Evaluating aerial net gunning and chemical immobilisation for capture of invasive sambar deer (Rusa unicolor) and red deer (Cervus elaphus) in alpine Australia
Eliane D. McCarthy


A
B
C
D
E
Abstract
Deer species are expanding in distribution and abundance in Australia. There is increasing focus on the ecology of these species, but effective deer capture methods are needed for telemetry studies.
The aims of this study were to develop, assess and refine a helicopter-based capture technique for wild sambar deer (Rusa unicolor) and red deer (Cervus elaphus), based on trials in south-eastern Australia.
We captured and collared 14 sambar deer and five red deer in two operations in Kosciuszko National Park in 2021, using a combination of aerial net gunning and chemical immobilisation delivered via hand injection. Captured animals were fitted with GPS collars to track their movements and activity post-capture. Physiological measurements were recorded as a means of assessing responses to capture and to optimise animal welfare outcomes.
Twenty-two deer were pursued and captured, with a total mortality rate of 14% (n = 3). The frequency of mortalities decreased from the first operation (17%) to the second operation (10%), largely attributed to allowable helicopter pursuit times being reduced. Post-release movement data indicated that delayed mortality due to capture did not occur. Activity of collared deer was lowest immediately following collaring, suggesting the procedure caused short-term decreases in deer activity.
Aerial capture of wild sambar deer and red deer poses animal welfare risks, but these can be minimised through the refinement in net gunning and pharmacological procedures to minimise animal stress.
This study describes an effective method for the safe capture of sambar deer and red deer, which will assist future wildlife researchers to further refine helicopter-based capture protocols and to collect spatial ecology and survival information about these species.
Keywords: animal welfare, collaring, immobilisation, invasive species, mortality, net gun, satellite telemetry, ungulates.
Introduction
Invasive species threaten biodiversity globally, and understanding their ecology is imperative to reducing their impacts (Vilà et al. 2011; Doherty and Ritchie 2017). In their native ranges, deer can affect forest composition (Russell et al. 2017), and high deer densities have been shown to adversely affect tree recruitment and forest regeneration (Borowski et al. 2021). In Australia, deer were first introduced in the 1800s, with releases common for hunting, and their subsequent spread has led to the establishment of wild populations in a range of ecosystems across all Australian states and mainland territories (Davis et al. 2016). Six deer species have now established in Australia, and some of these species have supplanted native herbivores as the dominant mammalian herbivores in a few ecosystems (Hartley et al. 2022). In Australia, deer have been associated with native vegetation loss and soil erosion (Ward-Jones et al. 2019), have the potential to transmit diseases to livestock (Cripps et al. 2019) and can negatively impact public health (e.g. through vehicle collisions and pollution of drinking water) (Hampton and Davis 2020). Climate-matching analyses show that Australia’s two largest deer species, sambar deer (Rusa unicolor), native to southern Asia (Timmins et al. 2015), and red deer (Cervus elaphus), native to Europe and parts of central and western Asia (Lovari et al. 2018), have the potential to extend their distribution across much of Australia (Davis et al. 2016). In Kosciuszko National Park, where deer and other exotic herbivores are overabundant, they have damaging impacts on biodiversity (Ward-Jones et al. 2019; Hartley et al. 2022). Australia’s invasive deer populations may continue to increase in geographic distribution and total population size unless effective population control measures are undertaken (Moriarty 2004; Gormley et al. 2011; Burgin et al. 2015).
Telemetry studies offer a means of studying wild animals, including deer, that exhibit predator avoidance towards humans (Frid and Dill 2002; Stankowich 2008) and are therefore difficult to monitor through direct observation. Tracking animal movement allows researchers to gain insights into animal life survival, reproductive output, behaviour, habitat preferences, home range, dispersal, exploratory movements and responses to environmental change (Kays et al. 2015). This movement data can provide information on target species mobility and rate of population spread (Alford et al. 2009), and can be used to prioritise areas for control by aiding in the identification of areas of preferential habitat utilisation (Moseby et al. 2021). During control operations, tracked individuals can also function as Judas animals, giving away the location of their conspecifics (Moseby et al. 2021), or be monitored for their responses to culling (Spitz et al. 2019), informing future management efforts. Little is known about sambar deer or red deer movement and the habitat preferences they exhibit in alpine Australia (Davis et al. 2016; Hampton et al. 2019a). Capturing these large deer (sambar deer males ~ 300 kg (English 1988) and red deer males ~ 180 kg (Searle 1981)) is logistically complex and can result in unfavorable animal welfare outcomes if not undertaken carefully (Hampton et al. 2021).
There are very few published methods for the capture of sambar deer. Other species of cervid have been captured using physical restraint (e.g. trapping or netting) (Shury 2014), chemical restraint (e.g. darting or ‘remote chemical immobilisation’) (Kreeger and Arnemo 2018) or a combination of the two approaches (Hampton et al. 2019a). Red deer have been captured using both physical and chemical restraint across their native and introduced ranges (e.g. Roberts 2012; Amos et al. 2014; Becciolini et al. 2019; Latham et al. 2020). Physical restraint techniques have included helicopter net gunning and ground-based nets and box traps (Marco and Lavín 1999). Chemical restraint techniques have included helicopter (e.g. Latham et al. 2020) and ground-based darting (Arnemo et al. 1994; Amos et al. 2014). Helicopter net gunning has been used for red deer capture for several decades in New Zealand and was used in the 1980s in Queensland, Australia (Porter 1986). We are not aware of aerial support to capture sambar deer, although in India, six sambar deer were chemically immobilised from the ground (Chatterjee et al. 2014) and in Taiwan, 12 sambar deer were captured using a baited ground net and remote chemical immobilisation delivered via blowpipe (Yen et al. 2019). In Australia, two captive sambar deer have been captured via ground-based darting (Moore 1994). A recent review identified methods for the safe and reliable capture of sambar deer as a knowledge gap in Australian deer management (Hampton et al. 2019a).
Helicopter net gunning is a procedure whereby animals are entangled and captured in a weighted net fired from a gun, out of a helicopter (Yerex 2001). Ground- and helicopter-based net gunning is commonly used to capture deer (Flueck et al. 2005; Van de Kerk et al. 2020), including in Australia, where the methods have previously been used to capture red deer (Porter 1986) and fallow deer (Dama dama) (Bengsen et al. 2021) from a helicopter, and trialled unsuccessfully for capturing hog deer (Axis porcinus) from the ground (Mayze and Moore 1990). Mortality rates resulting from net gun capture of ungulates have been shown to generally be lower than for other capture methods. For example, in one study, helicopter net gunning mortality rates were lower (0–2%) in white-tailed deer (Odocoileus virginianus) than for other methods (0–7% for drive nets and 1–21% for Clover traps) (Peterson et al. 2003). A recent study captured 27 fallow deer using a net gun from a helicopter in north-eastern New South Wales, Australia, and reported zero mortalities and no observable effects of capture on the longer-term movement and activity of collared animals (Bengsen et al. 2021). However, other studies report deer sustaining injuries or dying as result of net gun capture. In one study targeting white-tailed deer, 8.4% of animals sustained injuries as a result of net-gunning (Webb et al. 2008), and in another study, 1.4% of animals died as a result of capture (Jacques et al. 2009). Helicopter net gunning is sometimes augmented by chemical restraint that may be delivered via hand injection (Ortega et al. 2020) or intranasal administration (Cattet et al. 2004). Chemical restraint is used in this way in an attempt to reduce anxiety and fear and physiological abnormalities such as hyperthermia (Thompson et al. 2020). However, use of chemical restraint is also associated with some negatives, such as the possibility of drug-induced lethargy (Ortega et al. 2020).
Reporting on the animal welfare effects of capture methods and immobilisation techniques enables researchers to identify best practice techniques and refine future practices (McMahon et al. 2012; Hampton et al. 2021). Animal capture is a high-risk activity in wildlife research that frequently leads to animal injuries and mortalities (and injuries to human operators) (Kreeger et al. 1990; Webb et al. 2008; Latham et al. 2020; Hampton and Arnemo 2022). Mortalities are commonly seen during cervid capture due to processes such as ballistic trauma, hyperthermia and capture myopathy (Beringer et al. 1996; Van de Kerk et al. 2020). In addition, in the days following capture, animals can exhibit changes in movement rates (Cattet et al. 2008; Northrup et al. 2014). It is important that wildlife practitioners aim to minimise impacts on animals, because procedures that cause adverse effects not only raise ethical concerns, but also influence animal behaviour, affecting research results.
Herein we describe the outcomes of a helicopter net gunning operation attempting to capture sambar deer and red deer across two operational periods in April 2021 (operation one), where the method was trialled, and November 2021 (operation two), where a refined method was deployed, in the mountainous sub-alpine area of Kosciuszko National Park, south-eastern New South Wales. The capture method used a combination of helicopter capture and chemical immobilisation (Hampton et al. 2019a). Captured animals were fitted with GPS collars to track their movements and activity post-capture. Here, we focus specifically on the animal welfare outcomes during capture and use the short-term post-release movement and activity data to assess behavioural responses of collared deer and mortality. This study validates our method for the safe capture of sambar deer and red deer.
Materials and methods
Study site
Aerial net gunning operations took place in the southern section of Kosciuszko National Park (36.54°S, 148.35°E), spanning 186 km2 in southeastern New South Wales, Australia (Fig. 1). Operations took place over 11 days, when weather conditions were favourable (wind <15 km h−1 and no rain), within two 2-week periods in April (autumn) and November (spring) 2021. The capture area is within Australia’s Snowy Mountains region, and elevation ranges from 1150 to 1917 m above sea level (NASA/METI/AIST/Japan Spacesystems and US/Japan ASTER Science Team 2019). The region is mountainous, and 32.48% of the area has a slope of 18° or greater (NSW Department of Planning and Environment 2021). Vegetation in the capture area is dominated by eucalypt woodlands with tussock grass, fern and shrubby understoreys, and wet open tussock grasslands (Australian Government Department of Climate Change, Energy, the Environment and Water 2021). The capture area has extensive creek and river systems with multiple tributaries (Fig. 1). Temperature from the nearest weather station (~3 km from northern boundary of capture area), measured at 0900 and 1500 hours on operational days, ranged from −2.4 to 10.6°C in operation one and from 4.6 to 12.4°C in operation two (Bureau of Meteorology 2020). Sambar deer, fallow deer and red deer were present within the capture area, and overall deer population density was estimated within 3 weeks of both aerial net gunning operations using distance sampling, via aerial thermal imaging surveys that took place across a larger area (279 km2) of the southern section of Kosciuszko National Park, including the capture area. The first thermal survey took place during 1−3 April 2021, when deer density was estimated at 15.23 ± 4.00 deer km−2 (18 days prior to commencement of operations), and the second thermal survey took place during 16−17 November 2021, when deer density was estimated at 11.08 ± 2.99 deer km−2 (5 days after completion of operations; E. O’Dwyer-Hall, unpubl. data).
Map of capture area, targeting sambar deer (Rusa unicolor) and red deer (Cervus elaphus), in Kosciuszko National Park, Australia. Yellow bounding box indicates capture area, yellow points indicate locations of successfully captured deer and red points indicate locations of deer mortalities during capture. Watercourses are shown on map in blue (major) and green (minor). The location of the capture area relative to Australia is indicated by the frame in the top left corner. Image sources: Esri, Maxar, Earthstar Geographics.
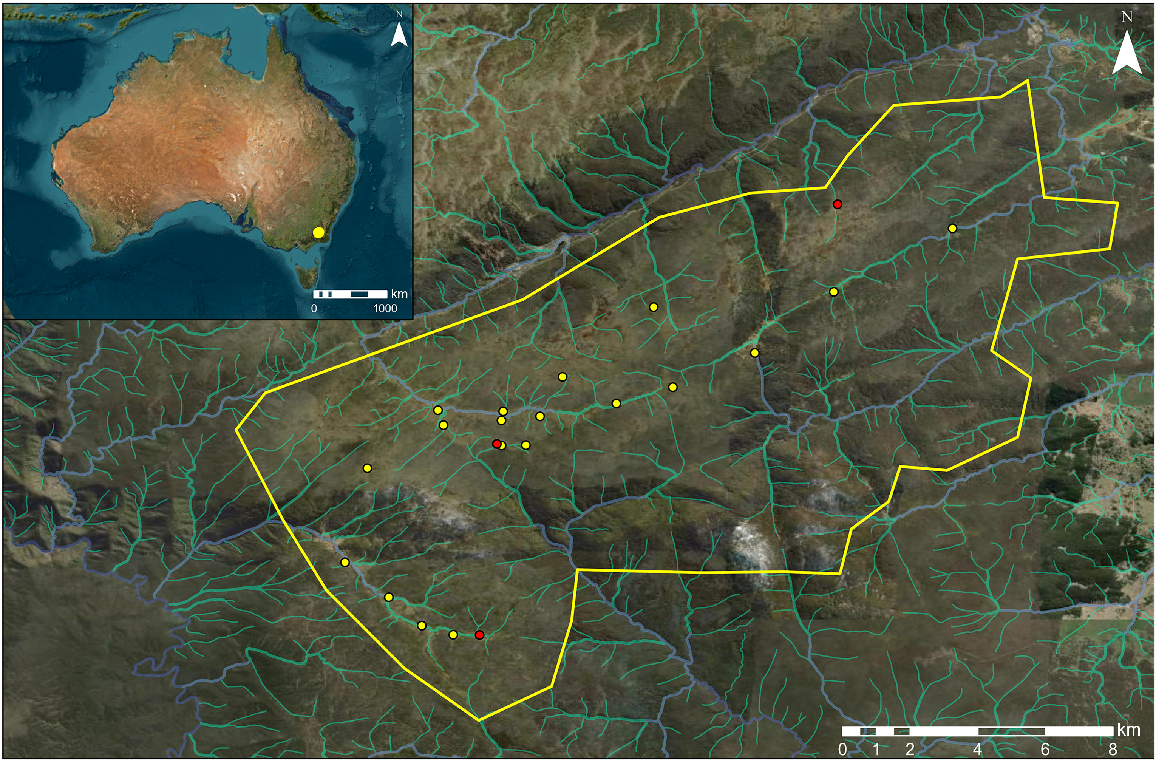
Field trials
To ensure safe and effective operations, the net gun was deployed in a series of ground and aerial trials to ensure even spread of the net at operation heights 5–10 m above ground level. Running shots were also trialled on a static target to assist pilot and net gun operator to identify operational requirements prior to attempting capture of live targets.
Equipment and procedure
A Eurocopter AS350 B3 Squirrel helicopter, crewed with pilot, navigator and net gun operator (NSW National Parks and Wildlife Service Feral Animal Aerial Shoot Team trained shooter) was used to search the area for deer. Once a deer was sighted, the pursuit phase began, categorised into two parts. Part one involved directing the deer toward open and reasonably level ground, with an emphasis on maintaining a low level of stress and physical exertion on the deer. Part two involved a more rapid pursuit to approach within firing range and net deployment. Part one of the pursuit was limited to 10 min; however, for deer moving uphill, this was reduced to 5 min to reduce the likelihood of fatigue. Once the deer was in a sparsely vegetated flat area suitable for net gunning, part two commenced, which was a maximum of 5-min high intensity chase. During this time, the helicopter flew at a low level (2–5 m above ground level) and speed (≤45 km h−1). Times for each phase of the pursuit were recorded (approximate times were recorded in operation one) (Supplementary Table S1) and ceased if the deer was observed to be panting or running unsteadily or the maximum pursuit times reached. A .308 breakaway net gun (ACE Capture, Invercargill, New Zealand) loaded with .308 blanks (22 grain, Australian Munitions ADI AP70N gunpowder) launched four 186-g weights through four barrels angled to spread a 170 × 170 mm (April) or 280 × 280 mm (November) aperture net when fired downward at heights of <5 m (Fig. S1). Following an initial shot, additional nets were fired if deemed necessary by the shooter, based on animal size, to further restrain the animal (Table S1).
Following capture, the catch team helicopter landed >100 m from the deer and proceeded quietly towards it. The deer’s head was covered with a blanket and a blindfold was fitted if safe to do so. In operation two, an additional net was draped over entangled deer to provide an additional level of containment. Following this, a second helicopter with a pilot, veterinarian, veterinary nurse and collar technician landed nearby to the catch team helicopter. Morphometric measurements (body length) were taken from recumbent sambar deer to allow estimation of body mass. Length−weight relationships were developed from adult male and female sambar deer shot and weighed in Victoria in 2012 (Table S1; Bartareau 2019; Watter et al. 2020; Hampton et al. 2021). We did not have access to equivalent data for red deer, so body mass estimates were based on published estimates for adult male and female red deer (Amos 2015). Final estimated mass and drug doses were adapted upon visual inspection of each deer by the veterinarian (Tables 1 and S1). The veterinarian immobilised animals via intramuscular hand injection into the hindquarters. Sambar deer and red deer received medetomidine (40 mg/mL; prepared by a commercial compounding pharmacy; Bova Compounding, Caringbah, NSW, Australia) and tiletamine-zolazepam (Zoletil®; Virbac, Milperra, NSW, Australia). Medetomidine was given to all deer (~0.1 mg kg−1 in operations one and two), and sambar deer also received tiletamine-zolazepam (~1.0–5.0 mg kg−1; Tables 1 and S1). With the exception of one animal, red deer did not receive tiletamine−zolazepam. Two considerations informed the rationale for the different approaches taken to immobilisation of sambar deer versus red deer. Studies have shown that net-gunned red deer can be safely handled without any chemical restraint (Latham et al. 2020), and that red deer captured via other methods can be safely handled with relatively low immobilisation doses (Wolkers et al. 1994). For sambar deer, however, there is little known about effective capture methods (Hampton et al. 2019a). In addition, owing to the much larger size of sambar deer, the risk of injury to staff was deemed to be much higher for incompletely immobilised animals (Hampton et al. 2019a). The tiletamine−zolazepam dose used was reduced between operations one (~2.5 mg kg−1) and two (~1.0 mg kg−1) (Table 1), in order to reduce recovery times in operation two. Following drug administration, each deer was observed until completely immobilised (recumbency); in some cases, re-administration of medetomidine and/or tiletamine−zolazepam was required if deer were incompletely immobilised (see Table S1). The induction time, the duration between the administration of the drugs and immobilisation being achieved, was recorded for each deer (Table 2; Hampton et al. 2021).
Collar ID | Species | Sex | Body mass (kg) | Drug doses (mg/kg) | Physiological variables (mean) | Immobilisation quality score A | ||||||
---|---|---|---|---|---|---|---|---|---|---|---|---|
Medetomidine | Tiletamine− zolazepam | Atipamezole | HR (bpm) | RR (rpm) | SpO2 (%) | Rectal temperature (°C) | ||||||
11 | Sambar | Female | 163 | 0.22 | 5.20 | 0.77 | 70 | 42 | 93 | 37.9 | 3.9 | |
7 | Sambar | Male | 190 | 0.16 | 5.25 | 0.92 | 70 | 40 | 83 | 39.6 | 4.0 | |
13 | Sambar | Female | 159 | 0.13 | 4.72 | 0.79 | 77 | 23 | 86 | 39.1 | 4.0 | |
12 | Sambar | Female | 143 | 0.14 | 7.01 | 1.58 | 85 | 41 | 84 | 38.5 | 4.0 | |
5 | Red | Female | 150 B | 0.11 | 2.50 | 0.57 | 56 | 44 | 92 | 41.0 | 4.0 | |
9 | Sambar | Male | 202 | 0.18 | 3.09 | 0.62 | 80 | 42 | 90 | 39.6 | 4.0 | |
10 | Red | Male | 180 B | 0.10 | NA | 0.25 | NR | 32 | 86 | 41.4 | 3.5 | |
15 | Red | Female | 150 B | 0.11 | NA | 0.30 | 75 | 12 | 89 | 39.3 | 4.0 | |
6 | Sambar | Male | 160 | 0.12 | 4.69 | 0.69 | 78 | 32 | 83 | 38.3 | 4.0 | |
8 | Sambar | Male | 228 | 0.11 | 5.49 | 0.66 | 65 | 35 | 81 | 38.8 | 4.0 | |
37 | Red | Female | 160 B | 0.11 | NA | 0.47 | 66 | 33 | 89 | 39.7 | 4.0 | |
40 | Red | Male | 180 B | 0.11 | NA | 0.28 | 77 | 25 | 91 | 40.2 | 4.0 | |
43 | Sambar | Male | 181 | 0.12 | 1.10 | 0.56 | 77 | 37 | 87 | 39.4 | 4.0 | |
35 | Sambar | Female | 135 | 0.14 | 1.33 | 0.65 | 80 | 37 | 91 | 39.8 | 4.0 | |
48 | Sambar | Male | 193 | 0.13 | 1.09 | 0.59 | 83 | 34 | 93 | 39.5 | 3.0 | |
49 | Sambar | Male | 211 | 0.10 | 1.18 | 0.59 | 85 | 30 | 95 | 35.3 | 4.0 | |
41 | Sambar | Female | 138 | 0.18 | 1.45 | 0.82 | 85 | 35 | 92 | 38.5 | 2.0 | |
47 | Sambar | Male | 195 | 0.10 | 0.92 | 0.58 | 84 | 40 | 86 | 38.5 | 4.0 | |
46 | Sambar | Male | 237 | 0.11 | 1.06 | 0.58 | 91 | 30 | 89 | 38.1 | 4.0 |
For sambar deer, body mass estimates used are derived from the body mass regression relationship provided in Supplementary Material S1.
NR, not recorded.
Capture metrics | Operation 1 | Operation 2 | |||
---|---|---|---|---|---|
Sambar (n = 42) | Red (n = 9) | Sambar (n = 26) | Red (n = 7) | ||
Proportion: collaring attempts successful | 0.17 (0.07–0.31) | 0.33 (0.07–0.70) | 0.27 (0.12–0.48) | 0.29 (0.04–0.71) | |
Proportion: shots missed | 0.29 (0.17–0.44) | 0.11 (0.02–0.44) | 0.00 (0.00–0.13) | 0.00 (0.00–0.35) | |
Proportion: targeted deer that were abandoned A | 0.52 (0.38–0.67) | 0.44 (0.19–0.73) | 0.69 (0.50–0.84) | 0.71 (0.36–0.92) | |
Proportion: mortality at time of capture for captured deer | 0.12 (0.00–0.53) | 0.25 (0.01–0.81) | 0.12 (0.00–0.53) | 0.00 (0.00–0.84) | |
Proportion: mortality post-capture | 0.00 (0.00–0.41) | 0.00 (0.00–0.71) | 0.00 (0.00–0.41) | 0.00 (0.00–0.84) | |
Proportion: traumatic injuries at time of capture | 0.43 (0.10–0.82) | 0.00 (0.00–0.71) | 0.57 (0.18–0.90) | 1.00 (0.16–1.00) | |
Proportion: hyperthermia | 0.00 (0.00–0.41) | 0.67 (0.09–0.99) | 0.00 (0.00–0.41) | 0.50 (0.01–0.99) | |
Proportion: prolonged recovery time | 0.86 (0.42–1.00) | 0.33 (0.01–0.91) | 0.00 (0.00–0.41) | 0.50 (0.01–0.99) | |
Mean induction time (min) | 19.00 (4.00–34.00) | 4.00 (0.00–9.00) | 10.00 (6.00–14.00) | 4.00 (0.00–13.00) | |
Mean processing time (min) | 28.00 (16.00–40.00) | 17.00 (12.00–21.00) | 26.00 (23.00–29.00) | 22.00 (22.00–22.00) | |
Mean recovery time (min) | 47.00 (25.00–69.00) | 44.00 (0.00–181.00) | 10.00 (8.00–13.00) | 11.00 (0.00–74.00) |
Once drugs were administered and the deer was immobilised, the blanket was removed and a blindfold was attached, allowing the capture and veterinary team to untangle and remove the nets from the animal. Hobbles were applied to front and back legs, and personnel were protected from antlers by applying antler covers (sections of ‘pool noodles’) to antler tips and/or shielding antlers using an antler protection board (see Fig. S1). The deer was repositioned in sternal recumbency with the pharynx higher than the rumen, nose downhill and tongue extended, to reduce the chances of regurgitation of ruminal fluid and aspiration of fluid to the lungs. The animal’s rectal temperature, heart rate, respiratory rate and oxygen saturation (Mindray PM-60 Vet Pulse Oximeter) were recorded every 5 min until medetomidine was antagonised via administration of the reversal agent atipamezole (0.1 mg kg−1) (see Table S1). A sterile eye lubricant was applied, and any injuries were recorded. If oxygen saturation fell below 90%, intranasal oxygen was administered. This was delivered via a size C medical oxygen bottle with an Allied Healthcare Products B&F oxygen pressure regulator in operation one, and an INHALO® medical oxygen cylinder (CD size) with an integrated oxygen regulator in operation two. Oxygen was administered at a flow rate of 1–2 L min−1 and was increased to 5–10 L min−1 if oxygen saturation fell below 85%. If oxygen saturation fell below 80%, animals were immediately given a third of a dose of the reversal agent (see below) by intramuscular injection. If the rectal temperature of a deer fell below 38.0°C (i.e. hypothermia), the deer would be warmed using blankets, or above 40°C (i.e. hyperthermia), the deer would be cooled using water spray and fanning (Hampton et al. 2022). If respiration rate fell to <20 breaths min−1, a third of a dose of atipamezole was administered. Once inspection had determined that the deer was in good physical condition with no significant injuries (i.e. those that would inhibit normal movement), several morphometric measurements were recorded: length from nose to the base of tail (cm); neck circumference (cm at mid neck); and full girth (cm). The veterinarian provided a qualitative immobilisation quality score for each deer (Table S2; Harms et al. 2018).
A GPS tracking collar with inbuilt mortality sensor, programmed to record animal locations hourly, was then fitted (G52D Iridium, Advanced Telemetry Systems, Isanti, MN, USA). Each collar was fitted with an accelerometer, which estimated the proportion of time each collar or deer was moving between hourly fixes. Chloromide® antiseptic spray (Troy Animal Healthcare, Glendenning, NSW, Australia) was applied to any wounds. The processing time, the time between administration of the immobilising dose and the first reversal dose, was recorded for each deer (Table S1). Once all procedures were finalised, medetomidine was reversed with atipamezole ~0.3 mg kg−1 by intramuscular injection. Some deer were given further doses of atipamezole if they did not show signs of recovering within ~15 min of the administration of a first dose (Table S1). Each of these cases were designated as a ‘prolonged recovery’.
Immediately after administration of atipamezole, hobbles were removed, the blindfold was replaced with a small cloth, and catch and veterinarian teams (excluding the veterinarian) moved to >20 m from the deer to allow it a clear path of escape upon recovery. The veterinarian remained to support and monitor the deer in sternal recumbency. When the veterinarian had assessed that the deer had recovered sufficiently, the blindfold cloth and antler protection were removed, and the veterinarian moved slowly away from the recovering deer. A firearm was available to enable immediate euthanasia to be performed should a severe adverse animal welfare event (e.g. a broken leg) result from an unsteady recovery (Hampton and Arnemo 2022). The recovery time was also recorded for each deer – the time from the first reversal dose to the time the deer was first standing (Table S1). This study was approved by The University of Sydney Animal Ethics Committee (Project number: 2020/1844).
Post-release monitoring
To measure movement and mortality for 45 days post-capture, we used generalised additive models (GAMs). Previous studies evaluating post-release welfare and movement of captured deer have monitored deer movement for 30 days post-capture for mule (Odocoileus hemionus) and fallow deer (Northrup et al. 2014; Bengsen et al. 2021); 50 days for roe deer (Capreolus capreolus) (Morellet et al. 2009) and 45 days for red deer (Becciolini et al. 2019).
Data were remotely downloaded for all collars deployed on sambar deer (14) and red deer (five) after 45 days. Mean hourly movement and activity were calculated for each deer for 1–45 days following collaring. Day 1 consisted of hourly data from the first 24 h following collaring for each deer. Days 2–45 commenced at the same time each day for each deer, depending on their time of collaring on day 1.
For each deer, accelerometry data was used to calculate mean proportion of time spent active per day for days 1–45 following collaring. Daily mean Euclidean distance between hourly location fixes from 1 to 45 days following collaring was also calculated. Then, GAMs were used to assess changes in movement and activity over the monitoring period, because the collared deer were predicted to have non-linear responses over time (Becciolini et al. 2019; Bengsen et al. 2021).
To examine trends in deer activity in the days following collaring, four GAMs were fitted, modelling changes in activity separately for males and females of the two species. The models were fitted with mean daily activity (proportion of time spent active) as the response variable, days since capture as the smooth term and operation as an explanatory variable and multiplier in the smoothed term. Separate smooth functions were generated to model activity 1–45 days since capture for operations one and two. Models were fitted with a binomial distribution in mgcv; however, underdispersion was detected in the binomially distributed models, which was corrected using a quasi-GAM model (Zuur et al. 2009).
To examine changes in deer movement in the days following collaring, four GAMs were fitted, as above, with mean Euclidean distance between hourly location fixes, averaged daily, as the response variable, days since capture as the smooth term and operation as an explanatory variable and multiplier in the smoothed term. Again, separate smooth functions were generated to model mean distance between hourly location fixes, averaged daily, for the two operation periods. The response variable underwent logarithmic transformation to improve normality of the residuals. GAMs were fitted with Gaussian distributions, restricted maximum likelihood and thin plate regression spline smoothers. All data wrangling and analyses were performed in R ver. 4.1.2, interfaced through RStudio 2021.09.1. Data visualisations were generated using ggplot2 and visreg packages.
For capture outcomes and animal welfare metrics, we report descriptive statistics (means ± s.d. and 95% confidence intervals [CIs]) to avoid performing multiple post hoc statistical tests with low power (Hampton et al. 2019b; Hampton et al. 2021). Ninety-five percent CIs were calculated using the Clopper–Pearson exact method to account for many cases in which sample sizes were small (n < 10) and data did not follow a normal distribution.
Results
Over the 11 days of aerial net gunning operations, totals of 14 sambar deer (nine males and five females, all adult except for one yearling male) and five red deer (two males, three females, all adults) were captured and collared. A single net each was deployed on nine deer (five sambar deer, four red deer), two nets each were deployed on eight deer (seven sambar deer, one red deer) and three nets each were deployed on two deer (both sambar deer; Table S1).
Based on numbers of successful captures compared with total capture attempts (Table 2), the probability of a targeted deer being successfully collared (i.e. captured and not dying) across both operation periods was 0.21 (95% CI = 0.12–0.32) for sambar deer and 0.31 (95% CI = 0.11–0.59) for red deer. The probability of mortality for captured deer was 0.12 (95% CI = 0.016–0.38) for sambar deer and 0.17 (95% CI = 0.0042–0.64) for red deer. All missed shots (n = 13), where a net was fired but failed to capture a deer, occurred during operation one (Table 2). Therefore, the probability of successfully capturing a sighted deer increased from 0.20 in operation one to 0.27 for operation two. Failed netting attempts during the first operation were largely due to firing among vegetation, but in operation two, nets were not fired if deer were <10 m from heavy vegetation and larger aperture nets (280 × 280 mm) were available for stags. Mean calculated weight for male sambar deer was 199.6 ± 22.0 kg and for female sambar deer was 147.7 ± 11.4 kg. Mean estimated weight for male red deer was 180 ± 0 kg and for female red deer was 153.3 ± 4.7 kg.
Refinement of procedures between operations one and two led to improvements in most (but not all) animal welfare metrics (Table 2). Of the 19 deer collared, two sambar deer (one in operation one and one in operation two) required a second administration of immobilisation drugs in order to achieve the desired plane of immobilisation, and one sambar deer in operation one required three administrations. All other deer (n = 16; 84%) were satisfactorily immobilised with a single injection. This trend contributed to a considerable reduction in mean induction time for sambar deer between operation one and two (Table 2). There was also improvement in recoveries: of the 19 deer captured and collared, six sambar deer and two red deer required two reversal doses. All other deer (n = 11; 58%) were satisfactorily reversed from a single atipamezole injection, and the frequency of prolonged recovery declined between operations one and two (Table 2). The was achieved largely through refinement of drug doses: higher initial doses of atipamezole were given to both species in operation two (mean doses of 0.56 mg kg−1 for sambar deer and 0.28 mg kg−1 for red deer) when compared with operation one (mean doses of 0.18 mg kg−1 for sambar deer and 0.22 mg kg−1 for red deer) (Table 1). This improvement was also reflected in recovery times: in operation one, the mean recovery time for sambar deer and red deer was 47 and 44 min, respectively; in operation two, this was reduced to 10 min and 11 min, respectively (Table 2). Chemical immobilisation quality was similar for all operational stages and species, with mean values of 4.0 and 3.8 for sambar deer and red deer, respectively, in operation one and 3.6 and 4.0 for sambar deer and red deer, respectively, in operation two.
Mortalities and injuries
Three mortalities occurred in total: two in operation one and one in operation two. The two mortalities that occurred in operation one (one sambar deer male, one red male) arose from an unknown acute cause while the animals were being pursued, with no definitive diagnosis made. As a result of these mortalities, and the presumed role that stress imposed by pursuit played in them, the maximum allowed time for phase one was reduced from 10 min to 5 min for operation two. In operation two, one sambar deer died at the time of capture when it became entangled in the capture net, fell into a small water body (a pond) and drowned. The yearling male was dragged from the pond and resuscitation was attempted by the navigator for 60 s with no response. Following this incident, aerial net gunning was not attempted on any deer <100 m from a water body. Five male deer (four sambar deer, one red deer) sustained an antler injury during capture, and five deer (four sambar deer, one red deer) bit their tongue during capture and sustained a superficial tongue laceration (Table S1). No other injuries were observed (Table 2).
Post-release movement and activity
For all deer groups, the mean daily activity (proportion of time spent active) tended to be lowest immediately following collaring. For red deer males, mean activity increased rapidly between days 1 and 10, before declining slightly, and then continuing to increase (Fig. 2). For red deer, mean daily activity varied from 0.10 (day 1) to 0.57 (day 38) for males and 0.22 (day 2) and 0.43 (day 35) for females. For sambar deer, mean daily activity varied from 0.23 (day 3) to 0.44 (day 27) for males and 0.21 (day 1) and 0.46 (day 33) for females. GAM results showed that mean daily activity for both deer species varied significantly over time for all models (red males: F = 20.55, P < 0.001, red females: F = 14.00, P < 0.001, sambar deer males: F = 15.90, P < 0.001, sambar deer females: F = 7.84, P < 0.001; Supplementary Material S2). Mean activity in the 45 days following collaring varied significantly between operations one and two for male and female sambar deer and red female deer, with these groups showing higher levels of activity in operation two compared with operation one. Red male deer activity did not vary significantly between the periods (red males: t = −0.44, P = 0.662, red females: t = 15.06, P < 0.001, sambar deer males: t = 18.98, P < 0.001, sambar deer females: t = 5.29, P < 0.001; Supplementary Material: S2).
GAM plots showing daily mean proportion of time active for 14 sambar deer (Rusa unicolor; nine males, five females) and five red deer (Cervus elaphus; two males, three females) for 1–45 days following collaring. For both species, male and females are modelled separately. Red lines represent modelled activity responses for deer collared in operation one (April), and blue lines represent responses from operation two (November). Shaded error bands show 95% confidence intervals.
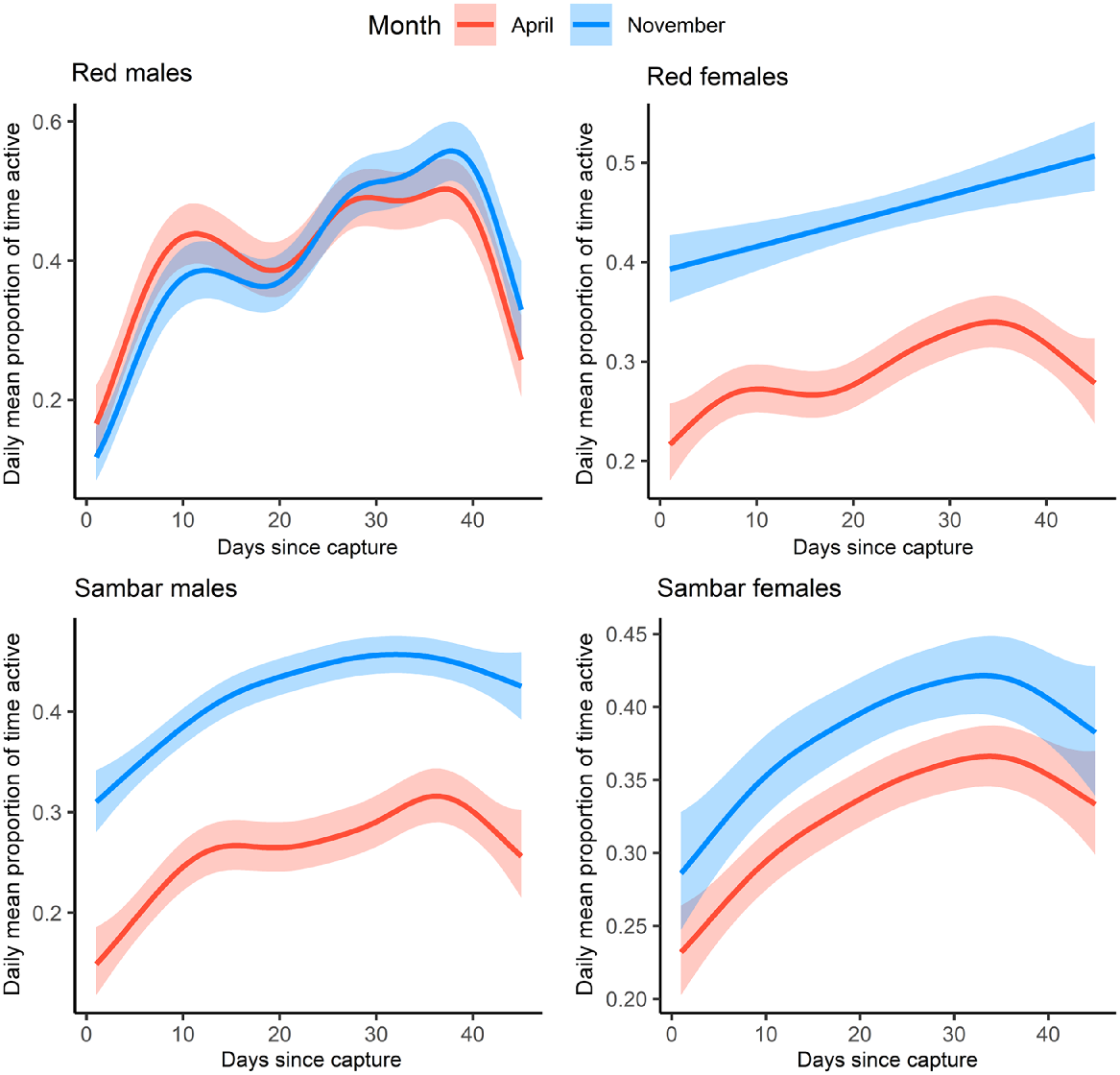
Modelled movement responses showed that for red deer males, mean movement (hourly distance travelled) increased sporadically throughout the monitoring period, but for red deer females, mean movement declined for ~15 days following collaring, before increasing slightly for the remainder of the monitoring period (Fig. 3). For female sambar deer, and male sambar deer collared in operation one, modelled mean movement peaked immediately following collaring, then decreased in the days immediately following collaring, before plateauing around 10 days after collaring, and then remained relatively stable, with smaller positive and negative fluctuations for the remainder of the monitoring period (Fig. 3). This trend was less pronounced for male sambar deer collared in operation two (Fig. 3). These peaks in modelled movement immediately following collaring were due to some sambar deer dispersing large distances in the days following collaring, then remaining within a small area for the remainder of the monitoring period (Figs 4 and S2). Deer mean movement for red deer varied from 19 m per hour (day 4) to 400 m per hour (day 43) for males and 12 m per hour (day 2) to 187 m per hour (day 1) for females. For sambar deer, mean movement varied from 21 m per hour (day 3) to 143 m per hour (day 1) for males and 22 m (day 4) to 118 m per hour (day 1) for females. Mean movement varied significantly over time for female sambar deer, but not for male and female red and male sambar deer (red males: F = 1.40, P = 0.140, red females: F = 1.94, P = 0.109, sambar deer males: F = 1.45, P = 0.240, sambar deer females: F = 3.90, P < 0.001; Supplementary Material: S2). Mean movement in the 45 days following collaring varied significantly between the two collaring periods in all models except the model explaining female sambar deer movement (red males: t = −3.56, P < 0.001, red females: t = 4.42, P < 0.001, sambar deer males: t = 9.39, P < 0.001, sambar deer females: t = 1.59, P < 0.001; Supplementary Material: S2).
GAM plots showing daily mean distance moved per hour for 14 sambar deer (Rusa unicolor; nine males, five females) and five red deer (Cervus elaphus; two males, three females) for 1–45 days following collaring. Sambar deer and red males and females are modelled separately. Red lines represent modelled movement responses for deer collared in operation one (April), and blue lines represent responses from operation two (November). Shaded error bands show 95% confidence intervals.
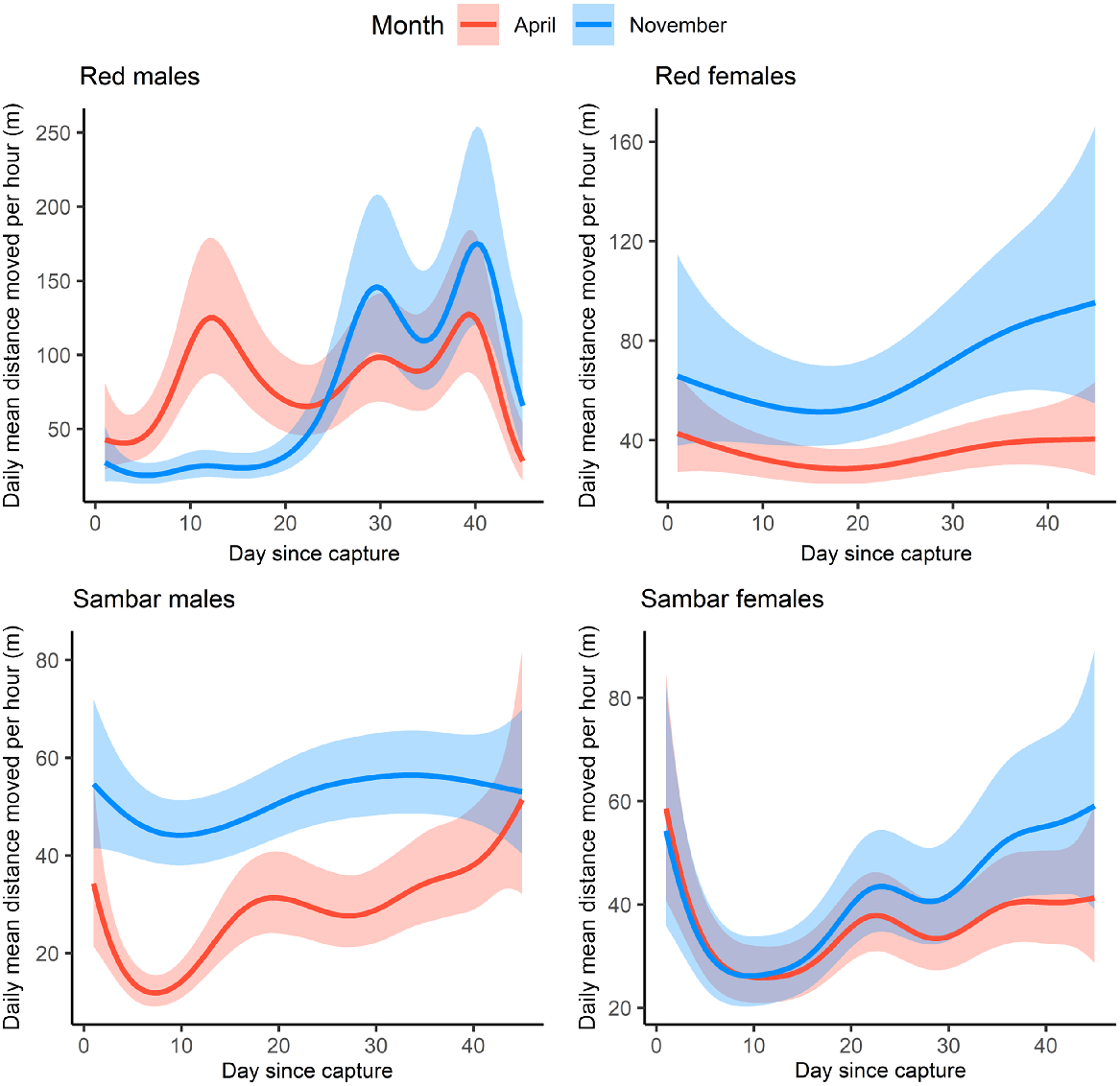
Post-capture movements of sambar deer (Rusa unicolor) and red deer (Cervus elaphus) in alpine Australia. Movement tracks are coloured according to speed (m h−1), measured as Euclidean distance between successive locations recorded hourly. Black points on maps indicate the starting point of each movement path. All movement paths are oriented north.
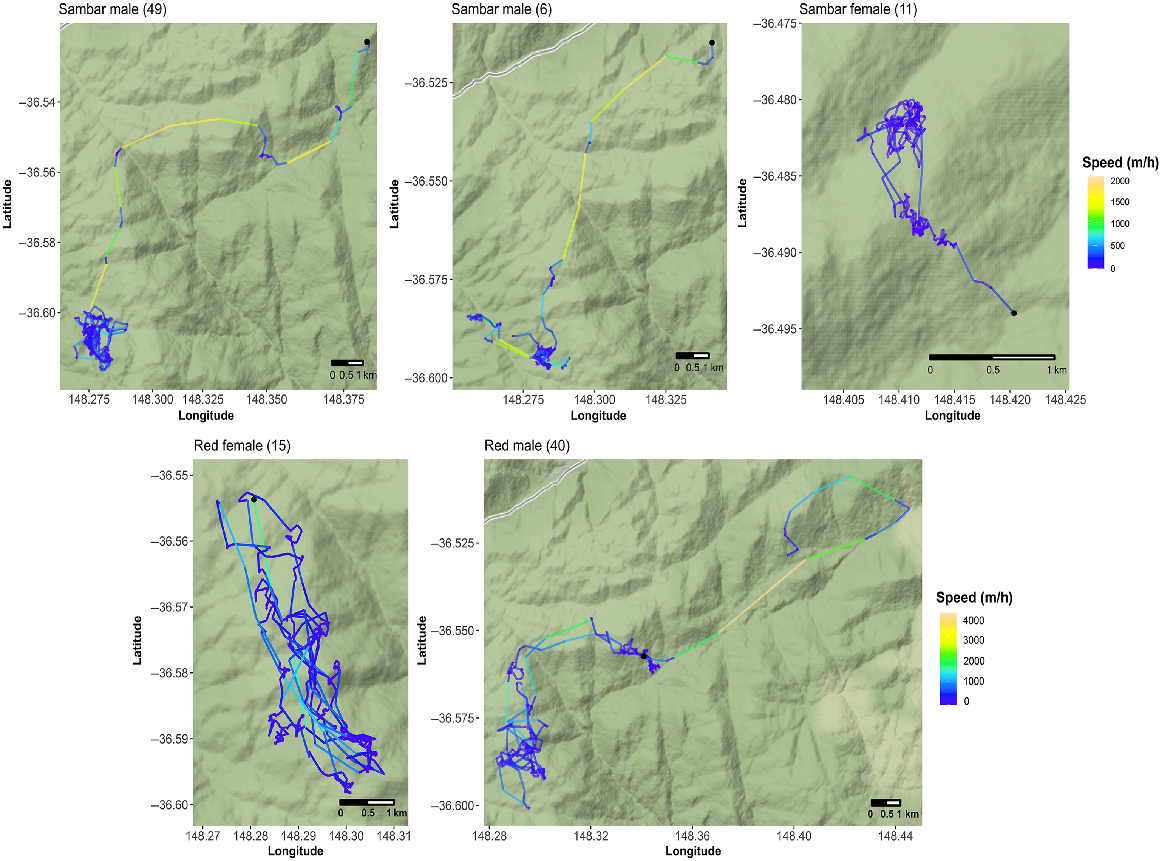
Discussion
Although aerial net gunning and chemical immobilisation have previously been employed for red deer (e.g. Porter 1986; Latham et al. 2020), there are few reported studies employing these techniques (or any other capture methods) for sambar deer (e.g. Chatterjee et al. 2014; Yen et al. 2019). Furthermore, few studies have reported the outcomes of combining net gunning and chemical immobilisation for cervids (Ortega et al. 2020; McNay et al. 2022; Roug et al. 2022). Our results showed that this combination is effective for both sambar and red deer, and although mortalities occurred, we were able to considerably refine methods between our two operations and improve animal welfare outcomes.
Refinement of capture methods yielded reductions in mortalities, induction times and recovery times for our second round of captures. There were no known mortalities at the time of capture resulting from chemical immobilisation, and post-capture, there were no mortalities during the 45-day monitoring period. However, mortality rate at the time of capture across all operations was 14% (Table 2). Collared deer showed acute responses, but these were not sustained beyond the days immediately following collaring (Figs 2 and 3). Activity responses of sambar deer and red deer were lowest immediately following collaring and tended to increase throughout the monitoring period (Fig. 2). Satellite telemetry showed that some deer moved large distances immediately following capture, and following this, movement rates were variable for the remainder of the monitoring period (Fig. 3).
Animal welfare outcomes
The overall probability of mortality during capture was 17% for red deer and 12% for sambar deer (Table 2); however, the small sample size meant that 95% CIs were broad (Table 2; Hampton et al. 2019b). Between operations one and two, however, the capture method was refined and the frequency of injury and mortality during capture was generally reduced (Table 2). In operation one, the mortalities of one sambar deer and one red deer while being chased by the helicopter led us to reduce the maximum time allowed for phase two from 10 to 5 min for operation two, in line with the maximum chase time reported for helicopter net gunning of red deer in a montane environment in New Zealand (Latham et al. 2020). Subsequently, no captured deer died during chases in operation two, although other factors may have been involved in the two deaths (e.g. individual susceptibility). Reductions in maximum allowed chase times have been associated with fewer animal mortalities for helicopter darting of chital deer (Axis axis) in a tropical environment in Australia (Hampton et al. 2021; Amos et al. 2023).
The mortality of a male sambar deer due to drowning in a small water body while entangled in a net resulted in a change to the capture method whereby animals were only pursued when tracking directly away from water. The capture method described here could potentially be adjusted or refined to suit capture attempts in environments different to the one in which we worked. Our study was in a relatively cold (compared with much of Australia) alpine environment. Should the method be deployed to capture sambar deer within their native Asian range, which is predominately tropical and sub-tropical (Timmins et al. 2015), we suspect that sambar deer would be more susceptible to heat stress.
Immobilisation methods
Chemical restraint of wild Cervidae always incurs animal welfare risks at the time of capture and during the post-capture period (Dechen Quinn et al. 2012; Caulkett and Arnemo 2014; Ortega et al. 2020; Thompson et al. 2020). Therefore, it is important to monitor the post-release responses of animals to new or modified capture methods. Chemical immobilisation was used to mitigate the risk to human handlers, but helicopter net gunning operations in northern New South Wales showed that fallow deer can be captured and processed safely and efficiently without the need for chemical restraint (Bengsen et al. 2021). However, physically restraining smaller fallow deer poses greater human and animal welfare risks than restraining red deer (~50–80 kg heavier than fallow) or sambar deer (~140–200 kg heavier than fallow) (Geist and Bayer 1988). Red deer have been captured via net gunning without chemical immobilisation (Latham et al. 2020), and future studies could investigate applying that method in sambar deer.
Induction and recovery times were reduced for both deer species between operations one and two (Table 2), due to improved body mass estimation procedures and refined drug regimes. The prolonged recovery times observed in operation one were reduced in the second operation by reducing the dose of tiletamine–zolazepam (Table 1). The non-reversible nature of tiletamine–zolazepam has practical disadvantages related to prolonged recoveries, increased risk of associated physiological states such as hypothermia, and increased requirements for monitoring (Mayberry et al. 2014). Once tiletamine–zolazepam doses were reduced in November, mean recovery times were considerably reduced (10 min in November compared with 47 min in April), and rapid recoveries were observed with the completely reversible immobilisation regime of medetomidine alone that was given to red deer (Tables 1 and S1). To allow comparable induction times with greater ease of administration, intranasal drug administration could be considered. Intranasal drug administration is generally easier for non-veterinarians in the field, has fewer and less severe complications and requires less training and experience than intravenous or intramuscular drug administration (Shury et al. 2010).
Post-release behaviour
We reported on the movement and activity of collared red and sambar deer for 45 days post-capture to allow for comparisons with similar studies (Northrup et al. 2014; Becciolini et al. 2019; Bengsen et al. 2021). Although no mortalities were reported during this period, accelerometer data showed that red and sambar deer of both sexes tended to exhibit the lowest activity rates immediately following collaring. Then, throughout the monitoring period, activity rates tended to increase until 30–40 days post-collaring for both species, when activity decreased for all modelled responses, except for female red deer collared in operation two, whose activity continued to increase (Fig. 2). Similarly, activity increased over the first 10 days following capture, before stabilising for fallow deer captured via an aerial net gun, processed without chemical immobilisation (Bengsen et al. 2021). Accelerometer data may be a more sensitive indicator of mild impairment, so for the present study, the increase in activity over the monitoring period, may indicate that deer are recovering from impairment as a result of the collaring procedure (Bengsen et al. 2021).
Free-ranging deer are known to exhibit changes to movement rates and site fidelity resulting from capture and chemical immobilisation procedures (e.g. Morellet et al. 2009; Northrup et al. 2014). For male and female sambar deer, movements peaked immediately following capture and steadily decreased for ~10 days following capture and collaring before plateauing or increasing (Fig. 3). Some deer appeared to exhibit a disturbance response immediately following capture, like sambar deer males 49 and 6, which travelled more than 12 and 8 km (respectively) from their capture locations in ~7 days, undertaking faster long-distance movements interspersed with short periods of slower speed movements, before remaining within a small area and moving slower for the remainder of the monitoring period (Figs 3, 4 and S2). Sambar deer experiencing acute stress are known to undertake long-distance movements interspersed with periods of rest (Semiadi et al. 1994), so capture may have contributed to increased movement rates for a short period following capture. However, this disturbance response was not displayed by all sambar deer; some sambar deer of both sexes remainined within a small distance of the capture area for the entire monitoring period, like sambar deer female 11 (Fig. 4). Short-term increases in movements for the period following capture have previously been reported for many Cervidae species, including moose (Alces alces) and male roe deer, which exhibit flight behaviour and show greater displacement from their home ranges following capture (Morellet et al. 2009; Neumann et al. 2011), as well as for an antelope species, nilgai (Boselaphus tragocamelus; Baumgardt et al. 2023). Like sambar deer, male roe deer and moose are largely solitary and tend not to exhibit strong social bonds (Geist 1963; Hewison et al. 1998), so may be more likely to move large distances following capture.
Comparing movement and activity rates pre- and post-capture would have allowed the delineation of movement changes due to capture (e.g. Morellet et al. 2009; Neumann et al. 2011; Northrup et al. 2014; Baumgardt et al. 2023) but was not possible in the present study. Elevated movement rates for the period immediately following collaring did not occur in red deer (Fig. 3), which did not move as far from their point of capture as sambar deer (Fig. S2), despite moving greater average maximum distances than sambar deer throughout the monitoring period (Fig. S2). Red deer are more social (Clutton-Brock et al. 1982) and may seek to re-establish social bonds following disturbance, remaining closer to their capture area, as shown for red deer female 15 and male 40 (Fig. 4). Conversely, post-capture movements of red deer hinds were not consistent with a flight response to move away from the capture area into forest cover for a period of ~8 days following capture (Becciolini et al. 2019). In the present study, low sample sizes preclude us from more definitely characterising red deer movement post-capture.
Conclusion
This study used an aerial net gun and chemical immobilisation to capture 14 free-ranging invasive sambar deer and five red deer in alpine Australia. The method was used across two periods, with refinement of procedures in the second period reducing mortality probability to ≦0.12, with further refinement and reduction of adverse event frequency likely to be feasible. The method presented here can be adapted and refined, to capture sambar deer and red deer within their native and invasive ranges.
Data availability
The data that support the present study will be shared upon reasonable request to the corresponding author.
Conflicts of interest
Thomas Newsome is an Associate Editor of Wildlife Research but was blinded from the peer-review process for this paper.
Declaration of funding
This project was funded by the New South Wales National Parks and Wildlife Service and New South Wales Environmental Trust.
Acknowledgements
We acknowledge the Ngarigo people as the Traditional Owners of the land on which this research was conducted. We thank Dave Forsyth, Matt Amos and Neal Finch for assistance with calculating sambar deer body mass from morphometric data. Aerial net gunning operations were safely and efficiently implemented due to the skills and professionalism of NSW National Parks Park Air pilots Grant Simpson, Brenden Lindsay, Peter Alexander and Tate Steen, along with air crew Ben Winter, Callum Woulfe, Jake Laurie and Ben Burrows. Deer team members Michelle Janes, Ani Gruber, Jessica Cass, Cassie Kuner, Matt Alexander and Rodney Freeman provided extensive support in the development and implementation of the field work, along with Senior Field Officer Rob Burke and Ranger Campbell Young. Veterinary Nurse Emily Hunt from Yass Valley Veterinary provided animal health and monitoring expertise with Darren Larkman providing videography and photographic support.
References
Alford RA, Brown GP, Schwarzkopf L, Phillips BL, Shine R (2009) Comparisons through time and space suggest rapid evolution of dispersal behaviour in an invasive species. Wildlife Research 36(1), 23-28.
| Crossref | Google Scholar |
Amos M, Baxter G, Finch N, Murray P (2014) At home in a new range: wild red deer in south-eastern Queensland. Wildlife Research 41(3), 258-265.
| Crossref | Google Scholar |
Amos M, De Ridder TR, Pople A, Brennan M, Hampton JO (2023) Further refinement of helicopter capture for Australian chital deer (Axis axis). Australian Mammalogy
| Crossref | Google Scholar |
Arnemo JM, Negard T, Søli NE (1994) Chemical capture of free-ranging red deer (Cervus elaphus) with medetomidine-ketamine. Rangifer 14(3), 123-127.
| Crossref | Google Scholar |
Australian Government Department of Climate Change, Energy, the Environment and Water (2021) National Vegetation Information System (NVIS) Version 6.0 – Australia – Extant Vegetation. Available at https://fed.dcceew.gov.au/maps/e7c56ffd33714b1bbf64893b4f13c34a/about [Accessed 6 July 2022]
Bartareau TM (2019) Estimating body mass of Florida white-tailed deer from standard age and morphometric measurements. Wildlife Research 46(4), 334-342.
| Crossref | Google Scholar |
Baumgardt JA, Foley AM, Sliwa KM, DeYoung RW, Ortega-S JA, Hewitt DG, Campbell TA, Goolsby JA, Lohmeyer KH (2023) Effects of helicopter net gunning on the survival and movement behaviour of nilgai antelope. Wildlife Research
| Crossref | Google Scholar |
Becciolini V, Lanini F, Ponzetta MP (2019) Impact of capture and chemical immobilization on the spatial behaviour of red deer Cervus elaphus hinds. Wildlife Biology 2019(1), 1-8.
| Crossref | Google Scholar |
Bengsen AJ, Hampton JO, Comte S, Freney S, Forsyth DM (2021) Evaluation of helicopter net-gunning to capture wild fallow deer (Dama dama). Wildlife Research 48(8), 722-729.
| Crossref | Google Scholar |
Beringer J, Hansen LP, Wilding W, Fischer J, Sheriff SL (1996) Factors affecting capture myopathy in white-tailed deer. The Journal of Wildlife Management 60(2), 373-380.
| Crossref | Google Scholar |
Borowski Z, Gil W, Bartoń K, Zajączkowski G, Łukaszewicz J, Tittenbrun A, Radliński B (2021) Density-related effect of red deer browsing on palatable and unpalatable tree species and forest regeneration dynamics. Forest Ecology and Management 496(1), 119442.
| Crossref | Google Scholar |
Bureau of Meteorology (2020) Latest weather observations for thredbo top station. Available at http://www.bom.gov.au/products/IDN60801/IDN60801.95909.shtml [Accessed 21 April 2022]
Burgin S, Mattila M, McPhee D, Hundloe T (2015) Feral deer in the suburbs: an emerging issue for Australia? Human Dimensions of Wildlife 20(1), 65-80.
| Crossref | Google Scholar |
Cattet MRL, Caulkett NA, Wilson C, Vandenbrink T, Brook RK (2004) Intranasal administration of xylazine to reduce stress in elk captured by net gun. Journal of Wildlife Diseases 40(3), 562-565.
| Crossref | Google Scholar | PubMed |
Cattet M, Boulanger J, Stenhouse G, Powell RA, Reynolds-Hogland MJ (2008) An evaluation of long-term capture effects in ursids: implications for wildlife welfare and research. Journal of Mammalogy 89(4), 973-990.
| Crossref | Google Scholar |
Chatterjee D, Sankar K, Qureshi Q, Malik PK, Nigam P (2014) Ranging pattern and habitat use of sambar (Rusa unicolor) in Sariska Tiger Reserve, Rajasthan, Western India. DSG Newsletter 26(1), 60-71.
| Google Scholar |
Cripps JK, Pacioni C, Scroggie MP, Woolnough AP, Ramsey DSL (2019) Introduced deer and their potential role in disease transmission to livestock in Australia. Mammal Review 49(1), 60-77.
| Crossref | Google Scholar |
Davis NE, Bennett A, Forsyth DM, Bowman DMJS, Lefroy EC, Wood SW, Woolnough AP, West P, Hampton JO, Johnson CN (2016) A systematic review of the impacts and management of introduced deer (family Cervidae) in Australia. Wildlife Research 43(6), 515-532.
| Crossref | Google Scholar |
Dechen Quinn AC, Williams DM, Porter WF (2012) Postcapture movement rates can inform data-censoring protocols for GPS-collared animals. Journal of Mammalogy 93(2), 456-463.
| Crossref | Google Scholar |
Doherty TS, Ritchie EG (2017) Stop jumping the gun: a call for evidence-based invasive predator management. Conservation Letters 10(1), 15-22.
| Crossref | Google Scholar |
Flueck WT, Smith-Flueck JAM, Bonino NA (2005) A preliminary analysis of death cause, capture-related mortality, and survival of adult red deer in northwestern Patagonia. Ecología Austral 15(1), 23-30.
| Google Scholar |
Frid A, Dill LM (2002) Human-caused disturbance stimuli as a form of predation risk. Conservation Ecology 6(1), 11.
| Crossref | Google Scholar |
Geist V (1963) On the behaviour of the North American moose (Alces alces andersoni Peterson 1950) in British Columbia. Behaviour 20(3-4), 377-415.
| Crossref | Google Scholar |
Geist V, Bayer M (1988) Sexual dimorphism in the Cervidae and its relation to habitat. Journal of Zoology 214(1), 45-53.
| Crossref | Google Scholar |
Gormley AM, Forsyth DM, Griffioen P, Lindeman M, Ramsey DSL, Scroggie MP, Woodford L (2011) Using presence-only and presence-absence data to estimate the current and potential distributions of established invasive species. Journal of Applied Ecology 48(1), 25-34.
| Crossref | Google Scholar | PubMed |
Hampton JO, Davis NE (2020) Impacts of introduced deer in Victoria. Victorian Naturalist 137(6), 276-281.
| Google Scholar |
Hampton JO, Finch NA, Watter K, Amos M, Pople T, Moriarty A, Jacotine A, Panther D, McGhie C, Davies C, Mitchell J, Forsyth DM (2019a) A review of methods used to capture and restrain introduced wild deer in Australia. Australian Mammalogy 41(1), 1-11.
| Crossref | Google Scholar |
Hampton JO, MacKenzie DI, Forsyth DM (2019b) How many to sample? Statistical guidelines for monitoring animal welfare outcomes. PLoS ONE 14(1), e0211417.
| Crossref | Google Scholar | PubMed |
Hampton JO, Amos M, Pople A, Brennan M, Forsyth DM (2021) Minimising mortalities in capturing wildlife: refinement of helicopter darting of chital deer (Axis axis) in Australia. Wildlife Research 48(4), 304-313.
| Crossref | Google Scholar |
Hampton JO, Gill SJ, Spielman D, Peters A, Vitali S, Boardman W, Portas T, Coulson G (2022) Veterinary procedures for Australian wildlife. In ‘Wildlife research in Australia: practical and applied methods’. (Eds BP Smith, HP Waudby, C Alberthsen, JO Hampton) pp. 360–376. (CSIRO Publishing: Clayton, Australia)
Harms NJ, Jung TS, Hallock M, Egli K (2018) Efficacy of a butorphanol, azaperone, and medetomidine combination for helicopter-based immobilization of bison (Bison bison). Journal of Wildlife Diseases 54(4), 819-824.
| Crossref | Google Scholar | PubMed |
Hartley R, Blanchard W, Schroder M, Lindenmayer DB, Sato C, Scheele BC (2022) Exotic herbivores dominate Australian high-elevation grasslands. Conservation Science and Practice 4, e601.
| Crossref | Google Scholar |
Jacques CN, Jenks JA, Deperno CS, Sievers JD, Grovenburg TW, Brinkman TJ, Swanson CC, Stillings BA (2009) Evaluating ungulate mortality associated with helicopter net-gun captures in the northern Great Plains. Journal of Wildlife Management 73(8), 1282-1291.
| Crossref | Google Scholar |
Kays R, Crofoot MC, Jetz W, Wikelski M (2015) Terrestrial animal tracking as an eye on life and planet. Science 348(6240), aaa2478.
| Crossref | Google Scholar |
Kreeger TJ, White PJ, Seal US, Tester JR (1990) Pathological responses of red foxes to foothold traps. The Journal of Wildlife Management 54(1), 147-160.
| Crossref | Google Scholar |
Latham ADM, Davidson B, Warburton B, Yockney I, Hampton JO (2020) Efficacy and animal welfare impacts of novel capture methods for two species of invasive wild mammals in New Zealand. Animals 10(1), 44.
| Crossref | Google Scholar |
Lovari S, Lorenzini R, Masseti M, Pereladova O, Carden RF, Brook SM, Mattioli S (2018) Cervus elaphus (errata version published in 2019). The IUCN Red List of Threatened Species 2018: e.T55997072A142404453. Available at https://dx.doi.org/10.2305/IUCN.UK.2018-2.RLTS.T55997072A142404453.en [Accessed 15 August 2022]
Marco I, Lavín S (1999) Effect of the method of capture on the haematology and blood chemistry of red deer (Cervus elaphus). Research in Veterinary Science 66(2), 81-84.
| Crossref | Google Scholar | PubMed |
Mayberry C, Bencini R, Mawson PR, Maloney SK (2014) Sedation of western grey kangaroos (Macropus fuliginosus ocydromus) with tiletamine-zolazepam. Animal Welfare 23(2), 141-144.
| Crossref | Google Scholar |
McMahon CR, Hindell MA, Harcourt RG (2012) Publish or perish: why it’s important to publicise how, and if, research activities affect animals. Wildlife Research 39(5), 375-377.
| Crossref | Google Scholar |
McNay RS, Lamb CT, Giguere L, Williams SH, Martin H, Sutherland GD, Hebblewhite M (2022) Demographic responses of nearly extirpated endangered mountain caribou to recovery actions in Central British Columbia. Ecological Applications 32(5), e2580.
| Crossref | Google Scholar | PubMed |
Morellet N, Verheyden H, Angibault J-M, Cargnelutti B, Lourtet B, Hewison MAJ (2009) The effect of capture on ranging behaviour and activity of the European roe deer Capreolus capreolus. Wildlife Biology 15(3), 278-287.
| Crossref | Google Scholar |
Moriarty A (2004) The liberation, distribution, abundance and management of wild deer in Australia. Wildlife Research 31(3), 291-299.
| Crossref | Google Scholar |
Moseby KE, Read JL, Andersen GE (2021) Goat movement patterns inform management of feral goat populations in semiarid rangelands. Wildlife Research 48(1), 44-54.
| Crossref | Google Scholar |
NASA/METI/AIST/Japan Spacesystems and US/Japan ASTER Science Team (2019) ASTER Global Digital Elevation Model V003. Available at https://doi.org/10.5067/ASTER/ASTGTM.003 [Accessed 7 July 2022]
Neumann W, Ericsson G, Dettki H, Arnemo JM (2011) Effect of immobilizations on the activity and space use of female moose (Alces alces). Canadian Journal of Zoology 89(11), 1013-1018.
| Crossref | Google Scholar |
Northrup JM, Anderson CR, Jr, Wittemyer G (2014) Effects of helicopter capture and handling on movement behavior of mule deer. The Journal of Wildlife Management 78(4), 731-738.
| Crossref | Google Scholar |
NSW Department of Planning and Environment (2021) Steep land (edition 1). Available at https://www.planningportal.nsw.gov.au/opendata/dataset/steep [Accessed 6 July 2022]
Ortega AC, Dwinnell SP, Lasharr TN, Jakopak RP, Denryter K, Huggler KS, Hayes MM, Aikens EO, Verzuh TL, May AB, Kauffman MJ, Monteith KL (2020) Effectiveness of partial sedation to reduce stress in captured mule deer. The Journal of Wildlife Management 84(8), 1445-1456.
| Crossref | Google Scholar |
Peterson MN, Lopez RR, Frank PA, Peterson MJ, Silvy NJ (2003) Evaluating capture methods for urban white-tailed deer. Wildlife Society Bulletin (1973–2006) 31(4), 1176-1187.
| Google Scholar |
Roug A, Larsen R, Walden X, Hersey K, McMillan B, Caulkett N (2022) Physiological effects of azaperone and midazolam on netgun-captured mule deer (Odocoileus hemionus). Journal of Wildlife Diseases 58(1), 168-182.
| Crossref | Google Scholar | PubMed |
Russell MB, Woodall CW, Potter KM, Walters BF, Domke GM, Oswalt CM (2017) Interactions between white-tailed deer density and the composition of forest understories in the northern United States. Forest Ecology and Management 384(1), 26-33.
| Crossref | Google Scholar |
Searle AK (1981) Red deer in Queensland. Queensland Agricultural Journal 107(1), 17-20.
| Google Scholar |
Semiadi G, Muir PD, Barry TN (1994) General biology of sambar deer (Cervus unicolor) in captivity. New Zealand Journal of Agricultural Research 37(1), 79-85.
| Crossref | Google Scholar |
Shury TK, Caulkett NA, Woodbury MR (2010) Intranasal naltrexone and atipamezole for reversal of white-tailed deer immobilized with carfentanil and medetomidine. The Canadian Veterinary Journal 51(5), 501-505.
| Google Scholar | PubMed |
Spitz DB, Rowland MM, Clark DA, Wisdom MJ, Smith JB, Brown CL, Levi T (2019) Behavioral changes and nutritional consequences to elk (Cervus canadensis) avoiding perceived risk from human hunters. Ecosphere 10(9), e02864.
| Crossref | Google Scholar |
Stankowich T (2008) Ungulate flight responses to human disturbance: a review and meta-analysis. Biological Conservation 141(9), 2159-2173.
| Crossref | Google Scholar |
Thompson DP, Crouse JA, McDonough TJ, Barboza PS, Jaques S (2020) Acute thermal and stress response in moose to chemical immobilization. The Journal of Wildlife Management 84(6), 1051-1062.
| Crossref | Google Scholar |
Timmins R, Kawanishi K, Giman B, Lynam A, Chan B, Steinmetz R, Sagar Baral H, Samba Kumar N (2015) Rusa unicolor (errata version published in 2015). The IUCN Red List of Threatened Species 2015: e.T41790A85628124. Available at https://dx.doi.org/10.2305/IUCN.UK.2015-2.RLTS.T41790A22156247.en [Accessed 15 August 2022]
Van de Kerk M, McMillan BR, Hersey KR, Roug A, Larsen RT (2020) Effect of net-gun capture on survival of mule deer. The Journal of Wildlife Management 84(4), 813-820.
| Crossref | Google Scholar |
Vilà M, Espinar JL, Hejda M, Hulme PE, Jarošík V, Maron JL, Pergl J, Schaffner U, Sun Y, Pyšek P (2011) Ecological impacts of invasive alien plants: a meta-analysis of their effects on species, communities and ecosystems. Ecology Letters 14(7), 702-708.
| Crossref | Google Scholar | PubMed |
Ward-Jones J, Pulsford I, Thackway R, Bishwokarma D, Freudenberger D (2019) Impacts of feral horses and deer on an endangered woodland of Kosciuszko National Park. Ecological Management & Restoration 20(1), 37-46.
| Crossref | Google Scholar |
Watter K, Thomas E, White N, Finch N, Murray PJ (2020) Reproductive seasonality and rate of increase of wild sambar deer (Rusa unicolor) in a new environment, Victoria, Australia. Animal Reproduction Science 223(1), 106630.
| Crossref | Google Scholar |
Webb SL, Lewis JS, Hewitt DG, Hellickson MW, Bryant FC (2008) Assessing the helicopter and net gun as a capture technique for white-tailed deer. The Journal of Wildlife Management 72(1), 310-314.
| Crossref | Google Scholar |
Wolkers J, Wensing T, Bruinderink GWTAG (1994) Sedation of wild boar (sus scrofa) and red deer (cervus elaphus) with medetomidine and the influence on some haematological and serum biochemical variables. Veterinary Quarterly 16(1), 7-9.
| Crossref | Google Scholar | PubMed |
Yen S-C, Wang Y, Yu P-H, Kuan Y-P, Liao Y-C, Chen K-H, Weng G-J (2019) Seasonal space use and habitat selection of sambar in Taiwan. Journal of Wildlife Management 83(1), 22-31.
| Crossref | Google Scholar |