Pirra Jungku and Pirra Warlu: using traditional fire-practice knowledge and contemporary science to guide fire-management goals for desert animals
Sarah Legge


A
B
C
D
E
F
G
H
I
Handling Editor: Marlee Hutton
Abstract
Indigenous people influenced fire in Australia’s deserts for millennia, until colonisation interrupted traditional fire practices. Many groups are reinvigorating those practices to achieve inter-linked biodiversity, cultural, and social benefits. Contemporary fire management integrates modern planning and delivery approaches with cultural process. However, deserts have changed since colonisation (e.g. invasive species, biodiversity loss, climate change) and fire-management outcomes for Country are less certain. Some Indigenous groups are integrating scientific methods into their programs, to examine biodiversity outcomes and refine management.
Karajarri and Ngurrara Traditional Owners wanted to understand how fire affects Kuwi (mammals, reptiles) on their Country, to guide fire management. Karajarri and Ngurrara Rangers worked together on this project, exchanging skills and knowledge with each other, and with scientists.
We established 32 monitoring sites at four locations on Karajarri and Ngurrara Country. We undertook 11 survey trips from 2018 to 2022, gathering data from 81 site visits. Using Landsat satellite imagery we described fire patterns around each site, focussing on seral stage (as defined by Traditional Owners), patch size, and seral diversity at small (500 m), medium (1 km), and large (5 km) spatial scales.
There were 378 mammal captures (11 species) and 3392 reptile captures (66 species). Mammal species richness and captures were lowest in Wuntara (recently burnt) and highest in Nyirrinyanu (mature) spinifex. Mammal species richness increased with seral diversity within 1 km around the trapping site. Reptile species richness and captures were unaffected by seral stage. However, many species were caught significantly more often in specific seral stages. Neither reptile richness nor capture rate were related to patch size or seral diversity.
Retaining a mix of seral stages can maintain diverse reptile and mammal assemblages. The management challenge will be increasing the extent of mature/long-unburnt spinifex, currently the rarest seral stage. Ranger fire management has already reduced mean fire size by 40–52%, and further reductions are possible.
Our work improves the understanding of fire effects on northern desert fauna, guides management, provides important ecological information from a little-studied region of Australia’s deserts, and increases the recognition of Indigenous conservation management of Country.
Keywords: adaptive management, biodiversity monitoring, Caring for Country, desert fauna, fire ecology, fire management, Indigenous conservation management, Indigenous rangers, species succession.
Short summary and translation |
Karajarri and Ngurrara Rangers collaborated with scientists to investigate how fire management in the Great Sandy Desert could help mammals and reptiles. We found that some species prefer particular spinifex post-fire ages, from recently burnt to long-unburnt, and that patchworks of spinifex ages benefit mammals. This means that we should aim to reduce fire size and increase the area of mature/long-unburnt spinifex. Our work provides ecological information from a little-studied region of Australia, and highlights the role of Indigenous land managers in biodiversity conservation. |
Translated summary: The two ranger groups have decided not to translate the summary into their languages. Although there are many language speakers from Ngurrara, the most fluent Karajarri speaker passed away last year, and translating complex sentences is now challenging. Out of mutual respect for their collaboration, the rangers prefer not to include a summary that is only in a Ngurrara language. However, we use words from both languages in the text below. A short video about our project can be seen here. |
Introduction
Fire has been part of Australia’s ecosystems, including its deserts, through evolutionary time (Martin 2006). Compared with the deserts of other continents, Australian deserts experience fire frequently (Morton et al. 2011) for the following four reasons: they experience slightly higher rainfall; the rainfall is strongly seasonal (especially in northern deserts); the vegetation is dominated by a highly flammable grass (spinifex, Triodia spp.); and lightning ignitions are common (Edwards et al. 2008; Pausas and Keeley 2009; Bradstock 2010). Average fire frequencies depend on annual rainfall and its seasonality, and vegetation type, varying from once every 3–4 years in north-western deserts where rainfall is highest, to once every 20–30+ years in southern deserts.
Following their arrival to the deserts about 50,000 years ago, Aboriginal peoples developed fire practices for many uses, including to manage resources, signal to neighbours, protect sites from wildfire, and ‘clean up’ Country (Gould 1971; Kimber 1983; Lowe 2002; Bird et al. 2005; Pike 2008). Cultural protocols determined who burned, and when, according to seasonal calendars and rainfall. These fire practices influenced the fire regime (Bowman 1998) by increasing the number of ignitions, broadening the seasons when fire occurred, decreasing fire size and severity, and increasing fine-scale seral diversity (Burrows and Christensen 1991; Burrows et al. 2006; Blackwood et al. 2022). People used deserts unevenly through space and time, so some regions may have been burnt intensively by people over some periods, whereas fire was probably applied sparingly in other regions and periods. Thus, the fire regimes at any location were likely to be an interplay between the extent of Indigenous burning and the influence of climate on fire activity (Bliege Bird et al. 2012, 2016; Wright et al. 2021).
With European colonisation, the Australian deserts were depopulated over a short timeframe. The people-managed fire regime was replaced (at least in some areas, and times, Wright et al. 2021) by a wildfire-dominated system of extensive, severe fires, that regularly exceed 10,000 km2 in size (Edwards et al. 2008; Turner et al. 2011). This change in a key disturbance regime may have contributed to declines of many desert plant and animal species (Allan and Southgate 2002; Greenwood et al. 2022). The most profound declines occurred in small to medium-sized mammals; about 60% of species in this group have become extinct since colonisation, and many other species have undergone marked range contractions (Burbidge and McKenzie 1989; McKenzie et al. 2007; Woinarski et al. 2015). As well as the direct changes to habitat quality wrought by the fire regime shift, the arrival of introduced species, and interactions between fire and invasives, are likely to have led to these declines. For example, frequent, severe fire can facilitate predation by introduced cats (Felis catus) and foxes (Vulpes vulpes), leading to population decline in native species (Lundie-Jenkins 1993; Short and Turner 1994; Leahy et al. 2016; Murphy et al. 2018; Doherty et al. 2022).
For desert Indigenous people, traditional fire practice is an indivisible component of healthy Country. Traditional fire practice protects tangible cultural and environmental assets, and intangible values such as intergenerational transfer of cultural and ecological knowledge, maintenance of cultural authority, and connection to Country (Weir et al. 2011; Cameron 2020). For many Indigenous groups, traditional fire use has been adapted into a contemporary context, incorporating new technologies (e.g. aerial incendiary, remote sensing of burnt areas) and coordinated management programs that typically aim to restore ‘right-way fire’ across traditional lands, for intertwined biodiversity and cultural outcomes (Yanunijarra Aboriginal Corporation 2012; KTLA 2014; Putnis et al. 2021; Ruscalleda-Alvarez et al. 2023).
Desert Indigenous people have a deep knowledge of how fire affects Country and culturally significant species, including bushmeat species (Lowe 2002; Pike 2008). However, Country has changed through the introduction of new predators (cats, foxes), herbivores (e.g. camels Camelus dromedarius) and weeds (e.g. buffel grass, Cenchrus ciliaris), and the direct and interacting effects of climate change, which means that traditional practice is adapting to the contemporary context. Moreover, although the new approaches used to complement on-ground burning, such as aerial incendiary, are invaluable for enabling small teams of Indigenous rangers to implement fire management over very large, remote areas (>3000 km2), the resulting fire patterns differ from those arising from traditional fire practices (Perry et al. 2018; Ruscalleda-Alvarez et al. 2023). Consequently, the impacts of contemporary fire management for biodiversity are less certain.
Context for this study
Scientific knowledge about the effects of fire on much of the Australian desert fauna self-evidently lags behind Indigenous traditional knowledge, but the scientific method is being increasingly used by Indigenous groups to support their goals for managing fire on Country, in the same way that contemporary operational technologies have been incorporated into traditional fire practice. Indigenous rangers and Traditional Owners are collaborating with scientists to understand and measure how their management affects biocultural assets, and to use this information to adapt management.
In the Pirra Jungku/Pirra Warlu project, the Karajarri Rangers initiated a collaboration with scientists from Environs Kimberley, the National Environmental Science Program’s Threatened Species Recovery Hub, Western Australia’s Department of Biodiversity, Conservation and Attractions, and the Indigenous Desert Alliance, to understand how fire affects desert fauna, so that this information could be used to fine-tune management targets and report on management outcomes to their community and funders. Within a year, the Ngurrara Rangers joined the project. The ranger teams led the project, by setting the research questions and leading the surveys, and organising permits (Karajarri and Ngurrara were the first ranger teams in Western Australia to hold ethics and scientific licences). The project is designed as two-way science, where Indigenous knowledge of Country and scientists’ technical expertise are shared to enhance the partnership and understanding.
Karajarri and Ngurrara fire management focusses on achieving the key attributes of ‘right-way fire’ described by desert peoples: many small fires, creating high seral diversity over given areas, that includes some mature and long-unburnt vegetation (Yanunijarra Aboriginal Corporation 2012; KTLA 2014; IDA 2023; Ruscalleda-Alvarez et al. 2023). As part of the Pirra Jungku project, we carried out an analysis of historical aerial photographs over Karajarri Country from the 1940s, when people were still living traditional nomadic lifestyles. This work described the fire sizes and patch density created by traditional fire practices; fires were a tenth of the size they are today, seral diversity in a given area was higher, and there was more mature and long-unburnt vegetation, making the average post-fire vegetation age almost 50% older than in 2020 (Blackwood et al. 2022). Given these differences, the ranger teams wanted to know what the implications for desert fauna were, and whether they needed to strive for even smaller fires and finer-scale mosaics. ‘We want to see what animals are here, check out if the Country is in good shape, and if fire management is working’ [Sheen Kitty].
Here, we use data collected over 5 years of monitoring on Karajarri and Ngurrara Country to understand how mammals and reptile communities respond to fire, especially to variation in the age and size of seral stages, and to seral diversity. We focussed on these ground-dwelling groups because we expected them to be most sensitive to changes in ground-layer structure caused by fire. This purpose of the study is to inform key targets for the rangers’ fire-management programs, including fire sizes to aim for when conducting prescribed burning, and whether creating fine-scale seral mosaics and increasing the extent of long-unburnt vegetation is important. The Great Sandy Desert has been little-surveyed by scientists. The only broad-scale sampling within our study area was conducted over 40 years ago (McKenzie 1981), and our information on species present adds to fundamental scientific knowledge about species’ distributions.
Methods
Language conventions: we introduce some Karajarri and Ngurrara words in underlined and bold font respectively. Where words from both languages are used, we present them in the order Karajarri/Ngurrara.
Study area
The study was conducted in the Great Sandy Desert of north-western Australia, between 2018 and 2022. The Karajarri Native Title Determination covers 32,000 km2 south and inland from Bidyadanga and 80 Mile Beach (Fig. 1). Most of the determination (25,000 km2) is designated as an Indigenous Protected Area. Conservation and cultural management is undertaken by Karajarri Rangers, with oversight from elders. The Ngurrara Native Title Determination neighbours Karajarri’s eastern boundary and extends south of Fitzroy Crossing (Fig. 1). It covers 76,000 km2 and includes the Warlu Jilajaa Jumu Indigenous Protected Area (16,400 km2). The Ngurrara Rangers look after Ngurrara Country, under the direction of their cultural leaders. In this part of Australia, people living a nomadic lifestyle left the deserts late compared with most other regions. The main exodus of the Ngurrara and Karajarri people to coastal missions and pastoral stations occurred between the 1900s and 1960s, with the final departures occurring in the early 1980s (Lowe 2002; Bent et al. 2016). ‘My grandmother used to travel through here. She told us lots of stories from these places, and she can remember animals that are gone now’ [J. Shovellor].
The Karajarri and Ngurrara Native Title Determinations in the Great Sandy Desert of north-western Australia. The grey shading shows fire frequency over 20 years (2000–2020). The left map shows the four trapping locations, and the annual rainfall isohyets (http://www.bom.gov.au/climate/maps/averages/rainfall/).
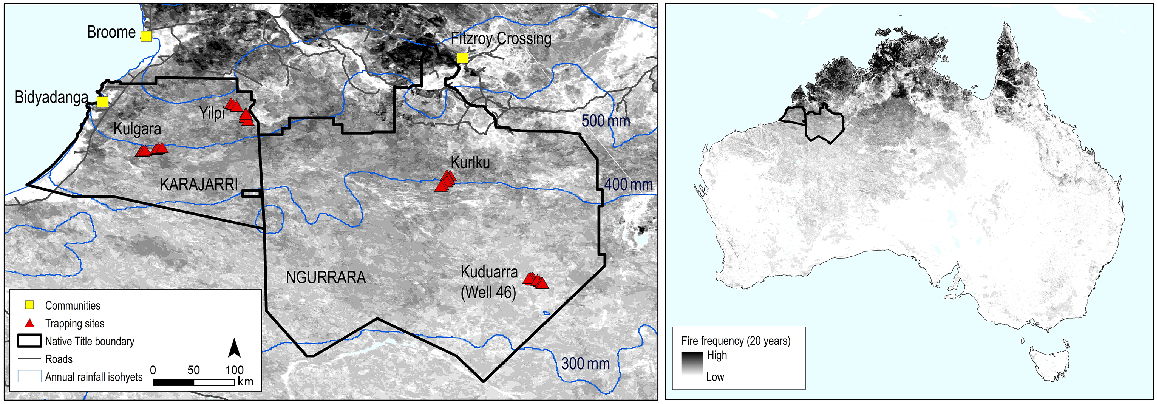
Rainfall in these north-western deserts is influenced by the tropical monsoon. Rainfall is therefore higher, and more seasonal than in more southerly deserts, but remains variable among years. Karajarri, being closer to the coast, receives more rain than Ngurrara. Annual rainfall since 1980 has averaged 502 mm (range 124–1093 mm) for Karajarri, and 403 mm (range 110–698 mm) for Ngurrara (based on interpolated rainfall datasets from SILO (https://www.longpaddock.qld.gov.au/silo/), with the points for each selected as midway between the locations sampled in this study). Most rain (>90%) falls between December and March when the tropical monsoon dips furthest to the south (Supplementary material Fig. S1).
We conducted our study at two locations (Yilpi and Kulgara) on Karajarri Country, and two locations (Kuduarra and Kurlku) on Ngurrara Country (Fig. 1). The vegetation at all four locations is Maranguru/Pirntirri (but usually called Jitapuru), spinifex (Triodia spp.) sandplains, bordering low Niyamarri/Jilji, sand dune country. This is a dominant vegetation group in the Australian deserts (Thackway and Cresswell 1995). Acacias and low trees (4–6 m high) are scattered sparsely, with acacias sometimes forming small, closed canopy thickets that can shade out spinifex (Fig. S2). Vegetative growth in these spinifex deserts is determined by rainfall, with biomass being strongly correlated with the accumulated rainfall since the last fire (Ruscalleda-Alvarez et al. 2023). Once spinifex clumps reach sufficient size, fires can travel easily among them. Generally, this occurs every 4–5 years on Karajarri Country, and every 5–8 years on Ngurrara Country, but with a gradient in fire frequency that corresponds to variation in rainfall (Fig. 1).
Fire management
Fire was used intensively by nomadic Karajarri and Ngurrara peoples, to aid with hunting, promote certain food plants (e.g. Jilarlgka, bush tomato, Solanum spp.; Yukurli, dodder Cassytha filiformis; Jampar, bush orange, Capparis umbonata; Mungkarliny, bush potato, Ipomoea costata; Yarrinyarri, bush onion, Cyperus bulbosus), communicate, ease passage, and ‘clean up’ Country. Maintaining fire practices is considered critical for supporting knowledge transfer, cultural identity, and the health of Country. Burning from the ground is now being supplemented by aerial incendiary techniques, with burning conducted each year in Marul/Makurra season (early dry, just after rainy season), to limit the spread of fire in Laja/Parranga (hot season), according to a burn plan that is approved by cultural leaders. Both ranger teams have increased the scale and intensity of their fire management since 2019, with the aim of maintaining biodiversity, cultural practice, and the health of Country. ‘In this desert Country, my grandfather’s Country, we have lots of story places, but it’s been hard to get to these areas in the last years to look after things. Now, with helicopters for fire management we can reach places we can’t get to with the vehicle.’ [B. Taylor]. Since landscape-scale, coordinated fire management began in 2019, the mean fire size has been reduced by 52% (to 25.1 km2) on Karajarri Country and 40% (to 21.6 km2) on Ngurrara Country (Karajarri Rangers, Ngurrara Rangers, unpubl. data). The mean fire-return interval across Karajarri and Ngurrara between 2000 and 2023 was 5.2 and 7.1 years respectively (Fig. S1).
Trapping-study design
We set up eight trapping sites to sample Kuwi, mammals and reptiles, at each of four locations (i.e. 32 sites in total) (Fig. 1). At each location, sites were positioned 50–500 m off an unsealed access track, stretching over a length of 18–31 km, with sites at least 1 km apart but usually >2 km apart. Sites were established in diverse seral stages, from recently burnt to long-unburnt, and resampled as they aged (Table 1). Eight sites were burned during the study, causing sudden seral-stage changes (five in prescribed burns, three in wildfires). We sampled one to three locations (i.e. 8–24 sites) each year between 2018 and 2022, with each of the four locations being sampled in 2–4 separate years. We undertook surveys at two times of year, namely early dry (April/May), and late dry (September/October), when access is not prevented by rain and temperatures are still high, favouring reptile activity.
English name for seral stage | Karajarri name for seral stage | Ngurrara name for seral stage | [Photographic representation] | |
---|---|---|---|---|
Recently burnt | Jalalu | Wuntara, Nyunma; Waruwaru | ![]() | |
Wuntara: ‘after a fire has gone through’ Waruwaru: ‘after a fire and a little bit of regrowth’
| ||||
Recovering | Wiyapuka | Parrawa | ![]() | |
‘Laparnwarti jitapuru, getting mature spinifex, area where grass has been burnt within the past 3 years or so and spinifex is regrowing but still easy to see turkeys and other bushmeat’
| ||||
Mature | Nyirrinyanu | ![]() | ||
‘Yitilijanka jitapura, grass is dense and mature, stops the hunter from walking and pierces his skin’
| ||||
Long-unburnt | Yurnara | ![]() | ||
‘Jarlujangka jitapuru, old spinifex from a long time ago, ready for burning again’
|
Note the year ranges are slightly different on Ngurrara because it receives less rainfall than does Karajarri. The number of site-samples in each seral stage is shown. In the text, we use the Ngurrara names for seral stages. More photographic examples of habitat and seral stages at sites are in Fig. S2.
At each site, we opened 10 20-L pitfall buckets, four funnel traps (79 cm × 18 cm × 18 cm; Professional Trapping Supplies), and four camera-traps (SWIFT Enduros) set along two parallel 50–60-m-long and 30-cm-high aluminium flywire drift fences set 50 m apart, within a 0.25-ha quadrat. We placed a layer of sand and egg cartons inside pitfalls for shelter, covered with discs of reflective roofing insulation to reduce overheating risk, and give extra protection to small reptiles from carnivorous mammals. We covered funnels with a 30-cm layer of spinifex, held down with weighted shade-cloth. The camera-traps were strapped to a stake that angled at about 20° off vertical; the cameras were 20–30 cm from the ground and trained onto the drift fences at about 45° off the perpendicular. They were baited with oats and peanut butter balls to attract small mammals. Surveys were undertaken usually for 4 days, but occasionally up to 7 days, with the survey effort accounted for in the statistical analysis where appropriate. Traps were checked at dawn, and late afternoon, each day.
Animals captured in pitfall and funnel traps were identified to species. Captured animals were usually released immediately near their point of capture; on occasions when identification was challenging, animals were held for a few hours for other team members to verify identification. All detections of the same species on the same camera-trap in one 12-h (day or night) period were considered as a single capture, to mirror captures in the pitfalls and funnel traps, where an individual can be caught only once in a 12-h period. When small mammals were difficult to identify in camera-trap images, we grouped species. For example, very small rodents, Jilku/Punypuny, were either sandy inland mice or western delicate mice (P. hermannsburgensis, Pseudomys pilbarensis sp. nov.) (Roycroft et al. 2024); distinguishing these species is challenging even when they are in the hand, so we grouped all captures of small rodents into one ‘species type’. Distinguishing between stripe-faced and lesser hairy-footed dunnarts (Sminthopsis macroura, S. crassicaudata) from images was not possible (thus, grouped to Sminthopsis sp.). Sometimes mammals could be identified only as ‘native rodent’, or even ‘mammal’. In these cases, the capture could be used in analyses of overall capture rates but was excluded from species richness analyses when it would artificially inflate the value (e.g. if the only mammal detected at a site was Sminthopsis sp., the site had a species richness of one; if the site returned both S. macroura and S. sp., the site species richness was still one).
Overall, we conducted 11 survey trips, gathering data from 81 site-surveys (on three surveys, we opened only 4, 6, or 7 sites of the set of 8 for logistical reasons), and ~7200 trap-nights (accounting for some trap closures to manage ants or heat). During 74 site-surveys (i.e. all but seven of the full set), we estimated the characteristics of the ground cover at each site by walking in random lines though the site for about 100 steps and recording whether each toe-fall pointed to bare ground, leaf and woody litter, or grass and herbs, then calculating percentages for these categories.
Trapping trips were guided by two-way science principles. Rangers were trained in, and co-led, scientific aspects of the survey, including understanding the question and management context, the practices of trap-checking, species identification using taxonomic keys and guidebooks, and data entry. Capture totals for each species, in each seral stage, were tallied each day and added to a large scoreboard, so that rangers could see results accumulating in real time. Scientists on the survey learned cultural knowledge where appropriate, including names, stories, and cultural practices such as burning and hunting. A short video describing the trapping surveys is available here.
Fire history
We used Landsat satellite imagery to describe the fine-scale fire patterns around each site. Imagery back to 2008 for Ngurrara (Landsat 5, 7, 8, 9), and back to 2013 for Karajarri (Landsat 6, 7), was downloaded from the United States Geological Survey (https://earthexplorer.usgs.gov/). Fire scars were mapped approximately every 2 months by creating a difference image by using cloud-free images. A manual threshold was set for each image (or each portion of an image if there was a lot of variation within a single difference image) to classify areas of change, i.e. areas that burnt during the two consecutive time periods. We then manually cleaned out false positives owing to cloud cover, water, or other landscape features before exporting the burnt areas. For resourcing reasons, the Landsat series for Karajarri went back only to 2013, which meant that, for sites trapped in 2018, seral stages older than 5 years would be merged. To gain a longer time series we supplemented the Landsat data by downloading processed MODIS imagery for earlier years from the North Australia Fire Service (www.firenorth.org.au/nafi3), so that in every year of trapping, we had a time series of at least 8 years. MODIS imagery has lower spatial resolution than does Landsat (250 vs 30 m). By missing small fire scars, MODIS potentially overestimates fire size and underestimates seral heterogeneity relative to Landsat (Ruscalleda-Alvarez et al. 2021). Nevertheless, the value of gaining a longer time series for the sites trapped in earlier years caused us to combine information from the two imagery sources.
Following Ruscalleda-Alvarez et al. (2023), we calculated fire metrics relating to the seral stage (time since fire), patch size, and seral diversity at different scales, for each site, and every year it was sampled. Time since fire was first calculated as the number of wet seasons since the last fire. If a site was sampled in September after a fire burned in May of the same year, the time since fire was zero, but if the fire occurred in May of the previous year, the time since fire was one. These values were then transformed into one of four seral stages that are recognised by Traditional Owners (Table 1), with slight variations between Karajarri and Ngurrara, reflecting the lower rainfall, and thus slower vegetative recovery, of the latter. Seral stages as shown by satellite imagery were validated during surveys at sites. The patch size was the area in hectares of the same-aged vegetation that contained the trapping site. To estimate seral diversity, we calculated the number of vegetation patches of different time-since-fire in buffers around each trapping site of 500 m (small scale), 1 km (medium scale), and 5 km (large-scale) radii. Spatial data manipulation was conducted using ArcMap 10.8.1.
Data preparation and analysis
For a subset of site-surveys (n = 74), we used ANOVA (in JMP statistical software: https://www.jmp.com/en_au/software.html) to compare the proportion of the ground that was bare, covered in leaf and woody litter, or covered by grass, across the seral stages.
We examined the immediate effect of fire on species richness and capture rates, using ANOVA (in JMP) to compare the change in richness and captures (for reptiles and mammals separately) at the eight sites that were burnt between consecutive surveys, versus sites that were sampled in two consecutive surveys without intervening fire.
For each survey, and for native mammals and reptiles separately, we calculated the species richness (number of species detected in each class), and the daily capture rate (total captures divided by duration of survey). We did not adjust species richness by survey duration because examination of species accumulation over the survey period showed that 90% of species are captured at least once by the end of the 4th day.
To examine variation in species richness and daily capture rates of mammals and reptiles, we used linear regression mixed models using glmmTMB package v. 1.1.9 (available at https://doi.org/10.32614/CRAN.package.glmmTMB) with an underlying Gaussian distribution. We examined model assumptions using DHARMa package v. 0.4.6 (available at https://doi.org/10.32614/CRAN.package.DHARMa). To improve the dispersion of residuals in capture-rate models, the square root (mammals) and log (reptiles) of daily capture rates were used as the response variable. Site was fitted as the random term to control for repeat sampling at sites. We examined eight potential fixed effects, including location (Yilpi, Kulgara, Kuduarra, Kurlku), season (early dry, late dry), year (2018, 2019, 2020, 2021, 2022), rainfall in the previous wet season (mm; estimated using interpolated rainfall surface from SILO), seral stage (recently burnt, Wuntara; recovering, Parrawa; mature: Nyirrinyanu; long-unburnt: Yurnara), patch size (logged), and seral diversity at the small, medium and large scale (continuous variables). We assessed fixed effects for collinearity. Patch size was correlated (r > 0.5) with the seral diversity metrics (especially small and medium scale), and the seral diversity metrics of each scale were correlated with each other (especially small and medium scale), so these variables were not included within the same model. We built candidate models using forward selection of the non-correlated fixed effects and the interaction between location and season. Models were evaluated using the Akaike information criterion corrected for small samples (AICc); best-ranked models were those with the smallest AICc and others with a ΔAICc within two of the best model. When two or more models ranked highly, we undertook model averaging using MumIn package v. 1.47.5 (available at https://doi.org/10.32614/CRAN.package.MuMIn). Using emmeans package v. 1.10.1 (available at https://doi.org/10.32614/CRAN.package.emmeans) we undertook post-model tests to (1) determine the overall importance of fixed effects and (2) to examine pairwise comparisons that adjust P-values using a Tukey method to reduce the probability of making a Type I error. We created figures using Sigmaplot and ggplot2 package v. 3.5.0 (https://doi.org/10.32614/CRAN.package.ggplot2) and analysis was undertaken using R 4.3.3 (R Core Team 2024).
Using the same analytical approach as for species richness and total capture rates, we explored the responses of individual species to seral succession and diversity. For mammals we investigated the presence/absence of species caught at more than 30% of site-surveys by using a binomial link function. For reptiles, where a larger number of species were caught at a larger number of site-surveys, we were able to fit models to explore variation in abundance. For species that were sufficiently frequently encountered (caught at >50% of the site-surveys), we assessed daily capture rates adjusted with a square-root transformation where needed, to improve the dispersion of residuals. For one species, we used a negative binomial distribution by using daily counts, with an offset of the logged survey duration to improve the dispersion of residuals.
Many Kuwi, mammal and reptile species were caught at less than 30 and 50% of sites, making the analyses described above not possible. We therefore extended our exploration of the seral preferences of species (caught at least eight times) by summing their captures in each of the four seral stages and using chi-squared heterogeneity tests (in JMP) to compare the observed captures against the expected (their total captures split between seral stages according to the proportions in which they were sampled). Correct identification to species is difficult for Proablephurus reginae/tenuis, and Gehyra kimberlyensis/purpurescens; the species in each case have similar ecologies, so we grouped these captures for analysis. We caught several species of delma (Delma borea/butleri/desmosa/nasuta/tincta) each with a low capture total, and we grouped these for analysis.
We also considered whether rare, or rarely detected species (i.e. those caught <6 times across all 81 surveys) were more likely to occur in a seral stage, by testing whether the distribution of summed captures across the stages conformed to expectation, by using a chi-squared heterogeneity test.
Results
Fire effects on ground cover
Ground characteristics varied across seral stages. The proportion of bare ground was highest in Wuntara (recently burnt, 62%), and lowest in Yurnara (long-unburnt) (F3,70 = 9.82, P < 0.0001; Fig. 2). The proportion of the ground covered by grass or herbs was lowest in Wuntara (recently burnt), higher in Parrawa (recovering), Nyirrinyanu (mature), and Yurnara (long-unburnt) (F3,70 = 4.64, P = 0.005; Fig. 2). Leaflitter was lowest during Wuntara and Parrawa, and highest in Yurnara (F3,70 = 3.89, P = 0.01; Fig. 2).
Changes in ground cover (Walyara, bare ground; leafy and woody litter; and grass-herb, Maramarr, Marranguru/Jitapura) as vegetation recovers after fire from recently burnt Wuntara, to recovering Parrawa, to mature Nyirrinyanu, then to long-unburnt Yurnara. Graphs present means with standard errors for site-surveys in each seral stage. Sample for each seral stage = 10, 37, 19, 8 respectively.
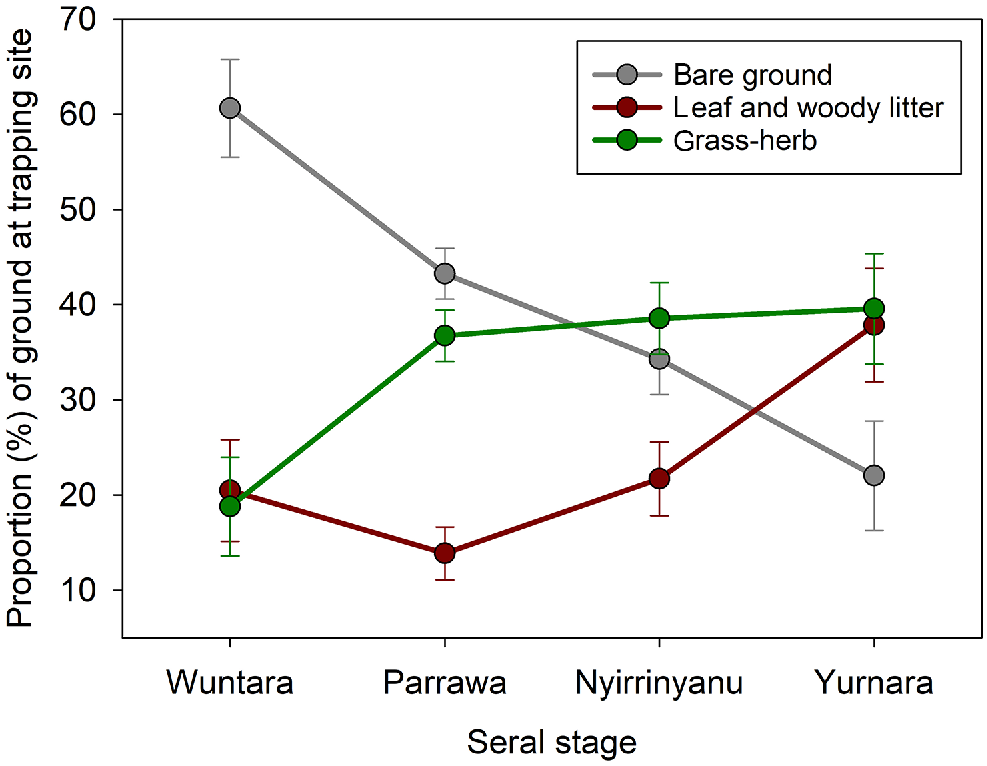
Mammal and reptile captures
We recorded 378 mammal captures, and 3392 reptile captures, over the study (5.3 and 47% trapping success respectively). Most mammal captures were on camera-traps (56%), followed by pitfalls (44%). A single mammal was caught in a funnel-trap. Most reptile captures were in pitfalls (82%), and funnel-traps (13%), with 4% of captures on camera-traps. We caught 11 different mammal species (Supplementary material Table S1a). The five species captured most often were Pseudomys hermannsburgensis/pilbarensis sp. nov, Notomys alexis, Sminthopsis youngsoni, Leggadina lakedownensis, and Pseudomys desertor. Two introduced species, feral cats Minyawi/Minyawi and European foxes Ngatin/Kirlmarrara were also caught on camera-traps (nine captures each, where a capture could be one to multiple detections in a 12-h period), but these captures were omitted from analyses. We caught 65 different reptile species (Table S1b); the five reptiles captured most were Lerista bipes, Pampirta/WijiCtenophorus isolepis, Pantily/WakuraDiplodactylus laevis, Liwirungu/WurrkarnCtenotus pantherinus and Pantily/WakuraLucasium stenodactylum.
Immediate effect of fire at sites
Changes in reptile species richness and daily capture rates at sites that burnt between consecutive surveys were not significantly different from the changes at sites that did not burn between consecutive surveys, and none of the changes were significantly different from zero (richness: F1,45 = 1.59, P = 0.29; captures: F1,45 = 0.26, P = 0.61; Fig. 3). Sites that burned experienced a significant reduction in mammal daily capture rates (F1,45 = 5.70, P = 0.021) but not species richness (F1,45 = 0.37, P = 0.55; Fig. 3).
Species richness and overall abundance with seral succession and diversity
Overall species richness ranged from 0 to 4 across all the site-surveys. The best models for mammal species richness included season, seral stage, rainfall, and seral diversity (Table 2a). Species richness significantly increased with rainfall, was highest in Parrawa and Nyirrinyanu (recovering and mature) seral stages, and increased with seral diversity at the medium scale (1 km) (Table 3a, Fig. 4). Species richness tended to increase in the early dry, but the effect was not significant (Table 3a).
Response variable | Best ranked models | AICc | |
---|---|---|---|
(a) Mammals | |||
Species richness | Season + seral_stage + rainfall + PD_1km + (1|site) | 199.6 | |
Daily capture rate | Seral_stage + (1|site) | 109.00 | |
Seral_stage + season + (1|site) | 110.03 | ||
Seral_stage + rainfall + (1|site) | 108.21 | ||
(b) Reptiles | |||
Species richness | Location × season + (1|site) | 396.24 | |
Location × season + rainfall + (1|site) | 397.54 | ||
Location × season + log(patch_size) | 367.12 | ||
Location × season + rainfall + log(patch_size) + (1|site) | 398.08 | ||
Daily capture rate | Location × season + rainfall + PD_500m + (1|site) | 44.50 | |
(c) Individial species | |||
Pseudomys hermannsburgensis/pilbarensis sp. nov. Jilku/Punypuny | PD_500m + (1|site) | 107.56 | |
PD_500m + season × location + (1|site) | 105.88 | ||
PD_500m + season × location + seral_stage + (1|site) | 107.09 | ||
PD_500m + season × location + seral_stage + rainfall + (1|site) | 107.09 | ||
Notomys alexis Jilku/Punypuny | Location + (1|site) | 73.27 | |
Location + rainfall + (1|site) | 74.99 | ||
Location + PD_1km + (1|site) | 73.44 | ||
Location + PD_1km + rainfall + (1|site) | 75.22 | ||
Lucasium stenodactylum Pantily/Wakura | Seral_stage + rainfall + (1|site) | 81.30 | |
Seral_stage + rainfall + log(patch_size) + (1|site) | 81.17 | ||
Seral_stage + rainfall + log(patch_size) + season + (1|site) | 82.95 | ||
Ctenotus pantherinus Liwirungu/Wurrkarn | Location + year + (1|site) | 102.82 | |
Location + year + seral_stage + (1|site) | 102.94 | ||
Diplodactylus laevis Pantily/Wakura | Location × season + rainfall + (1|site) | 341.47 | |
Location × season + rainfall + seral_stage + (1|site) | 341.91 | ||
Location × season + rainfall + log(patch_size) + (1|site) | 341.29 | ||
Location × season + rainfall + seral_stage + log(patch_size) + (1|site) | 343.03 | ||
Ctenophorus isolepis Pampirta/Wiji | Location + year + seral_stage + season + (1|site) | 122.18 | |
Lerista bipes Winkujartu ‘lice-eating snake’ | Location × season + PD_500m + rainfall + (1|site) | 117.67 | |
Location × season + PD_500m + rainfall + seral_stage + (1|site) | 118.40 |
The model sets include the best model, and any other models within 2 AICc.
Response variable | Fixed effect | Df1 | Df2 | F ratio | P-value | |
---|---|---|---|---|---|---|
(a) Mammals | ||||||
Species richness | Season | 1 | 72 | 3.56 | 0.0633 | |
Seral_stage | 3 | 72 | 9.09 | <0.0001 | ||
Rainfall | 1 | 72 | 5.57 | 0.0210 | ||
PD_500m | 1 | 72 | 5.00 | 0.0285 | ||
Daily capture rate | Seral_stage | 3 | 74 | 7.00 | 0.0003 | |
Season | 1 | 74 | 0.12 | 0.7248 | ||
Rainfall | 1 | 74 | 1.00 | 0.3206 | ||
(b) Reptiles | ||||||
Species richness | Location | 3 | 69 | 23.51 | <0.0001 | |
Patch size | 1 | 69 | 0.37 | 0.5454 | ||
Rainfall | 1 | 69 | 0.28 | 0.5981 | ||
Season | 1 | 69 | 3.07 | 0.0842 | ||
Location:season | 3 | 69 | 6.81 | 0.0004 | ||
Daily capture rate | Location | 3 | 69 | 24.50 | <0.0001 | |
Season | 1 | 69 | 22.08 | <0.0001 | ||
Rainfall | 1 | 69 | 4.97 | 0.0291 | ||
PD_500m | 1 | 69 | 5.21 | 0.0256 | ||
Location:season | 3 | 69 | 12.81 | <0.0001 | ||
(c) Individual species | ||||||
Pseudomys hermannsburgensis/pilbarensis sp. nov. Jilku/Punypuny | Seral_stage | 3 | 67 | 0.13 | 0.9434 | |
Location | 3 | 67 | 0.58 | 0.6315 | ||
PD_500m | 1 | 67 | 4.02 | 0.0489 | ||
Rainfall | 1 | 67 | 0.01 | 0.9267 | ||
Season | 1 | 67 | 0.25 | 0.6162 | ||
Location:Season | 3 | 67 | 0.84 | 0.4781 | ||
Notomys alexis Jilku/Punypuny | Location | 3 | 74 | 4.97 | 0.0034 | |
PD_1km | 1 | 74 | 0.47 | 0.4948 | ||
rainfall | 1 | 74 | 0.13 | 0.7215 | ||
Lucasium stenodactylum Pantily/Wakura | Seral_stage | 3 | 72 | 3.55 | 0.0187 | |
Patch size | 1 | 72 | 0.78 | 0.3794 | ||
Rainfall | 1 | 72 | 4.17 | 0.0448 | ||
Season | 1 | 72 | 0.08 | 0.7748 | ||
Ctenotus pantherinus Liwirungu/Wurrkarn | Seral_stage | 3 | 68 | 0.26 | 0.8567 | |
Location | 3 | 68 | 3.75 | 0.0148 | ||
Year | 4 | 68 | 2.09 | 0.0923 | ||
Diplodactylus laevis Pantily/Wakura | Seral_stage | 3 | 66 | 0.17 | 0.9186 | |
Location | 3 | 66 | 3.63 | 0.0173 | ||
Patch size | 1 | 66 | 0.50 | 0.4830 | ||
Rainfall | 1 | 66 | 8.41 | 0.0051 | ||
Season | 1 | 66 | 0.04 | 0.8486 | ||
Location:season | 3 | 66 | 7.22 | 0.003 | ||
Ctenophorus isolepis Pampirta/Wiji | Location | 3 | 67 | 3.03 | 0.0354 | |
Year | 4 | 67 | 9.31 | <0.0001 | ||
Seral_stage | 3 | 67 | 5.68 | 0.0016 | ||
Season | 1 | 67 | 8.87 | 0.0040 | ||
Lerista bipes Winkujartu ‘lice-eating snake’ | Seral_stage | 3 | 66 | 0.19 | 0.9006 | |
Location | 3 | 66 | 20.14 | <0.0001 | ||
PD_500m | 1 | 66 | 15.58 | 0.0002 | ||
Rainfall | 1 | 66 | 20.42 | <0.0001 | ||
Season | 1 | 66 | 18.64 | 0.0001 | ||
Location:season | 3 | 66 | 8.47 | 0.0001 |
Significant variables in the best models for mammal species richness and daily capture rates. Values present estimated means and 95% confidence intervals.
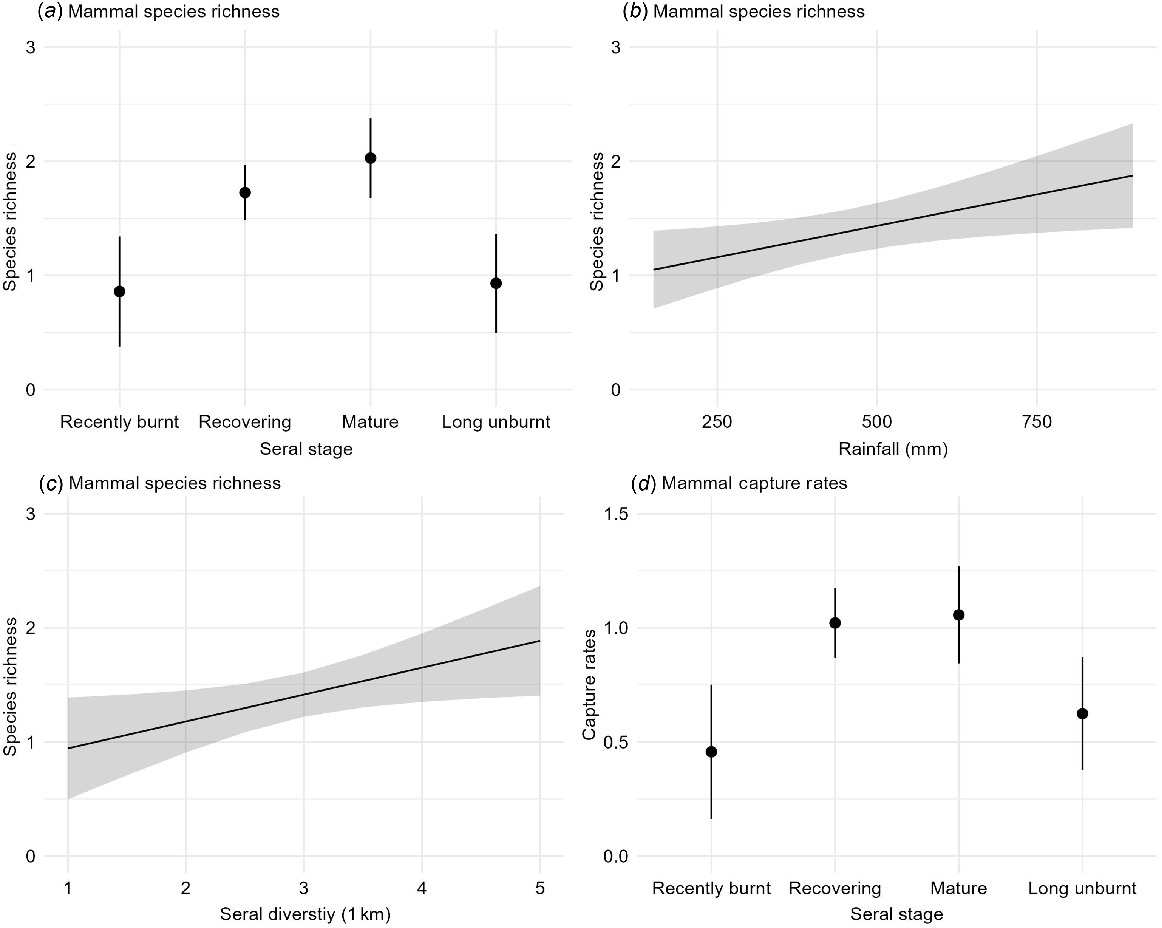
Daily capture rates ranged from 0 to 3.5 mammals/day. The best models for daily capture rate included seral stage, season, and rainfall (Table 2a). Capture rates were significantly higher in Parrawa and Nyirrinyanu (recovering and mature) seral stages (Fig. 4), whereas seasonal and rainfall differences were not significant (Table 3a).
Overall species richness ranged from 5 to 20 across all the site-surveys. The best models contained location, season (and their interaction), rainfall and patch size (Table 2b). Species richness varied significantly among locations and seasons. At Kulgara and Yilpi, the species richness was higher in the early dry than the late dry, whereas at Kuduarra and Kurlku (the two drier locations, Fig. 1), species richness was similar or increased between the early and late dry seasons (Fig. 5). Rainfall and patch size were in the best-ranked models for species richness (Table 2b), but neither were significant (Table 3c).
Significant variables in the best models for reptile species richness and daily capture rates. Figures present estimated means and 95% confidence intervals.
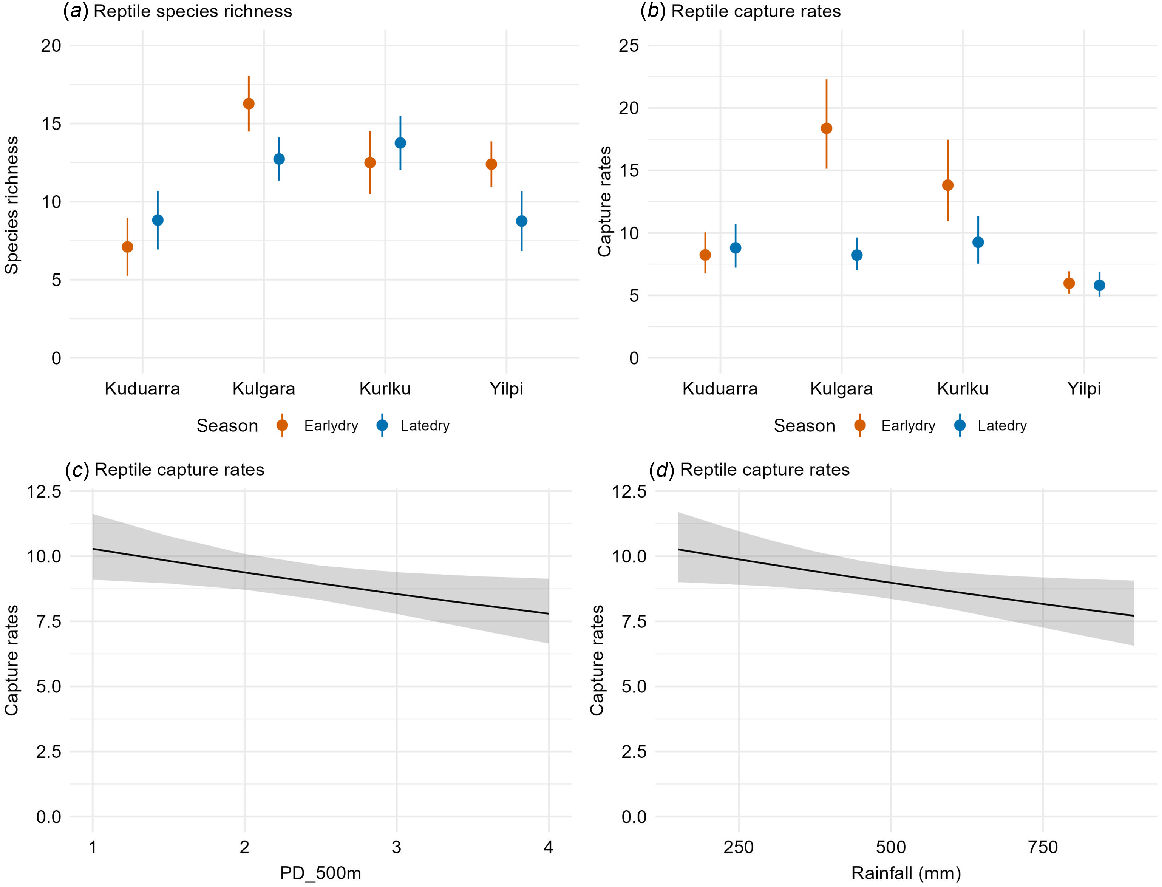
Daily capture rates ranged from 3.6 to 24.5 reptiles/day. In the best model (Table 2b), daily capture rates significantly declined between the early and late dry at Kurlku and Kulgara, but did not change at Yilpi and Kuduarra (Fig. 5). Daily capture rates significantly declined as the seral diversity at the small scale (within 500 m) increased (Table 3b, Fig. 5). Daily capture rates also significantly declined as rainfall decreased, probably driven by patterns in three frequently caught species (see below).
Fire response of individual species
Two mammal species were captured at more than 30% of sites. The model results are shown in Tables 2c and 3c. Pseudomys hermannsburgensis/pilbarensis sp. nov. occurrence had a significant negative relationship with seral diversity at the small scale (500 m). It was also more likely to be caught in the recovering seral stage, and there were interactions between location and season, but although these effects were in the best models (Table 2c), they were not significant (Table 3c). The occurrence of Notomys alexis differed significantly between locations, with the highest probability of occurring at Kurlku. It also increased with seral diversity at the medium scale (1 km), and rainfall, but these effects were not significant (Table 3c, Fig. 6).
Variables in the best models for the probability of occurrence for two mammal species, and in the daily capture rate for five reptile species. Values present estimated means and 95% confidence intervals. Graphs are included only for fixed effects in the best-ranked models. Statistics for fixed effects are in Table 3c.
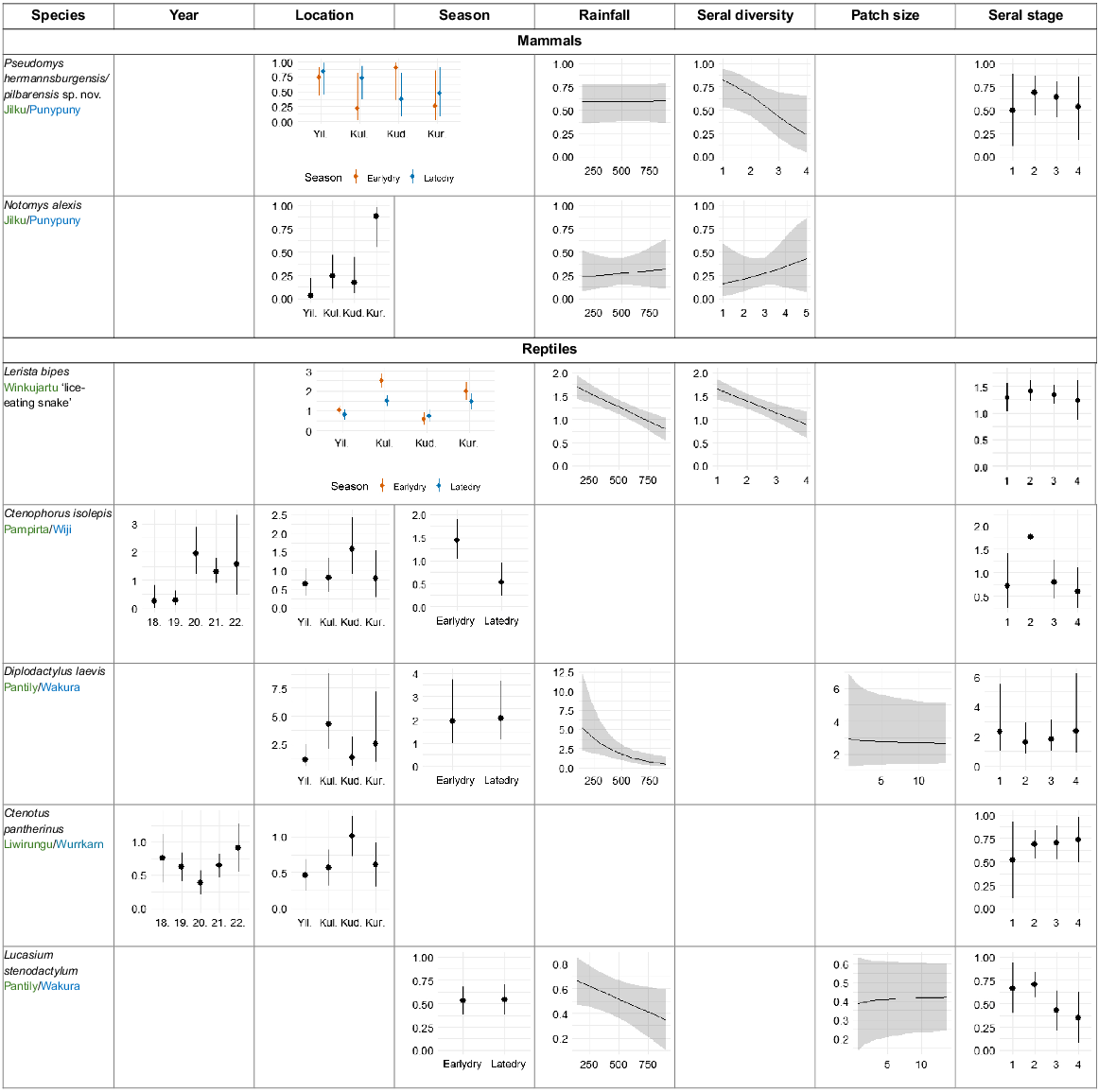
Five reptile species were caught at >50% of the site-surveys. Across this set of species, rainfall had a significant negative effect on captures for three of these species, namely Lerista bipes, Diplodacytlus laevis, and Lucasium stenodactylum (Tables 2c, 3c, Fig. 6), which probably also drove the lower capture rates for reptiles as rainfall increased (reported above). Higher rainfall drives higher vegetation growth, which may disadvantage these three species, because they were most frequently caught in recently burnt and recovering spinifex (see below). Location was important for four species, but for Lerista bipes this depended on season. Season was also a significant factor for Ctenophorus isolepis, whose capture rates were higher in the early dry season. Season was included in the best models for Diplodactylus laevis and Lucasium stenodactylum, but was not significant in both cases. Seral stage was included in top models for all five species, but was a significant predictor for only one species, Ctenophorus isolepis, with capture rates highest in the recovering stage. Seral stage diversity at small scale (500 m) was negatively associated with the capture rates of Lerista bipes (Tables 2c, 3c, Fig. 6).
Seral preferences of species
Notomys alexis was caught disproportionately often in Parrawa, the recovering seral stage. The small Pseudomys hermannsburgensis/pilbarensis sp. nov. was caught less than expected at Wuntara, recently burnt sites, but the larger Pseudomys desertor was caught more than expected in Nyirrinyanu, mature sites. Sminthopsis youngsoni captures were spread among seral stages as expected, but when captures of all Sminthopsis spp. were considered, there were more than expected in Nyirrinyanu and fewer in Wuntara (Fig. 7; full results in Table S1a).
The distribution of captures of (a) reptile and (b) mammal species across seral stages. In cases where the captures of species in a genus were low, and those species have similar ecologies, we grouped the captures in the genus to increase the sample size for analysis. Pseudomys h/p refers to Pseudomys hermannsburgensis/pilbarensis sp. nov.; Delma spp. refers to D. borea, D. butleri, D. desmosa, D. nasuta, D. tincta. Proportions of the overall sample of 81 site-surveys that belong to each of the four seral stages are shown by the vertical grey lines (0.12, 0.38, 0.24, 0.16 for recently burnt, recovering, mature and long-unburnt respectively). Total captures for each species are in square brackets after the species name. Species whose captures deviated significantly from expected using heterogeneity tests are marked as *** (P < 0.0001), ** (P < 0.01), * (P < 0.05), + (P < 0.1). The figure includes only those species with sufficient captures for statistical analysis; the full list of species caught, their captures, and the results of the heterogeneity tests, are in Table S1.
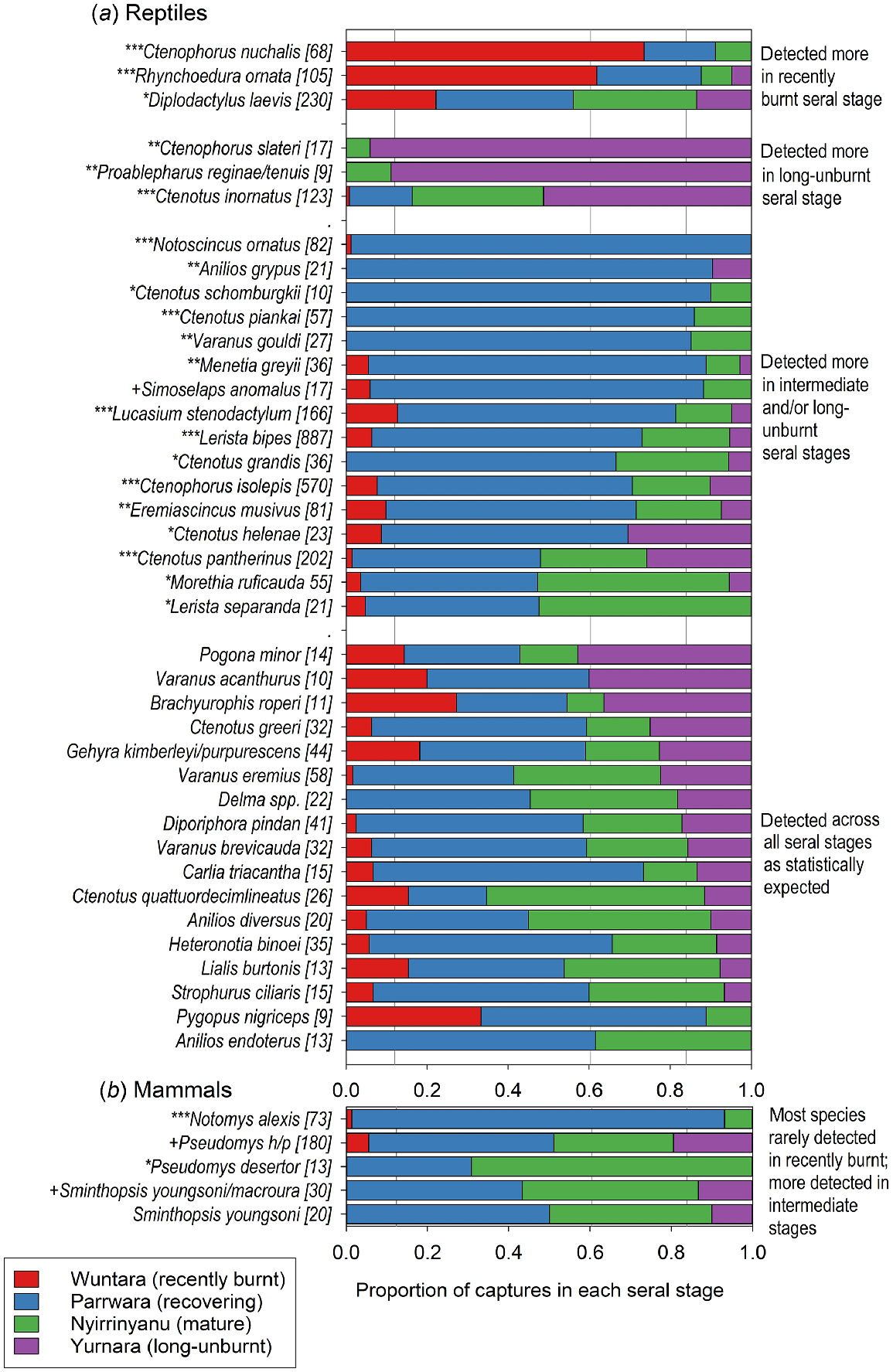
Three reptile species (Ctenotus nuchalis, Diplodactylus laevis, Rhynchoedura ornata) were caught in Wuntara, recently burnt sites, significantly more than expected. Four species (Ctenophorus slateri, Proablephurus reginae, Proablephurus tenuis, Ctenotus inornatus) were caught in Yurnara, long-unburnt sites, significantly more than expected. Sixteen reptile species were caught significantly more often than expected in the intermediate and older seral stages, especially Parrawa, recovering, but also in Nyirrinyanu, mature, or Nyirrinyanu and Yurnara, mature and long-unburnt. A further 21 species showed no statistical preference for seral stages; some had a high number of captures, but some had low overall captures, and a greater sample may reveal seral preferences (Fig. 7; full results in Table S1b).
We also considered the distribution of captures of rare species (i.e. caught <6 times) across the seral stages. For reptiles, captures in the four seral stages were 8, 28, 19, and 14, which was not significantly different from expected (9, 33, 16, 11) (, P = 0.27). For mammals, captures were 1, 5, 13, and 2, which was tending to differ from expected (3, 10, 5, 3) by skewing to the Nyirrinyanu, mature seral stage (, P = 0.093).
Discussion
The importance of traditional fire practices for biodiversity, cultural and social benefits is beginning to be recognised globally (Hoffman et al. 2021). Our work contributes to this awareness, and provides important ecological information from a little-studied region of Australia’s deserts (McKenzie 1981). Desert fire management by Indigenous rangers focusses on creating key attributes of right-way fire, namely, burning many small fires that contribute towards increasing the seral diversity, and the extent of mature and long-unburnt vegetation (IDA 2023; Ruscalleda-Alvarez et al. 2023). Our study aimed to understand the benefits of a right-way fire regime for small, ground-dwelling Kuwi, reptiles and mammals, including what size of fire and scale of seral diversity the ranger teams should aim for, and how important it is to protect long-unburnt vegetation. Our results suggest that these reptile and mammal communities respond differently to fire and have different patterns of post-fire recovery. Mammal species richness and capture rates were lowest after fire, recovered as the vegetation recovered, and species richness was positively associated with seral diversity. In contrast, the overall species richness and abundance of reptiles was little-affected by fire or the seral stage, but this result masked strong associations between many individual species and certain seral stages, including mature and long-unburnt vegetation. Surprisingly, given the strong evidence for species succession with post-fire vegetation recovery, reptile species richness and capture rates were either unrelated, or were negatively related, to increased seral diversity. Reptile responses were also influenced by location, season, and rainfall, whereas mammals responded only to rainfall. Below, we discuss some of the key findings, including significant species detections, and responses to seral stage and size, seral diversity, and long-unburnt vegetation. We finish by discussing what our results mean for management.
Significant species detections
Our surveys showed some significant records. We detected Larnkurr/Jumpiyinti, the brushtail possum, Trichosurus vulpecula, at Yilpi, on the edge of the Edgar Ranges on Karajarri Country. Larnkurr was previously detected, in hair tufts in dingo scats, in this area in the 1970s during surveys by Western Australian Government (McKenzie 1981). We detected Larnkurr on a camera trap image. Yilpi lies on the southern edge of the likely distribution of the northern subspecies, T. v. arnhemensis (northern brushtail possum), but records of Larnkurr from the northwest deserts are very few, and more clarity on the distributions of this subspecies plus those of the two other subspecies that could occur here (T. v. vulpecula, and T. v. hypoleucas) is needed (Woinarski et al. 2014; TSSC 2021). Brushtail possums have declined in many areas, and the northern subspecies is listed as nationally Vulnerable, with declines attributed to changed fire regimes, and predation by cats (and foxes, at the southern edge of their range) (Woinarski et al. 2014; TSSC 2021).
We also detected kaluta, Dasykaluta rosamondae, at Kuduarra (on the Canning Stock Route) on Ngurrara Country. We caught this species four times. The kaluta has previously been recorded in the Pilbara region, only reaching the south-western edge of the Great Sandy Desert bioregion, so our observations extend the distribution known to scientists well into the Great Sandy Desert, by about 500 km. Similarly, we made many detections of the Dampierland plain slider, Lerista separanda, across all four locations on Karajarri and Ngurrara, with Kuduarra being about 450 km away from existing records. The distribution of this species has been described by scientists as centred on the Dampierland bioregion (Chapple et al. 2019), but our records, and those of Doughty et al. (2011) from the Pilbara, suggest that the species may have a larger, desert-centred distribution that extends north into the Dampierland bioregion, and could mean that it no longer qualifies as a Priority 2-listed species in Western Australia (https://bio.wa.gov.au/guide/conservation-status-definitions#p2). The Karajarri name for Lerista. spp is Winkujarta, lice-eating snake, because people used these legless skinks to clean lice out of their hair.
Seral stage and size
Mammal richness and capture rates declined immediately after fire, and increased over the next two seral stages, in a very similar pattern as the recovery of the spinifex layer itself (Fig. 2). Fire removes food resources (plant material and arthropods) for most mammals, and, by removing cover, exposes them to increased predation risk, including from introduced predators (McGregor et al. 2015; Leahy et al. 2016). The pattern in overall capture rates was driven by the three most-captured species, Pseudomys hermannsburgensis/pilbarensis sp. nov and Notomys alexis. These species have been reported to prefer recovering spinifex, 2–5 years after fire (Letnic and Dickman 2005; Bennison et al. 2018). However, less common mammal species seem to follow the same pattern, of being unlikely to occur in recently burnt habitats, and more likely to occur in older seral stages. Pseudomys desertor had the strongest preference for mature and long-unburnt seral stages, consistent with previous studies that have shown it to be more common in long-unburnt vegetation (Masters 1993; Letnic and Dickman 2005).
We found that 23 reptile species were mostly caught in certain seral stages. This was over half of the species with enough captures to test for seral preferences. This seral succession is likely to occur because some reptile species have preferences for microhabitat structure and floristics that are optimised at different stages of seral recovery (Masters 1996; Pianka 1996; Letnic et al. 2004; Pianka and Goodyear 2012). For example, shuttling heliotherm skinks need cover to protect them from the heat of the sun, and probably also from diurnal predators such as Kirrkirr/Jarnun (hawk, kite) (Pianka 1996); they are rarely present in recently burnt areas but re-establish as spinifex regrows (Letnic et al. 2004; Daly et al. 2008; Gordon et al. 2010). The clear sign of succession in our study is interesting, because fire intervals in the northern and western part of the Karajarri and Ngurrara Native Title areas can be as short as 4 or 5 years. Signs of succession are weaker in the tropical savannas, potentially because the short fire intervals of 1–4 years leave little time for populations to establish as seral stages reach the preferred stage (Trainor and Woinarski 1994; Valentine and Schwarzkopf 2009). The strong successional patterns in our study despite rather short fire intervals suggest that most species are present across most areas, sometimes at undetectable levels of abundance, and populations can increase rapidly through in situ reproduction and/or emigration.
The seral preferences we observed have been reported previously (with minor variations) for Ctenophorus nuchalis and Rhynchoedura ornata (recently burnt); Ctenophorus isolepis, Ctenotus helenae, Ctenotus grandis, Ctenotus pantherinus, Ctenotus piankai, Ctenotus schomburgkii, Lerista bipes, Notoscincus ornatus, and Menetia greyii (recovering and/or mature seral stages) (Masters 1996; Letnic et al. 2004; Pianka and Goodyear 2012; Smith et al. 2013). The seral preferences we observed for several more species have not previously been reported (to our knowledge), but make sense given aspects of their ecology; Proablepharus reginae, P. tenuis and Morethia ruficauda are litter-dwellers. Leaf litter takes several years to redevelop after fire (Fig. 2), and we mostly caught these species in long-unburnt vegetation. Ctenotus inornatus, like many diurnal, shuttling heliotherm skinks, probably needs spinifex for shade and protection from predators including varanids, snakes, and cats (Greer 2024). This species was caught most often in long-unburnt vegetation. Ctenophorus slateri is usually found on small rocky outcrops (Greer 2024) that offer protection from fire, suggesting a degree of fire sensitivity, and we caught this species mostly in long-unburnt sites. Diplodactlyus laevis is nocturnal, shelters in spider burrows, and is usually found in open habitats (Chapple et al. 2019; Greer 2024), and we found it preferred recently burnt areas. Simoselaps anomalus is a nocturnal sand-swimming snake that preys on small reptiles, especially Lerista spp. (Greer 2024), so its seral preference may follow that of its prey; both S. anomalus and Lerista bipes were mostly caught in recovering spinifex.
Although seral stage was important (in different ways) for both mammals and reptiles, we did not find strong evidence that the size of the seral patch was important. Patch size crept into one of the best-ranked models for reptile species richness, and for the capture rates of Diplodactylus laevis and Lucasium stenodactylum, but in all cases was not significant. One of the few desert studies that has looked at effects of patch size also found it to be uninfluential (Doherty et al. 2024). In contrast, mammal abundance and richness were higher in larger unburned areas of the Mojave Desert (Hulton VanTassel et al. 2015), and given the importance of scale in so many aspects of ecology, our result is surprising, and warrants further investigation (Mason and Lashley 2021).
Seral diversity
Given the seral preferences shown by many reptile species, it follows that areas containing mixed seral stages should contain a greater variety of species assemblages, as has been shown for desert plants, which also show post-fire successional patterns (Wright and Clarke 2007; Greenwood et al. 2024). In addition, sites (such as our trapping sites) positioned within areas with more surrounding seral diversity could support higher species richness, for four reasons. First, the processes of colonisation into patches as they reach preferred stages should be faster with a greater proximity of source populations. Second, there could be higher rates of individuals ‘leaking’ into neighbouring, non-preferred seral stages. Third, some species are edge specialists; for example, the Yalki/Wilka (sand goanna, Varanus gouldii) has been shown to exploit the edges between seral stages (Bliege Bird et al. 2013). Fourth, seral diversity should increase the breadth of accessible resources within an animal’s home range (e.g. annual grass seed in recently burnt areas adjacent to cover in mature and long-unburnt areas). Examples of species that may benefit from such fine-scale seral diversity include Jitarru/Mirtuluju (bilby, Macrotis lagotis) (Southgate et al. 2007); Kuriti/Marrany (dingo, Canis familiaris dingo) (Bliege Bird et al. 2018); and Pikkarta/Jamarnti (euro, Osphranter robustus) (Codding et al. 2014).
However, our results on the relationships between seral diversity and biodiversity were mixed. We found no sign that seral diversity around a site, at small (500 m), medium (1 km) or large (5 km) scales, resulted in increased species richness or reptile captures at that site, and reptile capture rates decreased with seral diversity at the small scale. In contrast, seral diversity at the medium scale was positively associated with species richness in mammals. The difference between reptiles and mammals may be because mammals move further than most reptile species and are therefore more likely to cross seral boundaries (Dickman et al. 1995; Pianka 1996; Letnic 2001; Haythornthwaite and Dickman 2006). In addition, mammals may be more susceptible to threats, such as predation, that are themselves affected by seral diversity. For example, cats can be attracted to large, severe fires (that cause a single, extensive, early seral stage) while ignoring small, mild fires that result in a greater seral diversity (McGregor et al. 2014; Doherty et al. 2022). In addition, dingoes and goannas may increase activity in areas with a greater seral diversity. High dingo activity may cause a reduction in cat activity (Bliege Bird et al. 2013, 2018), and thus reduced predation pressure on small mammals because dingoes eat fewer small mammals than cats (Murphy et al. 2019).
Alternatively, areas with a high seral diversity may benefit biodiversity because they limit the spread, or affect the behaviour, of wildfire (Letnic and Dickman 2005; Pastro et al. 2011; Bliege Bird et al. 2012; D’Souza et al. 2013; Greenwood et al. 2022). Extensive, severe desert wildfires have been shown to cause reductions in species richness and abundance, most commonly in mammals (Letnic et al. 2004; Pastro et al. 2011; McDonald et al. 2016; Moore et al. 2024), but also in some reptiles, such as Wurrkarn/Minijarti, the great desert skink (Liopholis kintorei), a culturally significant and nationally threatened species (Cadenhead et al. 2016; Indigenous Desert Alliance 2023). Key resources for ground-dwelling mammals and reptiles, such as woody debris, seeds, and invertebrates, are reduced or altered by fire (Wright and Clarke 2007; Pianka and Goodyear 2012). Species recovery after extensive, intense fires is slower across many animal groups including invertebrates (Langlands et al. 2012; Bastow 2020) and vertebrates (Shaw et al. 2021; Legge et al. 2022) potentially from depleted resources, but more likely from increased exposure to predation (Leahy et al. 2016; Moore et al. 2018; Doherty et al. 2022).
Mature and long-unburnt vegetation
Another feature of areas with high seral diversity is that they may contain and protect (by limiting or interacting with wildfire spread) areas of mature and long-unburnt habitat, which is important for a subset of species. To some extent, topographical features such as paleodrainages and rocky areas can create fire shadows that protect long-unburnt vegetation, but such areas are limited in the Great Sandy Desert, which is characterised by large unbroken expanses of sandplains and dunes. We found that up to eight reptile species were mostly, or almost only, found in Nyirrinyanu, mature vegetation, and Yurnara, long-unburnt vegetation, and several more species appeared to prefer these later seral stages (Fig. 6), but their overall captures were too low to be sure. All mammals except for Notomys alexis were more common in Nyirrinyanu, mature vegetation, and Yurnara, long-unburnt vegetation. Notomys alexis is able to evade predators even when ground cover is sparse with its fast hopping speed, and by sheltering in burrows (Spencer et al. 2014). However, even N. alexis was rarely caught in Wuntara, recently burnt vegetation.
The importance of long-unburnt vegetation for maintaining species assemblages in deserts recurs through an accumulating number of studies (Kelly et al. 2012; Langlands et al. 2012; Taylor et al. 2012; Nimmo et al. 2013; Farnsworth et al. 2014). Mature and long-unburnt vegetation appears essential for the persistence of some species, for example, Pseudomys desertor, Dasycercus spp., Sminthopsis psammophila (Masters 1993; Letnic and Dickman 2005; Riley et al. 2021), great desert skinks (Moore et al. 2015), and yulu, Acanthophis pyrrhus (McDonald et al. 2012).
Management implications
Our results help guide fire-management planning, not just for Karajarri and Ngurrara, but also other desert ranger groups. Australian deserts contain the largest network of Indigenous-managed land globally (O’Bryan et al. 2021). Our work can also guide other desert land managers including the Western Australian Department of Biodiversity, Conservation and Attractions. Our study encourages us to create a mix of seral stages across Country, so that there is good habitat for a range of species. We should increase the area of Nyirrinyanu and Yurnara, mature and long-unburnt spinifex, because some species depend on this seral stage, yet it burns readily in wildfires. The average fire-return intervals across Karajarri and Ngurrara between 2000 and 2023 were 5.2 years and 7.1 years respectively (Fig. S1), but now we know that some species do best in spinifex that is at least 5 years old (5 years since fire). Our old people achieved older post-fire vegetation by creating a fine-scale patchwork of seral stages that tends to limit wildfire spread. We can aim to emulate this. The spatial scale of the patchwork may be less important for reptile species, but having multiple seral stages within a 1–5 km radius area (i.e. 6.3–31.4 km2) could benefit mammals. Since we began landscape-scale, coordinated fire management in 2019, we have reduced the mean size of fires by 52% (down to 2510 ha) and 40% (down to 21.6 km2) on Karajarri and Ngurrara respectively (Karajari Rangers, Ngurrara Rangers, unpubl. data). We can aim to drive those numbers lower.
Two-way collaboration
The Pirra Pirra Jungku/Pirra Warlu project has been a successful two-way collaboration between Traditional Custodians and scientists. The key feature underpinning this success has been the leadership of the Karajarri Rangers, and later also the Ngurrara Rangers. The rangers integrated the scientific method into their management, while maintaining authority over the project direction and conduct. The project aims, methods and results were discussed continuously by the Indigenous and non-Indigenous project partners, and co-presented to steering committees and Prescribed Body Corporates for ongoing endorsement. The rangers sought their own funding for the project, held all the required permits, and own the data. They have communicated the project at ranger meetings, scientific conferences and to government funders; hosted ranger exchanges so that trapping techniques could be learned peer-to-peer; and schooled the science partners in cultural dimensions of fire practice and looking after Country. The fire-history information and analyses have supported collaborative fire-management planning with neighbouring groups, pastoral stations, communities and elders, to discuss priorities, share resources, trouble-shoot issues such as weather events, access constraints and other pertinent operational steps. Overall, the project has built confidence in the ranger teams to lead and deliver large, long-term projects. It has also encouraged evolution in reporting, to include biodiversity outcomes of management as well as the more usual ‘input’ reporting metrics such as ranger time. As a result of its appeal, the Pirra Jungku/Pirra Warlu model is expanding to include other neighbouring desert ranger groups, including the Nyangumarta and Ngururrpa teams.
Data availability
Data are held by the Karajarri Rangers (Karajarri Traditional Lands Trust) and the Ngurrara Rangers (Yanunijarra Aboriginal Corporation).
Conflicts of interest
One of the authors (Sarah Legge) is an editor-in-chief with Wildlife Research and another (Hannah Cliff) was a guest editor for the special issue that includes this paper. To mitigate these potential conflicts of interest they were blinded from the review process.
Declaration of funding
This work was funded by the Australian Government’s Indigenous Protected Area Program, Indigenous Ranger Program, and the National Environmental Science Program via the Threatened Species Recovery Hub; by the Western Australia State Natural Resource Management, Aboriginal Ranger Program, Lotteries West, and via inkind support from the Department of Biodiversity, Conservation and Attractions; by the Indigenous Desert Alliance/10 Deserts; and by the Australian Research Council (LP220200184).
Acknowledgements
We thank the Karajarri and Ngurrara Traditional Owners, and respectfully acknowledge their past and present elders, their custodianship of Country, and their support for this project. Many rangers, elders and coordinators have participated in these surveys, we thank them for their hard work and commitment. Led by Bayo Taylor and Jacko Shoveller, the Karajarri rangers included Eugene ‘EB’ Bumba, Frederick ‘Gulu’ Shovellor, Julian Nagomara, Kamahl Bangu, Marissa Munro, Paddy ‘Moonie’ Kittimbolt, Shannica ‘Shani Bod’ Boddington, Sheen Kitty, and Vanessa ‘Beno’ Kitty. Karajarri wish to especially mention Jess Bangu, a cultural leader and mentor for the ranger team and community, who passed away last year. Led by Chantelle Murray and Frankie McCarthy, the Ngurrara Rangers included Alexander Grace, Alfie Thirkall, Barnsey, Bevan Bent, Daren Smith, Darlene Kogolo, Edmond Smiler, Elton ‘Toy’ Smiler, Francis Thirkall, Isiah Jack, Jamin Bent, Justin Andrews, Lucas Brown, Lynette Rogers, Raylene ‘Emily’ Lenmardi, Regina Thirkall, Resheeda Thirkall, Stanley Thirkall, Sumayah Suprise, Thomas Nnarda, and Tracy Munda. Staff, interns and volunteers from Environs Kimberley, WA DBCA, the NESP Threatened Species Recovery Hub, the Indigenous Desert Alliance and the Kimberley Land Council gave time and expertise, and supported the project in diverse ways from refining fire mapping to digging pitfall traps to helping with communication products. Special shout out for Ed Blackwood, Annika Spiridis, Nico Rakotopare, and Jaana Dielenberg.
References
Bastow J (2020) The impacts of a wildfire in a semiarid grassland on soil nematode abundances over 4 years. Biology and Fertility of Soils 56, 675-685.
| Crossref | Google Scholar |
Bennison K, Godfree R, Dickman CR (2018) Synchronous boom–bust cycles in central Australian rodents and marsupials in response to rainfall and fire. Journal of Mammalogy 99, 1137-1148.
| Crossref | Google Scholar |
Bird DW, Bird RB, Parker CH (2005) Aboriginal burning regimes and hunting strategies in Australia’s Western Desert. Human Ecology 33, 443-464.
| Crossref | Google Scholar |
Blackwood EMJ, Karajarri Rangers, Bayley S, Bijlani H, Fensham RJ, Lindsay M, Noakes E, Wemyss J, Legge S (2022) Pirra Jungku: comparison of traditional and contemporary fire practices on Karajarri Country, Western Australia. Ecological Management & Restoration 23, 83-92.
| Crossref | Google Scholar |
Bliege Bird R, Codding BF, Kauhanen PG, Bird DW (2012) Aboriginal hunting buffers climate-driven fire-size variability in Australia’s spinifex grasslands. Proceedings of the National Academy of Sciences 109, 10287-10292.
| Crossref | Google Scholar |
Bliege Bird R, Tayor N, Codding BF, Bird DW (2013) Niche construction and Dreaming logic: aboriginal patch mosaic burning and varanid lizards (Varanus gouldii) in Australia. Proceedings of the Royal Society B: Biological Sciences 280, 20132297.
| Crossref | Google Scholar |
Bliege Bird R, Bird DW, Codding BF (2016) People, El Niño southern oscillation and fire in Australia: fire regimes and climate controls in hummock grasslands. Philosophical Transactions of the Royal Society B: Biological Sciences 371, 20150343.
| Crossref | Google Scholar |
Bliege Bird R, Bird DW, Fernandez LE, Taylor N, Taylor W, Nimmo D (2018) Aboriginal burning promotes fine-scale pyrodiversity and native predators in Australia’s Western Desert. Biological Conservation 219, 110-118.
| Crossref | Google Scholar |
Bowman DMJS (1998) The impact of Aboriginal landscape burning on the Australian biota. New Phytologist 140, 385-410.
| Crossref | Google Scholar | PubMed |
Bradstock RA (2010) A biogeographic model of fire regimes in Australia: current and future implications. Global Ecology and Biogeography 19, 145-158.
| Crossref | Google Scholar |
Burbidge AA, McKenzie NL (1989) Patterns in the modern decline of Western Australia’s vertebrate fauna: causes and conservation implications. Biological Conservation 50, 143-198.
| Crossref | Google Scholar |
Burrows ND, Christensen P (1991) A survey of Aboriginal fire patterns in the Western Desert of Australia. In ‘Fire and the environment: ecological and cultural perspectives. Proceedings of an International Symposium, Knoxville, Tennessee, 20–24 March 1990. General Technical Report SE69’. (Eds SC Nodvin, TA Waldrop) pp. 297–310. (United States Department of Agriculture: Ashville, NC, USA)
Burrows ND, Burbidge AA, Fuller PJ, Behn G (2006) Evidence of altered fire regimes in the Western Desert region of Australia. Conservation Science Western Australia 5, 272-284.
| Google Scholar |
Cadenhead NCR, Kearney MR, Moore D, McAlpin S, Wintle BA (2016) Climate and fire scenario uncertainty dominate the evaluation of options for conserving the great desert skink. Conservation Letters 9, 181-190.
| Crossref | Google Scholar |
Cameron L (2020) ‘Healthy Country, Healthy People’: Aboriginal Embodied Knowledge Systems in Human/Nature Interrelationships. The International Journal of Ecopsychology (IJE) 1, 3.
| Google Scholar |
Codding BF, Bliege Bird R, Kauhanen PG, Bird DW (2014) Conservation or co-evolution? Intermediate levels of aboriginal burning and hunting have positive effects on kangaroo populations in Western Australia. Human Ecology 42, 659-669.
| Crossref | Google Scholar |
Daly BG, Dickman CR, Crowther MS (2008) Causes of habitat divergence in two species of agamid lizards in arid central Australia. Ecology 89, 65-76.
| Crossref | Google Scholar | PubMed |
Dickman CR, Predavec M, Downey FJ (1995) Long-range movements of small mammals in arid Australia: implications for land management. Journal of Arid Environments 31, 441-452.
| Crossref | Google Scholar |
Doherty TS, Geary WL, Jolly CJ, Macdonald KJ, Miritis V, Watchorn DJ, Cherry MJ, Conner LM, González TM, Legge SM, Ritchie EG, Stawski C, Dickman CR (2022) Fire as a driver and mediator of predator–prey interactions. Biological Reviews 97, 1539-1558.
| Crossref | Google Scholar | PubMed |
Doherty TS, Bohórquez Fandiño DF, Watchorn DJ, Legge SM, Dickman CR (2024) Experimentally testing animal responses to prescribed fire size and severity. Conservation Biology 38, e14231.
| Crossref | Google Scholar | PubMed |
Doughty P, Rolfe JK, Burbidge AH, Pearson DJ, Kendrick PG (2011) Herpetological assemblages of the Pilbara biogeographic region, Western Australia: ecological associations, biogeographic patterns and conservation. Records of the Western Australian Museum, Supplement 78, 315-341.
| Crossref | Google Scholar |
D’Souza JB, Whittington A, Dickman CR, Leung LK-P (2013) Perfect storm: demographic responses of an irruptive desert mammal to prescribed burns following flooding rain. Austral Ecology 38, 765-776.
| Crossref | Google Scholar |
Edwards GP, Allan GE, Brock C, Duguid A, Gabrys K, Vaarzon-Morel P (2008) Fire and its management in central Australia. The Rangeland Journal 30, 109-121.
| Crossref | Google Scholar |
Farnsworth LM, Nimmo DG, Kelly LT, Bennett AF, Clarke MF (2014) Does pyrodiversity beget alpha, beta or gamma diversity? A case study using reptiles from semi-arid Australia. Diversity and Distributions 20, 663-673.
| Crossref | Google Scholar |
Gordon CE, Dickman CR, Thompson MB (2010) Partitioning of temporal activity among desert lizards in relation to prey availability and temperature. Austral Ecology 35, 41-52.
| Crossref | Google Scholar |
Gould RA (1971) Uses and effects of fire among the Western Desert Aborigines of Australia. Mankind 8, 14-24.
| Crossref | Google Scholar |
Greenwood L, Bliege Bird R, Nimmo D (2022) Indigenous burning shapes the structure of visible and invisible fire mosaics. Landscape Ecology 37, 811-827.
| Crossref | Google Scholar |
Greenwood L, Bliege Bird R, McGuire C, Jadai N, Price J, Skroblin A, van Leeuwen S, Nimmo D (2024) Indigenous pyrodiversity promotes plant diversity. Biological Conservation 291, 110479.
| Crossref | Google Scholar |
Greer AE (2024) Encyclopedia of Australian Reptiles. Version: 1 June 2024. Available at https://www.encyclopediaofaustralianreptiles.com/
Haythornthwaite AS, Dickman CR (2006) Long-distance movements by a small carnivorous marsupial: how Sminthopsis youngsoni (Marsupialia: Dasyuridae) uses habitat in an Australian sandridge desert. Journal of Zoology 270, 543-549.
| Crossref | Google Scholar |
Hoffman KM, Davis EL, Wickham SB, Schang K, Johnson A, Larking T, Lauriault PN, Quynh Le N, Swerdfager E, Trant AJ (2021) Conservation of Earth’s biodiversity is embedded in Indigenous fire stewardship. Proceedings of the National Academy of Sciences 118, e2105073118.
| Crossref | Google Scholar |
Hulton VanTassel HL, Barrows CW, Anderson KE (2015) Post-fire spatial heterogeneity alters ground-dwelling arthropod and small mammal community patterns in a desert landscape experiencing a novel disturbance regime. Biological Conservation 182, 117-125.
| Crossref | Google Scholar |
Indigenous Desert Alliance (2023) Looking after Tjakura, Tjalapa, Mulyamiji, Warrarna, Nampu. A national recovery plan for the Great Desert Skink (Liopholis kintorei). Department of Climate Change, Energy, the Environment and Water, Canberra, ACT, Australia. Available at www.dcceew.gov.au/environment/biodiversity/threatened/recovery-plans
Kelly LT, Nimmo DG, Spence-Bailey LM, Taylor RS, Watson SJ, Clarke MF, Bennett AF (2012) Managing fire mosaics for small mammal conservation: a landscape perspective. Journal of Applied Ecology 49, 412-421.
| Crossref | Google Scholar |
Kimber R (1983) Black lightning: Aborigines and fire in central Australia and the Western Desert. Archaeology in Oceania 18, 38-45.
| Crossref | Google Scholar |
Langlands PR, Brennan KEC, Ward B (2012) Is the reassembly of an arid spider assemblage following fire deterministic? Austral Ecology 37, 429-439.
| Crossref | Google Scholar |
Leahy L, Legge SM, Tuft K, McGregor HW, Barmuta LA, Jones ME, Johnson CN (2016) Amplified predation after fire suppresses rodent populations in Australia’s tropical savannas. Wildlife Research 42, 705-716.
| Crossref | Google Scholar |
Legge S, Rumpff L, Woinarski JCZ, Whiterod NS, Ward M, Southwell DG, Scheele BC, Nimmo DG, Lintermans M, Geyle HM, Garnett ST, Hayward-Brown B, Ensbey M, Ehmke G, Ahyong ST, Blackmore CJ, Bower DS, Brizuela-Torres D, Burbidge AH, Burns PA, Butler G, Catullo R, Chapple DG, Dickman CR, Doyle KE, Ferris J, Fisher D, Gallagher R, Gillespie GR, Greenlees MJ, Hohnen R, Hoskin CJ, Hunter D, Jolly C, Kennard M, King A, Kuchinke D, Law B, Lawler I, Lawler S, Loyn R, Lunney D, Lyon J, MacHunter J, Mahony M, Mahony S, McCormack RB, Melville J, Menkhorst P, Michael D, Mitchell N, Mulder E, Newell D, Pearce L, Raadik TA, Rowley JJL, Sitters H, Spencer R, Valavi R, West M, Wilkinson DP, Zukowski S (2022) The conservation impacts of ecological disturbance: time-bound estimates of population loss and recovery for fauna affected by the 2019–2020 Australian megafires. Global Ecology and Biogeography 31, 2085-2104.
| Crossref | Google Scholar |
Letnic M (2001) Long distance movements and the use of fire mosaics by small mammals in the Simpson Desert, central Australia. Australian Mammalogy 23, 125-134.
| Crossref | Google Scholar |
Letnic M, Dickman CR (2005) The responses of small mammals to patches regenerating after fire and rainfall in the Simpson Desert, central Australia. Austral Ecology 30, 24-39.
| Crossref | Google Scholar |
Letnic M, Dickman CR, Tischler MK, Tamayo B, Beh C-L (2004) The responses of small mammals and lizards to post-fire succession and rainfall in arid Australia. Journal of Arid Environments 59, 85-114.
| Crossref | Google Scholar |
Lundie-Jenkins G (1993) Ecology of the rufous hare-wallaby, Lagorchestes hirsutus Gould (Marsupialia: Macropodidae) in the Tanami Desert, Northern Territory. I Patterns of habitat use. Wildlife Research 20, 457-475.
| Crossref | Google Scholar |
Martin HA (2006) Cenozoic climatic change and the development of the arid vegetation in Australia. Journal of Arid Environments 66, 533-563.
| Crossref | Google Scholar |
Mason DS, Lashley MA (2021) Spatial scale in prescribed fire regimes: an understudied aspect in conservation with examples from the southeastern United States. Fire Ecology 17, 3.
| Crossref | Google Scholar |
Masters P (1993) The effects of fire-driven succession and rainfall on small mammals in spinifex grassland at Uluru National Park, Northern Territory. Wildlife Research 20, 803-813.
| Crossref | Google Scholar |
Masters P (1996) The effects of fire-driven succession on reptiles in spinifex grasslnads at Uluru National Park, Northern Territory. Wildlife Research 23, 39-47.
| Crossref | Google Scholar |
McDonald PJ, Luck GW, Pavey CR, Wassens S (2012) Importance of fire in influencing the occurrence of snakes in an upland region of arid Australia. Austral Ecology 37, 855-864.
| Crossref | Google Scholar |
McDonald PJ, Stewart A, Schubert AT, Nano CEM, Dickman CR, Luck GW (2016) Fire and grass cover influence occupancy patterns of rare rodents and feral cats in a mountain refuge: implications for management. Wildlife Research 43, 121-129.
| Crossref | Google Scholar |
McGregor HW, Legge S, Jones ME, Johnson CN (2014) Landscape management of fire and grazing regimes alters the fine-scale habitat utilisation by feral cats. PLoS ONE 9, e109097.
| Crossref | Google Scholar | PubMed |
McGregor H, Legge S, Jones ME, Johnson CN (2015) Feral cats are better killers in open habitats, revealed by animal-borne video. PLoS ONE 10, e0133915.
| Crossref | Google Scholar | PubMed |
McKenzie NL, Burbidge AA, Baynes A, Brereton RN, Dickman CR, Gordon G, Gibson LA, Menkhorst PW, Robinson AC, Williams MR, Woinarski JCZ (2007) Analysis of factors implicated in the recent decline of Australia’s mammal fauna. Journal of Biogeography 34, 597-611.
| Crossref | Google Scholar |
Moore D, Kearney MR, Paltridge R, McAlpin S, Stow A (2015) Is fire a threatening process for Liopholis kintorei, a nationally listed threatened skink? Wildlife Research 42, 207-216.
| Crossref | Google Scholar |
Moore D, Kearney MR, Paltridge R, McAlpin S, Stow A (2018) Feeling the pressure at home: predator activity at the burrow entrance of an endangered arid-zone skink. Austral Ecology 43, 102-109.
| Crossref | Google Scholar |
Moore H, Yawuru Country Managers, Bardi Jardi Oorany Ranger, Nyul Nyul Rangers, Nyikina Mangala Rangers, Gibson L, Dziminski M, Radford IJ, Corey B, Bettink K, Carpenter F, Mcphail R, Sonneman T, Greatwich B (2024) Where there’s smoke there’s cats: long unburnt habitat is crucial to mitigating the impacts of cats on the Ngarlgumirdi, greater bilby (Macrotis lagotis). Wildlife Research 51(5),.
| Crossref | Google Scholar |
Morton SR, Stafford Smith DM, Dickman CR, Dunkerley DL, Friedel MH, McAllister RRJ, Reid JRW, Roshier DA, Smith MA, Walsh FJ, Wardle GM, Watson IW, Westoby M (2011) A fresh framework for the ecology of arid Australia. Journal of Arid Environments 75, 313-329.
| Crossref | Google Scholar |
Murphy SA, Paltridge R, Silcock J, Murphy R, Kutt AS, Read J (2018) Understanding and managing the threats to night parrots in south-western Queensland. Emu - Austral Ornithology 118, 135-145.
| Crossref | Google Scholar |
Murphy BP, Woolley L-A, Geyle HM, Legge SM, Palmer R, Dickman CR, Augusteyn J, Brown SC, Comer S, Doherty TS, Eager C, Edwards G, Fordham DA, Harley D, McDonald PJ, McGregor H, Moseby KE, Myers C, Read J, Riley J, Stokeld D, Trewella GJ, Turpin JM, Woinarski JCZ (2019) Introduced cats (Felis catus) eating a continental fauna: the number of mammals killed in Australia. Biological Conservation 237, 28-40.
| Crossref | Google Scholar |
Nimmo DG, Kelly LT, Spence-Bailey LM, Watson SJ, Taylor RS, Clarke MF, Bennett AF (2013) Fire mosaics and reptile conservation in a fire-prone region. Conservation Biology 27, 345-353.
| Crossref | Google Scholar | PubMed |
O’Bryan CJ, Garnett ST, Fa JE, Leiper I, Rehbein JA, Fernández-Llamazares Á, Jackson MV, Jonas HD, Brondizio ES, Burgess ND, Robinson CJ, Zander KK, Molnár Z, Venter O, Watson JEM (2021) The importance of Indigenous Peoples’ lands for the conservation of terrestrial mammals. Conservation Biology 35, 1002-1008.
| Crossref | Google Scholar | PubMed |
Pastro LA, Dickman CR, Letnic M (2011) Burning for biodiversity or burning biodiversity? Prescribed burn vs. wildfire impacts on plants, lizards, and mammals. Ecological Applications 21, 3238-3253.
| Crossref | Google Scholar |
Pausas JG, Keeley JE (2009) A burning story: the role of fire in the history of life. BioScience 59, 593-601.
| Crossref | Google Scholar |
Perry JJ, Sinclair M, Wikmunea H, Wolmby S, Martin D, Martin B (2018) The divergence of traditional Aboriginal and contemporary fire management practices on Wik traditional lands, Cape York Peninsula, northern Australia. Ecological Management & Restoration 19, 24-31.
| Crossref | Google Scholar |
Pianka ER, Goodyear SE (2012) Lizard responses to wildfire in arid interior Australia: long-term experimental data and commonalities with other studies. Austral Ecology 37, 1-11.
| Crossref | Google Scholar |
R Core Team (2024) ‘R: a language and environment for statistical computing [Computer software].’ (R Foundation for Statistical Computing: Vienna, Austria). Available at https://www.R-project.org/
Riley J, Turpin JM, Zeale MRK, Jayatilaka B, Jones G (2021) Diurnal sheltering preferences and associated conservation management for the endangered sandhill dunnart, Sminthopsis psammophila. Journal of Mammalogy 102, 588-602.
| Crossref | Google Scholar | PubMed |
Roycroft E, Ford F, Ramm T, Schembri R, Breed WG, Burns PA, Rowe KC, Moritz C (2024) Speciation across biomes: rapid diversification with reproductive isolation in the Australian delicate mice. Molecular Ecology 33, e17301.
| Crossref | Google Scholar |
Ruscalleda-Alvarez J, Moro D, van Dongen R (2021) A multi-scale assessment of fire scar mapping in the Great Victoria Desert of Western Australia. International Journal of Wildland Fire 30, 886-898.
| Crossref | Google Scholar |
Ruscalleda-Alvarez J, Cliff H, Catt G, Holmes J, Burrows N, Paltridge R, Russell-Smith J, Schubert A, See P, Legge S (2023) Right-way fire in Australia’s spinifex deserts: an approach for measuring management success when fire activity varies substantially through space and time. Journal of Environmental Management 331, 117234.
| Crossref | Google Scholar | PubMed |
Shaw RE, James AI, Tuft K, Legge S, Cary GJ, Peakall R, Banks SC (2021) Unburnt habitat patches are critical for survival and in situ population recovery in a small mammal after fire. Journal of Applied Ecology 58, 1325-1335.
| Crossref | Google Scholar |
Short J, Turner B (1994) A test of the vegetation mosaic hypothesis: a hypothesis to explain the decline and extinction of Australian mammals. Conservation Biology 8, 439-449.
| Crossref | Google Scholar |
Smith AL, Michael Bull C, Driscoll DA (2013) Successional specialization in a reptile community cautions against widespread planned burning and complete fire suppression. Journal of Applied Ecology 50, 1178-1186.
| Crossref | Google Scholar |
Southgate R, Paltridge R, Masters P, Carthew S (2007) Bilby distribution and fire: a test of alternative models of habitat suitability in the Tanami Desert, Australia. Ecography 30, 759-776.
| Crossref | Google Scholar |
Spencer EE, Crowther MS, Dickman CR (2014) Risky business: do native rodents use habitat and odor cues to manage predation risk in Australian deserts? PLoS ONE 9, e90566.
| Crossref | Google Scholar | PubMed |
Taylor RS, Watson SJ, Nimmo DG, Kelly LT, Bennett AF, Clarke MF (2012) Landscape-scale effects of fire on bird assemblages: does pyrodiversity beget biodiversity? Diversity and Distributions 18, 519-529.
| Crossref | Google Scholar |
Trainor CR, Woinarski JCZ (1994) Responses of lizards to three experiments fires in the savanna forests of Kakadu National Park. Wildlife Research 21, 131-147.
| Crossref | Google Scholar |
TSSC (2021) Conservation advice Trichosurus vulpecula arnhemensis Northern Brushtail Possum. Department of Agriculture, Water and the Environment, Canberra, ACT, Australia. Available at https://www.environment.gov.au/cgi-bin/sprat/public/publicspecies.pl?taxon_id=83091
Turner D, Lewis M, Ostendorf B (2011) Spatial indicators of fire risk in the arid and semi-arid zone of Australia. Ecological Indicators 11, 149-167.
| Crossref | Google Scholar |
Valentine LE, Schwarzkopf L (2009) Effects of weed-management burning on reptile assemblages in Australian tropical savannas. Conservation Biology 23, 103-113.
| Crossref | Google Scholar | PubMed |
Woinarski JCZ, Burbidge AA, Harrison PL (2015) Ongoing unraveling of a continental fauna: decline and extinction of Australian mammals since European settlement. Proceedings of the National Academy of Sciences 112, 4531-4540.
| Crossref | Google Scholar |
Wright BR, Clarke PJ (2007) Fire regime (recency, interval and season) changes the composition of spinifex (Triodia spp.)-dominated desert dunes. Australian Journal of Botany 55, 709-724.
| Crossref | Google Scholar |
Wright BR, Laffineur B, Royé D, Armstrong G, Fensham RJ (2021) Rainfall-linked megafires as innate fire regime elements in arid Australian spinifex (Triodia spp.) grasslands. Frontiers in Ecology and Evolution 9, 666241.
| Crossref | Google Scholar |