A multi-modal approach to enhance Toxoplasma gondii detection in the Australian landscape
Amanda Jane Breidahl
A
B
C
D
Abstract
The disease toxoplasmosis, caused by infection with the intra-cellular protozoan parasite Toxoplasma gondii, is a potential threat to the conservation of some endothermic vertebrates. Toxoplasmosis has been reported to cause disease and acute death in many marsupials, including endangered bandicoots. Methods are required to quantify the environmental contamination with T. gondii and, hence, assess the relative threat it poses to resident species. A multi-modal approach to assess an environment for the presence of pathogens increases the likelihood of a more accurate estimate of the level of contamination and inferred risk of infection to target species.
This project aimed to use a multi-modal approach to estimate the environmental contamination with T. gondii across two separate landscapes with differing cat populations in the northern hinterland of Western Port, Victoria, Australia.
The following four parameters were used: the presence of IgG antibodies to T. gondii in free-ranging southern brown bandicoots (Isoodon obesulus) using the modified agglutination test (MAT); the presence of T. gondii DNA in the tissues of free-ranging rabbits (Oryctolagus cuniculus) and mice (Mus musculus) by real-time quantitative polymerase chain reaction (qPCR); and the presence of T. gondii oocysts in the soil using filtration methods and real-time qPCR.
Toxoplasma gondii was not identified in any of the soil samples (n = 594), southern brown bandicoots (n = 24) or mice (n = 267). Only one rabbit tested positive for T. gondii (n = 118).
The tests across soils and three different mammalian species all delivered similar results, leading to the conclusion that the landscapes measured had low T. gondii contamination at the time of assessment.
This Australian study is the first to show that the use of pathogen detection in target and two sentinel species, combined with soil analysis, may be a useful approach to assess the environmental risk of T. gondii infection in endangered and vulnerable species. Further research is recommended across sites with a gradient of T. gondii contamination and into the use of other sentinel species. Further refining of techniques for direct soil analysis in Australian conditions is recommended.
Keywords: assessment, Australia, bandicoot, environmental contamination, mice, rabbit, sentinel species, soil, Toxoplasma gondii.
Introduction
Many marsupial species have suffered significant declines since European settlement (Woinarski et al. 2014) and are subject to conservation efforts (Hill et al. 2018), including reintroduction, translocation and assisted colonisation programs. The risk of disease is an important consideration for such programs (International Union for Conservation of Nature 2013); however, there is often limited knowledge about the prevalence and impacts of disease in wildlife populations (Vaughan-Higgins 2019). Disease has been identified as one of the causes of population decline in Australian fauna both historically (Abbott 2006; Abbott 2008) and recently, including koalas (Phascolarctos cinereus) (Burbidge et al. 2008), amphibians (Scheele et al. 2019) and Tasmanian devils (Sarcophilus harrisii) (Pye et al. 2016).
Toxoplasmosis is a global disease resulting from infection with the obligate, intracellular, protozoan parasite Toxoplasma gondii. Toxoplasma gondii oocysts are shed with faeces into the environment by domestic and feral cats (Felis catus), the only known definitive host in Australia (Dubey 2010). Assessing exposure risk to wildlife of pathogens with indirect transmission pathways, such as T. gondii, poses a particular challenge, owing to the variable presence of intermediate hosts and environmental factors (Lange et al. 2016).
A range of captive Australian marsupials have been reported with clinical toxoplasmosis, often fatal, including koalas (Dubey et al. 1991), bare nosed wombats (Vombatus ursinus) (Hartley 2006), and Bennets and Tamar wallabies (Macropus rufogriseus and Macropus eugenii) (Dubey and Crutchley 2008). Disease in captive animals is usually attributed to factors such as stress, contaminated food and feline access to pens (Dubey 2010). Infection can also be highly pathogenic in free-ranging Australian marsupial species (Canfield et al. 1990), with clinical signs reported from a range of species, including the bare-nosed wombat (Donahoe et al. 2015) and the eastern barred bandicoot (Perameles gunnii) (Obendorf and Munday 1990). Factors contributing to clinical disease in free-ranging populations are not fully understood but include stress, such as poor nutritional and immune status, and exposure dose and genotype of T. gondii (Dubey 2010; Parameswaren et al. 2010). Several free-ranging Australian populations have been surveyed for production of antibody to T. gondii, with seroprevalence varying from 0% in koalas (Taggart et al. 2019), to 6.3% in common brushtail possums (Trichosurus vulpecula) (Eymann et al. 2006), 15.5% in western grey kangaroos (Macropus fuliginosus) (Parameswaren et al. 2009), 71% in the spotted quoll (Dasyurus maculatus) (Hollings et al. 2013) and from 9.4 to 100% in the eastern quoll (Dasyurus viverrinus) (Hollings et al. 2013; Fancourt et al. 2014). Seroprevalence appears to be influenced by many factors, including cat density (Hollings et al. 2013), urban and peri-urban location (Hillman et al. 2017) and cooler, wetter geographic clines (Hollings et al 2013; Fancourt et al 2014). The ecology of a species may also influence risk of infection, with the arboreal lifestyle of free-ranging koalas limiting exposure to the parasite (Taggart et al. 2019), whereas animals grazing in wetter, cooler areas may be more exposed, as are higher-order carnivores (Hollings et al. 2013). It is difficult to know how many marsupial species are infected with T. gondii and what effect the parasite has on the ecology of free-ranging populations, because investigations, particularly longitudinal studies, are limited (Hillman et al. 2016).
The eastern barred bandicoot and the southern brown bandicoot (Isoodon obesulus) are listed as Endangered in Victoria and Australia-wide respectively (Flora and Fauna Gaurantee Act 1988 (Vic.), Threatened Species List June 2023 – Department of Energy, Environment and Climate Action). They are subject to active conservation management and thought to be highly susceptible to toxoplasmosis (Obendorf and Munday 1990; Groenewegen et al. 2017). A Tasmanian free-ranging population of eastern barred bandicoots was found to have a seroprevalence of 6.7% (Obendorf et al 1996). Infection, but not clinical disease, has also been reported from quenda (Isoodon fusciventer) (Hillman et al. 2017), now considered a species separate from the southern brown bandicoot (Travouillon and Phillips 2018).
Bandicoots become infected by ingesting vegetation, soil or water contaminated with sporulated oocytes or by feeding on transport hosts, such as invertebrates (Bettiol et al. 2000a). Subsequently, multi-organ infection can lead to clinical signs (neurological, respiratory, optical and gastro-intestinal) ranging from mild disease to acute death (Bettiol et al. 2000b). Although toxoplasmosis is an important disease threat to bandicoots individually, the impact that T. gondii has on bandicoot population dynamics is unknown. A pilot re-introduction program of 18 eastern barred bandicoots to French Island did demonstrate that T. gondii infected several animals, resulting in at least two deaths and one case of clinical disease (Groenewegen et al. 2017). Hence, the risk of exposure to the parasite must be considered as part of National Recovery Plans (Department of Land, Water, Environment and Planning 2021) and conservation programs for these species (Groenewegen et al. 2017).
Exposure to a pathogen can be estimated by various methods, including prevalence studies of target (Obendorf et al. 1996) and sentinel species (Anderson et al. 2017) and direct environmental sampling (Lélu et al. 2011). For example, in New Zealand, prevalence of Mycobacterium bovis across a landscape has been measured assessing prevalence of infection in the wildlife reservoir, common brushtail possums, the target species, domestic cattle (Bos taurus) (Anderson et al. 2017) and pigs (Sus scrofa) released for the purpose of acting as sentinels (Nugent et al. 2014). Given the indirect life cycle and broad host susceptibility of T. gondii, and the likely clustered distribution of infective oocysts in the environment, a multi-modal approach may improve the predicted estimates of environmental contamination with this parasite. Two species that could act as sentinel species for T. gondii infection in bandicoot species are the introduced European rabbit (Oryctolagus cuniculus) and the feral house mouse (Mus musculus). Despite having slightly different diets, they occupy similar habitats, with fossorial habits similar to those of bandicoots and are therefore likely to have a similar exposure and risk of infection (Tann et al. 1991; Valentine et al. 2012). They are relatively resistant to developing clinical toxoplasmosis after infection (Dubey 2010), widespread across Australia, abundant, easy to harvest and have a short lifespan and small territorial range. Free-ranging rabbits have been shown to become infected with T. gondii in a range of Australian conditions (Cox et al. 1981). Natural infection with T. gondii in mice has not yet been demonstrated in Australia but has been reported in the United Kingdom (Marshall et al. 2004), Czechoslovakia (Hůrková-Hofmannová et al. 2014) and California, USA (Dabritz et al. 2008).
This study aimed to assess a multi-modal approach to enhance the detection and infer relative abundance of T. gondii in the environment, with specific reference to southern brown bandicoots. Seroprevalence of T. gondii in the target bandicoot species, detection of T. gondii infection in two sentinel species by real-time polymerase chain reaction (PCR) and environmental sampling for T. gondii oocyst DNA by real-time PCR were used to compare two sites, one with and one without the presence of cats.
Materials and methods
Study sites
Two study sites were selected on the basis of the presence or absence of free-roaming cats and the presence of free-ranging southern brown bandicoots (Maclagan et al. 2018), mice and rabbits (Nicholls et al. 2018) (Fig. 1). Study Site A, the Royal Botanic Gardens Cranbourne (RBGC) (−38.127307, 145.285873), ~70 km south-east of Melbourne, consists of 380 ha of remnant bush (Maclagan et al. 2018), as well as formal, irrigated native gardens. It has a predator-exclusion fence. Regular surveys with spotlights and trail cameras have not recorded any cats at the site from January 2011 to December 2016 (Nicholls et al. 2018). Study Site B represents three broad areas outside the RBGC: (1) an area with a radius of up to 1 km immediately surrounding the RBGC, including a golf course, roadside verges and local farms, (2) a private, rural property west of Tooradin (−38.213357, 145.431655), and (3) rural roadsides and drains surrounding the townships of Koo Wee Rup (KWR) (−38.193288, 145.488764) and Bayles (−38.177209, 145.568017). Free-ranging cats are known to occur across Study Site B (Nicholls et al. 2018).
Collection or capture of study animals
Rabbits were sourced opportunistically from feral pest control programs from both study sites from July 2016 until February 2018. Rabbit heads were collected and stored at −20°C. They were later thawed, and both eyes, the tongue, 10 g of skeletal muscle and the entire brain were dissected from the carcase and re-frozen at −20°C.
Trapping for live southern brown bandicoots and mice occurred at Study Site A over 8 nights in March 2017 (425 trap nights) and at Study Site B over 16 nights in March–April 2017 (574 trap nights). At Study Site A, traps were set at 50 m intervals on both sides of access tracks in the bushland and at irregular intervals close to known bandicoot habitat within the formal gardens. At Study Site B, traps were set at 25 m intervals along three roads known to be frequented by southern brown bandicoots on the basis of previous studies (Maclagan 2016) between Koo Wee Rup and Bayles (see Fig. 2). Sixteen further traps were set at a private rural property, ~50 m apart. At each trap site, one wire mesh cage trap (500 × 250 × 350 mm) and two collapsible aluminium Elliott traps (150 × 160 × 450 mm) were placed in concealed positions under low vegetation (Tasker and Dickman 2001). Traps were baited with a rolled oat and peanut butter mixture (Paull et al. 2011), set prior to dusk and checked within 1 h either side of dawn the following morning. Any non-target species were identified and immediately released. All trapped mice were immediately euthanased on site, using inhalant isoflurane anaesthetic. Brain, heart, lung, liver, kidney, spleen and skeletal muscle were later collected and frozen at −20°C.
Sites of trap placement for southern brown bandicoots (Isoodon obesulus obesulus) and Mus musculus and soil collection sites. (a) Study Site A: Royal Botanic Gardens Cranbourne (RBGC) (red dots) and some of Study Site B outside the RBGC (blue dots). (b) Remainder of Study Site B, showing trap placement along Railway Road, Koo Wee Rup; Boundary Drain Road, from Koo Wee Rup to Bayles and at ‘Harewood’, Tooradin in the east.
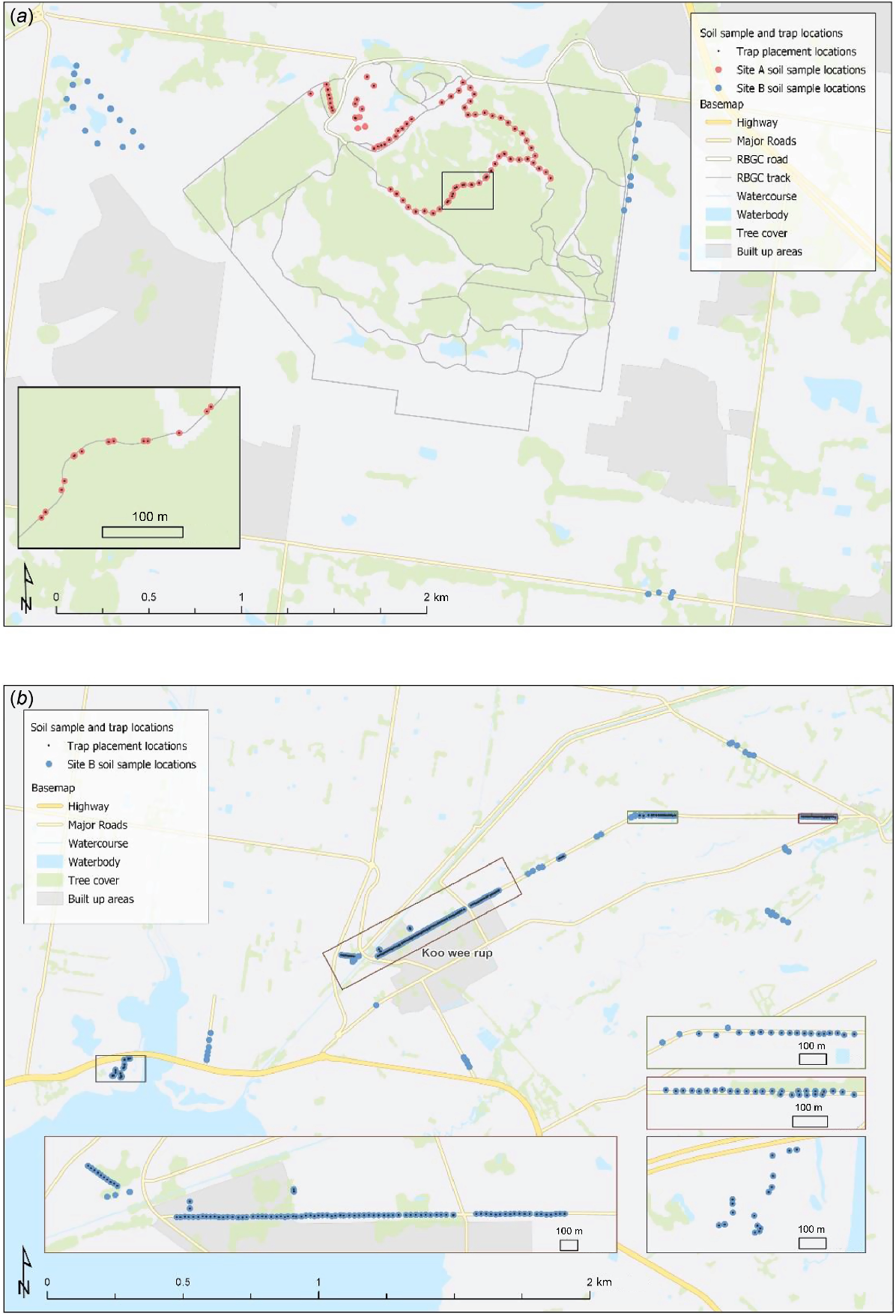
Trapped southern brown bandicoots were transferred into a calico bag and weighed. Bandicoots weighing less than 400 g were released, because they were considered too young to anaesthetise. Those weighing >400 g were transported to a central processing site and were anaesthetised using 5% isoflurane and 2 L O2 min−1 reduced to a maintenance rate of 1.5% isoflurane. Up to 2 mL of blood was withdrawn from the femoral vein with a 25-gauge needle. The blood was placed into labelled serum separator tubes (BD Vacutainer, Franklin Lakes, NJ, USA) and centrifuged for 3 min at 3600 rpm at ambient room temperature. Serum was frozen at −20°C until analysis. Bandicoots were released at the capture site at dusk.
Modified agglutination test
A modified agglutination test (MAT) was run on the stored bandicoot serum samples by using the Toxo Screen DA kit (Biomerieux, Marcy-l’Étoile, France) with modifications, as used by the National Association of Testing Authority (NATA)-accredited Mt Pleasant Laboratories (Department of Primary Industries, Water and Environment), Launceston, Tas., and as described by Adriaanse et al (2020). The Adriaanse et al. (2020) study found that sensitivity and specificity of the MAT were 96.2 and 82.1% respectively in feral cats. This is the most frequently used method to detect T. gondii immunoglobulin G (IgG) antibodies in marsupials.
Mammalian DNA extraction and real-time quantitative polymerase chain reaction (qPCR)
Tissue samples from the necropsied rabbits and mice were thawed. For rabbits, 2 g of brain, 1 g of eye retinal tissue, 1 g of tongue and 1 g of skeletal muscle were pooled. For mice, all collected tissues were pooled and weighed. Tris-ethylenediaminetetraacetic acid (EDTA) lysis buffer (40 mM of Tris, 10 mM of EDTA, Sigma–Aldrich, St Louis, MO, USA) was added to the tissues at the ratio of 10 mL per 5 g of tissue, vortexed and incubated for 10 min in a 90°C water bath. Further steps in the artificial tissue digestion were performed as per Cuttell et al (2012), with minor adjustments according to Adriaanse et al. (2020).
The real-time quantitative PCR (qPCR) was run in 20 μL volumes containing GoTaq Probe qPCR Master mix (Promega, Madison, WI, USA) with 1 μL of known quantity of equine herpes virus (EHV4) genomic DNA as an internal control, and 2 μL of template DNA. Toxoplasma gondii genomic DNA sourced from culture was used as a positive control for each qPCR run. All primers and probes were sourced from Integrated DNA Technologies (IDT, Coralville, IA, USA). Primers, probes, their gene targets and final concentrations are outlined in Table 1.
Item | Sequence | Gene target | Concentration per reaction | Citation | |
---|---|---|---|---|---|
Toxorep probe | 5Cy5/ACG CTT TCC/TAO/TCG TGG TGA TGG CG/31AbRQSp/ | 529 bp highly repetitive element | 150 nM | Lélu et al. (2012) | |
Toxorep forward primer | 5′-AGA GAC ACC GGA ATG CGA TCT-3′ | 150 nM | |||
Toxorep reverse primer | CCC TCT TCT CCA CTC TTC AAT TCT | 150 nM | |||
EHV probe | 5′-/5HEX/TTT CGC GTG/ZEN/CCT CCT CCA G/31ABkFQ/−3′ | gB | 100 nM | Ng-Nguyen et al. (2017) | |
EHV forward primer | 5′-GAT GAC ACT AGC GAC TTC GA-3′ | 40 nM | |||
EHV reverse primer | 5′-CAG GGC AGA AAC CAT AGA CA-3′ | 40 nM | |||
Mammalian probe | 5′-/56-FAM/CGT GCC TAC/ZEN/CAT GGT GAC CAC/31ABkFQ/-3′ | 18S ribosomal RNA | 350 nM | This study | |
Mammalian forward primer | 5′-CGA ACG TCT GCC CTA TCA AC-3′ | 250 nM | |||
Mammalian reverse primer | 5′-CGT TTC TCA GGC TCC CTC T-3′ | 250 nM |
A Magnetic Induction Cycler (MIC) Personal qPCR Cycler (Bio Molecular Systems, Upper Coomera, Qld, Australia) was used to perform the reactions. The first cycle was set for incubation at 95°C for 2 min, and there followed 40 cycles of alternating denaturation at 95°C for 15 s and annealing and extension at 60°C for 60 s. Cycling analysis was set to ‘dynamic’, with a quantification threshold (Cq) of 0.01 (Automatic) and a fluorescence cut-off level of 5%, except for EHV4, which had a fluorescence cut-off of 20%. The first cycle was ignored.
A threshold cycle (Ct) of ≤36 was identified as a positive cut-off, at which concentrations of 0.00005 pg μL−1 were the lowest dilution that a sample was reliably identified as positive. A sample was deemed to be positive if both wells were positive. DNA extraction and qPCR were repeated if EHV4 in the sample was greater by two or more Ct compared with the negative control or if the mammalian DNA extraction control failed to amplify. The estimated sensitivity and specificity for the qPCR protocol was 90.1% and 96.0% respectively (Adriaanse et al. 2020). This is the most frequently used and accurate current method to detect T. gondii antigen in tissue samples.
Soil sampling, oocyst recovery and soil qPCR
In total, 297 soil samples were collected during the first 2 weeks of September 2017 (Fig. 2). Soil samples were taken within 10 m of every trap GPS position at both study sites. Further soil samples were taken from random locations along the roadside verges corresponding to where rabbits were sourced (Fig. 2). At each sampling site, five soil samples were taken from five relatively evenly spaced spots within a 1 m2 area, by using a cylindrical core 25 mm wide × 50 mm deep. Samples were placed inside ziplock bags, double bagged, labelled and stored in the dark at 4°C. All samples remained moist and were processed within 3 months of collection.
The oocyst recovery protocol was adapted from Lélu et al. (2011) and Gotteland et al. (2014) and had previously been validated in our laboratory (Adriaanse 2018). The technique departed from Lélu et al (2011) in that it did not include an initial overnight soaking step with sulfuric acid and we used a mechanical bead beater to rupture the oocysts, instead of freeze–thaw cycles.
The qPCR followed the same protocol as used for the mammalian qPCR, with the exception that instead of the mammalian primers and probes, the qPCR assay included primers and probes specific for the amplification of eukaryote DNA as an isolation and DNA extraction control. These forward and reverse primers, 5′ GCC CTA TCA ACT TTC GAT GGT AG 3′ and 5′ CTA CCA CAT CCA AGG AAG GC 3′, (450 nM), and probe, 5′ CGG AGA GGG AGC CTG AGA AAC G 3′ (250 nM), were added to the final mastermix and optimised to run as a multiple assay with T. gondii and EHV4 as the target and internal qPCR control respectively. The cycling conditions and analysis were the same as for the mammalian qPCRs. The cut-off level of Cq37 was found to be the highest cycling level to detect the presence of T. gondii DNA at a dilution of 0.000001 pg.
Statistics
Prevalence and 95% confidence intervals were calculated using the Wilson method (Sergeant 2018).
Ethics statement
All trapping and animal work adhered to the guidelines provided in Use of Australian Native Mammals in Research and Teaching (NHMRC 2014) and were performed under the University of Melbourne Animal Ethics Committee Approval Number 1613973.1, the Department of Environment, Land, Water and Planning (DELWP) Permit Number 10008263 and a Royal Botanic Gardens Cranbourne Research Permit RBGC-1701.
Results
At Study Site A, 15 individual southern brown bandicoots were captured (8 males and 7 females) in total, 13 of which were suitable for sampling. Eight bandicoots (seven males and one female) were captured over 411 trap nights in remnant, natural bushland within the site, and seven (six females and one male) on 1 night (14 trap nights) in the formal gardens. Three mice were trapped and euthanased and eleven dead rabbits were obtained (Table 2). At Study Site B, 14 individual southern brown bandicoots (3 males and 10 females, 1 of unknown sex) were captured, 11 of which were suitable for sampling. In addition, 264 mice were captured and euthanased and 107 dead rabbits were obtained (Table 2).
Site | CTNs | ETNs | SBB trapped | SBB sampled | Mice | Rabbits | |
---|---|---|---|---|---|---|---|
Site A | 425 | 850 | 15 | 13 | 3 | 11 | |
Site B | 574 | 1148 | 14 | 11 | 264 | 107 | |
Total | 999 | 1998 | 29 | 24 | 267 | 118 |
CTN, cage trap night; ETN, Elliot trap night.
All 24 southern brown bandicoot blood samples tested negative for IgG antibodies to T. gondii on the MAT, resulting in an apparent seroprevalence of 0% (95% confidence interval: 0–13.80%). The mice qPCR results (n = 267) were all negative for T. gondii DNA, giving an apparent prevalence of 0% (0–1.42%) (Table 3). All qPCR testing of rabbits (n = 118) was negative for T. gondii DNA, except for one rabbit from Study Site B (sourced in April 2017 near the Koo Wee Rup sewerage treatment plant). This gave an apparent prevalence of T. gondii infection in rabbits of 0.85% (1/118) (0.15–4.64%) over both study sites and 0.93% (1/107) from Study Site B (0.17–5.10%). In total, 594 soil aliquots were tested from 297 sites, all of which tested negative for T. gondii DNA, giving an apparent prevalence of 0% (0/297) (0–1.28%) (Table 3).
Item | SBB (%) (CL) | Rabbit (%) (CL) | Mouse (%) (CL) | Soil (%) (CL) | |
---|---|---|---|---|---|
(MAT) | (qPCR) | (qPCR) | (qPCR) | ||
Study Site A | 0 (22.8) n = 13 | 0 (25.9) n = 11 | 0 (56.1) n = 3 | 0 (5.1) n = 72 | |
Study Site B | 0 (25.9) n = 11 | 0.93 (5.1) n = 107 | 0 (1.4) n = 264 | 0 (1.7) n = 225 | |
Combined | 0 (13.8) n = 24 | 0.85 (4.6) n = 118 | 0 (1.4) n = 267 | 0 (1.3) n = 297 |
The 95% upper confidence limit (CL) is indicated in parentheses after the prevalence value. MAT, modified agglutination test; qPCR, real-time qualitative PCR; n, sample size.
Discussion
The lack of detected exposure in target and sentinel species, as well as oocysts in soil samples, suggests that the environments sampled have a very low contamination with T. gondii, and that the risk of infection for southern brown bandicoots at these sites is also low. However, the interpretation of the apparent absence of a pathogen is complex.
The results from Study Site A were consistent with an absence of cats since 2011, given oocyst survival rate in the environment is unlikely to extend beyond 2 years (Dubey 2010). However, the lack of detected cases at Study Site B, which had a known feline presence (Nicholls et al. 2018), was more surprising, because other studies have shown a correlation between likelihood of infection with T. gondii in intermediate hosts and cat density (Fancourt et al. 2014). It may be that either the cat density for Study Site B was relatively low, or that few cats were shedding oocysts. Climate should not have been a contributing factor to the apparent low prevalence of T. gondii at Study Site B, which experiences cool, wet seasons over winter, with a mean annual rainfall of 770.5 mm (Bureau of Meteorology 2019), generally favouring oocyst survival (Dubey 2010). In addition, sampling occurred during September, in optimal climatic conditions.
Other factors may have affected the seroprevalence findings in bandicoots. The low sample size resulted in a relatively wide 95% confidence interval, which includes the seroprevalence values of 3.7% (n = 54) in urban environments and 2.9% (n = 104) in bushland habitats observed in quenda (Hillman et al. 2017). Further, because the MAT measures only IgG antibodies, any animals in the initial 2–3 weeks of infection, before production of IgG, would not have been identified (Bettiol et al. 2000b), nor would any that died undetected from the acute form of the disease (Wobeser 2007), or as a result of predation or misadventure secondary to infection with T. gondii. However, it is unlikely that the sentinel eutherian species, which all, bar one rabbit, tested negative as well, would also have died from toxoplasmosis prior to production of IgG (Dubey 2010). The findings therefore do not support the hypothesis of a high prevalence in bandicoots masked by a lack of sero-conversion or high rate of undetected acute deaths.
In contrast to the lack of detection of infection with T. gondii in rabbits in the present study, rabbits at nearby Phillip Island, which has climatic conditions similar to those of Study Site B, have recently been determined to have a 10.5% (n = 134) prevalence of infection by using the same qPCR protocol as in this study (Adriaanse 2018). The only T. gondii-positive rabbit in our study was sourced from near the Koo Wee Rup township sewerage treatment plant, with effluent potentially having been the source of infection for this rabbit, as was postulated by Cox et al. (1981). The reasons for the differences between the Phillip Island and present study are not clear, but could reflect a higher cat density (3.4 cats km−2) (Legge et al. 2017) and prevalence of infection in cats at the former (Adriaanse et al. 2020). By contrast, the lack of demonstrated infection with T. gondii in wild mice reported here is consistent with the result of the two other, smaller studies in Australia, which included 4 (Smith and Munday 1965) and 55 mice (Taggart 2019). It is possible that the short lifespan and small home range of mice decreases the probability of exposure to T. gondii oocysts (Afonso et al. 2007). Mice may therefore be a less useful sentinel species than are rabbits, particularly in Australian conditions.
No T. gondii oocysts were detected in soil samples from either study site. The variations we made were similar to those made by Escotte-Binet et al. (2019), which additionally included an initial step of using a stomacher 250 μm filtration bag to remove larger soil particles. It is possible the addition of this new step, combined with using larger soil volumes, would have enhanced our chances of oocyst recovery, because Australian conditions may not have the same density of oocysts as in France (Gotteland et al. 2014). The probability of obtaining positive soil samples in our study may have been affected by the soil types (Lélu et al. 2011) and the decision to collect soil on the basis of animal capture sites, rather than targeting areas that are likely to have the highest oocyst concentrations, such as cat latrines (Afonso et al. 2010), or using a truly randomised grid. However, concentrating sampling in areas where cat latrines were likely to occur would bias prevalence and would not necessarily reflect the movement of oocysts through the environment via water and transport hosts, nor the actual risk of exposure of the intermediate hosts of interest at their feeding sites. Adriaanse (2018) did collect soil samples (n = 412) on a strictly randomised grid pattern but also had 0% detection of T. gondii oocysts, despite a high seroprevalence in cats of 91.8% (n = 97) and a cat density of 3.4 km−2 (Legge et al. 2017). Soil samples may thus not be a sensitive method to detect environmental T. gondii contamination, at least not in the Australian environments investigated so far.
Conservation management of marsupials thought to be particularly sensitive to toxoplasmosis, such as bandicoots, benefit from the development of strategies that allow increased sensitivity of detection T. gondii in the ecosystem, as well as informing on the likely environmental pathogen pressure of T. gondii for that specific host species. Our results broadly support the hypothesis that eutherian species with similar ecological niches as the target host may be able to act as sentinels for exposure to T. gondii. The consistent findings over two different sites and four parameters support the conclusion that the level of environmental contamination with T. gondii oocysts at the two sites studied was relatively low at the time of investigation. Importantly, multi-modal approaches to assessing level of exposure to a pathogen, incorporating laboratory methods of high sensitivity and specificity (Adriaanse et al. 2020), provide greater certainty of results than does using a single parameter. However, because the sites investigated appeared to have near-zero prevalence, it was not possible to confirm a significant associations among the prevalence of exposure in the target host (southern brown bandicoot), sentinels (mouse and rabbit) and soil oocyst concentration, or to extrapolate the usefulness of this methodology at higher levels of contamination.
This is the first prevalence study for T. gondii in southern brown bandicoots and mice in Victoria and the first large-scale study for this parasite in Australian mice. Further research is recommended across sites with a gradient of T. gondii contamination, along with further investigation into the use of other sentinel species and refining techniques for direct soil analysis in Australian conditions.
Data availability
The data that support this study are available in the article. This paper forms part of the A. J. Breidahl’s Masters of Veterinary Science thesis (Breidahl 2020) (see http://hdl.handle.net/11343/241952).
Declaration of funding
This research was funded by a University of Melbourne Early Career Researcher Grant awarded to Dr Jasmin Hufschmid (Melbourne Veterinary School, Faculty of Science, The University of Melbourne). Zoos Victoria and Phillip Island Nature Parks provided some of the equipment and personnel used in the field work. Equine Herpes Virus genomic DNA was sourced from cultured cells provided by Dr Carol Hartley, Faculty Veterinary and Agricultural Science, The University of Melbourne. Toxoplasma gondii genomic DNA was sourced from culture provided by Dr Chris Tonkin, Walter Eliza Hall Institute.
References
Abbott I (2006) Mammalian faunal collapse in Western Australia, 1875–1925: the hypothesised role of epizootic disease and a conceptual model of its origin, introduction, transmission, and spread. Australian Zoologist 33, 530-561.
| Crossref | Google Scholar |
Abbott I (2008) Historical perspecitves of the ecology of some conspicuous vertebrate species in south-west Western Australia. Conservation Science Western Australia 6, 1-214.
| Google Scholar |
Adriaanse K, Firestone SM, Lynch M, Rendall AR, Sutherland DR, Hufschmid J, Traub RJ (2020) Comparison of the modified agglutination test and real-time PCR for detection of Toxoplasma gondii exposure in feral cats from Phillip Island, Australia, and risk factors associated with infection. International Journal for Parasitology: Parasites and Wildlife 12, 126-133.
| Crossref | Google Scholar | PubMed |
Afonso E, Thulliez P, Pontier D, Gilot-Fromont E (2007) Toxoplasmosis in prey species and consequences for prevalence in feral cats: not all prey species are equal. Parasitology 134, 1963-1971.
| Crossref | Google Scholar | PubMed |
Afonso E, Thulliez P, Gilot-Fromont E (2010) Local meteorological conditions, dynamics of seroconversion to Toxoplasma gondii in cats (Felis catus) and oocyst burden in a rural environment. Epidemiology & Infection 138, 1105-1113.
| Crossref | Google Scholar | PubMed |
Anderson DP, Gormley AM, Bosson M, Livingstone PG, Nugent G (2017) Livestock as sentinels for an infectious disease in a sympatric or adjacent-living wildlife reservoir host. Preventive Veterinary Medicine 148, 106-114.
| Crossref | Google Scholar | PubMed |
Bettiol SS, Obendorf DL, Nowarkowski M, Milstein T, Goldsmid JM (2000a) Earthworms as paratenic hosts of toxoplasmosis in eastern barred bandicoots in Tasmania. Journal of Wildlife Diseases 36, 145-148.
| Crossref | Google Scholar | PubMed |
Bettiol SS, Obendorf DL, Nowarkowski M, Goldsmid JM (2000b) Pathology of experimental toxoplasmosis in eastern barred bandicoots in Tasmania. Journal of Wildlife Diseases 36, 141-144.
| Crossref | Google Scholar | PubMed |
Breidahl AJ (2020) An investigation of the role Toxoplasma gondii may play in the health of the southern brown bandicoot (Isoodon obesulus) and an assessment of environmental contamination with T. gondii. MVetSci thesis, Melbourne Veterinary School, The University of Melbourne, Parkville, Vic., Australia. Available at http://hdl.handle.net/11343/241952
Burbidge AA, Mckenzie NL, Brennan KEC, Woinarski JCZ, Dickman CR, Baynes A, Gordon G, Menkhorst PW, Robinson AC (2008) Conservation status and biogeography of Australia’s terrestrial mammals. Australian Journal of Zoology 56, 411-422.
| Crossref | Google Scholar |
Bureau of Meteorology (2019) Climate statistics for Australian locations: Monthly climate statistics. All years of record. Summary statistics CRANBOURNE BOTANIC GARDENS. (BOM) Available at http://www.bom.gov.au/climate/averages/tables/cw_086375.shtml
Canfield PJ, Hartley WJ, Dubey JP (1990) Lesions of toxoplasmosis in Australian marsupials. Journal of Comparative Pathology 103, 159-167.
| Crossref | Google Scholar | PubMed |
Cox JC, Edmunds JW, Shepherd RC (1981) Toxoplasmosis and the wild rabbit Oryctolagus cuniculus in Victoria, Australia with suggested mechanisms for dissemination of oocysts. Journal of Hygiene 87, 331-337.
| Crossref | Google Scholar | PubMed |
Cuttell L, Corley SW, Gray CP, Vanderlinde PB, Jackson LA, Traub RJ (2012) Real-time PCR as a surveillance tool for the detection of Trichinella infection in muscle samples from wildlife. Veterinary Parasitology 188, 285-293.
| Crossref | Google Scholar | PubMed |
Dabritz HA, Miller MA, Gardner IA, Packham AE, Atwill ER, Conrad PA (2008) Risk factors for Toxoplasma gondii infection in wild rodents from central coastal California and a review of T. gondii prevalence in rodents. Journal of Parasitology 94, 675-683.
| Crossref | Google Scholar | PubMed |
Donahoe SL, Šlapeta J, Knowles G, Obendorf D, Peck S, Phalen DN (2015) Clinical and pathological features of toxoplasmosis in free-ranging common wombats (Vombatus ursinus) with multilocus genotyping of Toxoplasma gondii type II-like strains. Parasitology International 64, 148-153.
| Crossref | Google Scholar | PubMed |
Dubey JP, Crutchley C (2008) Toxoplasmosis in wallabies (Macropus rufogriseus and Macropus eugenii): blindness, treatment with atovaquone, and isolation of Toxoplasma gondii. Journal of Parasitology 94, 929-933.
| Crossref | Google Scholar | PubMed |
Dubey JP, Hedstrom O, Machado CR, Osborn KG (1991) Disseminated toxoplasmosis in a captive koala (Phascolarctos cinereus). Journal of Zoo and Wildlife Medicine 22, 348-350.
| Google Scholar |
Escotte-Binet S, Da Silva AM, Cancès B, Aubert D, Dubey J, La Carbona S, Villena I, Poulle ML (2019) A rapid and sensitive method to detect Toxoplasma gondii oocysts in soil samples. Veterinary Parasitology 274, 108904.
| Crossref | Google Scholar | PubMed |
Eymann J, Herbert CA, Cooper DW, Dubey JP (2006) Serologic survey for Toxoplasma gondii and Neospora caninum in the common brushtail possum (Trichosurus vulpecula) from urban Sydney, Australia. Journal of Parasitology 92, 267-272.
| Crossref | Google Scholar | PubMed |
Fancourt BA, Nicol SC, Hawkins CE, Jones ME, Johnson CN (2014) Beyond the disease: Is Toxoplasma gondii infection causing population declines in the eastern quoll (Dasyurus viverrinus)? International Journal for Parasitology Parasites and Wildlife 3, 102-112.
| Crossref | Google Scholar | PubMed |
Gotteland C, Gilot-Fromont E, Aubert D, Poulle M-L, Dupuis E, Dardé M-L, Forin-Wiart M-A, Rabilloud M, Riche B, Villena I (2014) Spatial distribution of Toxoplasma gondii oocysts in soil in a rural area: influence of cats and land use. Veterinary Parasitology 205, 629-637.
| Crossref | Google Scholar | PubMed |
Groenewegen R, Harley D, Hill R, Coulson G (2017) Assisted colonisation trial of the eastern barred bandicoot (Perameles gunnii) to a fox-free island. Wildlife Research 44, 484-496.
| Crossref | Google Scholar |
Hartley MP (2006) Toxoplasma gondii infection in two common wombats (Vombatus ursinus). Australain Veterinary Journal 84, 107-109.
| Crossref | Google Scholar |
Hillman AE, Lymbery AJ, Andrew Thompson RC (2016) Is Toxoplasma gondii a threat to the conservation of free-ranging Australian marsupial populations? International Journal for Parasitology Parasites and Wildlife 5, 110-115.
| Crossref | Google Scholar | PubMed |
Hillman AE, Lymbery AJ, Elliot AD, Andrew Thompson RC (2017) Urban environments alter parasite fauna, weight and reproductive activity in the quenda (Isoodon obesulus). Science of the Total Environment 607–608, 1466-1478.
| Crossref | Google Scholar | PubMed |
Hollings T, Jones M, Mooney N, McCallum H (2013) Wildlife disease ecology in changing landscapes: mesopredator release and toxoplasmosis. International Journal for Parasitology Parasites and Wildlife 2, 110-118.
| Crossref | Google Scholar | PubMed |
Hůrková-Hofmannová L, Qablan MA, Juránková J, Modrý D, Piálek J (2014) A survey of Toxoplasma gondii and Neospora caninum infecting house mice from a hybrid zone. Journal of Parasitology 100, 139-141.
| Crossref | Google Scholar | PubMed |
International Union for Conservation of Nature (2013) ‘Guidelines for reintroductions and other conservation translocations.’ (IUCN: Gland, Switzerland) Available at https://portals.iucn.org/library/efiles/documents/2013-009.pdf
Lange M, Kramer-Schadt S, Thulke HH (2016) Relevance of indirect transmission for wildlife disease surveillance. Frontiers in Veterinary Science 3, 110.
| Crossref | Google Scholar |
Legge S, Murphy BP, Mcgregor H, Woinarski JCZ, Augusteyn J, Ballard G, Baseler M, Buckmaster T, Dickman CR, Doherty T, Edwards G, Eyre T, Fancourt BA, Ferguson D, Forsyth DM, Geary WL, Gentle M, Gillespie G, Greenwood L, Hohnen R, Hume S, Johnson CN, Maxwell M, Mcdonald PJ, Morris K, Moseby K, Newsome T, Nimmo D, Paltridge R, Ramsey D, Read J, Rendall A, Rich M, Ritchie E, Rowland J, Short J, Stokeld D, Sutherland DR, Wayne AF, Woodford L, Zewe F (2017) Enumerating a continental-scale threat: how many feral cats are in Australia? Biological Conservation 206, 293-303.
| Crossref | Google Scholar |
Lélu M, Gilot-Fromont E, Aubert D, Richaume A, Afonso E, Dupuis E, Gotteland C, Marnef F, Poulle ML, Dumètre A, Thulliez P, Dardé ML, Villena I (2011) Development of a sensitive method for Toxoplasma gondii oocyst extraction in soil. Veterinary Parasitology 183, 59-67.
| Crossref | Google Scholar | PubMed |
Lélu M, Villena I, Dardé ML, Aubert D, Geers R, Dupuis E, Marnef F, Poulle ML, Gotteland C, Dumètre A, Gilot-Fromont E (2012) Quantitative estimation of the viability of Toxoplasma gondii oocysts in soil. Applied and Environmental Microbiology 78, 5127-5132.
| Crossref | Google Scholar | PubMed |
Maclagan S (2016) Ecology and conservation of the southern brown bandicoot in an urbanising landscape. The Victorian Naturalist 133, 103-106.
| Google Scholar |
Maclagan SJ, Coates T, Ritchie EG (2018) Don’t judge habitat on its novelty: assessing the value of novel habitats for an endangered mammal in a peri-urban landscape. Biological Conservation 223, 11-18.
| Crossref | Google Scholar |
Marshall PA, Hughes JM, Williams RH, Smith JE, Murphy RG, Hide G (2004) Detection of high levels of congenital transmission of Toxoplasma gondii in natural urban populations of Mus domesticus. Parasitology 128, 39-42.
| Crossref | Google Scholar | PubMed |
Ng-Nguyen D, Stevenson MA, Traub RJ (2017) A systematic review of taeniasis, cysticercosis and trininellosis in Vietnam. Parasites and Vectors 10, 150.
| Crossref | Google Scholar |
Nicholls DG, Coates TD, Ibbetson SA (2018) Assessment of the monitoring of ground-dwelling mammals in the northern Western Port, Victoria. The Victorian Naturalist 135, 96-107.
| Google Scholar |
Nugent G, Yockney IJ, Whitford EJ, Cross ML (2014) Assessing the effectiveness of tuberculosis management in brushtail possums (Trichosurus vulpecula), through indirect surveillance of Mycobacterium bovis infection using released sentinel pigs. Veterinary Medicine International 2014, 361634.
| Crossref | Google Scholar |
Obendorf DL, Statham P, Driessen M (1996) Detection of agglutinating antibodies to Toxoplasma gondii in sera from free-ranging eastern barred bandicoots (Perameles gunnii). Journal of Wildlife Diseases 32, 623-626.
| Crossref | Google Scholar | PubMed |
Parameswaren N, O’Handley RM, Grigg ME, Fenwick SG, Thompson RCA (2009) Seroprevalence of Toxoplasma gondii in wild kangaroos using an ELISA. Parasitology International 58, 161-165.
| Crossref | Google Scholar |
Parameswaren N, Thompson RCA, Sundar N, Pan S, Johnson M, Smith NC, Grigg ME (2010) Non-archetypal Type II-like and atypical strains of Toxoplasma gondii infecting marsupials of Australia. International Journal for Parasitology 40, 435-640.
| Crossref | Google Scholar |
Paull DJ, Claridge AW, Barry SC (2011) There’s no accounting for taste: bait attractants and infrared digital cameras for detecting small to medium ground-dwelling mammals. Wildlife Research 38, 188-195.
| Crossref | Google Scholar |
Pye RJ, Pemberton D, Tovar C, Tubio JMC, Dun KA, Fox S, Darby J, Hayes D, Knowles GW, Kreiss A, Siddle HVT, Swift K, Lyons AB, Murchison EP, GM Woods (2016) A second transmissible cancer in Tasmanian devils. Proceedings of the National Academy of Sciences 113, 374-379.
| Crossref | Google Scholar |
Scheele BC, Pasmans F, Skerratt LF, Berger L, Martel A, Beukema W, Acevedo AA, Burrowes PA, Carvalho T, Catenazzi A, De la Riva I, Fisher MC, Flechas SV, Foster CN, Frías-Álvarez P, Garner TWJ, Gratwicke B, Guayasamin JM, Hirschfeld M, Kolby JE, Kosch TA, La Marca E, Lindenmayer DB, Lips KR, Longo AV, Maneyro R, Mcdonald CA, Mendelson J, III, Palacios-Rodriguez P, Parra-Olea G, Richards-Zawacki CL, Rödel M-O, Rovito SM, Soto-Azat C, Toledo LF, Voyles J, Weldon C, Whitfield SM, Wilkinson M, Zamudio KR, Canessa S (2019) Amphibian fungal panzootic causes catastrophic and ongoing loss of biodiversity. Science 363, 1459-1463.
| Crossref | Google Scholar | PubMed |
Sergeant ESG (2018) Epitools epidemiological calculators. Ausvet. Available at http://epitools.ausvet.com.au [accessed 3 March 2020]
Smith ID, Munday BL (1965) Observations on the incidence of Toxoplasma gondii in native and introduced feral fauna in eastern Australia. Australian Veterinary Journal 41, 285-286.
| Crossref | Google Scholar |
Taggart PL, Fancourt BA, Fabijan J, Peacock DE, Speight KN, Caraguel CGB, McAllister MM (2019) No evidence of Toxoplasma gondii exposure in South Australian koalas (Phascolarctos cinereus). The Journal of Parasitology 105, 638-641.
| Google Scholar |
Tann CR, Singleton GR, Coman BJ (1991) Diet of the House Mouse, Mus domesticus, in the Mallee Wheatlands of north-western Victoria. Wildlife Research 18, 1-12.
| Crossref | Google Scholar |
Tasker EM, Dickman CR (2001) A review of Elliott trapping methods for small mammals in Australia. Australian Mammalogy 23, 77-87.
| Crossref | Google Scholar |
Travouillon KJ, Phillips MJ (2018) Total evidence analysis of the phylogenetic relationships of bandicoots and bilbies (Marsupialia: Peramelemorphia): reassessment of two species and description of a new species. Zootaxa 4378, 224-256.
| Crossref | Google Scholar |
Valentine LE, Anderson H, Hardy GESJ, Fleming PA (2012) Foraging activity by the southern brown bandicoot (Isoodon obesulus) as a mechanism for soil turnover. Australian Journal of Zoology 60, 419-423.
| Crossref | Google Scholar |