Modelling the management of an invasive species at landscape scale: are oral contraceptives the missing ingredient for success?
S. Croft

A
B
Abstract
Invasive non-native species are on the rise worldwide, exacerbating already significant environmental and economic impacts. Concurrently, public attitudes towards methods of controlling these species are changing, with greater demand for non-lethal solutions. This has fostered research into developing new, effective technologies and strategies for wildlife management.
On the basis of a case study focusing on the eastern grey squirrel (Sciurus carolinensis) in the UK, this study aimed to understand the potential for population management, using either live trapping or oral contraception. First, assuming completely coordinated landscape-scale control, and then, coordinated control only on parts of the landscape, accounting for the potential that landowners’ attitudes towards alternative methods may differ and reflect those of the wider public.
We used an existing spatially explicit individual-based modelling approach applying various parameterisations to define management. We varied the density of traps or hoppers delivering contraceptives, the duration of deployment, contraceptive efficacy, initial population size, and the probability of landowner participation. The latter was based on a previous public survey, suggesting that 40% of the respondents were open to management using trapping and 64% to using contraception.
With complete coordinated control, trapping was generally faster and more cost-effective than was contraception. However, when differences in social acceptance were considered, reducing participation and, consequently, the spatial coverage of management, contraception was found to maintain greater population reductions than was trapping with similar effort, assuming a contraceptive efficacy of 75% or higher.
This study added another layer of complexity to managing invasive non-native species, namely the potential effect that landowners’ attitudes to different methods of population management might have on the level of coordinated control at landscape scale. In a situation such as the one modelled by this study, where management is not a legal requirement, this human dimension must be considered alongside cost-effectiveness, to develop successful control in line with management goals.
Further work is required to establish the actual attitudes of landowners, and in different contexts (e.g. urban, rural areas), how this may change as new approaches become available, and then how spatial variation (clustering) in the resulting wildlife population control may affect outcomes.
Keywords: comparison, contraception, cost, human dimension, individual-based model, invasive non-native species, population management, social acceptance, spatial coverage, trapping.
Introduction
In the UK and in Italy, the widespread non-native eastern grey squirrel (Sciurus carolinensis) has caused the decline of the native red squirrel (Sciurus vulgaris) through resource competition and transmission of diseases such as the squirrel poxvirus (Rushton et al. 2000; Gurnell et al. 2004). Red squirrels, that in 1945 were distributed across >80% of the UK, are now present in <20% of the country (https://www.squirrelaccord.uk/squirrels/distribution; accessed 2 June 2023) and the species is listed by the International Union for Conservation of Nature as ‘Endangered’ in England and Wales. Grey squirrels are also associated with significant economic and environmental impacts. For instance, the cost of this species’ damage to trees in England and Wales is estimated to be between US$2.5 and US$45 million per year in lost timber value, reduced carbon capture, damage mitigation, and trees to replace those that died as a result of grey squirrel bark stripping (Richardson et al. 2021). This impact becomes particularly relevant in the context of the UK Government’s commitment to increase woodland cover substantially across the country by 2050, as part of the strategy to reach net-zero carbon emissions (HM Government 2021). Reducing the density of grey squirrels is thus regarded as key to mitigating their impacts and to support the re-colonisation of red squirrels across their former range (Bertolino et al. 2014; Schuchert et al. 2014; Goldstein et al. 2016).
Traditional methods to control grey squirrel populations include poisoning by using anticoagulants, now illegal in the UK, trapping and dispatching, and shooting. In most areas, culling via trapping and shooting has failed to reduce grey squirrel numbers and their range, probably because of a combination of a lack of coordinated control, and significant costs and effort required to sustain this method in time and space (Gurnell 1999; Derbridge et al. 2016). Notable exceptions include the eradication of grey squirrels in Anglesey, which was successfully undertaken via culling, although this took 15 years and cost approximately £1 million (Derbridge et al. 2016). The failure of controlling numbers and range expansion of this species has resulted in ~2.5 million grey squirrels estimated to live in the UK, against the remaining 287 000 red squirrels (Croft et al. 2017; Mathews et al. 2018).
In recent years, increasing public opposition towards culling, together with the fact that culling has been ineffective in reducing grey squirrel populations, has led to demand for alternative options for grey squirrel control (Bremner and Park 2007; Dunn et al. 2018; Lioy et al. 2019; Jacoblinnert et al. 2022). Fertility control has been tested to determine its effects on population size and on the rate of population recovery in several rodent species with mixed results (e.g. Shi et al. 2002; Massei and Cowan 2014; Chen et al. 2022; Liu et al. 2023). This method is generally well accepted or even preferred to other methods that are predicted to be less effective (Dunn et al. 2018; Quinn et al. 2019; Hunold and Mazuchowski 2020). For instance, control strategies consisting solely of immunocontraceptive vaccines to eradicate the non-native grey squirrel from Ireland were assessed to be less effective than culling but were often preferred by public interest groups (Goldstein et al. 2016). Similarly, a survey on the UK public’s attitudes to grey squirrel control highlighted that contraception was the preferred method, supported by 63% of the 3758 respondents, which found this method acceptable or highly acceptable, whereas lethal methods ranked as the least acceptable. In this survey, more than two-thirds of respondents (67%) consider poisoning as either unacceptable or highly unacceptable, whereas the corresponding figure in the case of contraception was only 14% (Dunn et al. 2018).
Although oral contraceptives that can be delivered in baits to grey squirrels are not yet available, research is ongoing to develop these drugs (Beatham et al. 2021; Gill et al. 2022) and modelling has been used to assess whether fertility control could be employed to complement traditional methods of grey squirrel control. For instance, by modelling the impact of culling and fertility control, Rushton et al. (2002) concluded that an integrated control strategy, incorporating both trapping and immunocontraception, may be the best option for controlling grey squirrels, and suggested that this combined strategy needs to include the relative costs of these control options. Krause et al. (2014) used a model to simulate population reduction of fox squirrels (Sciurus niger) in two fertility control treatments (60% and 80% of females sterilised) and found that contraception can reduce fox squirrel populations if the vaccine is effective for at least 2 years or if at least 71% of the females are treated. Conversely, modelling by Goldstein et al. (2016) suggested that a 5-year immunocontraception program for female grey squirrels was not as effective as was culling in decreasing abundance. A greater difference between the two methods was observed in the overall abundance of the population, with high intensity region-wide contraception scenarios predicted to lower abundance after 25 years by 8–11%, compared with 26–43% for high-intensity culls at the same regional scale. The results also suggested that both culling, and fertility control strategies had the strongest effects when populations exhibited the lowest population growth (Goldstein et al. 2016).
Relatively little attention has been paid to factors that may affect the impact of management on population size. These factors include timing and density of traps employed for culling and of feeders (hoppers) used to distribute contraceptives, initial density of grey squirrels, duration, and efficacy of contraceptives (the latter being defined as proportion sterilised over the total number of animals that consumed a contraceptive), and size and distribution of woodland patches where population management is being undertaken. Croft et al. (2021) employed a spatially explicit individual-based population model to assess the relative effort required to manage and eradicate grey squirrels through culling, fertility control, or culling followed by fertility control, supported by empirical parameter estimates. In this study, the ‘effort’, expressed as density and number of deployment days of both traps and hoppers, was kept constant, so as to allow comparisons between the two population management methods. When these methods were modelled at a regional scale, both fertility control and culling were applied to woodlands greater than one hectare (ha) in area, thus potentially leaving many relatively small squirrel refugia unaffected by population control (Croft et al. 2021). The results suggested that, at least for the initial squirrel densities assumed, fertility control alone was unlikely to achieve rapid enough reduction and could not completely replace culling. When fertility control was applied to populations at low density, following short-term culling, eradication could be achieved within the same timescales as culling alone, but with substantially lower costs.
Besides the method chosen to reduce grey squirrel densities, the level of coordination of population control at landscape scale, in terms of proportion and distribution of sites where this control is undertaken, is likely to affect the local densities of squirrels. The availability of sites where squirrel population control can be undertaken and the method implemented will depend on landowners’ permission to work in these area. Building on the results of Croft et al. (2021), the aim of this study was to estimate the effects of population management by using either live trapping or oral contraception, first, assuming complete landscape-scale coordinated control of squirrel populations, and then, coordinated control only on parts of the landscape, accounting for the potential that attitudes of landowners towards alternative squirrel population management methods may differ and reflect those of the wider public.
Material and methods
Model framework
For this study, we retained the model architecture and setup (i.e. study area and parameterisation) described in Croft et al. (2021). Written in the Python2™ programming language (source code files are provided in Supplementary material S1), this model applied an individual-based approach with agents (squirrels) operating across an abstraction of a real-world landscape comprising woodland patches of varying quality (spanning 12 100 ha and supporting ~30 000 squirrels; mean of 2.5 squirrels/ha). Woodland patches were connected by a network defining the likelihood of movement among them, accounting for landscape resistance (‘cost-distance’). Squirrels were distinguished by their demographic class (e.g. stage and sex) and exhibited defined stochastic behaviours (e.g. survival, reproduction, dispersal), resolved annually, to emulate a realistic population and spatial dynamics.
In addition to ecological behaviour, the model also included mechanisms to simulate two types of population management, namely (1) removal by using single-capture live cage traps; and (2) oral contraception by using bait hoppers. Both types captured the individual-level interactions of squirrels, derived from empirical data (Beatham et al. 2021; see Croft et al. 2021, appendix C, for details of parameter derivation), reflecting actual management efficacy with respect to ‘effort’, the latter defined by the specific density and duration (number of days) that traps and hoppers were deployed each year. Efficacy of trapping, or more specifically numbers of squirrel interactions with traps, was largely defined by total trap-days (number of traps multiplied by the days deployed) per year, but accounting for fixed limits of removal each day. Efficacy of (oral) contraception was the product of several components split across (1) delivery/consumption, which, like trapping, was defined by hopper-days (although unconstrained by the same fixed limits on daily interactions), but also by the relative squirrel to hopper ratio (mean squirrel density divided by the mean hopper density) to account for the effects of competition without removals, and (2) contraception given consumption, defined by fixed probabilities of a squirrel becoming infertile following hopper visitation (contraceptive efficacy) and, subsequently, returning to fertility as the effect of contraception wore off.
Simulations
All patches were initialised with adult squirrels equal to their capacity, with their gender being assigned at random. Simulations then consisted of an initial ‘burn-in’ phase of 10 years to allow population demographics sufficient time to stabilise (preliminary testing not included here showed that proportions of each stage/sex cohort reached equilibrium after approximately 5 years), followed by a control phase of 100 years, in which various combinations of management (trapping and/or contraception defined on an annual basis) could be applied. For each parameterisation, we performed 100 repetitions outputting a population summary (numbers by stage, sex, and fertility status) per patch per year.
Management scenarios
The first aim of the study was to test and compare management using trapping or contraception under a range of different parameterisations, hereafter referred to as management routines, within the bounds of what may be considered feasible in the real world. For simplicity, these initial scenarios were conducted assuming comprehensive coverage across the entire model landscape. Unlike Croft et al. (2021), this included small woodlands (less than 1 ha in area) by ensuring a minimum of one device (trap or hopper) was placed in each patch.
Guidance on squirrel management using trapping typically recommends repeated bursts of deployment (5 days/month) dispersed throughout January to September (a maximum of 9 months of the year) at densities varying from 0.25 traps/ha in coniferous woodland to 1 trap/ha in broadleaf (Mayle et al. 2007; Gurnell and Pepper 2016). However, in practice, both the timing and duration of deployments can vary greatly (Schuchert et al. 2014). To capture the range of potential combinations (routines) for trapping deployment (on the basis of the recommendations and practical experience outlined in Mayle et al. 2007; Schuchert et al. 2014; Gurnell and Pepper 2016; see Croft et al. 2021), we considered trap densities of 0.125, 0.25, 0.5, 1, 2, up to 4 traps/ha, paired with deployment durations of 5, 10, 15, 30, and 45 days/year (1, 2, 3, 6 and 9 blocks of 5 days). The duration of effect of contraceptives as well as the proportion of animals consuming the bait and becoming infertile have not yet been established, but it is likely that either 1 or 2 5-day deployments of baits delivering contraceptives may be necessary to induce infertility prior to the squirrels’ two breeding cycles in late winter/early spring and in early summer. Here, we considered hopper densities of 0.5, 1, 2, and 4 hoppers/ha, paired with deployment durations of 5 and 10 days/year (1 or 2 separate blocks of 5 days). Although no data are available on the effectiveness of oral contraceptives, preliminary work suggests that at least 60% of animals administered an oral contraceptive could become infertile (Massei et al. 2020). Using a sensitivity analysis to illustrate the impact of this component, we also considered a range of rates of induced infertility, i.e. 50%, 60%, 70%, 80%, 90%, and 100%, in combination with each contraceptive delivery routine. In all contraceptive management routines, we make the conservative assumption that squirrels were infertile only for a year after treatment i.e. they had a 100% return rate. Finally, for each management routine, we tested various initial population sizes from a low of 20% to 100% of the initial population at saturation, with intermediates of 60% and 80%. Initial populations below the default 100% saturation (i.e. carrying capacity) of the model were achieved by applying a single year of trapping of given effort as an addition at the end of the ‘burn-in’ period. This approach can be considered analogous to the sequential management strategy described in Croft et al. (2021).
To assess the performance of each management routine, we produced summary statistics (median and range across repetitions) relating to (1) the speed of population reduction (percentage reduction after the first year of management and time to complete eradication) which is particularly important in terms of damage mitigation, and (2) management effort, in terms of labour costs which usually comprise the largest portion of expense (Rushton et al. 2002). For the latter, we estimated both annual effort (ongoing costs without eradication) and total effort to achieve eradication on the basis of the expected number of visits by staff involved in squirrel population control that would be required to maintain either traps or hoppers. For each trapping period of 5 days, a total of seven visits are required, one to deploy the trap and pre-bait, then one to set the trap, and one to check/reset the trap every day. For each contraception period of 5 days, only three visits are required (one to deploy and pre-bait, one to load contraceptive, and one to collect the hopper) on the basis of the same assumption that we have made previously, namely that hoppers can hold at least 5 days’ worth of bait.
Realistically, completely coordinated management across a landscape is unlikely. Although it is unknown how the results of public surveys exploring attitudes towards methods to manage grey squirrels will reflect the attitudes of landowners, it seems reasonable to assume that there will be similar variation in support for different management options (Dunn et al. 2018). To explore the potential impact of such variation, we ran simulations considering that only a proportion of the landscape was covered by squirrel population management. As previously, a fixed management routine was considered (optimal routines derived from simulations using full coverage), but was applied to patches stochastically, on the basis of a fixed probability of participation, and, in the absence of ownership information, the decision for each patch was assumed to be independent (i.e. we assumed each woodland had a different owner with a particular attitude to grey squirrel management). Although it is conceivable that ownership and/or attitude to management may exhibit an element of spatial autocorrelation, polarising participation across a landscape and deviating away from our simplifying assumption here, this potential bias was beyond the scope of this study, which presents a first attempt to illustrate the gross effects of incomplete access on landscape-scale management. On the basis of previous work (Dunn et al. 2018), each patch/owner was estimated to have a 40% probability of engaging with live trapping and a 64% probability of engaging with contraception (a maximum of 80% would consider engaging in management of any kind). Note that Dunn et al. (2018) did not provide any data on relative preference of management methods, and so it was not possible to determine, if a contraceptive were available, what proportion of those engaged in lethal methods would adopt non-lethal control. As the extreme case, we therefore assumed that all practitioners currently in favour of lethal control would switch to a non-lethal alternative if available according to the probabilities already outlined. To compare trapping and contraception, we examined squirrel numbers in unmanaged and managed patches and across the landscape, aggregating across repetitions to produce a median and range.
Results
Full spatial coverage
Summary results for all tested combinations of both trapping and contraception routines and initial conditions (population saturation) are provided in Supplementary material S2.
As expected, trapping efficacy in terms of percentage reduction in the first year of management was strongly (positively) correlated with trap-days (Fig. 1b). The relationship was broadly maintained regardless of initial population, although there was a marginal trend at lower trapping efforts, with higher initial population showing lower maximum reduction, likely owing to the fewer number of traps available per squirrel (limiting factor).
Full coverage (trapping). Plots showing population reduction in the first year of management (speed) against (a) total effort (labour cost) to achieve eradication, (b) annual effort deployed, for various trapping routines (trap densities and durations) and initial population saturation (denoted by marker shape). Marker colour denotes annual effort in trap-days, with darker colours representing higher values. Trapping performance is strongly correlated with trap-days, regardless of initial population. There is an optimal effort to achieve eradication of about 11 trap-days.ha−1.year−1, which balances (initial) reduction speed with (longer-term) eradication effort. Maintenance (zero growth) can be achieved with 1.875 trap-days.ha−1.year−1, regardless of initial population.
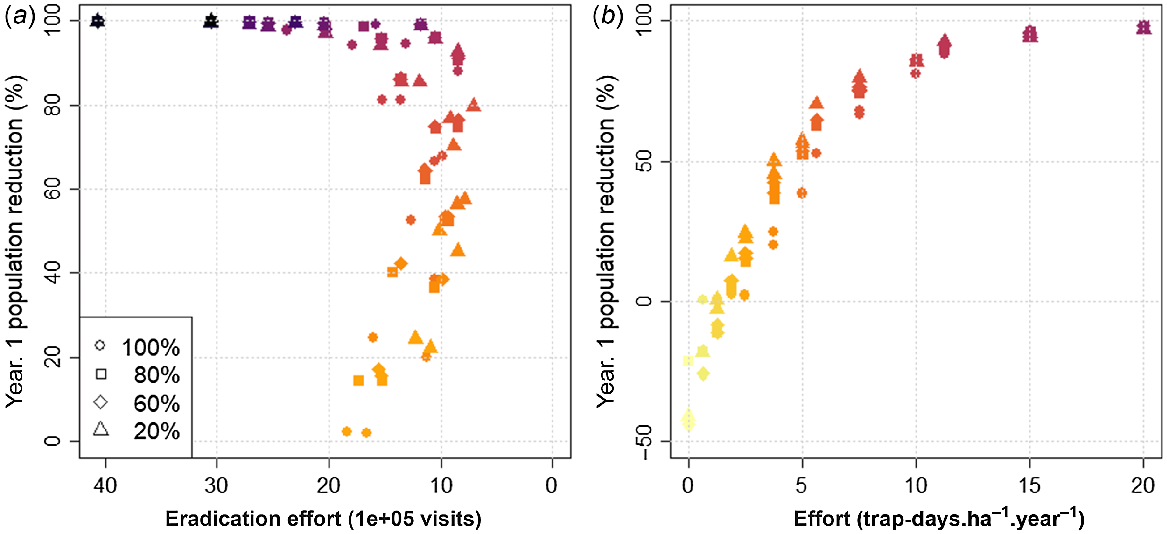
Eradication within the 100-year limit simulated was achieved in almost all levels of effort (trap-days per hectare per year) tested. Failure to eradicate occurred only when effort was lower than or equal to 1.875 trap-days.ha−1.year−1, or 42 366 trap visits/year spread across the 12 100-ha landscape. Eradication within the 15-year period equivalent to the large-scale removal of grey squirrels on Anglesey required a minimum effort of 5 trap-days/ha, 85 267 trap visits/year (Supplementary material S2: Table 1). Balancing both the speed of reduction (percentage reduction in the first year of management) and total management effort to eradication (total number of trap visits), there appeared to be an optimal trapping effort of ~11.25 trap-days.ha−1.year−1, equivalent to 211 932 visits/year (Fig. 1a). At this level of trapping effort, population reduction was between 88% and 93%, depending on initial population saturation, with eradication achieved in a median of 4 years at a total effort of 847 728 visits. At higher effort, any gains in the speed of population reduction, both the initial annual rate and the overall time to eradication, are outweighed by additional cost of control. For example, with an effort of 20 trap-days.ha−1.year−1, initial population reduction increased to 98% (5–10% increase from 11.25 trap-days/ha/year), but at a total eradication effort of 2 374 246 visits, i.e. being about three times more expensive overall than the 11.25 trap-days.ha−1.year−1.
In terms of population maintenance (zero growth) following initial reduction (simulations with 20%, 60% and 80% initial population saturation only), the results suggested that approximately 1.875 trap-days.ha−1.year−1 at an equivalent effort of 42 336 visits are required (Fig. 1b). Where trapping effort is lower, populations can rebound, up to a maximum increase of 40% after the first year as effort approaches zero.
The efficacy of management using contraception was more complex and based on several factors (Fig. 2). These factors included contraceptive efficacy (i.e. the proportion of animals that consumed a contraceptive and became infertile and the duration of infertility) and the ratio between number of feeders of baits containing contraceptives and number of squirrels. Performance in terms of the speed of population reduction (in the first year of simulation) was positively related to the number of hopper-days and contraceptive efficacy, and negatively related to hopper density by way of the squirrel to hopper ratio (the average number of squirrels per hopper).
Full coverage (contraception). Plots showing the impact on management efficacy relating to several factors associated with contraception: annual effort in hopper days (a, b), squirrel to hopper ratio (c, d), and contraceptive efficacy (e, f). Plots a, c, and e show population reduction in the first year of management (speed) against total effort (labour cost) to achieve eradication with marker colours corresponding to the magnitude of each influencing factor (x-axes in b, d, and f respectively). Plots b, d, and f show population reduction in the first year of management (speed) against each factor individually. In all plots, darker colours represent higher values for each of the respective factors. Marker shape denotes the initial population saturation (size). Where combinations of marker colour and symbol are present in b, d, or f but not in a, c, or e respectively this indicates eradication (zero density) was not achieved within the simulation time. Contraception is slower than trapping and, in most instances, requires greater effort to achieve eradication despite a lower maintenance burden. Unlike trapping, performance is related to hopper days (positively), the squirrel to hopper ratio (negatively) and contraceptive efficacy (positively). Optimal contraceptive delivery (balancing speed with effort) can be achieved with 20 hoppers.ha−1.year−1. and hopper density such that there are a maximum of 0.5 squirrels per hopper (or 2 hoppers per squirrel). Additional effort beyond this yields little benefit and is therefore redundant.
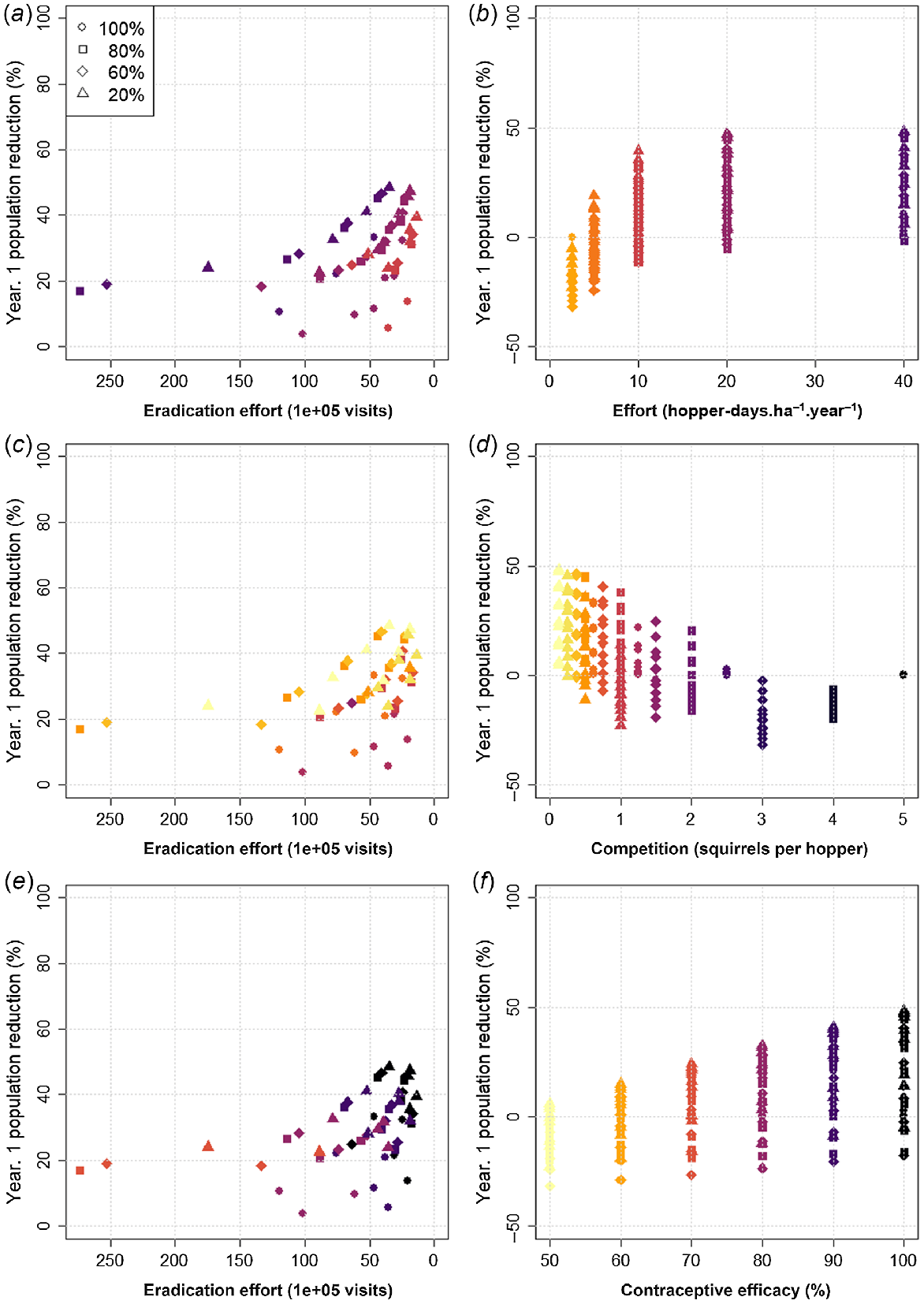
With a ‘perfect’ (100%) contraceptive, eradication within 100-years required a minimum of 10 hopper-days.ha−1.year−1 and a squirrel to hopper ratio of 1.5 or less. Eradication with a contraceptive efficacy as low as 80% could be guaranteed (eradication in all repetitions within 100 years) but required a delivery effort of at least 20 hopper-days.ha−1.year−1 and hopper density such that the squirrel to hopper ratio did not exceed 0.75. Eradication with a 70% effective contraceptive was possible but not guaranteed and only where delivery was maximised. Eradication within 15 years was not possible with contraception alone, but could be achieved with a ‘perfect’ contraceptive and high deployment effort (20–40 hoppers.ha−1.year−1), if preceded by a year of intense trapping (at least 40% population reduction; Supplementary material S2: Table 2).
Like trapping, there appeared to be an optimal level of effort for contraceptive delivery when using 20 hoppers.ha−1.year−1 (equivalent to 145 362 hopper visits per year) and a hopper density such that the number of squirrels per hopper was no more than 0.5 (at least two hoppers per squirrel). When these criteria were met, population reduction from the first year of management reached 48% and the total effort to eradication was approximately 2 000 000 hopper visits, which was about half the numbers removed and double the effort to eradication compared with optimal trapping before accounting for an imperfect contraceptive (<100% efficacy of contraception given consumption).
For a ‘perfect’ contraceptive, a minimum annual delivery effort of 5 hopper-days.ha−1.year−1, equivalent to a total of 36 543 hopper visits per year, was required with hopper density to maintain a squirrel to hopper ratio of 2.5 or less. As was the case for eradication, maintenance was achievable for lower contraceptive efficacies (including 50%), but generally (below an efficacy of 90%) with higher annual effort than that for trapping depending on initial population saturation (initial squirrel to hopper ratio). For example, with a 60% effective contraceptive, maintenance could be achieved with an annual effort of 72 711 hopper visits, assuming an initial population reduction of at least 40% (60% saturation).
Partial spatial coverage
Fig. 3 illustrates the impact of management when partially applied across a landscape. For management based on optimised trapping effort of 11.25 trap-days.ha−1.year−1 applied across 40% of patches, a total effort of 84 770 visits/year, eradication was no longer possible. In managed patches, population reduction was rapid but due to constant reinvasion from unmanaged patches, the population retained an effective density of approximately 1.1 squirrels/ha. Across the landscape, squirrel density fell from 2.5 to 1.9 squirrels/ha. For management based on contraception delivered with 4 hoppers/ha deployed for 5 days/year (20 hopper-days) applied across 64% of patches, a total effort of 93 000 visits/year, eradication was also unachievable. Population reductions were notably slower than trapping and in managed populations less effective, reaching densities of 1.2 and 1.5 squirrels/ha given a 100% and 75% effective contraceptive respectively. However, when aggregated with unmanaged populations across the landscape, average densities reached approximately 1.5 and 1.8 squirrels/ha respectively, lower than that of trapping.
Partial coverage. Plots showing squirrel numbers in unmanaged patches (a, b), managed patches (c, d), and across all patches (e, f), against time, given management using trapping at 11.25 trap-days/year in 40% of patches (a, c, e) or contraception with delivery at 4 hoppers/ha for 5 days and contraceptive efficacy of 75% (red) and 100% (black) in 64% of patches (b, d, f). Solid lines denote median values and dotted lines the range (minumum, maximum). Even in intensively trapped patches, eradication is no longer possible. Even though population reduction in managed patches is slower and ultimately lower with contraception than with trapping, greater coverage (5000 ha vs 7500 ha) means that higher reduction in numbers across the landscape overall are possible with a perfect contraceptive and can match trapping with a 75% effective contraceptive.
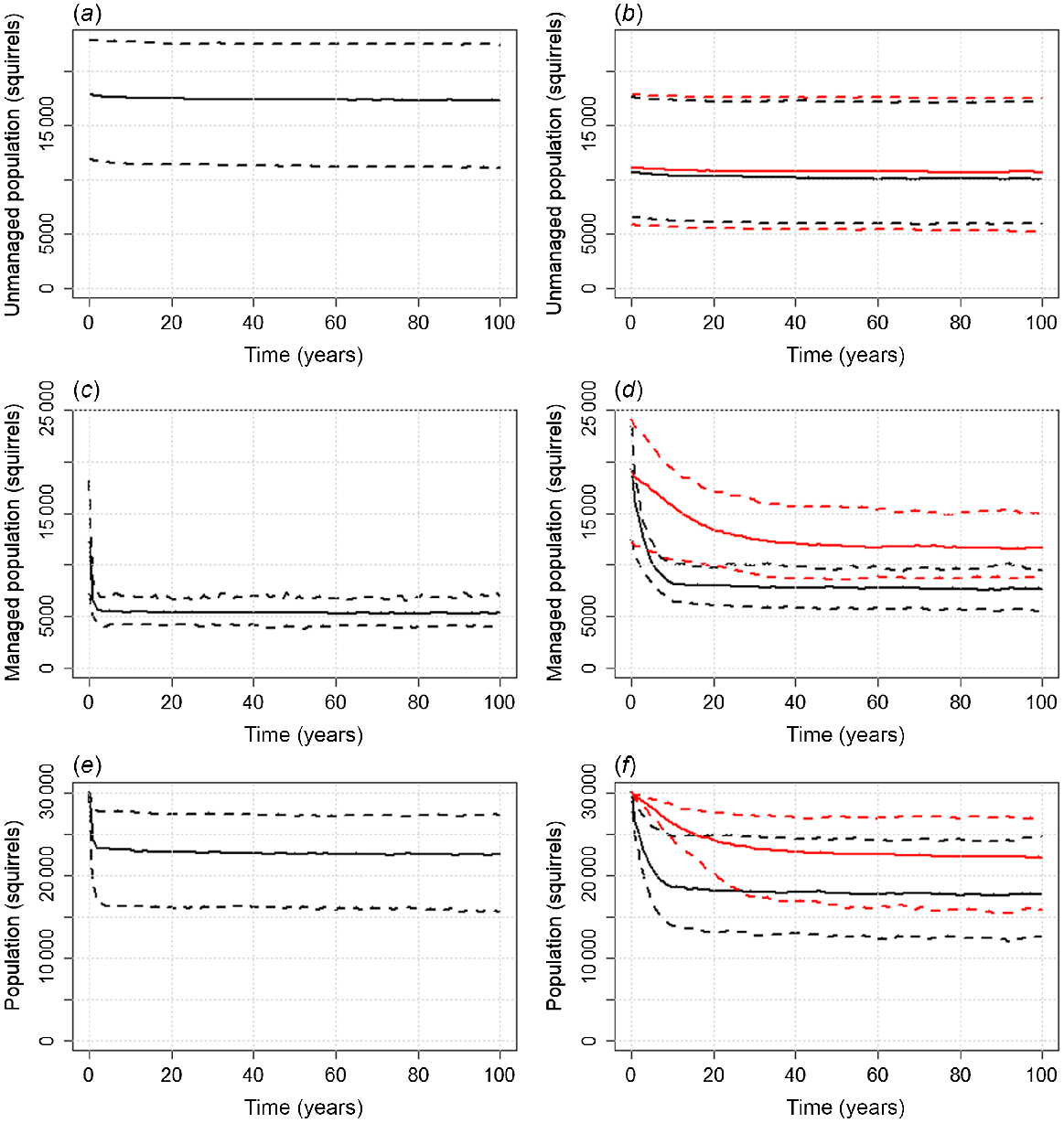
Discussion
In this study, we have used an existing spatially explicit individual-based modelling approach to compare the efficacy and relative costs (in terms of labour) of different management routines based on either trapping or oral contraception, accounting for practical constraints such as landowner engagement, which in turn affects the proportion of land where squirrel management can occur. As expected from previous studies, our findings showed that contraception alone is always slower than is removal and therefore (despite lower maintenance costs) generally requires a greater effort to achieve eradication. Following an initial 1-year population reduction (here achieved through trapping), contraception can maintain populations with less effort than does trapping, assuming a contraceptive efficacy of 90% or greater. However, importantly, when we account for potential differences in the acceptability of each approach, which will affect the spatial coverage of population control, contraception can maintain populations at a lower overall density than does trapping for similar effort, assuming a contraceptive efficacy of at least 75%, in agreement with Croft et al. (2021). For grey squirrel population management, this provides a minimum threshold for developing a contraceptive that should be at least 75% effective in inducing infertility.
Spatially explicit individual-based models such as the one we used in this study combine spatial variables and species’ life-history traits. The performance of such models is sensitive to the accuracy of the estimates of the life-history parameter input, such as fecundity, mortality, dispersal distance, and density, (Rushton et al. 1997, 2000). Several studies on the population dynamics of grey squirrels have produced reliable estimates of life-history traits of this species (reviewed in Tattoni et al. 2006) and have been used to underpin the model here, alongside empirical data on potential contraceptive delivery collected from field studies (Beatham et al. 2021). Our findings are consistent with those produced by other established models (e.g. Rushton et al. 1997, 2000; Lurz et al. 2001; Goldstein et al. 2016). For example, Goldstein et al. (2016) predicted a marked decrease in squirrel population abundance when 70% of all yearling and adult squirrels were culled either in the buffer area or in all patches for 5 years, with effects lasting over 20 years from the cessation of the cull program. Similarly, our model suggested that with a 68% reduction (0.25 traps/ha for 30 days/year) eradication could be achieved in 5 years. Goldstein et al. (2016) also suggested that small patches, not considered in the final model to avoid undue complexity, are important to invasion dynamics and species movement. This reflected findings on grey squirrel distribution in Italy, where small habitat patches, often smaller than 5 ha, have been shown to be important in grey squirrel metapopulation dynamics (Wauters et al. 1997; Martinoli et al. 2010; Bertolino et al. 2014). Both studies may explain the difference in findings between the current model and a previous one by Croft et al. (2021) that had not incorporated patches of <1 ha for squirrel population control. There have been limited landscape-scale control programs to validate the performance of our model against empirical observations. A notable exception is the large-scale eradication of grey squirrels on Anglesey in the UK, similar in size to our model study area, which was achieved in 15 years, with an average trapping effort of approximately 0.5 traps/ha deployed for 10 days each year (Schuchert et al. 2014). We predict that, at the same level of trapping as in Anglesey, initial reduction would be ~40% after 1 year but also that eradication would, on average, take a further 11 years to achieve (a median time of 12 years in total), at a much greater cost per squirrel removed. Indeed, the results from Anglesey, where grey squirrels were eradicated in 15 years (1998–2013), the total project expenditure per squirrel culled between 1998 and 2010 was £136, whereas in the last period of eradication, between 2011 and 2013, the project spent £5846/squirrel to remove the last remaining 26 animals (Bertolino et al. 2016).
Unlike previous studies, the current work explored a wide range of potential deployment strategies both for trapping and contraception. This provided a comparison among approaches but also highlighted constraints within each approach. For trapping, these constraints are dictated solely by trapping days, whereas the efficacy of contraceptive delivery to reduce squirrel numbers is affected by two factors, namely the number of hopper-days and the squirrel to hopper ratio, the latter being captured by hopper density. Similarly, the human dimension side of controlling populations of grey squirrels, particularly in urban areas where people appear to have strong views against lethal control, has been mentioned in previous studies, but its impact on squirrel population reduction at landscape scale has received little attention. For instance, in an Italian urban park where local citizens opposed culling, surgical sterilisation of hundreds of grey squirrels was employed to achieve a local eradication (Scapin et al. 2019). In the UK, community groups involved in grey squirrel control mentioned they would prefer alternatives to trapping and dispatching (Crowley et al. 2018; Shuttleworth et al. 2020). This attitude reflects the results of a recent survey on public acceptability of population control methods for grey squirrels, which found that contraception was the method preferred by 63% of the 3758 respondents (Dunn et al. 2018). The only study that explored how public attitudes may shape the outcomes of different methods of squirrel population control (La Morgia et al. 2017) used a structured decision-making technique based on a Bayesian decision network model to identify priority areas for management activities and to integrate the attitude of citizens towards various population control methods. The study concluded that, where citizens’ support was limited, this resulted in a reduced overall utility of interventions aimed at reducing squirrel numbers.
Compared to La Morgia et al. (2017), our study examined another aspect of human dimension, namely how the level of coordinated control at landscape scale, achieved by either culling or fertility control, might impact on population reductions of grey squirrels. A key result of this study is that the model suggested that when differences in social acceptance of each method were considered, reducing participation and, consequently, the spatial coverage of management, contraception maintained greater population reductions than did trapping with similar effort, assuming a contraceptive efficacy of >75%. The study reiterated the importance of landowners’ attitudes to squirrel control, but also suggested how different attitudes at landscape scale could still result in a significant reduction of population size. Interestingly, looking beyond population size, like La Morgia et al. (2017), we note that there are important differences between the spatial impact of trapping and contraception, which should be considered when defining management goals. For instance, if our goal is focused on reducing bark stripping, and if it is shown that small reductions in squirrel numbers are needed to alleviate most of the damage, then contraception applied over a larger area than trapping would provide a better option than would trapping. However, if greater reductions in squirrel numbers were required to alleviate tree damage or if the goal was focused on red squirrel conservation, then trapping, even when applied over a smaller area, would be the only option capable of producing the required reductions in managed patches.
Within this study, we make several simplifying assumptions that require further investigation. First, we assumed that landowners’ attitudes follow those of the wider public, but target surveys of these stakeholders will be required to understand the management landscape more accurately in terms of what may be possible and practical. In addition, further research should establish how spatial variations (clustering) in squirrel population control may also affect outcomes. It is also likely that differences in timing will also be important for landscape-scale management. As we have seen for partial coverage, reinvasion can be a limiting factor to maintaining low densities of squirrels. Intra-annual population dynamics (seasonal breeding cycles) and movement among patches may lessen the overall impact of management if not applied at the same time. Reducing the current time-step from annual to weekly would allow more realistic representation of the interplay between ecological process and management. We estimate cost in terms of labour, but note that much of this could be offset by involving volunteers and community groups; this may be particularly relevant for more socially acceptable methods such as fertility control. Citizen science and volunteer engagement with wildlife management have increased dramatically in the recent decades (e.g. Locke et al. 2019) and volunteers are already playing a significant role in implementing methods to manage overabundant wildlife. For instance, volunteer groups have been key to monitor reinvasion by grey squirrels on Anglesey in Wales (Shuttleworth et al. 2020). Labour cost is nevertheless reflective of total effort, irrespective of whether staff time has an actual monetary cost or is provided free of charge by volunteer groups. Trap density and trap-days (or hopper density and hopper-days plus cost of contraceptive) should also be added to the total costs. Our model makes the conservative assumption that fertility control only lasts for a single year. Whereas further information is needed on effective dosages and longevity of an oral contraceptive, should the effects of any contraceptive last for longer, then the relative costs of control, and therefore comparison with trapping, may substantially change.
In conclusion, the result that contraception can maintain populations at a lower density than does trapping for a similar effort (assuming a contraceptive efficacy of at least 75%) is likely to appeal to those that are opposed to lethal control. The second key result of this study, namely that where coordinated control cannot be maintained across the region, contraception will result in a greater population reduction than does trapping with a similar effort (assuming a contraceptive efficacy of >75%) would also be relevant to landowners and stakeholders opposed to culling. If these predictions can be confirmed in field trials, managers will have a greater flexibility in selecting population control methods that match stakeholders’ attitudes and expectations towards reducing grey squirrel impacts.
Notwithstanding the above developments, the approach used in this study could be replicated for other invasive and non-native species, particularly for widespread, charismatic wildlife, in contexts where public opinion is divided in terms of acceptance of different methods of population control. These species include several species of parakeets, deer, and raccoons (Procyon lotor) in many European countries, but also native species involved in human–wildlife conflicts such as urban wild boar (Sus scrofa) and several species of monkeys. The model could be used to illustrate to stakeholders the consequences of having a different level of acceptance of lethal and non-lethal methods on a local scale. Public attitudes towards wildlife control often depend on whether a species is regarded as ‘cute’ or as a ‘pest’. For instance, grey squirrels in many urban areas in the UK and in Italy are welcomed by tourists and citizens who oppose lethal methods of population control (Genovesi and Bertolino 2001), whereas other species such as commensal rodents are regarded as pests, with relatively little concerns on the methods employed to reduce their numbers (e.g. Morzillo and Mertig 2011). In instances where stakeholders’ attitudes might interfere with the ultimate aim of reducing the number of animals, the results of this study could be used as a basis to design communication plans aimed at shifting public attitudes towards methods that are more likely to deliver the desired outcomes.
Data availability
Summary model outputs underlying figures and associated model code files will be publicly available through the Supplementary material. All other data that support this study will be shared upon reasonable request to the corresponding author.
Conflicts of interest
Giovanna Massei is a guest Associate Editor of Wildlife Research but was blinded from the peer-review process for this paper. Otherwise, the authors declare no conflicts of interest.
Declaration of funding
This work was funded by the UK Government Department for Environment, Food and Rural Affairs (Defra) under project code WMWL1800.
References
Beatham SE, Goodwin D, Coats J, Stephens PA, Massei G (2021) A PIT-tag-based method for measuring individual bait uptake in small mammals. Ecological Solutions and Evidence 2(2), e12081.
| Crossref | Google Scholar |
Bertolino S, di Montezemolo NC, Preatoni DG, Wauters LA, Martinoli A (2014) A grey future for Europe: Sciurus carolinensis is replacing native red squirrels in Italy. Biological Invasions 16(1), 53-62.
| Crossref | Google Scholar |
Bertolino S, Lurz PW, Shuttleworth CM, Martinoli A, Wauters LA (2016) The management of grey squirrel populations in Europe: evolving best practice. In ‘The grey squirrel: ecology & management of an invasive species in Europe’. (Eds C Shuttleworth, P Lurz, J Gurnell) pp. 495–516. (European Squirrel Initiative: Stoneleigh Park, Warwickshire, UK)
Bremner A, Park K (2007) Public attitudes to the management of invasive non-native species in Scotland. Biological Conservation 139(3–4), 306-314.
| Crossref | Google Scholar |
Chen X, Hou X, Feng T, Han N, Wang J, Chang G (2022) Anti-fertility effect of levonorgestrel and/or quinestrol on striped field mouse (Apodemus agrarius): evidence from both laboratory and field experiments. Integrative Zoology 17, 1041-1052.
| Crossref | Google Scholar | PubMed |
Croft S, Chauvenet ALM, Smith GC (2017) A systematic approach to estimate the distribution and total abundance of British mammals. PLoS ONE 12(6), e0176339.
| Crossref | Google Scholar | PubMed |
Croft S, Aegerter JN, Beatham S, Coats J, Massei G (2021) A spatially explicit population model to compare management using culling and fertility control to reduce numbers of grey squirrels. Ecological Modelling 440, 109386.
| Crossref | Google Scholar |
Crowley SL, Hinchliffe S, McDonald RA (2018) Killing squirrels: exploring motivations and practices of lethal wildlife management. Environment and Planning E: Nature and Space 1(1–2), 120-143.
| Crossref | Google Scholar |
Dunn M, Marzano M, Forster J, Gill RMA (2018) Public attitudes towards ‘pest’ management: perceptions on squirrel management strategies in the UK. Biological Conservation 222, 52-63.
| Crossref | Google Scholar |
Genovesi P, Bertolino S (2001) Human dimension aspects in invasive alien species issues: the case of the failure of the grey squirrel eradication project in Italy. In ‘The great reshuffling: human dimensions of invasive alien species’. (Ed. JA McNeely) pp. 112–119. (IUCN Publications Services Unit: Cambridge, UK)
Gill R, Massei G, Pinkham R, Beatham S, Whitelaw B, McNicol C (2022) Grey squirrel control research – what new approaches may we anticipate for the future? Quarterly Journal of Forestry 116, 54-61.
| Google Scholar |
Goldstein EA, Butler F, Lawton C (2016) Modeling future range expansion and management strategies for an invasive squirrel species. Biological Invasions 18(5), 1431-1450.
| Crossref | Google Scholar |
Gurnell J (1999) Grey squirrels in woodlands: managing grey squirrels to prevent woodland damage. Enact 7, 10-14.
| Google Scholar |
Gurnell J, Lurz PWW, Shirley MDF, Cartmel S, Garson PJ, Magris L, Steele J (2004) Monitoring red squirrels Sciurus vulgaris and grey squirrels Sciurus carolinensis in Britain. Mammal Review 34, 51-74.
| Crossref | Google Scholar |
Hunold C, Mazuchowski M (2020) Human–wildlife coexistence in urban wildlife management: insights from nonlethal predator management and rodenticide bans. Animals 10(11), 1983.
| Crossref | Google Scholar | PubMed |
Jacoblinnert K, Jacob J, Zhang Z, Hinds LA (2022) The status of fertility control for rodents – recent achievements and future directions. Integrative Zoology 17, 964-980.
| Crossref | Google Scholar | PubMed |
Krause SK, Kelt DA, Van Vuren DH, Gionfriddo JP (2014) Regulation of tree squirrel populations with immunocontraception: a fox squirrel example. Human–Wildlife Interactions 8(2), 168-179.
| Google Scholar |
La Morgia V, Paoloni D, Genovesi P (2017) Eradicating the grey squirrel Sciurus carolinensis from urban areas: an innovative decision-making approach based on lessons learnt in Italy. Pest Management Science 73, 354-363.
| Crossref | Google Scholar | PubMed |
Lioy S, Marsan A, Balduzzi A, Wauters LA, Martinoli A, Bertolino S (2019) The management of the introduced grey squirrel seen through the eyes of the media. Biological Invasions 21(12), 3723-3733.
| Crossref | Google Scholar |
Liu M, Ren D, Wan X, Shen X, Zhao C, Xingan Wang Y, Bu F, Liu W, Zhang Z, Gao Y, Si X, Bai D, Yuan S, Zheng F, Wan X, Fu H, Wu X, Zheng A, Liu Q, Zhang Z (2023) Synergistic effects of EP-1 and ivermectin mixture (iEP-1) to control rodents and their ectoparasites. Pest Management Science 79(2), 607-615.
| Crossref | Google Scholar | PubMed |
Locke CM, Anhalt-Depies CM, Frett S, Stenglein JL, Cameron S, Malleshappa V, Peltier T, Zuckerberg B, Townsend PA (2019) Managing a large citizen science project to monitor wildlife. Wildlife Society Bulletin 43(1), 4-10.
| Crossref | Google Scholar |
Lurz PWW, Rushton SP, Wauters LA, Bertolino S, Currado I, Mazzoglio P, Shirley MDF (2001) Predicting grey squirrel expansion in North Italy: a spatially explicit modelling approach. Landscape Ecology 16, 407-420.
| Crossref | Google Scholar |
Martinoli A, Bertolino S, Preatoni DG, Balduzzi A, Marsan A, Genovesi P, Tosi G, Wauters LA (2010) Headcount 2010: the multiplication of the grey squirrel introduced in Italy. Hystrix 21(2), 127-136.
| Google Scholar |
Massei G, Cowan D (2014) Fertility control to mitigate human–wildlife conflicts: a review. Wildlife Research 41, 1-21.
| Crossref | Google Scholar |
Massei G, Cowan D, Eckery D, Mauldin R, Gomm M, Rochaix P, Hill F, Pinkham R, Miller LA (2020) Effect of vaccination with a novel GnRH-based immunocontraceptive on immune responses and fertility in rats. Heliyon 6, e03781.
| Crossref | Google Scholar | PubMed |
Mathews F, Kubasiewicz LM, Gurnell J, Harrower CA, McDonald RA, Shore RF (2018) A review of the population and conservation status of British mammals. A report by the Mammal Society under contract to Natural England, Natural Resources Wales, and Scottish Natural Heritage. Natural England, Peterborough, UK.
Morzillo AT, Mertig AG (2011) Urban resident attitudes toward rodents, rodent control products, and environmental effects. Urban Ecosystems 14, 243-260.
| Crossref | Google Scholar |
Quinn N, Kenmuir S, Krueger L (2019) A California without rodenticides: challenges for commensal rodent management in the future. Human–Wildlife Interactions 13, 212-225.
| Google Scholar |
Rushton SP, Lurz PWW, Fuller R, Garson PJ (1997) Modelling the distribution of the red and grey squirrel at the landscape scale: a combined GIS and population dynamics approach. Journal of Applied Ecology 34, 1137-1154.
| Crossref | Google Scholar |
Rushton SP, Lurz PWW, Gurnell J, Fuller R (2000) Modelling the spatial dynamics of parapoxvirus disease in red and grey squirrels: a possible cause of the decline in the red squirrel in the UK? Journal of Applied Ecology 37, 997-1012.
| Crossref | Google Scholar |
Rushton SP, Gurnell J, Lurz PWW, Fuller RM (2002) Modeling impacts and costs of gray squirrel control regimes on the viability of red squirrel populations. The Journal of Wildlife Management 66, 683-697.
| Crossref | Google Scholar |
Scapin P, Ulbano M, Ruggiero C, Balduzzi A, Marsan A, Ferrari N, Bertolino S (2019) Surgical sterilization of male and female grey squirrels (Sciurus carolinensis) of an urban population introduced in Italy. Journal of Veterinary Medical Science 81, 641-645.
| Crossref | Google Scholar |
Schuchert P, Shuttleworth CM, McInnes CJ, Everest DJ, Rushton SP (2014) Landscape scale impacts of culling upon a European grey squirrel population: can trapping reduce population size and decrease the threat of squirrelpox virus infection for the native red squirrel? Biological Invasions 16, 2381-2391.
| Crossref | Google Scholar |
Shi D, Wan X, Davis SA, Pech RP, Zhang Z (2002) Simulation of lethal control and fertility control in a demographic model for Brandt’s vole Microtus brandti. Journal of Applied Ecology 39, 337-348.
| Crossref | Google Scholar |
Shuttleworth CM, Robinson N, Halliwell EC, Clews-Roberts R, Peek H, Podgornik G, Stinson M, Rice S, Finlay C, McKinney C, Everest DJ (2020) Evolving grey squirrel management techniques in Europe. Management of Biological Invasions 11(4), 747.
| Crossref | Google Scholar |
Tattoni C, Preatoni DG, Lurz PWW, Rushton SP, Tosi G, Bertolino S, Martinoli A, Wauters LA (2006) Modelling the expansion of a grey squirrel population: implications for squirrel control. Biological Invasions 8(8), 1605-1619.
| Crossref | Google Scholar |
Wauters LA, Gurnell J, Currado I, Mazzoglio PJ (1997) Grey squirrel Sciurus carolinensis management in Italy – squirrel distribution in a highly fragmented landscape. Wildlife Biology 3(2), 117-124.
| Crossref | Google Scholar |