Investigating the impact of black rats (Rattus rattus) on the endemic and threatened avifauna of Christmas Island
Rosalie Willacy

A School of Earth and Environmental Sciences, The University of Queensland, St Lucia, Qld 4072, Australia.
B Parks Australia, Christmas Island, WA 6798, Australia.
C Research Institute of Environment and Livelihoods, Charles Darwin University, Casuarina, NT 0810, Australia.
Abstract
Invasive mammalian predators are a primary cause of extinctions, especially on islands. Baseline data on the impact of invasive mammalian predators are critical for deciding whether their eradication or control should be attempted, and for monitoring ecosystem changes over time if control does occur. Christmas Island is a tropical island with multiple invasive species, including two mammalian predators (black rats, Rattus rattus and feral cats, Felis catus). Black rats are known to prey upon island bird species (including their nests) elsewhere, but the severity of their impacts on Christmas Island birds is unclear. Describing these impacts would help managers decide whether rat control is warranted, especially as a cat eradication program underway on the island could conceivably result in increased rat density.
In this study, we quantify the impacts of rats on Christmas Island bird abundance and nesting success.
The abundance of four endemic forest bird species/subspecies (Christmas Island imperial pigeon, Ducula whartoni, emerald dove, Chalcophaps indica natalis, thrush, Turdus poliocephalus erythropleurus and white-eye, Zosterops natalis) was measured using transect surveys. Nest success was measured using remote sensing camera surveillance for one forest bird species (thrush) and one seabird species (red-tailed tropicbird, Phaethon rubicaudra westralis). We explored whether these measures were related to spatial variation in rat density and activity (measured by trapping and inkcards), the presence of other invasive species, habitat and seasonal variables.
Neither rat density nor activity explained the abundance of any forest bird species. Instead, seasonal and habitat features were more consistent predictors of bird abundance. White-eyes were more abundant near yellow crazy ant (Anoplolepis gracilipes) supercolonies. Nest success for thrushes and red-tailed tropicbirds was either not, or only very weakly, influenced by rats.
Black rats currently have little effect on the population sizes and nesting success of Christmas Island birds.
This study suggests that rat control is not currently a management priority, but ongoing monitoring of rat density, activity, and impacts is needed to ensure management can respond promptly if rat density or impacts change as cat control progresses towards eradication.
Keywords: black rat impact, forest birds, invasive species management, island, island birds, mesopredator release, nest predation, seabirds.
Introduction
Invasive mammalian predators are a primary cause of recent extinctions, especially on islands (Doherty et al. 2016). More than 140 bird, reptile and mammal extinctions that have occurred over the last 200 years have been attributed to invasive mammalian predators, and invasive mammalian predators threaten a further 596 endangered vertebrate species currently listed on the IUCN Red List (International Union for Conservation of Nature; Blackburn et al. 2004; Bonnaud et al. 2011; Medina et al. 2011; Szabo et al. 2012; Doherty et al. 2016; Dueñas et al. 2021). To address ongoing species declines, invasive species eradications have been attempted on numerous islands worldwide (DIISE 2015) with many conservation gains (Jones et al. 2016; Bolam et al. 2021). Eradications have succeeded mainly on small, uninhabited islands with reduced ecosystem complexity (DIISE 2015). However, knowledge gains and technological advancements mean that more challenging eradications are being attempted, including on larger, inhabited islands with multiple invasive species (Glen et al. 2013; Simberloff et al. 2018; DCCEEW 2022).
Invasive mammalian predators are embedded in ecosystems and interact with other native or invasive species present, often in complex ways (Zavaleta et al. 2001; Russell and Kaiser-Bunbury 2019). The impacts of each invasive mammalian predator species can vary with their abundance and behaviour, and with the tolerance of the prey species present, all of which may be dynamic across habitats, landscapes, or seasons. Invasive predators may cause detriment, or may provide ecological benefits, such as controlling potentially damaging native or invasive species (Rayner et al. 2007; Bergstrom et al. 2009; Dowding et al. 2009; Ritchie and Johnson 2009; Glen et al. 2013; Legge et al. 2017; Springer 2018; Ortega et al. 2021), providing food for native species (Carlsson et al. 2009; Travers et al. 2021), or pollination and dispersal for native plants or fungi (Vernes and McGrath 2009; Pattemore and Wilcove 2012; O’Rourke et al. 2020). Given these complexities, understanding the ecological role of invasive predators is critical for informing the need, priority and likely benefits of invasive species management.
Amongst rodents, black rats (Rattus rattus) have contributed to declines and extinctions of many terrestrial and seabird species on many islands (Towns et al. 2006; Jones et al. 2008; Szabo et al. 2012; IUCN 2017). For example, three thrush (Turdus sp.) subspecies extinctions that occurred on Norfolk, Lord Howe and New Caledonia’s Mare Island (IUCN 2017) were attributed to rat predation. Similarly, white-eyes (Zosterops sp.) have a record of extinction on rat-invaded islands (IUCN 2017). Black rats are adept nest predators (Harper and Bunbury 2015) and birds with easily accessible nests are particularly vulnerable. For example, pigeons, small burrowing and ground-nesting seabirds have suffered disproportionate declines and extinctions at the species and ultrataxon level (Szabo et al. 2012) due to invasive rat impacts (Towns et al. 2006; Jones et al. 2008). Rat densities on islands can be very high, with reports of up to 119 rats ha−1 (Harper and Bunbury 2015). Predation rates of island bird nests by rats can also be high; for example, 36% of Hawaiian Puaiohi (Myadestes palmeri) nests failed due to rat predation (Vanderwerf et al. 2014), while 22% of tropicbird nest failures on Europa Island were attributed to rat predation (Phaethon rubicaudra; Saunier et al. 2022). Rates of rat nest predation potentially vary due to differences in rat density, the availability of alternative resources, the presence of terrestrial land crabs (Woodward 2017), the sensitivity of bird species to rat impacts (with nesting location, egg size and shell density all potentially moderating impacts), and degree of adult nest defence (Jones et al. 2008).
In this study, we aimed to describe the impact of black rats on endemic and threatened avifauna of Christmas Island. As a result of the introduction of rats and other invasive species including another mammalian predator (cats, Felis catus) over the past 130 years, Christmas Island has lost at least four endemic vertebrate species – one reptile and three mammal species. Many of the remaining fauna are listed as threatened (James et al. 2019). The mechanisms driving the current and ongoing declines of several species on the island are complex and not well understood (Garnett et al. 2011; James and McAllen 2014), but black rats are listed as a key threat for several bird species (Algar and Johnston 2010). We used transect surveys to estimate the abundance of the endemic Christmas Island imperial pigeon (Ducula whartoni), emerald dove (Chalcophaps indica natalis), thrush (Turdus poliocephalus erythropleurus) and white-eye (Zosterops natalis), and explored the relationships between forest bird abundance, black rat density and activity, and other environmental covariates. We also monitored nests using motion-sensor cameras for two bird species, the Christmas Island thrush and the Indian Ocean red-tailed tropicbird (Phaethon rubricauda westralis), to examine the effects of rats on bird nesting success. Describing the nature and level of rat impacts on birds is key for informing the need for rat control on Christmas Island, and predicting the possible consequences if cat eradication leads to an increase in the severity of rat impacts.
Materials and methods
All methods referred to in this manuscript were approved by the University of Queensland Native/Exotic Wildlife and Marine Animals (NEWMA) animal ethics committee (SBS/481/16) and the Australian Government Director of National Parks.
Study site and bird conservation issues
Christmas Island (135 km2) is a basaltic sea mount capped with limestone, located within the equatorial tropics (10.448°S – 105.690°E) with a wet season driven by the north-western monsoon between approximately November and April, and a dry season driven by south-east trade winds from May to October (Director of National Parks 2014). The island geography is comprised of coastal cliffs and terraces and inland cliffs, and a central plateau of approximately 200 m elevation, with the highest point at 361 m. The central plateau is capped by basalt and largely covered by tall evergreen rainforest, coastal terraces are vegetated with deciduous forests, whereas inaccessible coastal cliffs are largely covered in scrub (Geoscience Australia 2014). Historic mining areas, where the closed forest vegetation and soil profile had been completely cleared leaving exposed limestone pinnacles, were later colonised by a dense layer of ground storey weeds, or scrub thickets of woody weeds (Walker et al. 2014). The Christmas Island settlement (population ~1800–3000) is in the north-eastern point of the island. Sixty-three percent of the island is designated as Christmas Island National Park, a Commonwealth reserve. Park management focuses on managing threats to the ecosystems (Director of National Parks 2014). Cat control has been underway since 2010, with that effort enhanced, and aiming for eradication, since 2020.
Christmas Island has high levels of endemism and high rates of extinction. At least 253 species are endemic including 21 vertebrates, of which six are now extinct or extinct in the wild – three reptile and three mammal species, including two endemic rats (Rattus macleari and Rattus nativitatis; Wyatt et al. 2008), while most of the remainder are listed as threatened (James et al. 2019). Christmas Island thrush (Endangered, Environment Protection and Biodiversity Conservation Act 1999; EPBC Act 1999), white-eye (Near Threatened; IUCN Red list), emerald dove (Endangered: EPBC Act 1999), imperial pigeon (Near Threatened; IUCN Red list) and the Indian Ocean red-tailed tropicbird (recommended Vulnerable, EPBC Act 1999; DCCEEW 2022) are believed to be threatened by black rats, and also by cats.
Transect surveys of bird abundance
To investigate relationships between forest bird abundance and invasive species, forest birds were surveyed along 22 fixed transects located across the island (Fig. 1). Transects followed forest tracks and were 800 m long. Each transect was surveyed three times per survey session. Three survey sessions were completed at 4-monthly intervals between April 2018 and February 2019 (see Fig. 1 for timetable). These sessions covered both dry and wet season conditions. The number of birds counted (seen and heard) for each species during each transect was the variable of interest, and was attributed with the transect identity, season, habitat type, year and season covariates (Table 1).
The location and timeframes of bird survey transects, thrush nest study sites, red-tailed tropicbird (RTTB) nest study sites, rat activity index (RAI) ink card monitoring transects and rat density monitoring sites in relation to Christmas Island habitat.
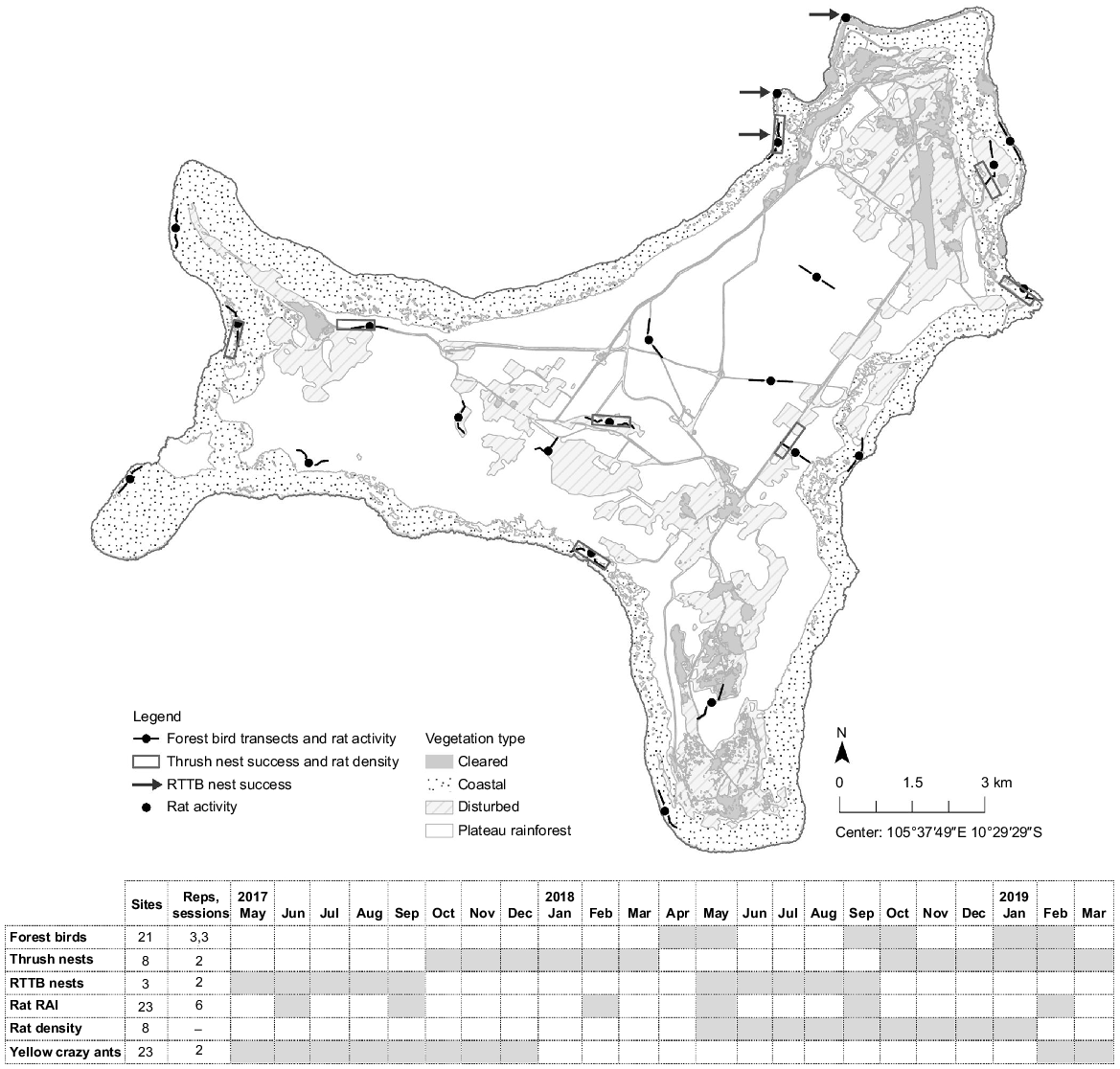
Candidate variables | Bird abundance | Nest success | |
---|---|---|---|
Random effects | Transect | Site (Thr) | |
Time | Season (wet; dry) | Year (Thr; RTTB) | |
Habitat type | Coast, plateau rainforest, disturbed | Coast, plateau rainforest, disturbed (Thr) Site 1; 2; 3 (RTTB) | |
Microhabitat | Leaf litter depth Shrub height Shrub density Tree density Canopy height Canopy cover Shannon’s species diversity index | Nest height (m; Th) | |
Invasive species | Rat relative activity Yellow crazy ant abundance Yellow crazy ant super colony present/adjacent or absent | Rat density (Thr) Rat relative activity (Thr; RTTB) Rat activity at nests (RTTB) Yellow crazy ant abundance (Thr; RTTB) Yellow crazy ant super colony present/adjacent or absent (Thr; RTTB) |
Nest monitoring
To estimate the impacts of rats (and other invasive species, including cats and yellow crazy ants) on bird nesting success, nest outcomes were monitored for two focal bird species, a forest exemplar (Christmas Island thrush) and a ground-nesting seabird exemplar (Indian Ocean red-tailed tropicbird).
For thrush, active nests were searched for in or near eight of the 22 bird transects (Fig. 1) between October and March in 2017–2018 and 2018–2019, by following behavioural cues of birds and by visually searching for nests within the branches and canopies of shrub and trees. For tropicbirds, sitting adults were found between May and September in 2017 and 2018 (see Fig. 1 for timetable) at three known breeding sites. Two of the sites were high density cliff-terrace breeding colonies, whereas the third site consisted of a lower density of scattered nests within cliff-top cavities located along a 500 m stretch of coastline (Fig. 1).
Once found, nests were monitored using infra-red motion-sensor cameras (Ltl Acorn 3510As, see Supplementary Table S1 for settings). Nests found at earlier stages i.e. nest building, laying and egg stages were given monitoring priority. Where possible, nests were selected at least 50 m apart to increase the independence of nest observations. Each nest was attributed with species, year and stage found. For thrush, the number of eggs and/or chicks present, the height of the nest from the ground and the tree species the nest was in were also recorded. Red-tailed tropicbirds only lay a single egg on the ground.
Camera data was analysed manually, and nest outcome (1: success, 0: failed) was determined for each nest, and for thrush nests, each individual egg or chick in the nest. Nests were classified as successful when fledglings left the nest. The nest was deemed to have failed when eggs were abandoned, chicks were abandoned, or nests were preyed upon as observed using camera data before the expected fledgling date (thrush: 28 days; red-tailed tropicbirds: 126 days). Where it could be determined, the stage and cause of failure were recorded; if unable to be determined, cause of failure was recorded as unknown. If the outcome of the nest could not be determined (e.g. the camera failed or moved) but sufficient time had passed so that nests could have successfully fledged, nest outcomes were recorded as unknown.
Environmental covariates
Each bird survey transect was attributed with a range of environmental covariates (Table 1).
The habitat type and average canopy height of each transect was derived from an existing vegetation map (Geoscience Australia 2014). Vegetation was grouped into broad habitat types: (1) coastal forest; (2) plateau rainforest; and (3) disturbed. Disturbed habitat included bare ground, infrastructure, mining, mixed weed and pioneer species, regrowth, and mine rehabilitation areas. Bird surveys were not undertaken within residential areas.
Microhabitat features were measured at two 10 × 10 m plots per transect, placed within 50 m of the transect line, each centred at randomly chosen locations at least 50 m apart. Shrubs (<3 m) and trees (>3 m) in the quadrats were counted, their height was measured with a clinometer, their diameter was measured with a tape at 1.3 m above the ground, and species were identified where possible. Data on shrub and tree stem density and heights were averaged across the two plots at each transect, and a Shannon’s species diversity index was calculated using R package ‘vegan’ (Oksanen 2020; RStudio Team 2021). In addition, the proportion of ground cover was categorised and estimated visually between 0 and 0.5 m from the ground and canopy cover was measured using a densitometer at five 1 × 1 m quadrats randomly placed within the larger plot.
Rat density estimates were generated for a subset of eight fixed forest bird transects/thrush nest monitoring study sites. Density estimates could not be generated for red-tailed tropicbird nest study areas due to site constraints (residential areas and steep cliff-tops). Rat density was measured using cage traps. The trapping array consisted of ~40 cage traps (wire cages 20 × 20 × 35 cm; Sheffield Animal Traps) set 20–25 m apart in a grid format, as measured using GPS (Global Positioning System) and hip-chain. Traps were elevated approximately 0.5–1.5 m above the ground on logs, limestone pinnacles and against trees with rat runs to avoid crab interference. Traps were lured with a peanut-butter oat mixture sprayed with Coopex™ to deter ants. Surveys were completed over 5 or 7 nights depending on capture rates; survey length was extended if recapture rates were less than 30% after five nights. All captured rats were marked using a unique hair snip, and gender, weight and tail length measurements were taken.
Density estimates were calculated using spatially explicit capture recapture analyses (Efford 2017) in R (RStudio Team 2021). The trapping area included a 100 m habitat mask applied beyond the grid where detected rats could reasonably range (Harper and Bunbury 2015; Efford 2021) using the ‘maptools’ package (Bivand 2021). The shoreline of Christmas Island (Geoscience Australia 2013) was used to exclude ocean from the habitat mask. Null models were run using the half-normal detection function and were tested for sex-dependent detection and learned trap responses (Efford 2017). Highest supported density models for each site were selected using the Akaike criterion (Burnham and Anderson 2002).
Rat activity was measured at or near all forest bird survey transects and red-tailed tropicbird nest monitoring sites using ink card monitoring (22 sites). Although rat activity is related to density, this relationship can vary spatially, and in response to resource availability. Rat activity could indicate predation risk for bird nests more strongly than rat density, for example if it has a greater bearing on nest encounter rates, or reflects rat hunger (Oppel et al. 2014). At each bird survey or nest monitoring site, 10 detector tunnels were set in a 500 m linear transect, with each tunnel spaced 50 m apart and at least 50 m from roads or tracks (Gillies and Williams 2013). Tunnels were 50 cm lengths of 100 mm PVC pipe with wire crab excluders installed at each end. They were fastened, approximately 0.5–1 m above the ground, to limestone pinnacles, vegetation, or logs. Black Trakka™ (Gotcha Traps) ink cards were fixed in tunnels using wire pins and were lured using a peanut butter and oat mixture sprayed with Coopex™. Rat activity was measured over 3-night surveys and occurred before density surveys at each site where density surveys were completed (see Fig. 1 for timetable). Presence/absence (1, 0) of rats at each tunnel was recorded at the completion of each 3-night survey. For each site, the proportion of detector tunnels with rat detections was calculated. Where cards were interfered with by land crabs (i.e. removed from the tunnels) during the survey period, they only contributed 0.5 to the total proportion (Gillies and Williams 2013). Rat activity was averaged across replicates at each transect with nest monitoring sites.
In addition to the site-level measure of rat density and activity described above, cat and rat activity indices were generated for individual red-tailed tropicbird nest sites using the 2018 sample of nest camera data only. Predator activity at nests was determined by dividing each 24 h period of camera trap data into 15-min intervals for which cat or rat presence was recorded (Rendall et al. 2014). The predator activity index equated to the number of 15-min intervals that had cat or rat presence as a proportion of the total number of 15-min intervals recorded at that nest.
Although our focus was rat impacts, we also considered the potential influence of other invasive species on bird abundance and nest success. Cat density is spatially uniform across the island (McGregor and Potts 2019) and so could not be examined, but the density of yellow crazy ants varies substantially. Previous work indicated that yellow crazy ants affect thrush and emerald doves, potentially by killing hatchlings and restricting access to food resources (Davies et al 2008). Yellow crazy ant abundance estimates were derived from ant count transects completed by Parks Australia in 2017 and 2019; counts were the sum of ants recorded at 11 survey points along a 50 m transect, with transects undertaken across the island. We used the counts from survey points closest to the bird transects and nest monitoring sites. Yellow crazy ants can form super colonies, which are areas of high ant abundance made up of several nests and queens. Survey sites were classed as being near super colonies (1) if they occurred within 50 m of an ant transect with ant abundances exceeding 37 at all survey points, visual signs including excessive ant activity on the forest floor and trees, or the presence of crab carcasses indicating super colony level impacts. Survey sites were classed as being close to super colonies (0.5) if sites occurred within 50 m of transects that met these criteria; or classed as super colony absence (0) if there was no sign of super colonies within 100 m of transects.
The influence of rats on bird abundance
We examined whether bird abundance was related to rat density and activity, as well other environmental variables including yellow crazy ant presence and density (Table 1). The abundance of each bird species was modelled separately as a function of the candidate variables (Table 1) using generalised linear mixed models and the package ‘glmmTMB’ (Brooks et al. 2017; RStudio Team 2021). The abundance of the bird species at the survey site was the response variable in all species models except for emerald dove for which presence/absence was used, as the counts were low for this species. Gaussian error distributions were assumed for all species except emerald dove for which a binomial error distribution with a logit link function was used. Survey transect was specified as a random effect for all species. Alternative candidate model sets were built to avoid collinear terms (Pearson’s r > 0.6) using the GGally package (Schloerke 2020). Foliage projective cover (FPC) was collinear with canopy height (r = 0.67) so model subsets contained either one of these variables but not both. The ratios of residual deviance to residual degrees of freedom were assessed to check each model for over-dispersion. Model assumptions were verified by plotting residuals versus fitted values.
We used an information-theoretic approach for the model selection process (Burnham and Anderson 2002). First, the importance of model terms was determined using backwards selection. All possible combinations of the highest-ranking terms were then explored (ΔAICc value <2 and excluding collinear variables) using the dredge function in the MuMIn package v1.43.17 (Barton 2020). Models with an ΔAICc value <2 were considered to have similar support (Grueber et al. 2011). To illustrate the relative significance of terms, continuous variables were standardised to lie between 0 and 1 and the selected models were run using standardised variables. Model averaging was then performed across the 95% confidence set of competitive models based on the Akaike Information Criterion value (AICc) corrected for small sample size using the MuMIn package v1.43.17 (Barton 2020). Variables in the full averaged model set with a 95% confidence interval that did not overlap zero were considered to have an influence on the response variable.
The influence of rats on bird nest success
We examined thrush nest success (success/failure) in relation to rat density and activity, as well as yellow crazy ant abundance, year and habitat variables (Table 1), using generalised linear mixed models (binomial family with a logit link function) and the ‘lme4’ package (Bates et al. 2015). Transect was included as a random effect and year and nest height were included as fixed effects. We examined nest success in red-tailed tropicbirds with two separate generalised linear models (binomial family with a logit link function). The first model (a) included site or year as fixed effects and evaluated the effect of rat activity measured at the site, yellow crazy ant abundance and yellow crazy ant super colony presence, on nest outcome. The second model (b) included site as a fixed effect and evaluated the effect of rat activity measured at the nest level by nest cameras on nesting success using the 2018 subset of red-tailed tropicbird nest monitoring data only (Table 1). The information-theoretic approach, as described above, was used to quantify model support. Model derived estimates of nest survival are presented with 95% confidence intervals.
Results
Patterns of bird abundance
Two hundred and fifteen bird surveys were completed at 22 transects in three survey sessions over 3 years. Thrush (in 214 surveys), imperial pigeon (in 211 surveys) and white-eye (in 215 surveys) were recorded on all or almost all surveys, whereas emerald dove were less commonly observed (in 156 surveys). Goshawk (in 13 surveys), swifts (in 88 surveys) and water hen (in 1 survey), occurred infrequently and were not included in analyses.
Rat activity was included in the top models explaining white-eye, imperial pigeon and emerald dove abundance, but not thrush abundance (Fig. 2; Table S2). There were fewer white-eye, and slightly more imperial pigeon and emerald dove where rat activity was higher, but these relationships were not significant (Fig. 2; Table S3). Yellow crazy ant abundance was included in the top models for all species except for the emerald dove, though was only significant for the white-eye (Fig. 2; Tables S2, S3). Where yellow crazy ant super colonies were present there were significantly more white-eye (Table S3; Fig. 2). There were also more thrush counted where yellow crazy ant super colonies were present, while imperial pigeon were less abundant when yellow crazy ant abundance was higher and if yellow crazy ant super colonies were present (Fig. 2; Table S3). None of these relationships were significant.
The 95% confidence set of model averaged coefficient estimates of influential predictor variables for Christmas Island bird abundance based on the generalised linear mixed models. Significant terms are those for which confidence intervals do not cross zero. Reference levels for categorical predictor variables are yellow crazy ant super colony absence, dry season and coastal habitat.
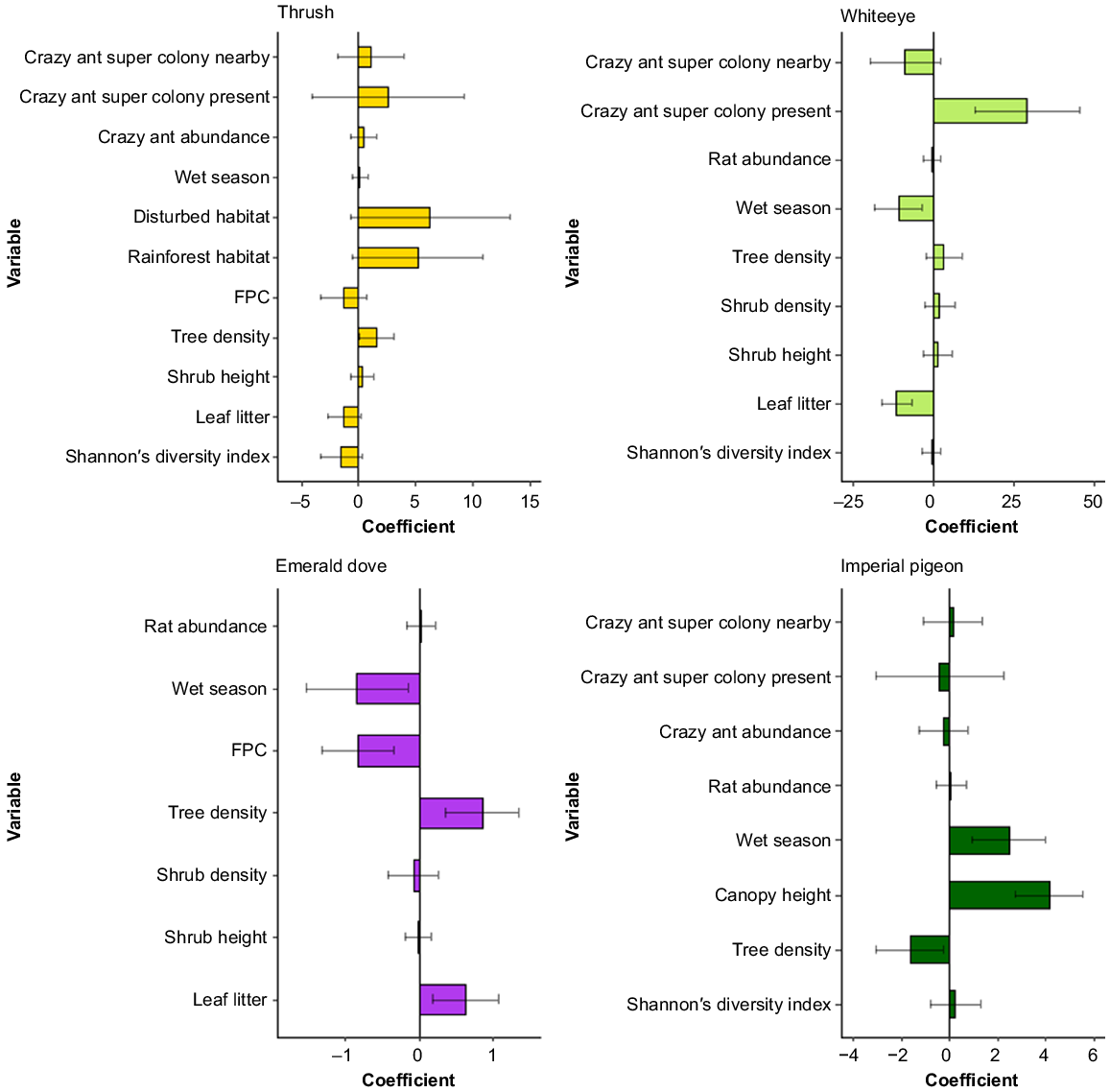
Season, habitat and microhabitat covariates were consistently included in the most parsimonious models of bird abundance, and often as significant predictors (Fig. 2; Tables S2, S3). Thrush were significantly more abundant with increasing tree density (Figs 2, S3). White-eye were significantly less abundant during the wet season, and significantly more abundant with increasing leaf litter cover (Figs 2, S3). White-eyes were also more common with increasing tree density and leaf litter cover, and were significantly less abundant with increasing canopy cover and during the wet season (Figs 2, S3). Finally, imperial pigeon were significantly more abundant with increasing canopy height and during the wet season, but were significantly less abundant with increasing tree density (Figs 2, S3).
Nest monitoring
We found 91 thrush nests in a range of tree species (Table S4). Seven nests were not active following camera installation, and the outcome was undetermined for a further 10 nests (these 17 nests were removed from analyses). Of the remaining 74 nests, 29 (39%) fledged 56 young (Fig. 3). Failure was due to nest damage, broken eggs, abandonment, and predation. Goshawks (Accipiter fasciatus natalis) were the most common predator of both chicks (n = 22) and eggs (n = 3), followed by rats (n = 2 chicks; six eggs), while a giant centipede (Scolopendra subspinipes) preyed on one chick. Causes of failure were undetermined for 20 individuals from 14 nests (Fig. 3). Predation of chicks occurred on average within 9.3 days of hatching.
Outcomes for individuals and nests over two breeding seasons for thrush (2017–2018 and 2018–2019) and red-tailed tropicbird (2017 and 2018) on Christmas Island, Indian Ocean. Totals include the number of individuals for which the outcome was unknown (‘unknown’), the number of successes (‘successfully fledged’) as well as failed nests by causes of failure.
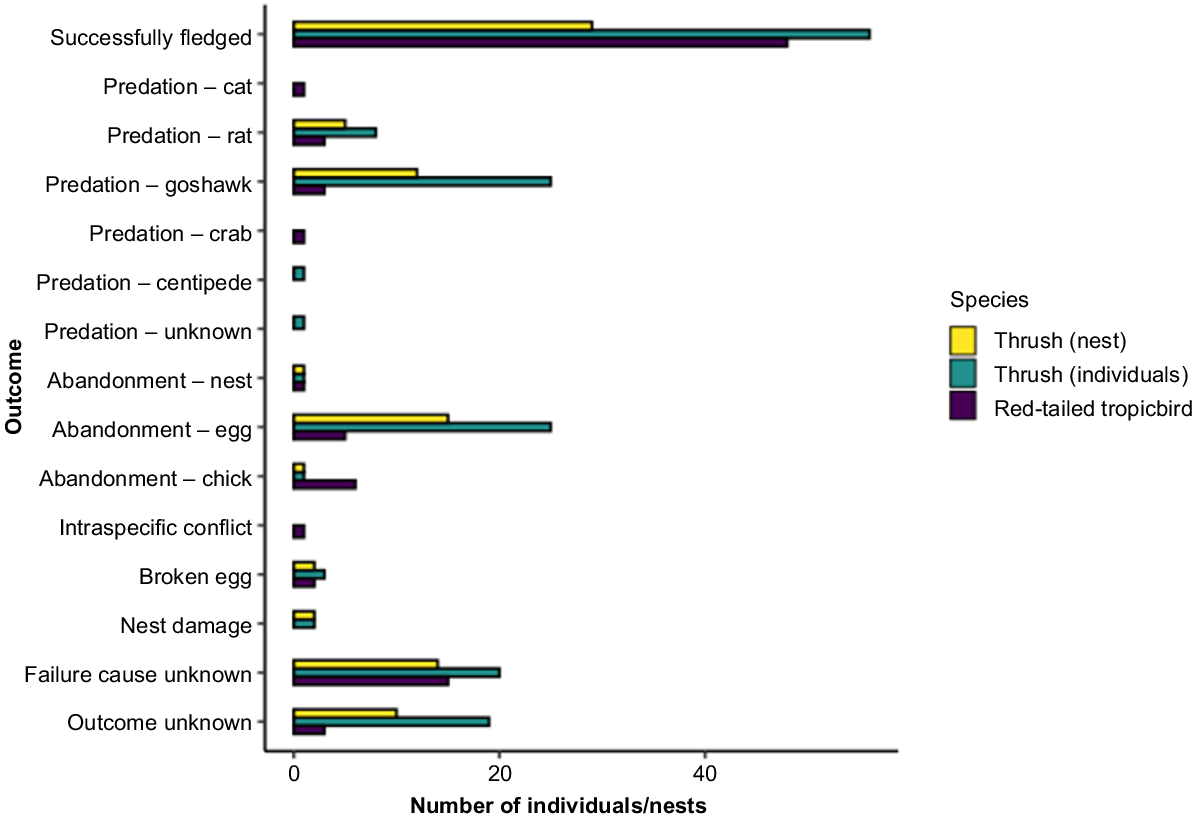
We monitored 89 red-tailed tropicbird nests. The outcome was undetermined for two nests and these were removed from subsequent analyses. Of the remaining 87, 46 (53%) fledged young (Fig. 3). Nest failures were mostly due to unknown causes, followed by abandonment at the egg phase, broken eggs, and intraspecific conflict. Goshawks and rats were the main predators (n = 3 chicks), followed by cats (n = 1 chick) and crabs (n = 1 chick; Fig. 3). Predation of chicks occurred on average 8.6 days after hatching.
Thrush nest success was positively and significantly related to rat density (Fig. 4; Tables 2, S5). The relationship between rat density and nest survivorship was positive. Rat activity was not related to nest survivorship (Table 2).
The predicted relationships between probability of successful nest outcome and rat activity for the thrush and red-tailed tropicbirds at the site (a) and nest (b) level. Rat activity did not rank highly in models describing nest survivorship for red-tailed tropicbirds at the site level and is shown here to illustrate there was no trend.
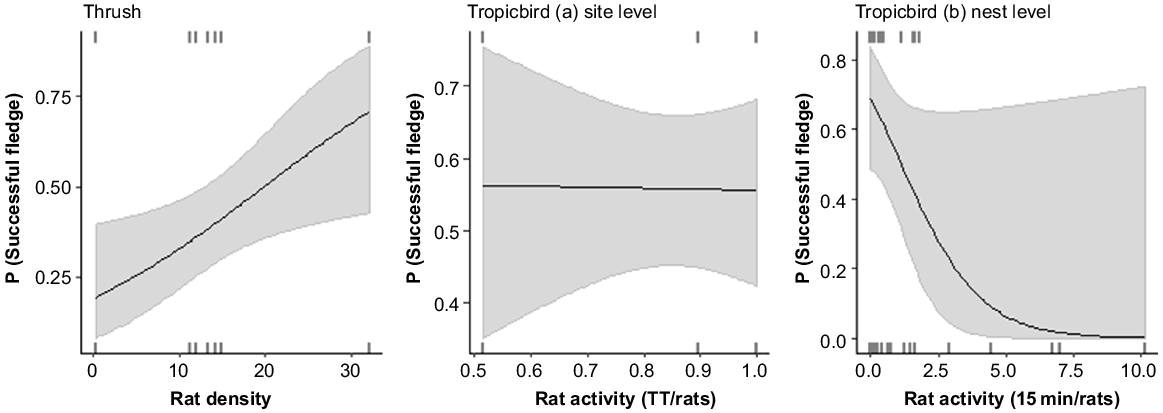
Models | K | AICc | AICc Wt | LL | |
---|---|---|---|---|---|
Thrush | |||||
Rat density | 3 | 97.2 | 0.36 | −45.4 | |
Rat density + Yellow crazy ant abundance | 4 | 98.6 | 0.19 | −45.0 | |
Rat density + Nest height | 4 | 98.9 | 0.16 | −45.1 | |
Rat density + Yellow crazy ant super colony | 4 | 99.0 | 0.15 | −45.2 | |
Rat density + Year | 4 | 99.2 | 0.14 | −45.3 | |
Red-tailed tropicbirds a) rat activity measured at the site level | |||||
Site | 3 | 116 | 0.6 | −54.9 | |
Site + Year | 4 | 118 | 0.2 | −54.7 | |
Year | 1 | 120 | 0.1 | −59.0 | |
Null model | 2 | 121 | 0.0 | −58.5 | |
Yellow crazy ant abundance | 2 | 122 | 0.0 | −58.7 | |
Yellow crazy ant super colony | 2 | 122 | 0.0 | −58.9 | |
Rat activity | 2 | 122 | 0.0 | −59.0 | |
Red-tailed tropicbirds b) rat activity measured at the nest level | |||||
Rat activity nest | 2 | 50.5 | 0.70 | −23.1 | |
Rat activity nest + Site | 4 | 54.5 | 0.10 | −22.7 | |
Site | 3 | 55.8 | 0.10 | −24.5 | |
Null model | 1 | 55.9 | 0.00 | −26.9 |
Site was included as a random effect for thrush and a fixed effect for red-tailed tropicbirds.
K is the number of estimated parameters including the intercept; AICc, corrected Akaike information criterion; AICc Wt, weights for AICc; LL, log likelihood.
Red-tailed tropicbird nest success was not related to rat activity measured at the site level (Fig. 4; Table 2). Models containing site and year were the highest ranked (Table 2), although no individual sites or years had significantly different nest survivorship (Table S5). However, when rat activity measured at the nest level (2018 data only; model b) was analysed, models containing this variable ranked highest (Table 2). Where rat activity at the nest was lower, nest success was higher; although this relationship was marginal (P = 0.07; Fig. 4; Table S5).
Discussion
This study focused on assessing the impact of rats on endemic Christmas Island birds. The goal was to improve our understanding of the extent and consequences of rat predation on the avifauna, and therefore inform the need for rat control, especially as an ongoing cat control program could plausibly result in increased rat density. There are two key results. First, spatial variation in the abundance of forest birds was not strongly affected by the density or activity of rats; instead, bird abundance was best explained by a combination of seasonal and habitat factors, and another invasive species, yellow crazy ants, had some effect for one bird species, the white-eye. Second, rat predation rates on both thrush and red-tailed tropicbird nests were low, and there was no persuasive evidence that higher rat activity led to increased rat predation at either thrush or red-tailed tropicbird nests. Taken together, these results suggest rats currently have a relatively small effect on the population size and nesting success of both forest birds and seabirds on Christmas Island. Below, we first discuss the impacts of other invasive species on Christmas Island birds, before considering why rats have little effect on these birds, and the implications of these results for the future management of cats and rats on Christmas Island.
Impacts of other invasive species on Christmas Island birds
Cat predation was not observed at any thrush nests and was observed at only one red-tailed tropicbird nest. However, our study did not capture an important thrush life-history stage extremely vulnerable to cat predation. Juvenile thrushes do not have well developed feathers and remain on the ground where they rely on parental feeding for approximately 30 days post-fledging (Davis et al. 2008). Diet studies show that cats prey upon thrushes on Christmas Island, and juveniles are likely to make up a significant proportion of this. Cats were significant predators of red-tailed tropicbirds before the cat eradication program began on Christmas Island (prior to 2010; Willacy et al. 2021), as has been observed elsewhere (Ratcliffe et al. 2010; Debrot et al. 2014; Vanderwerf and Young 2014; Boeken 2016; Luna et al. 2018).
White-eyes were significantly more abundant where yellow crazy ant super colonies were present. Davis et al. (2008) also found that white-eyes were more abundant in yellow crazy ant invaded forest on Christmas Island. Contact with yellow crazy ants is less likely for white-eye than for other birds as they rarely spend time on the ground where ant densities are highest, and their rapid flying behaviour while foraging may afford few opportunities for yellow crazy ants to swarm onto the birds. White-eyes may also benefit from the increased availability of scale insects caused by yellow crazy ants (Davis et al. 2008). The effect of yellow crazy ants, or centipedes, on nesting success could not be quantified because invertebrates do not typically trigger infra-red motion sensors. However, nest abandonment was observed in several thrush and red-tailed tropicbird nests with high ant activity when returning to collect cameras. Nest abandonment affected 18% of thrush nests and 14% of red-tailed tropicbird nests at the nest building (for thrush), egg laying and incubating stages, and may have been partly due to yellow crazy ant impacts (Davis et al. 2008; Varela et al. 2018; Willacy et al. 2021). Nest cameras inadvertently recorded a giant centipede preying upon a thrush chick; an impact previously undescribed on Christmas Island. On Phillip Island, New South Wales, native centipedes were the principal source of black-winged petrel (Pterodroma nigripennis) nestling mortality, with annual rates of predation varying between 11.1% and 19.6%, equating to 3724 nestlings (Halpin et al. 2021). Thus, further investigation of centipede impacts on declining Christmas Island birds is warranted.
There has been some loss of coastal terrace red-tailed tropicbird nesting habitat caused by the spread of the invasive vine Antigonon leptopus (Director of National Parks 2014), which smothers and destroys the native vegetation that the red-tailed tropicbirds nest beneath (Willacy et al. 2021). Invasive vines may contribute to nest failure if adults are selecting lesser quality nesting sites in more exposed locations. Defoliation of coastal vegetation at nesting colonies was observed as a result of late dry season sea spray events, and abandonment of nests and chick mortality occurred where nests or chicks were exposed. Intraspecific competition resulting in chick death was also observed when chicks moved into adjacent nest sites in response to vegetation defoliation caused by spray events and sun exposure.
Impacts of rats on Christmas Island birds
Spatial variation in black rat density and activity does not relate to spatial variation in the abundance of forest bird species on Christmas Island. In other words, bird abundance is not reduced in areas where rats are most abundant. Furthermore, while rats have been confirmed as a nest predator of thrush, the predation rate was minor compared to other sources of mortality. Rates of rat predation were lower (5% of nests) than recorded for species in the same family studied elsewhere. For example, black rats preyed upon 36% of critically endangered Hawaiian Puaiohi (M. palmeri) nests and some adult females (Vanderwerf et al. 2014), and predation by black rats is listed as a contributing factor in the extinction of several Turdus subspecies, including the Norfolk Island thrush (T. poliocephalus poliocephalus), Lord Howe Island thrush (T. poliocephalus vinitinctus) and New Caledonia’s Mare Island thrush (T. poliocephalus mareensis (IUCN 2017). Rat predation rates of red-tailed tropicbird nests on Christmas Island are also low (3%). While red-tailed tropicbird nesting success was slightly lower with increasing rat activity at nests, this effect was not significant. Again, this result contrasts with reports of rat predation on tropicbird nests elsewhere, which are extremely varied (Fleet 1972, 1974; Wirtz 1972; Ringler et al. 2015; Flores et al. 2017; Luna et al. 2018; Saunier et al. 2022).
There are four potential, non-exclusive reasons for why black rats have little impact on birds of Christmas Island. First, black rats occur at lower densities (4.2–32 rats ha−1) on Christmas Island than reported on other tropical islands. On Europa, where nesting success of red-tailed tropicbirds was <10%, black rat densities ranged from 8 to 65 ha−1 (Ringler et al. 2015) while on Kure Atoll, nest success of red-tailed tropicbirds was 0–11% and Pacific rat densities (R. exulans) ranged from 50 to 185 ha−1 (Wirtz 1972). Rats frequently visited tropicbird nests on Europa. On average, there were 0.34 visits h−1 nest−1 (Ringler et al. 2015). On Christmas Island, rat activity at nests was lower, averaging 0.04 visits h−1 nest−1 (range 0–0.40 h−1 nest−1) which could help to explain lower rat predation rates. However, note that on Europa there was no correlation between nest fate and the number of rat visits (Saunier et al. 2022).
Second, the availability of other plant and invertebrate food resources on Christmas Island may be higher than on other islands (especially low lying and structurally simple coral atoll ecosystems), reducing the need for rats to target bird nests for food (Harper and Bunbury 2015). Black rats are omnivorous (Harper and Bunbury 2015). Diet analyses have shown that rats on Christmas Island largely eat vegetative material and invertebrates including crustaceans (C. Pink 2017, pers. comm.). Ochrosia sp., Pandanus sp., Scaveola taccada and Terminalia catappa occur on Christmas Island, and rats are known to consume these plants on islands elsewhere (Abe 2007; Fleet 1972). Many endemic macroinvertebrates (27 mollusc, 15 crustacean and 150 insect species) are also known to occur on Christmas Island (James et al. 2019). If there are abundant alternative food resources that are less costly and dangerous to find and forage upon, rat predation on birds may be low (Atkinson 1985; Towns et al. 2006; Jones et al. 2008). Fleet (1972) observed rats attacking red-tailed tropicbirds from behind and recorded most rat kills (85%) on chicks <8 days old, before they had become aware of their surroundings. This suggests that predation becomes more difficult for rats as red-tailed tropicbirds become increasingly more active and aggressive, unless they are starved or otherwise stressed. Saunier et al., observed rats repeatedly visiting nests until nestlings were accessible to attempt predation (2022). Seasonal changes in food availability may therefore influence rat predation rates. For example, if rats are resource stressed, they may be more likely to attempt predation of chicks (Zarzoso-Lacoste et al. 2011). On Kure Atoll, it was suggested that a seasonal failure of the preferred food (S. taccada fruit and foliage) due to storm damage caused increased red-tailed tropicbird nest predation by rats (Fleet 1972).
Third, birds on Christmas Island have co-evolved with nest predators and may have adaptations to reduce or offset rat predation rates (Atkinson 1985). Maclear’s rat (R. macleari) and the bulldog rat (Rattus nativitatus) were native to Christmas Island, becoming extinct in 1903 and 1910 after black rats were introduced, most likely from the co-introduction of a novel pathogen (Wyatt et al. 2008). The ecology of these rat species is poorly known, but it is possible that the Maclear’s rat, with its large size and powerful teeth (Tidemann 1989), was a predator of bird nests. Christmas Island also has a diverse and abundant land crab fauna, and crabs are known to prey on bird eggs and nestlings. Only one crab predation event was observed, but crabs may not trigger infra-red cameras as they are ectothermic, and it is possible that some unattributed nest failures were caused by crab predation. Atkinson (1985) suggested that bird species occurring between about 15 degrees north and 20 degrees south of the equator co-exist with native rats and abundant crab fauna and therefore may have adaptations that make them less vulnerable or less naïve to invasive mammalian predators (Atkinson 1985). Thrush nests were commonly observed on the mid-ribs and leaves of arenga palms (Arenga listeri) which appear difficult to access for both rats and crabs.
The native predator (goshawk; A. fasciatus natalis) also had an impact on the nesting success of both the thrush and to a lesser degree, red-tailed tropicbirds. Saunier et al. (2022) noted that brood neglect contributed to nest failure rates on Europa Island caused by black rats and birds of prey (2022). Failure due to brood neglect on Christmas Island was comparatively low. It is possible that on Christmas Island, where the combined natural predation risk to nests is high, adults may defend nestlings for longer, which also makes them less vulnerable to black rat impacts. For red-tailed tropicbirds, the influence of marine productivity on the length of foraging trips and therefore the incidence of unattended nests available for predation could affect this to some extent (Sommerfeld 2008).
Finally, like most tropical passerines, the Christmas Island thrush has a life history characterised by long lifespans (Bird et al. 2020), long breeding seasons with multiple breeding attempts per season, and small clutches (average 2; range 1–4; Ricklefs 1997; Davis et al. 2008; James and McAllen 2014). Nest predation is generally higher in tropical bird species, which has likely driven these life history traits (Pianka 2008) and may mean that Christmas Island thrush are able to offset nest losses with more breeding attempts over their lifetime, compared with passerines in temperate regions (Roper et al. 2010). Similarly, seabirds have ‘slow’ life histories with high survival and low fecundity (typically 1 clutch per year) which makes their populations extremely vulnerable to predation of adults but not young, unless predation of offspring is sustained (Jones et al. 2008; Sandvik and Einar Erikstad 2008). Therefore, if rat densities are held low, such as by competition with land crabs, and if abundant vegetative and invertebrate resources are available, then rat predation may never reach levels sufficient to cause population decline in long-lived birds (Atkinson 1985).
These results suggest that the risk posed by invasive species to endemic bird species can be location specific. Population size, conservation status and life-history traits influenced by evolutionary history (egg size, clutch size, fecundity, nesting behaviour, timing of breeding, defence by adults influenced by multi-invader context and the presence of native predators) affect the vulnerability of bird species to rat impacts (Jones et al. 2008). Rat population density, the availability of alternative resources and the seasonality of those resources influence whether rats will co-exist or impact the avifauna of an island ecosystem (Wirtz 1972; Jones et al. 2008; Woodward 2017). Therefore, understanding island-, site- as well as species-specific invasive predator impacts and responses to invasive predator management are critical to evaluations of management planning in invaded ecosystems.
Implications for future management on Christmas Island
Black rats currently have little impact on the bird species studied on Christmas Island, suggesting rat threat mitigation is not a high management priority. However, rats are known to be a key predator of vertebrate groups other than birds, such as reptiles and invertebrates, and can also affect vegetation. Therefore, further study of the relationships between black rats and the full suite of native species would inform a more thorough ecosystem-wide management strategy. The baseline data from this study on forest bird abundance, forest and seabird nesting success, rat population metrics and rat impacts provide a critical platform for detecting the potential ecosystem changes that could result from cat eradication on Christmas Island. Understanding if and how rat and bird populations respond over time as eradication progresses would be gained through repeated monitoring. Understanding how changes in rat impacts, nest failure or background rates of mortality might affect bird populations, would help to set thresholds for management triggers and improve the cost-effectiveness of monitoring (Bal et al. 2021).
Data availability
The data that support this study will be shared upon reasonable request to the corresponding author.
Conflicts of interest
Sarah Legge is an Associate Editor of Wildlife Research but was blinded from the peer-review process for this paper.
Declaration of funding
Sources of funding for this research include The National Environmental Science Program’s Threatened Species Recovery Hub; Generous in-kind support from Parks Australia, Christmas Island National Park; The Australia and Pacific Science Foundation; Birdlife Australia (Stuart Leslie Bird Research Award); The Ecological Society of Australia (Holsworth Wildlife Research Endowment); The Australian Government Department of Infrastructure and Regional Development; and The Royal Society of Zoology, NSW (Ethel Mary Read Research Grant).
Acknowledgements
Thank you to Mark Holsworth, Sue Robinson, Dave Algar, Neil Hamilton, Janos Hennicke, Christina Lipka, Corey Mosen and Eva Pomeroy for advice; and to the many volunteers that assisted with fieldwork.
References
Abe T (2007) Predator or disperser? A test of indigenous fruit preference of alien rats (Rattus rattus) on Nishi-jima (Ogasawara Islands). Pacific Conservation Biology 13(3), 213-218.
| Crossref | Google Scholar |
Algar D, Johnston M (2010) Proposed management plan for cats and black rats on Christmas Island. (Western Australian Department of Environment and Conservation) Available at https://library.dbca.wa.gov.au/static/FullTextFiles/025426.pdf
Bal P, Rhodes JR, Carwardine J, Legge S, Tulloch A, Game E, Martin TG, Possingham HP, McDonald-Madden E (2021) How to choose a cost-effective indicator to trigger conservation decisions? Methods in Ecology and Evolution 12(3), 520-529.
| Crossref | Google Scholar |
Barton K (2020) Package “MuMIn” multi-model inference (1.43.17). Available at https://cran.r-project.org/web/packages/MuMIn/MuMIn.pdf
Bates D, Mächler M, Bolker B, Walker S (2015) Fitting linear mixed-effects models using lme4. Journal of Statistical Software 67(1), 1-48.
| Crossref | Google Scholar |
Bergstrom DM, Lucieer A, Kiefer K, Wasley J, Belbin L, Pedersen TK, Chown SL (2009) Indirect effects of invasive species removal devastate World Heritage Island. Journal of Applied Ecology 46(1), 73-81.
| Crossref | Google Scholar |
Bird JP, Martin R, Akçakaya HR, Gilroy J, Burfield IJ, Garnett ST, Symes A, Taylor J, Şekercioğlu ÇH, Butchart SHM (2020) Generation lengths of the world’s birds and their implications for extinction risk. Conservation Biology 34(5), 1252-1261.
| Crossref | Google Scholar |
Bivand R (2021) Tools for handling spatial objects [R package maptools version 1.1-1]. Comprehensive R Archive Network (CRAN). Available at https://CRAN.R-project.org/package=maptools
Blackburn TM, Cassey P, Duncan RP, Evans KL, Gaston KJ (2004) Avian extinction and mammalian introductions on oceanic islands. Science 305(5692), 1955-1958.
| Crossref | Google Scholar |
Boeken M (2016) Breeding success of Red-billed Tropicbirds Phaethon aethereus on the Caribbean Island of Saba. Ardea 104(3), 263-271.
| Crossref | Google Scholar |
Bolam FC, Mair L, Angelico M, Brooks TM, Burgman M, Hermes C, Hoffmann M, Martin RW, McGowan PJK, Rodrigues ASL, Rondinini C, Westrip JRS, Wheatley H, Bedolla-Guzmán Y, Calzada J, Child MF, Cranswick PA, Dickman CR, Fessl B, Fisher DO, Garnett ST, Groombridge JJ, Johnson CN, Kennerley RJ, King SRB, Lamoreux JF, Lees AC, Lens L, Mahood SP, Mallon DP, Meijaard E, Méndez-Sánchez F, Percequillo AR, Regan TJ, Renjifo LM, Rivers MC, Roach NS, Roxburgh L, Safford RJ, Salaman P, Squires T, Vázquez-Domínguez E, Visconti P, Woinarski JCZ, Young RP, Butchart SHM (2021) How many bird and mammal extinctions has recent conservation action prevented? Conservation Letters 14(1), 21.
| Crossref | Google Scholar |
Bonnaud E, Medina FM, Vidal E, Nogales M, Tershy B, Zavaleta E, Donlan CJ, Keitt B, Le Corre M, Horwath SV (2011) The diet of feral cats on islands: a review and a call for more studies. Biological Invasions 13(3), 581-603.
| Crossref | Google Scholar |
Brooks ME, Kristensen K, van Benthem KJ, Magnusson A, Berg CW, Nielsen A, Skaug HJ, Mächler M, Bolker BM (2017) Modeling zero-inflated count data with glmmTMB. BioRxiv 2017, 132753.
| Crossref | Google Scholar |
Burnham KP, Anderson DR (2002) ‘Model selection and multimodel inference’. (Springer: New York) 10.1007/B97636
Carlsson NOL, Sarnelle O, Strayer DL (2009) Native predators and exotic prey –an acquired taste? Frontiers in Ecology and the Environment 7(10), 525-532.
| Crossref | Google Scholar |
Davis NE, O’Dowd DJ, Green PT, Nally RM (2008) Effects of an alien ant invasion on abundance, behavior, and reproductive success of endemic island birds. Conservation Biology 22(5), 1165-1176.
| Crossref | Google Scholar |
DIISE (2015) The database of island invasive species eradications, developed by Island Conservation, University of California, Santa Cruz, IUCN Species Survival Commission Invasive Species Specialist Group, University of Auckland and Landcare Research New Zealand. Available at http://diise.islandconservation.org
Doherty TS, Glen AS, Nimmo DG, Ritchie EG, Dickman CR (2016) Invasive predators and global biodiversity loss. Proceedings of the National Academy of Sciences of the United States of America 113(40), 11261-11265.
| Crossref | Google Scholar |
Dowding JE, Murphy EC, Springer K, Peacock AJ, Krebs CJ (2009) Cats, rabbits, Myxoma virus, and vegetation on Macquarie Island: a comment on Bergstrom et al. (2009). Journal of Applied Ecology 46(5), 1129-1132.
| Crossref | Google Scholar |
Dueñas M-A, Hemming DJ, Roberts A, Diaz-Soltero H (2021) The threat of invasive species to IUCN-listed critically endangered species: a systematic review. Global Ecology and Conservation 26, e01476.
| Crossref | Google Scholar |
Efford M (2017) Secr 3.1 – spatially explicit capture–recapture in R. Available at https://mran.microsoft.com/snapshot/2017-10-27/web/packages/secr/vignettes/secr-overview.pdf.
Efford M (2021) Habitat masks in the package secr. Available at https://www.otago.ac.nz/density/pdfs/secr-habitatmasks.pdf
Fleet RR (1972) Nesting success of the red-tailed tropicbird on Kure Atoll. The Auk 89(3), 651-659.
| Crossref | Google Scholar |
Fleet RR (1974) The red-tailed tropicbird on Kure Atoll. Ornithological Monographs 16, iii-64.
| Crossref | Google Scholar |
Flores M, Lazo P, Campbell G, Simeone A (2017) Breeding status of the red-tailed tropicbird (Phaethon rubricauda) and threats to its conservation on Easter Island (Rapa Nui). Pacific Science 71(2), 149-160.
| Crossref | Google Scholar |
Geoscience Australia (2013) Christmas Island GIS 2013 datasets. Available at https://data.gov.au/dataset/ds-ga-24c5ba9d-03ed-e3b2-e053-10a3070a0ee1/details?q=
Gillies CA, Williams D (2013) DOC tracking tunnel guide v2.5.2: using tracking tunnels to monitor rodents and mustelids. (Department of Conservation, Science & Capability Group) Available at www.doc.govt.nz
Glen AS, Atkinson R, Campbell KJ, Hagen E, Holmes ND, Keitt BS, Parkes JP, Saunders A, Sawyer J, Torres H (2013) Eradicating multiple invasive species on inhabited islands: the next big step in island restoration? Biological Invasions 15(12), 2589-2603.
| Crossref | Google Scholar |
Grueber CE, Nakagawa S, Laws RJ, Jamieson IG (2011) Multimodel inference in ecology and evolution: challenges and solutions. Journal of Evolutionary Biology 24(4), 699-711.
| Crossref | Google Scholar |
Halpin L, Terrington D, Jones H, Mott R, Wong WW, Dow D, Carlile N, Clarke R (2021) Arthropod predation of vertebrates structures trophic dynamics in island ecosystems. Dryad Dataset. Available at https://datadryad.org/stash/dataset/doi:10.5061/dryad.9kd51c5g2
Harper GA, Bunbury N (2015) Invasive rats on tropical islands: their population biology and impacts on native species. Global Ecology and Conservation 3, 607-627.
| Crossref | Google Scholar |
IUCN (2017) The IUCN red list of threatened species, version 2017-2. Available at http://www.iucnredlist.org
James DJ, McAllen IAW (2014) The birds of Christmas Island, Indian Ocean: a review. Australian Field Ornithology 31(Supplement), S1-S175.
| Google Scholar |
James DJ, Green PT, Humphreys WF, Woinarski JCZ (2019) Endemic species of Christmas Island, Indian Ocean. Records of the Western Australian Museum 34(2), 55-114.
| Crossref | Google Scholar |
Jones HP, Tershy BR, Zavaleta ES, Croll DA, Keitt BS, Finkelstein ME, Howald GR (2008) Severity of the effects of invasive rats on seabirds: a global review. Conservation Biology 22(1), 16-26.
| Crossref | Google Scholar |
Jones HP, Holmes ND, Butchart SHM, Tershy BR, Kappes PJ, Corkery I, Aguirre-Muñoz A, Armstrong DP, Bonnaud E, Burbidge AA, Campbell K, Courchamp F, Cowan PE, Cuthbert RJ, Ebbert S, Genovesi P, Howald GR, Keitt BS, Kress SW, Miskelly CM, Oppel S, Poncet S, Rauzon MJ, Rocamora G, Russell JC, Samaniego-Herrera A, Seddon PJ, Spatz DR, Towns DR, Croll DA (2016) Invasive mammal eradication on islands results in substantial conservation gains. Proceedings of the National Academy of Sciences 113(15), 4033-4038.
| Crossref | Google Scholar |
Legge S, Murphy BP, McGregor H, Woinarski JCZ, Augusteyn J, Ballard G, Baseler M, Buckmaster T, Dickman CR, Doherty T, Edwards G, Eyre T, Fancourt BA, Ferguson D, Forsyth DM, Geary WL, Gentle M, Gillespie G, Greenwood L, Hohnen R, Hume S, Johnson CN, Maxwell M, McDonald PJ, Morris K, Moseby K, Newsome T, Nimmo D, Paltridge R, Ramsey D, Read J, Rendall A, Rich M, Ritchie E, Rowland J, Short J, Stokeld D, Sutherland DR, Wayne AF, Woodford L, Zewe F (2017) Enumerating a continental-scale threat: how many feral cats are in Australia? Biological Conservation 206, 293-303.
| Crossref | Google Scholar |
Luna N, Varela AI, Brokordt K, Luna-Jorquera G (2018) Assessing potential predation risk by introduced predators on unattended eggs in the red-tailed tropicbird, Phaethon rubricauda, on Rapa Nui (Easter Island). Tropical Conservation Science 11,.
| Crossref | Google Scholar |
Medina FM, Bonnaud E, Vidal E, Tershy BR, Zavaleta ES, Josh Donlan C, Keitt BS, Le Corre M, Horwath SV, Nogales M (2011) A global review of the impacts of invasive cats on island endangered vertebrates. Global Change Biology 17(11), 3503-3510.
| Crossref | Google Scholar |
Oksanen J (2020) Package “vegan” (2.5-7). CRAN. Available at https://cran.r-project.org/web/packages/vegan/index.html
Oppel S, Burns F, Vickery J, George K, Ellick G, Leo D, Hillman JC (2014) Habitat-specific effectiveness of feral cat control for the conservation of an endemic ground-nesting bird species. Journal of Applied Ecology 51(5), 1246-1254.
| Crossref | Google Scholar |
Ortega S, Rodríguez C, Mendoza-Hernández B, Drummond H (2021) How removal of cats and rats from an island allowed a native predator to threaten a native bird. Biological Invasions 23, 2749-2761.
| Crossref | Google Scholar |
O’Rourke RL, Anson JR, Saul AM, Banks PB (2020) Limits to alien black rats (Rattus rattus) acting as equivalent pollinators to extinct native small mammals: the influence of stem width on mammal activity at native Banksia ericifolia inflorescences. Biological Invasions 22(2), 329-338.
| Crossref | Google Scholar |
Pattemore DE, Wilcove DS (2012) Invasive rats and recent colonist birds partially compensate for the loss of endemic New Zealand pollinators. Proceedings of the Royal Society B: Biological Sciences 279(1733), 1597-1605.
| Crossref | Google Scholar |
Pianka ER (2008) Optimal reproductive tactics. In ‘Encyclopedia of ecology, five-volume set.’ pp. 2567–2572. (Academic Press) 10.1016/B978-008045405-4.00841-7
Ratcliffe N, Bell M, Pelembe T, Boyle D, Benjamin R, White R, Godley B, Stevenson J, Sanders S (2010) The eradication of feral cats from Ascension Island and its subsequent recolonization by seabirds. Oryx 44(1), 20-29.
| Crossref | Google Scholar |
Rayner MJ, Hauber ME, Imber MJ, Stamp RK, Clout MN (2007) Spatial heterogeneity of mesopredator release within an oceanic island system. Proceedings of the National Academy of Sciences 104(52), 20862-20865.
| Crossref | Google Scholar |
Rendall AR, Sutherland DR, Cooke R, White J (2014) Camera trapping: a contemporary approach to monitoring invasive rodents in high conservation priority ecosystems. PLoS ONE 9(3), e86592.
| Crossref | Google Scholar |
Ricklefs RE (1997) Comparative demography of new world populations of thrushes (Turdus Spp.). Ecological Monographs 67(1), 23-43.
| Crossref | Google Scholar |
Ringler D, Russell JC, le Corre M (2015) Trophic roles of black rats and seabird impacts on tropical islands: mesopredator release or hyperpredation? Biological Conservation 185, 75-84.
| Crossref | Google Scholar |
Ritchie EG, Johnson CN (2009) Predator interactions, mesopredator release and biodiversity conservation. Ecology Letters 12(9), 982-998.
| Crossref | Google Scholar |
Roper JJ, Sullivan KA, Ricklefs RE (2010) Avoid nest predation when predation rates are low, and other lessons: testing the tropical-temperate nest predation paradigm. Oikos 119(4), 719-729.
| Crossref | Google Scholar |
RStudio Team (2021) RStudio: integrated development for R. RStudio, PBC. Available at http://www.rstudio.com/
Russell JC, Kaiser-Bunbury CN (2019) Consequences of multispecies introductions on island ecosystems. Annual Review of Ecology, Evolution, and Systematics 50(1), 169-190.
| Crossref | Google Scholar |
Sandvik H, Einar Erikstad K (2008) Seabird life histories and climatic fluctuations: a phylogenetic-comparative time series analysis of North Atlantic Seabirds. Ecography 31(1), 73-83 Available at http://www.jstor.org/stable/30244552.
| Crossref | Google Scholar |
Saunier M, Amy M, Barbraud C, Pinet P, Ringler D, Russell JC, Le Corre M (2022) Seabird predation effects and population viability analysis indicate the urgent need for rat eradication from Europa Island, western Indian Ocean. Avian Conservation & Ecology 17(1), 32.
| Crossref | Google Scholar |
Schloerke B (2020) GGally: extension to “ggplot2”. CRAN. Available at https://cran.r-project.org/web/packages/GGally/index.html
Simberloff D, Keitt B, Will D, Holmes N, Pickett E, Genovesi P (2018) Yes we can! Exciting progress and prospects for controlling invasives on islands and beyond. Western North American Naturalist 78(4), 942-958 Available at https://scholarsarchive.byu.edu/wnan/vol78/iss4/50.
| Crossref | Google Scholar |
Szabo JK, Khwaja N, Garnett ST, Butchart SHM (2012) Global patterns and drivers of avian extinctions at the species and subspecies level. PLoS ONE 7(10), e47080.
| Crossref | Google Scholar |
Towns DR, Atkinson IAE, Daugherty CH (2006) Have the harmful effects of introduced rats on islands been exaggerated? Biological Invasions 8(4), 863-891.
| Crossref | Google Scholar |
Travers T, Lea M-A, Alderman R, Terauds A, Shaw J (2021) Bottom-up effect of eradications: the unintended consequences for top-order predators when eradicating invasive prey. Journal of Applied Ecology 58(4), 801-811.
| Crossref | Google Scholar |
Vanderwerf EA, Young LC (2014) Breeding biology of red-tailed tropicbirds Phaethon rubricauda and response to predator control on O’ahu, Hawai’i. Marine Ornithology 42, 73-76 Available at http://www.marineornithology.org/content/get.cgi?ident=42_1_73-76.
| Google Scholar |
VanderWerf EA, Crampton LH, Diegmann JS, Atkinson CT, Leonard DL (2014) Survival estimates of wild and captive-bred released Puaiohi, an endangered Hawaiian thrush. The Condor 116(4), 609-618.
| Crossref | Google Scholar |
Varela AI, Luna N, Luna-Jorquera G (2018) Assessing potential Argentine Ant recruitment to pipping eggs in the red-tailed tropicbird on Rapa Nui (Easter Island). Emu – Austral Ornithology 118(4), 381-385.
| Crossref | Google Scholar |
Vernes K, McGrath K (2009) Are introduced black rats (Rattus rattus) a functional replacement for mycophagous native rodents in fragmented forests? Fungal Ecology 2(3), 145-148.
| Crossref | Google Scholar |
Walker K, Porritt K, Sexton M (2014) Christmas Island vegetation and clearing dataset. Available at https://researchdata.edu.au/christmas-island-vegetation-clearing-dataset/1288111
Willacy RJ, Flakus S, Macgregor NA, Bell E, Tiernen B, James DJ, McAllen IAW, Woinarski JCK, Clarke RH, Brown SM, Baker GB, Garnett ST (2021) Indian Ocean red-tailed tropicbird Phaethon rubricauda westralis. In ‘The action plan for Australian birds’. (Eds ST Garnett, GB Baker) pp. 33–36. (CSIRO Publishing)
Wirtz WO (1972) Population ecology of the Polynesian Rat, Rattus exulans, on Kure Atoll, Hawaii. (University of Hawaii Press) Available at http://scholarspace.manoa.hawaii.edu/handle/10125/449
Woodward PW (2017) The natural history of Kure Atoll, northwestern Hawaiian islands. Atoll Research Bulletin 164, 1-318.
| Google Scholar |
Wyatt KB, Campos PF, Gilbert MTP, Kolokotronis S-O, Hynes WH, DeSalle R, Daszak P, MacPhee RDE, Greenwood AD (2008) Historical mammal extinction on Christmas Island (Indian Ocean) correlates with introduced infectious disease. PLoS ONE 3(11), e3602.
| Crossref | Google Scholar |
Zarzoso-Lacoste D, Ruffino L, Vidal E (2011) Limited predatory capacity of introduced black rats on bird eggs: an experimental approach. Journal of Zoology 285(3), 188-193.
| Crossref | Google Scholar |
Zavaleta ES, Hobbs RJ, Mooney HA (2001) Viewing invasive species removal in a whole-ecosystem context. Trends in Ecology & Evolution 16(8), 454-459.
| Crossref | Google Scholar |