrison et al. 2023). It remains important to continue to monitor adult body mass to account for, and potentially predict, population changes that can inform adaptive management (Page et al. 2019).
Assessment of translocation success criteria
Isolation inside Perup Sanctuary proved a success in protecting woylies from predators and allowing them to establish robust numbers; however, genetic health and representativeness remain to be evaluated. Modern woylie populations have lost between 46 and 91% of the genetic diversity within their mitochondrial DNA (Pacioni et al. 2015), and populations with low gene flow, such as those isolated by fences, are threatened with further and accelerated losses of genetic diversity and consequent inbreeding depression. For a complete appraisal of the success of this translocation, the fourth criterion (retention of >90% of founder genetic diversity) needs to be evaluated. The founder population contained individuals from multiple sub-populations to maximise diversity and representativeness of the extant diversity. Nevertheless, quantifying the genetic diversity within this population is important. Farquharson et al. (2021) found that genetic heterozygosity of the Perup Sanctuary population in 2020 was high and comparable to indigenous source populations (Dryandra, Perup and Kingston). However, they also found some evidence that inbreeding may be occurring here as well as within Karakamia Sanctuary and the indigenous wild populations in Kingston and Perup. While the potential for inbreeding merits further investigation (e.g. to ensure the modelling indicators are not an artefact of sampling), the authors recommend having a metapopulation management strategy that aims to promote increased genome-wide diversity and reduce inbreeding (e.g. through targeted translocations) and maintain overall species heterozygosity by ensuring large population sizes.
Population monitoring sampling design
Design modelling revealed that the current design (10 × 10 grid) was the best performing array. Our current use of 100 m spacing is slightly below the best performing spacing of 130 m, though it remains a robust design (RSE = 7) and is more practical to be run on foot (there are limited roads inside Perup Sanctuary so this grid must be serviced without vehicles). The design modelling was limited by the fact that the ‘secrdesign’ package currently only operates under the assumption that more than one animal can be detected per detector. When capturing woylies using cage traps, this is very rarely the case, and trap saturation is a commonly experienced problem when this species occurs at high densities. While the design modelling is valuable in informing a robust sampling design, it does not take into account the potential issue of trap saturation.
The optimal spacing for all grid types fell between 70 and 140 m and the design implemented from 2014 to 2021 was spaced well below this (three 9 × 9 grids at 25 m spacing). Empirical literature suggests that an effective sampling array should span at least one home range (Bondrup-Nielsen 1983; Efford and Boulanger 2019), which for woylies inside Perup Sanctuary is ~65 ha (Yeatman and Wayne 2015). This was satisfied by the transect design and the 10 × 10 grid, but each of the 9 × 9 grids only comprised approximately 5 ha. Simulation studies have demonstrated that SECR models from sampling designs encompassing less than one animals home range can still perform well (Sollmann et al. 2012), nonetheless, these estimates should be treated with caution as they may have overestimated density. Overestimations of density by the 9 × 9 grids may partially account for the observed decline in woylie density after 2021, and we recommend that density continue to be monitored closely using the current sampling design (10 × 10 grid, 100–150 m spacing) that spans more than one woylie home range.
Ongoing management of the sanctuary and the woylie population
Ongoing monitoring of the population is fundamentally important to ensure effective and adaptive management. The success to date is no guarantee of success going forward. The many failed historic translocations (Threatened Species Scientific Committee 2018; National Environmental Science Program Threatened Species Research Hub 2019), and the recent rapid >90% declines and local extinctions (Wayne et al. 2013a, 2015, 2017), are clear testimony of the ever-present risks to woylie populations. Done well, monitoring can prompt timely management intervention and identify the putative drivers of population change. Our population here appears to be limited by resource availability, and further understanding of such limitation would be valuable in understanding the ability of the landscape to support the woylie population.
It is also important to assess whether sustained high woylie densities have any adverse effects on the habitat and other species within the Perup Sanctuary. As ecosystem engineers and major soil bioturbators, woylies are expected to provide many benefits to the ecosystem (Garkaklis et al. 2004; Murphy et al. 2005; Fleming et al. 2014). While they are vectors in the dispersal of seeds and spores (Beca et al. 2021; Palmer et al. 2021), including those of hypogeal mycorrhizal fungi, they are also predators of plant propagules, roots and tubers, and some small animals (Zosky et al. 2017). Major changes in vegetation have been shown to result from high densities of another ecosystem engineer, the closely-related boodie (burrowing bettong, Bettongia lesueur) (Linley et al. 2017; Moseby et al. 2018), and vegetation changes have also been observed at multiple sanctuaries containing woylies (Wadderin Sanctuary, Short 2023; Scotia and Yookamurra; Kemp et al. 2021). A formal analysis of the impact of woylies on the plant and fungal communities in Perup Sanctuary would be valuable to assess resource availability.
Conclusion
The overall picture of the havened woylie population is one of success. Without the pressure of predation, woylies have thrived, and a viable insurance population has been established. However, the substantial differences in survivorship between founding cohorts is of significant concern and requires further investigation. We have demonstrated that our modelled preferred sampling design (10 × 10 grid, 100 m spacing) provides robust woylie density estimates that can inform future sustainable harvests from this population. Recent decreases in population density could be a result of altered sampling regimes, reduced rainfall and/or depletion of resources. Careful management and monitoring of this population is critical to its own viability and value as both an insurance population and source for translocations elsewhere, to conserve this important species.
Data availability
The data that support this study will be shared upon reasonable request to the corresponding author.
Declaration of funding
South West Catchments Council and the ‘Saving our Species’ Biodiversity Conservation Initiative (Government of Western Australia) provided funding support for some of the monitoring and management of woylie population in Perup Sanctuary. The funders played no role in the development of the study.
Acknowledgements
We acknowledge the Traditional Custodians of the lands and animals on which this study was conducted, the Kaneang, Pibelmen and Minang Nyoongar people. We thank the many professionals, staff, students, and volunteers who have supported the collection of this data as part of routine monitoring by DBCA and its predecessor agencies. Thanks to two anonymous reviewers for their thoughtful comments. All procedures were approved by the DBCA Animal Ethics committee (Animal Ethics approval numbers 2010/36; 2009/36; 2012/57; 2012/42; 2015/32; 2018/22F).
References
Ash E, Hallam C, Chanteap P, Kaszta Ż, Macdonald DW, Rojanachinda W, Redford T, Harihar A (2020) Estimating the density of a globally important tiger (Panthera tigris) population: using simulations to evaluate survey design in Eastern Thailand. Biological Conservation 241, 108349.
| Crossref | Google Scholar |
Bateman BL, Abell-Davis SE, Johnson CN (2012) Climate-driven variation in food availability between the core and range edge of the endangered northern bettong (Bettongia tropica). Australian Journal of Zoology 59, 177-185.
| Crossref | Google Scholar |
Bates D, Mächler M, Bolker B, Walker S (2015) Fitting linear mixed-effects models using lme4. Journal of Statistical Software 67, 1-48.
| Crossref | Google Scholar |
Beca G, Palmer B, Valentine LE, Erickson TE, Hobbs RJ (2021) Gut passage time and viability of seeds consumed by Australian marsupials. Australian Mammalogy 43, 363-367.
| Crossref | Google Scholar |
Bondrup-Nielsen S (1983) Density estimation as a function of live-trapping grid and home range size. Canadian Journal of Zoology 61, 2361-2365.
| Crossref | Google Scholar |
Burbidge AA (2024) Australian terrestrial mammals: how many modern extinctions? Australian Mammalogy 46, AM23037.
| Crossref | Google Scholar |
Bureau of Meteorology (2022) Monthly rainfall; Westbourne 9616. Available at http://www.bom.gov.au/jsp/ncc/cdio/weatherData/av?p_nccObsCode=139&p_display_type=dataFile&p_startYear=&p_c=&p_stn_num=009616)
Buscardo E, Souza RC, Meir P, Geml J, Schmidt SK, da Costa ACL, Nagy L (2021) Effects of natural and experimental drought on soil fungi and biogeochemistry in an Amazon rain forest. Communications Earth & Environment 2, 55.
| Crossref | Google Scholar |
Clark JD (2019) Comparing clustered sampling designs for spatially explicit estimation of population density. Population Ecology 61, 93-101.
| Crossref | Google Scholar |
Clayton JA, Pavey CR, Vernes K, Tighe M (2014) Review and analysis of Australian macropod translocations 1969–2006. Mammal Review 44, 109-123.
| Crossref | Google Scholar |
Danks M, Lebel T, Vernes K, Andrew N (2013) Truffle-like fungi sporocarps in a eucalypt-dominated landscape: patterns in diversity and community structure. Fungal Diversity 58, 143-157.
| Crossref | Google Scholar |
Duncan RP, Dexter N, Wayne A, Hone J (2020) Eruptive dynamics are common in managed mammal populations. Ecology 101, e03175.
| Crossref | Google Scholar | PubMed |
Efford M (2021) Opencr: Open population capture–recapture models. R package version 2.2.1. Available at https://CRAN.R-project.org/package=openCR/
Efford MG (2023a) Secrdesign: sampling design for spatially explicit capture–recapture. R package version 2.8.2. Available at https://CRAN.R-project.org/package=secrdesign
Efford MG (2023b) Secr 4.5 - spatially explicit capture–recapture in R. Available at https://CRAN.R-project.org/package=secr/
Efford MG, Boulanger J (2019) Fast evaluation of study designs for spatially explicit capture–recapture. Methods in Ecology and Evolution 10, 1529-1535.
| Crossref | Google Scholar |
Farquharson KA, McLennan EA, Wayne A, Smith M, Peel E, Belov K, Hogg CJ (2021) Metapopulation management of a critically endangered marsupial in the age of genomics. Global Ecology and Conservation 31, e01869.
| Crossref | Google Scholar |
Ferrari S, Cribari-Neto F (2004) Beta regression for modelling rates and proportions. Journal of Applied Statistics 31, 799-815.
| Crossref | Google Scholar |
Finlayson GR, Finlayson ST, Dickman CR (2010) Returning the rat-kangaroos: translocation attempts in the family potoroidae (superfamily Macropdoidea) and recommendations for conservation. In ‘Macropods: the biology of kangaroos, wallabies and rat-kangaroos’. (Eds G Coulson, MDB Eldridge) pp. 245–262. (CSIRO Publishing: Melbourne, Vic., Australia)
Fischer J, Lindenmayer DB (2000) An assessment of the published results of animal relocations. Biological Conservation 96, 1-11.
| Crossref | Google Scholar |
Fleming PA, Anderson H, Prendergast AS, Bretz MR, Valentine LE, Hardy GES (2014) Is the loss of Australian digging mammals contributing to a deterioration in ecosystem function? Mammal Review 44, 94-108.
| Crossref | Google Scholar |
Frick CH, Hole D, Sandow D, Olds LGM, Ostendorf B, Taggart D, Roshier D (2023) The utility of coded very high frequency telemetry for monitoring reintroduced mammal populations. Ecology and Evolution 13, e10193.
| Crossref | Google Scholar | PubMed |
Garkaklis MJ, Bradley JS, Wooller RD (2004) Digging and soil turnover by a mycophagous marsupial. Journal of Arid Environments 56, 569-578.
| Crossref | Google Scholar |
Groom C (2010) Justification for continued conservation efforts following the delisting of a threatened species: a case study of the woylie, Bettongia penicillata ogilbyi (Marsupialia: Potoroidae). Wildlife Research 37, 183-193.
| Crossref | Google Scholar |
Harrison ND, Phillips BL, Mitchell NJ, Wayne JC, Maxwell MA, Ward CG, Wayne AF (2023) Perverse outcomes from fencing fauna: loss of antipredator traits in a havened mammal population. Biological Conservation 281, 110000.
| Crossref | Google Scholar |
Hayward MW, Kerley GIH (2009) Fencing for conservation: restriction of evolutionary potential or a riposte to threatening processes? Biological Conservation 142, 1-13.
| Crossref | Google Scholar |
Johnson CN, Clinchy M, Taylor AC, Krebs CJ, Jarman PJ, Payne A, Ritchie EG (2001) Adjustment of offspring sex ratios in relation to the availability of resources for philopatric offspring in the common brushtail possum. Proceedings of the Royal Society of London. Series B: Biological Sciences 268, 2001-2005.
| Crossref | Google Scholar | PubMed |
Kemp JE, Jensen R, Hall ML, Roshier DA, Kanowski J (2021) Consequences of the reintroduction of regionally extinct mammals for vegetation composition and structure at two established reintroduction sites in semi-arid Australia. Austral Ecology 46, 653-669.
| Crossref | Google Scholar |
Krebs CJ (1966) Demographic changes in fluctuating populations of Microtus californicus. Ecological Monographs 36, 239-273.
| Crossref | Google Scholar |
Kristensen TV, Kovach AI (2018) Spatially explicit abundance estimation of a rare habitat specialist: implications for SECR study design. Ecosphere 9, e02217.
| Crossref | Google Scholar |
Legge S, Woinarski JCZ, Burbidge AA, Palmer R, Ringma J, Radford JQ, Mitchell N, Bode M, Wintle B, Baseler M, Bentley J, Copley P, Dexter N, Dickman CR, Gillespie GR, Hill B, Johnson CN, Latch P, Letnic M, Manning A, McCreless EE, Menkhorst P, Morris K, Moseby K, Page M, Pannell D, Tuft K (2018) Havens for threatened Australian mammals: the contributions of fenced areas and offshore islands to the protection of mammal species susceptible to introduced predators. Wildlife Research 45, 627-644.
| Crossref | Google Scholar |
Linley GD, Moseby KE, Paton DC (2017) Vegetation damage caused by high densities of burrowing bettongs (Bettongia lesueur) at arid recovery. Australian Mammalogy 39, 33-41.
| Crossref | Google Scholar |
Lüdecke D, Makowski D, Waggoner P (2021) Performance: An R Package for Assessment, Comparison and Testing of Statistical Models. Journal of Open Source Software 6(60), 3139.
| Crossref | Google Scholar |
Mattiske EM, Havel JJ (1998) Vegetation Mapping in the South West of Western Australia and Regional Forest Agreement vegetation complexes. Map sheets for Pemberton, Collie, Pinjarra, Busselton-Margaret River, Mt Barker, and Perth, Western Australia. Scale 1:250,000. Department of Conservation and Land Management, Perth.
Morris KD, Page M, Kay R, Renwick J, Desmond A, Comer S, Burbidge A, Kuchling G, Sims C (2015) Forty years of translocations in western Australia: lessons learned. In ‘Advances in reintroduction biology of Australian and New Zealand fauna’. (Eds DP Armstrong, MW Hayward, D Moro, PJ Seddon) pp. 217–235. (CSIRO Publishing)
Moseby KE, Read JL (2006) The efficacy of feral cat, fox and rabbit exclusion fence designs for threatened species protection. Biological Conservation 127, 429-437.
| Crossref | Google Scholar |
Moseby KE, Lollback GW, Lynch CE (2018) Too much of a good thing; successful reintroduction leads to overpopulation in a threatened mammal. Biological Conservation 219, 78-88.
| Crossref | Google Scholar |
Murphy MT, Garkaklis MJ, Hardy GESJ (2005) Seed caching by woylies Bettongia penicillata can increase sandalwood Santalum spicatum regeneration in western Australia. Austral Ecology 30, 747-755.
| Crossref | Google Scholar |
Murphy BP, Woolley L-A, Geyle HM, Legge SM, Palmer R, Dickman CR, Augusteyn J, Brown SC, Comer S, Doherty TS, Eager C, Edwards G, Fordham DA, Harley D, McDonald PJ, McGregor H, Moseby KE, Myers C, Read J, Riley J, Stokeld D, Trewella GJ, Turpin JM, Woinarski JCZ (2019) Introduced cats (Felis catus) eating a continental fauna: the number of mammals killed in Australia. Biological Conservation 237, 28-40.
| Crossref | Google Scholar |
Northover AS, Godfrey SS, Keatley S, Lymbery AJ, Wayne AF, Cooper C, Pallant L, Morris K, Thompson RCA (2019a) Increased Trypanosoma spp. richness and prevalence of haemoparasite co-infection following translocation. Parasites & Vectors 12, 126.
| Crossref | Google Scholar | PubMed |
Northover AS, Thompson RCA, Lymbery AJ, Wayne AF, Keatley S, Ash A, Elliot AD, Morris K, Godfrey SS (2019b) Altered parasite community structure in an endangered marsupial following translocation. International Journal for Parasitology: Parasites and Wildlife 10, 13-22.
| Crossref | Google Scholar | PubMed |
Ostendorf B, Boardman WSJ, Taggart DA (2016) Islands as refuges for threatened species: multispecies translocation and evidence of species interactions four decades on. Australian Mammalogy 38, 204-212.
| Crossref | Google Scholar |
Pacioni C, Wayne AF, Spencer PBS (2011) Effects of habitat fragmentation on population structure and long-distance gene flow in an endangered marsupial: the woylie. Journal of Zoology 283, 98-107.
| Crossref | Google Scholar |
Pacioni C, Hunt H, Allentoft ME, Vaughan TG, Wayne AF, Baynes A, Haouchar D, Dortch J, Bunce M (2015) Genetic diversity loss in a biodiversity hotspot: ancient DNA quantifies genetic decline and former connectivity in a critically endangered marsupial. Molecular Ecology 24, 5813-5828.
| Crossref | Google Scholar | PubMed |
Pacioni C, Williams MR, Lacy RC, Spencer PBS, Wayne AF (2017) Predators and genetic fitness: key threatening factors for the conservation of a bettong species. Pacific Conservation Biology 23, 200-212.
| Crossref | Google Scholar |
Pacioni C, Wayne AF, Page M (2019) Guidelines for genetic management in mammal translocation programs. Biological Conservation 237, 105-113.
| Crossref | Google Scholar |
Pacioni C, Trocini S, Wayne AF, Rafferty C, Page M (2020) Integrating population genetics in an adaptive management framework to inform management strategies. Biodiversity and Conservation 29, 947-966.
| Crossref | Google Scholar |
Page KD, Ruykys L, Miller DW, Adams PJ, Bateman PW, Fleming PA (2019) Influences of behaviour and physiology on body mass gain in the woylie (Bettongia penicillata ogilbyi) post-translocation. Wildlife Research 46, 429-443.
| Crossref | Google Scholar |
Palmer BJ, Valentine LE, Page M, Hobbs RJ (2020) Translocations of digging mammals and their potential for ecosystem restoration: a review of goals and monitoring programmes. Mammal Review 50, 382-398.
| Crossref | Google Scholar |
Palmer BJ, Beca G, Erickson TE, Hobbs RJ, Valentine LE (2021) New evidence of seed dispersal identified in Australian mammals. Wildlife Research 48, 635-642.
| Crossref | Google Scholar |
Priddel D, Wheeler R (2004) An experimental translocation of brush-tailed bettongs (Bettongia penicillata) to western New South Wales. Wildlife Research 31, 421-432.
| Crossref | Google Scholar |
Resende PS, Viana-Junior AB, Young RJ, Azevedo CS (2021) What is better for animal conservation translocation programmes: soft- or hard-release? A phylogenetic meta-analytical approach. Journal of Applied Ecology 58, 1122-1132.
| Crossref | Google Scholar |
Short J (2023) Irruptive dynamics of the brush-tailed bettong (Bettongia penicillata) when reintroduced to a fenced sanctuary with feral cats present. Wildlife Research 50, 85-95.
| Crossref | Google Scholar |
Skogvold K, Warren KS, Jackson B, Holyoake CS, Stalder K, Devlin JM, Vitali SD, Wayne AF, Legione A, Robertson I, Vaughan-Higgins RJ (2017) Infectious disease surveillance in the woylie (Bettongia penicillata). EcoHealth 14, 518-529.
| Crossref | Google Scholar | PubMed |
Smith M, Volck G, Palmer N, Jackson C, Moir C, Parker R, Palmer B, Thomasz A (2020) Conserving the endangered woylie (Bettongia penicillata ogilbyi): establishing a semi-arid population within a fenced safe haven. Ecological Management & Restoration 21, 108-114.
| Crossref | Google Scholar |
Sollmann R, Gardner B, Belant JL (2012) How does spatial study design influence density estimates from spatial capture–recapture models? PLoS ONE 7, e34575.
| Crossref | Google Scholar | PubMed |
Tetzlaff SJ, Sperry JH, DeGregorio BA (2019) Effects of antipredator training, environmental enrichment, and soft release on wildlife translocations: a review and meta-analysis. Biological Conservation 236, 324-331.
| Crossref | Google Scholar |
Threatened Species Scientific Committee (2018) Conservation Advice Bettongia penicillata woylie. Canberra: Department of the Environment and Energy. Available at http://www.environment.gov.au/biodiversity/threatened/species/pubs/213-conservation-advice-01022018.pdf.
Treloar S, Lohr C, Hopkins AJM, Davis RA (2021) Rapid population expansion of boodie (Burrowing Bettong, Bettongia lesueur) creates potential for resource competition with mala (Rufous Hare-wallaby, Lagorchestes hirsutus). Ecological Management & Restoration 22, 54-57.
| Crossref | Google Scholar |
Wayne AF, Maxwell MA, Ward CG, Vellios CV, Ward BG, Liddelow GL, Wilson I, Wayne JC, Williams MR (2013a) Importance of getting the numbers right: quantifying the rapid and substantial decline of an abundant marsupial, Bettongia penicillata. Wildlife Research 40, 169-183.
| Crossref | Google Scholar |
Wayne AF, Maxwell MA, Ward CG, Vellios CV, Wilson I, Wayne JC, Williams MR (2015) Sudden and rapid decline of the abundant marsupial Bettongia penicillata in Australia. Oryx 49, 175-185.
| Crossref | Google Scholar |
Wayne AF, Maxwell MA, Ward CG, Wayne JC, Vellios CV, Wilson IJ (2017) Recoveries and cascading declines of native mammals associated with control of an introduced predator. Journal of Mammalogy 98, 489-501.
| Crossref | Google Scholar |
Woinarski JCZ, Burbidge AA, Harrison PL (2015) Ongoing unraveling of a continental fauna: decline and extinction of Australian mammals since European settlement. Proceedings of the National Academy of Sciences 112, 4531-4540.
| Crossref | Google Scholar |
Yeatman GJ, Wayne AF (2015) Seasonal home range and habitat use of a critically endangered marsupial (Bettongia penicillata ogilbyi) inside and outside a predator-proof sanctuary. Australian Mammalogy 37, 157-163.
| Crossref | Google Scholar |
Zosky KL, Wayne AF, Bryant KA, Calver MC, Scarff FR (2017) Diet of the critically endangered woylie (Bettongia penicillata ogilbyi) in south-western Australia. Australian Journal of Zoology 65, 302-312.
| Crossref | Google Scholar |