Degradation of conventional, biodegradable and oxo-degradable microplastics in a soil using a δ13C technique
Yuxin Huo

A
Abstract
A significant amount of conventional plastics waste, especially in the form of microplastics (MPs), has accumulated in soils due to its limited degradation. Oxo-degradable and biodegradable plastics have also contributed to MP contamination in soils.
In this study, we examined the degradation of a conventional plastic [fruit and vegetable (F&V) bag], two biodegradable plastics (bin liner and mulch film) and an oxo-degradable plastic (drinking straw).
These plastics (5 mm) were mixed into a soil and incubated in the laboratory at 37 ± 1°C for 185 days. The CO2-carbon (C) mineralisation of the four plastics was determined using a δ13C technique, because the difference in the δ13C values of studied plastics and the experimental soil was ≥10‰.
Bin liner showed the greatest C mineralisation (5.7%), followed by mulch film (4.1%), straw (0.4%) and F&V bag (0.3%) at the end of the incubation period. All plastics, except the mulch film for 23–77 days of incubation, caused a positive priming effect on soil organic carbon (SOC). Fourier transform infra-red spectroscopy and scanning electron microscopy analyses were consistent with the C mineralisation data.
This study determines the degradation of various MPs in soil using a reliable and practical δ13C method, which has been lacking in this field of study. The priming effect of various MPs on SOC is a significant finding.
The lack of consideration of priming effect on SOC may overestimate the mineralisation of plastics in soil.
Keywords: biodegradable plastics, CO2, FTIR, microplastics, mineralisation, priming effect, SEM, δ13C technique.
Introduction
It has been estimated that over 6000 Mt of conventional plastic waste, mostly including polyethylene (PE), polypropylene (PP), polyvinyl chloride (PVC), polystyrene (PS) and polyethylene terephthalate (PET), has been generated since 1950 (Geyer et al. 2017). Much of this plastic waste ends up on land (e.g. landfills and agricultural soils), with estimates of 4–23 times greater plastic waste on land than in aquatic environments (Horton et al. 2017). In soil environments, conventional plastics may disintegrate to form micro- and nano-plastics (MPs and NPs) of sizes 1 μm to 5 mm and 1–1000 nm, respectively (Gigault et al. 2018; Frias and Nash 2019). In addition to conventional plastics, biodegradable plastics consisting of polymers [e.g. polylactic acid (PLA), polyhydroxyalkanoates (PHAs), polybutylene adipate terephthalate (PBAT), polycaprolactone (PCL) and polybutylene succinate (PBS)] and oxo-degradable plastics (i.e. conventional plastics containing pro-oxidants such as iron, cobalt and manganese to enhance their degradation through oxidation) also contribute to MPs and NPs in soils. Biodegradable and oxo-degradable MPs and NPs can be formed in compost facilities or under natural environments, which can potentially end up in soils (Kubowicz and Booth 2017; Yu et al. 2021). Consequently, a significant amount of MPs, mostly conventional plastics, have been observed in different soil settings, including farmlands, industrial areas, public parks, forests and deserts (Rafique et al. 2020; Abbasi et al. 2021; Álvarez-Lopeztello et al. 2021). Recently, Huo et al. (2022a) reviewed the presence of MPs in soils worldwide and found mean and median values of 6153 and 1080 particles kg−1 soil, respectively. The presence of MPs and NPs can cause a variety of adverse effects on soil ecosystems, such as affecting soil aggregation, inhibiting flora and fauna growth, and changing microbial community structures (Lehmann et al. 2021; Huo et al. 2022b).
Conventional plastics are highly resistant to degradation due to their long C-chain backbones and lack of degraders in soils. Indeed, research has shown that degradation of conventional PE, PET and PP buried in soils is very slow, with less than 5% weight loss after 6 months of incubation (Yussuf et al. 2018; Datta and Halder 2019; Beltrán-Sanahuja et al. 2021). Among the biodegradable plastics, PBS, PHA, PBAT and PCL showed relatively fast degradation in soil due to the presence of relevant degraders and easily breakable bonds in their backbone, especially PCL which degraded almost 50% (weight basis) within 1 year (Palsikowski et al. 2018; Al Hosni et al. 2019). A wide range of degradation rates have been observed for oxo-degradable plastics depending on pre-treatments (e.g. UV/heat exposure), addition of pro-oxidants, intrinsic plastic characteristics and incubation conditions (Chiellini et al. 2003; Gómez and Michel 2013; Kérouani et al. 2018). More than 60% of total mineralisation was observed for thermally pre-treated low density polyethylene (LPDE) with Totally Degradable Plastic Additives™ (TDPA™) after 600 days of soil incubation, whereas only 1% of total C was mineralised from PP with MasterBatch Pellets™ additive after 2 years of incubation (Chiellini et al. 2003; Gómez and Michel 2013). Nevertheless, the degradation of conventional and oxo-degradable or biodegradable MPs in soil environments is still uncertain, with limited research data (Ferreira-Filipe et al. 2022; Lin et al. 2022; Schöpfer et al. 2022).
Addition of C amendments, such as biochar and fresh organic matter, causes different priming effects (suppressing or accelerating) on the mineralisation of existing soil organic carbon (SOC) (Keith et al. 2011; Zhu et al. 2022). Similarly, plastics (a C substrate) could suppress or accelerate the mineralisation of SOC. However, there is no research on the priming effect of plastics on SOC. Conventional plastics are highly stable and thus their priming effect on SOC is likely to be indirect via influence on soil biota. However, biodegradable plastics, especially mixed with easily degradable fillers such as starch, cellulose and lignin, can serve as an easily available energy source for soil microorganisms that may influence the degradation of native SOC. In addition, some plastics contain toxic additives and plasticisers, which can negatively influence microbial activities and inhibit decomposition of native SOC (Zaborowska et al. 2020; Xiao et al. 2021; Mondal et al. 2022). Rillig et al. (2021) suggested that the negative priming from plastics could be due to the adsorption of dissolved SOC on plastic surfaces, or substrate switching, with easily mineralisable organic C in plastics being preferentially metabolised by microbes. These hypotheses need testing.
Degradation of plastics has been investigated using various analytical approaches, such as visual observation using scanning elecron microscopy (SEM), mechanical ductility measurements and observation of chemical changes in plastics using Fourier transform infra-red (FTIR) spectroscopy and Raman spectroscopy. These methodologies are qualitative and do not measure the actual degradation of plastics. Other methods, such as weight loss, CO2 evolution and O2 consumption have been also frequently used in plastic degradation studies (Tachibana et al. 2010; Al Hosni et al. 2019; Al-Salem et al. 2019). However, weight loss of plastics can be hampered by difficulties in separating small plastic fragments (micro and nano sized) and the release of additives from plastics. Carbon dioxide and O2 are affected by the priming effect of plastics on SOC, and thus may lead to underestimation or overestimation of plastic degradation. Some studies have measured the CO2 mineralisation of PE and PBAT plastics in soil using the carbon (13C/14C) isotope-labelling technique (Albertsson 1978; Zumstein et al. 2018). The use of isotope-labelling technique can distinguish CO2-derived SOC and plastic C mineralisation. However, use of this approach is limited because of specialised equipment requirements, high cost for acquiring radioactive 14C-labelled plastics and specific measures needed for the disposal of radiolabelled samples (Raddadi and Fava 2019). Some studies have used the difference in δ13C values between the original plastic and plastic samples removed from marine or soil environments to characterise the plastic degradation (Berto et al. 2017, 2019; Giebel et al. 2022). However, this method is unable to measure plastic degradation rate. Plastic degradation in soil can be determined through isotope analysis of CO2 respiration in control (without plastic) and plastic-added soil samples, and a linear mixing model to separate soil- from plastic-derived CO2. This approach has been widely used for differentiating C sourced from SOC and biochar (black C) and is easy to use under both laboratory and field conditions (Keith et al. 2011; Fang et al. 2014). To the best of our knowledge, only two studies have used this approach to investigate plastic mineralisation in soils (Guliyev et al. 2023; Zhang et al. 2023).
In this study, we employed the δ13C technique (based on different δ13C signatures of C in soil and plastics) to determine CO2 mineralisation of four commonly used plastics in a soil: conventional fruit and vegetable (F&V) bag, biodegradable bin liner, biodegradable mulch film and oxo-degradable straw. Priming effects of the four plastics on SOC mineralisation were also assessed. In addition, SEM and FTIR techniques were used to further investigate the plastic degradation processes.
Materials and methods
Plastics
Four commercial plastic products were selected based on their composition and δ13C values (Table 1). Biodegradable bin liner and mulch film plastics were made of PBAT and starch, the oxo-degradable straw was made of PP with a pro-oxidant called Reverte™ additive, while the conventional F&V bag comprised high density polyethylene (HDPE). The δ13C values of the four selected plastics were between −30.5‰ and −24.2‰.
Preferred name in the text | Plastic products (manufacturer/source) | Composition (stated on web sites) of plastic products | Major composition under FTIR | δ13C (‰) | C (%) | Thickness (μm) | |
---|---|---|---|---|---|---|---|
Bin liner | Biodegradable bin liner bag (Multix Greener) | Corn starch A | PBAT and starch | −24.23 | 53.4 | 12 | |
Mulch film | Biodegradable plastic mulch film (BioBag) | Mater-Bi (polyester and starch) B | PBAT and starch | −25.35 | 60.1 | 15 | |
Straw | Oxo-degradable drinking straw (Eco-straw) | PP and additive ‘ReverteTM’ C | PP | −27.48 | 86.1 | 60 | |
F&V bag | Conventional fruit and vegetable bag (Australian Woolworths supermarket) | – | HDPE | −30.54 | 84.8 | 5 |
Soil
Surface soil (0–25 cm; a Solonetz) was collected from a field near Gordonvale in North Queensland (17.085°S, 145.808°E), which has been continuously used for sugar cane cropping for more than 15 years. The soil had a distinctively different δ13C value (−15.2‰) than the four plastics, which allowed us to determine the proportion of plastic C and the soil C mineralised in soil–plastic mixtures. Soil was air-dried, ground and sieved to obtain ≤2 mm fraction. Data of important soil properties are presented in Supplementary Table S1. Soil pH and electrical conductivity were measured in a 1:5 solid:solution ratio in deionised water using a pH electrode and electrical conductivity meter, respectively. Particle size analysis was done by the hydrometer method. Total C and nitrogen (N) were measured by dry combustion using a vario MACRO cube CHNS elemental analyser (Elementar Analysensysteme GmbH, Hanau, Germany), with the CHN mode. The δ13C signatures of the soil and plastic samples were determined using a Costech Elemental Analyser fitted with a zero-blank auto-sampler coupled via a ConFlo IV to a Thermo Finnigan Delta VPLUS using Continuous-Flow isotope ratio mass spectrometry (IRMS) at James Cook University’s Advanced Analytical Centre (Cairns, Australia).
Incubation experiment
Plastic samples were cut into 5 mm × 5 mm pieces for use in the incubation experiment. Each plastic sample (2 g) was uniformly mixed with soil (250 g oven-dry basis) at 0.8% (w/w) and transferred into a 2 L wide-mouth jar (Ball Mason). A non-amended control soil, a blank control (without plastic and soil) and a positive control (Whatman cellulose filter paper + soil; 0.8% w/w) were included in the incubation experiment. Extra soil–plastic treatments were included in the experiment and removed after 77 and 185 days of incubation for analysis using FTIR and SEM (see below) to examine surface and chemical changes. Three replicates of each treatment were used in the incubation study. To support microbial growth during incubation, a nutrient solution (mg kg−1 soil) containing 183 mg N, 28 mg P, 22 mg K and 13 mg S was added into the soil and soil–plastic mixtures. To introduce a diverse range of microbial communities, a microbial inoculum obtained from a mixture of soils (i.e. eucalyptus forests, pine plantation, oat farmland, garden, grazed pastures and compost) was mixed with the nutrient solution in a 1:125 ratio. The water holding capacity (i.e. the moisture content of soil, when, after saturation, the downward percolation of water is essentially ceased) of control soil and soil–plastic mixtures was maintained at 70%. Two open glass bottles were placed in each jar, one containing 30 mL of 2 M sodium hydroxide (NaOH) to trap evolved CO2, and another containing 20 mL of distilled water to maintain moisture. The incubation experiment was conducted in darkness at a constant temperature of 37 ± 1°C for 185 days in the laboratory. The CO2 traps were replaced after 1, 4, 9, 23, 43, 77, 126 and 185 days of incubation.
Plastic mineralisation, priming effect and mean resistance time
The total CO2-C mineralised in various treatments was determined by titrating 1 mL of NaOH against 0.1 M HCI using phenolphthalein as an indicator. To determine δ13C in the CO2 evolved, a 10 mL aliquot from each CO2 trap (NaOH solution) was precipitated with 1.25 M SrCl2. The SrCO3 precipitates were analysed for δ13C using IRMS (as described above). From the δ13C data, the proportion of CO2-C derived from plastic was then determined using the following equation (Keith et al. 2011):
where is the δ13C value of the total CO2-C evolved from soil–plastic mixture, is the δ13C value for the CO2-C evolved from soil without plastic, and is the δ13C isotopic value of the plastic.
The priming effect of plastic CO2-C (PEp) (i.e. changes in soil CO2-C mineralisation induced by plastic) on soil was determined as follows:
where CP is the amount (mg kg−1 soil) of CO2-C mineralised from plastic-amended soil and CS is the amount (mg kg−1 soil) of CO2-C from non-amended control soil.
A two-pool exponential model was used to estimate the mean resistance time (MRT) of each plastic in the soil:
where CPt (%) is the cumulative proportion of plastic-C mineralised at time (t); CL and (100 − CL) are the proportions of labile and recalcitrant C pools in plastic-C, respectively; and kL and kR are the constants of labile and recalcitrant mineralisation rates, respectively. The MRT is the inverse of the labile (1/kL) or recalcitrant (1/kR) mineralisation rate constant. For each plastic, the proportion (CL) of labile plastic-C and its labile (kL) and recalcitrant (kR) mineralisation rate constants were estimated using a non-linear least squares regression analysis in R. Eqn 3 was fitted using the function nlsLM from the ‘minpack.lm’ package (Elzhov et al. 2022).
FTIR and SEM
The pristine (original) and plastic samples removed from the soil after 77 and 185 days of incubation were analysed by FTIR and SEM. The incubated plastic samples were rinsed thoroughly in ultrapure water, followed by an ultrasonic cleaning (Microson Ultrasonic Cell Disruptor, Misonix, Farmingdale, USA) for a few minutes to remove adhered soil particles.
The FTIR spectra were collected using a Bruker Alpha FTIR spectrometer with a diamond crystal attenuated total reflection (ATR) accessory (Bruker Optik GmbH, Ettlingen, Germany). For each sample, 32 scans were recorded in the range of 400–4000 cm−1 at a resolution of 4 cm−1. Both pristine and aged plastics were observed to evaluate changes in surface features using a JCM6000 Neoscope Tabletop SEM (JEOL Ltd, Tokyo, Japan). Washed and dried plastic specimens were coated with 10 nm gold nanoparticles to enhance sample conductivity. The SEM images were captured at 10 kV under 2400× magnification.
Statistical analyses
Generalised least-squares model (nlme package) (Pinheiro et al. 2021) was used for cumulative C mineralised and C mineralisation rates from different treatments in R. A correlation of replicates by time (a repeated measure) was specified in each analysis. ANOVA was used to determine the statistical significance of plastic and time factors, as well as their interactions. Plastics, time and their interactive effects played a vital role (P < 0.0001) in all the analyses described above. To further explore the differences between plastic treatments at various time points, pairwise tests using estimated marginal means with a Tukey adjustment were performed and adjusted P-values are presented in Table S2.
Results
Total and plastic CO2-C mineralisation
Total C mineralisation rates (mg CO2-C kg−1 soil day−1) were the greatest on the first day in all samples and then reduced sharply, except in the bin liner treatment for which the decrease was gradual over time (Fig. S1a). Mineralisation rates of bin liner and mulch film were consistently greater than for F&V bag and straw plastics during the 185-day incubation (Fig. 1a). The C mineralisation rate of bin liner plastic was greater than mulch film during the first 9 days of incubation; whereas, in the following period of 9–126 days, the C mineralisation rate was consistently greater for the mulch film. Similar trends of plastic C mineralisation rate (as a proportion of total plastic C) were observed for the four plastics (Fig. S2a).
(a) Plastic C mineralisation rate (mg CO2-C kg−1 soil day−1) and (b) cumulative plastic C mineralised (mg CO2-C kg−1 soil) from soil–plastic mixtures incubated at 37 ± 1°C over a 185-day period. Error bars represent ± s.e.m. (n = 3). Straw and F&V bag lines almost overlap and are hard to distinguish.
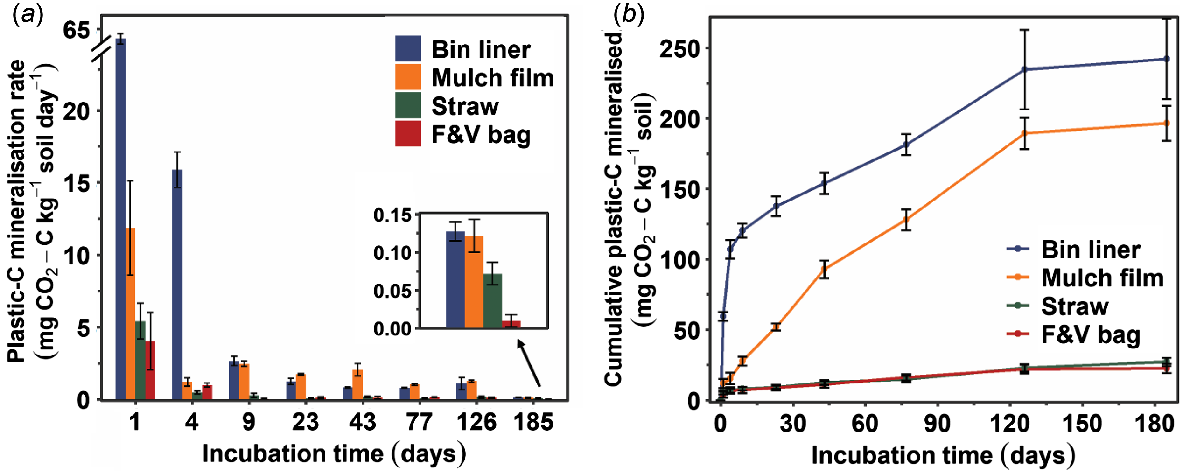
There was an increase in the cumulative total C (mg CO2-C kg−1 soil) mineralised from all four plastics treatments and the control soil (without plastics) with time until about 126 days of incubation, and all samples reached a plateau during 126–185 days (Fig. S1b). The cumulative plastic C mineralised from bin liner plastic was significantly greater (P < 0.0001) than from straw and F&V bag plastics over the 185-day incubation, except on day 1 (Fig. 1b). Similarly, the cumulative C mineralised from bin liner was significantly (P < 0.0001) greater than the mulch film during 4–23 days. However, later on the difference was non-significant and their cumulative C mineralised was similar towards the end of the incubation period. At the end of the incubation period, 5.7% and 4.1% of the total C was mineralised from bin liner and mulch film, respectively, whereas the total plastic-C mineralised was only 0.4% and 0.3% for straw and F&V bag plastics, respectively (Fig. S2b). Correspondingly, the estimated MRT of the recalcitrant C in bin liner was 15 years, while for straw and F&V bag plastics the MRT values were 163 and 193 years, respectively (Table S3). The MRT of mulch film could not be estimated because a positive infinity was obtained in the fitted model. The MRT of the labile C pool in all plastics was not estimated because the estimated labile C only constituted a small (less than 5%) proportion of the total C in all plastics.
FTIR analysis
The absorption bands and assigned functional groups of the four plastics are shown in Table S4. The composition of bin liner and mulch film had typical bands for PBAT and starch, including OH stretch in 3000–3600 cm−1 range due to the presence of starch, 1711 cm−1 (C=O stretch), 1267 cm−1 (C–O stretch) and 1017 cm−1 (C–O stretch or CH in-plane bend) (Ruggero et al. 2021). Straw plastic was characterised as PP, with absorption bands at 2838, 2917 and 2949 cm−1 (CH2 or CH3 stretch); 1456 and 1375 cm−1 (CH2 or CH3 bend); and 1167, 998, 973 and 841 cm−1 (isotactic PP bands) (Jung et al. 2018). The F&V bag was characterised as HDPE and its strong characteristic absorption bands were at 718 cm−1 (CH2 rock), 1471 cm−1 (CH2 bend) and 2847 and 2914 cm−1 (CH2 stretch) (Jung et al. 2018).
Chemical structures of the pristine bin liner and mulch film were similar (Fig. 2). A decrease in the broad band (3000–3600 cm−1) was observed in both plastic samples extracted after 77 days of incubation in the soil (Fig. 2a, b). The decrease in band intensity was greater for bin liner than for mulch film plastic. There were no observable changes in both bin liner and mulch film samples during 77–185 days of incubation. For the straw plastic, the intensity of a band at 1745 cm−1 was increased after 77 and 185 days of incubation compared to the original sample (Fig. 2c). The intensity of this band was greater for the straw sample isolated after 77 days of incubation than the sample after 185 days. Similar changes in the 1745 cm−1 band were also found for the F&V bag plastic (Fig. 2d). Additionally, new bands at 1033 and 1099 cm−1 appeared in the F&V bag spectra after 77 and 185 days of incubation.
ATR-FTIR spectra of original plastics and incubated plastics removed from soils after 77 and 185 days of soil incubation at 37 ± 1°C: (a) bin liner, (b) mulch film, (c) straw and (d) F&V bag. The enlarged regions of the spectra (specified with red rectangle), where substantial changes in absorption bands were observed, are presented on the right-hand side of the figure.
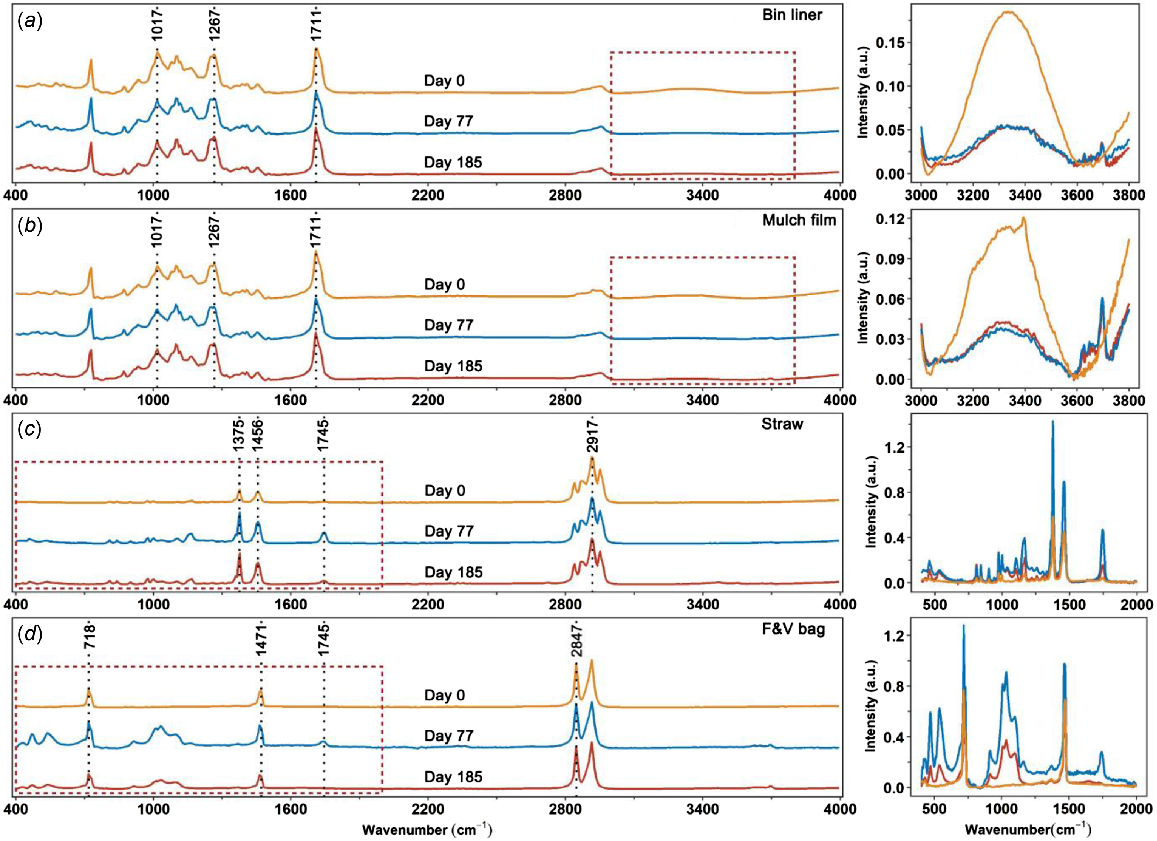
SEM observations
There was a varying degree of surface deterioration of plastics after incubation in the soil (Fig. S3). After 77 days of incubation, the surface of bin liner and mulch film plastics became rough and deteriorated, with a greater number of holes in the bin liner plastic than in the mulch film plastic (Fig. S3a, b). With increasing degradation, cavities spread in the bin liner became larger by the end of the incubation period compared to those after 77 days. Mulch film surface was eroded to a greater extent and more cavities appeared on the surface after an extended period of degradation in the soil. However, there were fewer holes distributed on the mulch film surface than the bin liner surface. There were bumps embedded in the original straw plastic and the bumps disintegrated after 77 days incubation (Fig. S3c). After 185 days, the bumps disappeared from the straw surface, which became relatively smooth. There were no obvious changes in the surface of F&V bag plastic after 77 days of incubation in the soil (Fig. S3d); however, a few small pits were observed on the surface in the sample after 185 days.
Mineralisation of SOC and priming effect
The mineralisation rates of SOC were greatest on the first day and decreased sharply in all plastic treatments except for the bin liner plastic where it declined gradually over the incubation period (Fig. S4a). The amount of cumulative soil-C mineralised increased for all plastic treatments during the first 126 days of incubation and then stabilised during 126–185 days (Fig. S4b). All plastics, except for mulch film during 23–77 days of incubation, showed a positive priming effect on SOC mineralisation (Fig. 3). The addition of bin liner caused the greatest positive priming effect on soil-C mineralisation, which significantly increased with time until sampling at 126 days and after this it remained the same. In contrast, the positive priming effects of F&V bag and straw gradually increased and then decreased over the incubation period (temporarily for F&V bag). Only the addition of mulch film resulted in temporarily reduced SOC mineralisation.
Discussion
Degradation of biodegradable bin liner and mulch film
A greater cumulative C mineralisation of bin liner than mulch film at the beginning of incubation was observed and this difference in C mineralised was much smaller towards the end of the experiment (Fig. 1b). These observations are consistent with SEM results (Fig. S3a, b). The corresponding MRT of bin liner was 15 years. However the MRT of mulch film was not properly obtained, indicating that a longer incubation period may be needed to obtain a robust estimate of its MRT. The total mineralisation of both bin liner (5.7%) and mulch film (4.1%) plastics in this study is much less than reported by other studies (Bettas Ardisson et al. 2014; Tosin et al. 2020). Tosin et al. (2020) found 41.9%, 83.3% and 88.0% total CO2 mineralised from Mater-Bi EF04P mulch film (i.e. a mulch film comprising biodegradable polyester, starch and a natural plasticiser) in a sandy loam soil after 119, 292 and 364 days of incubation, respectively. Similarly, more than 60% total C mineralisation of Mater-Bi mulch film was observed after 150 days of soil incubation, and reached 77.5% after 318 days (Bettas Ardisson et al. 2014). Although compositions of the plastics in these studies are broadly similar to the mulch film (Mater-Bi) and bin liner in our study, some differences in the fillers, incubation conditions and/or soil characteristics may have affected their abiotic degradation and/or mineralisation. An increase or decrease in carbonyl groups of Mater-Bi material after soil incubation has been observed in other studies, indicating abiotic or microbial degradation, respectively (Rapisarda et al. 2019; La Mantia et al. 2020). However, plastic mineralisation was not measured in these studies. In our study, no obvious changes in the intensity of carbonyl groups (1711 cm−1) were found in mulch film and bin liner plastics, possibly due to limited abiotic and/or biotic degradation of PBAT. Also, plastic mineralisation in the earlier studies was calculated using the difference in the total C mineralised from plastic treatments and the control soil (Bettas Ardisson et al. 2014; Tosin et al. 2020) – this approach assumes that addition of plastic substrate does not alter the CO2 mineralisation of native SOC. Based on this assumption, the amount of plastic-C mineralised may have been overestimated. In this study, although the mulch film plastic did not show much positive priming effect on SOC, the addition of bin liner plastic with a similar major composition caused a cumulative positive priming of up to 396 mg CO2-C kg−1 to SOC. Other studies have assessed the degradation of Mater-Bi material buried in soils based on weight loss. Weight losses of 3.4–37% have been observed after incubation of 90–120 days, depending on the incubation temperature and soil conditions (Accinelli et al. 2019; Rapisarda et al. 2019; La Mantia et al. 2020). Degradation in these studies may also have been overestimated because the weight loss of non-visible small fragments and additives released were not considered.
Different plastic characteristics (e.g. thickness and additives) may explain the difference in the degradation rate of PBAT/starch in the bin liner and mulch film. The cumulative mineralisation from the mulch film was significantly lower than from the bin liner at the beginning of incubation, suggesting that mulch film contained toxic additives and plasticisers that may be released during the initial incubation period, leading to decreased microbial abundance and activities, and thus lower mineralisation during the initial period than that observed for the bin liner. Bandopadhyay et al. (2020) investigated the effects of four biodegradable plastics on soil microbial communities and found that only Mater-Bi mulch film caused a significant decrease in bacterial 16S rRNA and fungal ITS gene copy abundances, which may partially explain the lower mineralisation of mulch film (also made of Mater-Bi) in our study. Starch also plays a major role in the degradation of PBAT/starch blend, with the degradation rate measured by weight loss. This increased with increasing starch concentrations in the range of 5–40% (Someya et al. 2007; Wang et al. 2015; Pokhrel et al. 2021). In our study, the cumulative C mineralisation of bin liner was greater than mulch film across the entire incubation, so possibly the starch proportion in the bin liner plastic was greater than in mulch film and so caused a greater mineralisation. Our FTIR results also showed a greater intensity of starch hydroxyl groups in the original bin liner than in the mulch film (Fig. 2a, b). The decreased intensity of the hydroxyl group after 77 and 185 days of incubation compared to pristine samples in both bin liner and mulch film treatments further suggests that starch played an important role in the degradation of both plastics. Thus, with a higher starch content in bin liner than in mulch film, greater mineralisation would be expected for the bin liner treatment. In addition, the bin liner plastic was thinner than the mulch film, which may have contributed to its greater mineralisation compared to mulch film. Thick plastics impede diffusion of moisture and microorganisms and therefore exhibit relatively slow hydrolysis and mineralisation (Rujnić-Sokele and Pilipović 2017; Barron and Sparks 2020).
Degradation of oxo-degradable straw
In general, degradation of PP plastic is very slow in soils (Raslan et al. 2018; Darwish et al. 2021). Half-life of up to 780 years has been estimated for a PP container, with a thickness of 800 μm (Chamas et al. 2020). Addition of a pro-oxidant into PP plastics can accelerate oxidative degradation reactions that lead to the formation of small molecular hydrocarbons and oxidation products, like ketones, carboxylic acid and alcohols, which can be easily assimilated by soil microorganisms (Ammala et al. 2011). The oxidative and microbial degradation processes of PP plastic were confirmed in our FTIR results. There was an increase in carbonyl groups at the 1745 cm−1 band in straw plastic, indicating that oxidative degradation occurred during the first 77 days of incubation. The intensity of carbonyl functional group substantially decreased towards the end of the incubation experiment, suggesting that soil microorganisms utilised PP plastic. Several studies have shown a decrease in the carbonyl group of PP plastic on exposure to a biotic environment (Arkatkar et al. 2010; Jain et al. 2022).
In this study, the total amount of C mineralised from straw plastic (PP plastic blended with a pro-oxidant) was less than 0.5% after 185 days of incubation. This is consistent with 1% C loss observed for PP with the pro-oxidant of ECM Masterbatch Pellets™ after 660 days of soil incubation (Gómez and Michel 2013). In contrast, Sable et al. (2019) found that up to 8.0% and 8.4% of C was mineralised from PP blended with the pro-oxidants of calcium and cobalt stearate, respectively, after 45 days compositing (at 58°C) with a municipal solid waste inoculum. Municipal waste compost has a high level of biological activity and a higher temperature (58°C), which may have enhanced both abiotic oxidation of plastics and microbial mineralisation in their study. Later studies demonstrated that the concentration of added pro-oxidants and abiotic treatments play vital roles in oxo-degradation of PP (Sable et al. 2020a, 2020b, 2021). Yussuf et al. (2018) found that PP containing TDPA® pro-degradant additive experienced more than 4% weight loss after outdoor burial for 6 months, whereas no weight loss was observed for pure PP during the same period. The PP containing pro-oxidant can be more easily degraded than pure PP, but it is still very slow in soil with an estimated 163 years of MRT of the recalcitrant C pool observed in this study. The SEM showed that straw plastic was relatively intact after 185 days of incubation corresponding to the small total C mineralisation. Bumps embedded in the straw sample had disintegrated after 77 days of incubation (Fig. S3c), probably due to the catalysation of the pro-oxidant. This could enhance oxidative and microbial degradation of straw plastic and lead to a greater C mineralisation rate than with the F&V bag especially at the beginning of incubation experiment.
Degradation of conventional F&V bag
Polyethylene is a highly recalcitrant and inert material that degrades very slowly in the environment. Only partial deterioration and negligible weight loss in a PE sheet were observed after keeping it in soil for 32 years (Otake et al. 1995). The recalcitrance of PE is due to its insolubility in water, hydrophobicity, high stability of C–C backbones, high degree of crystallinity and its large molecular weight (Tokiwa et al. 2009; Restrepo-Flórez et al. 2014). Despite its low degradability, more than 10% of LDPE was mineralised, when mixed with specific isolates from soil or landfill for 60 days (Das and Kumar 2015; Das et al. 2018). However, these conditions do not represent real soil environments, which contain a mixture of microbial species. In contrast, the mineralisation of PE plastics in natural soil has been found to be very small. Esmaeili et al. (2013) found that only 7.6% was mineralised from pure LDPE films after a 126-day soil incubation. The degradability of HDPE in our study was even lower than that of LDPE, probably due to longer C chains that lack functional sites for microbial degradation. Albertsson (1978) found that only approximately 0.5% of HDPE films were mineralised in cultivated soil after incubation for 2 years using a relatively accurate 14C labelling technique, similar to our results with 0.3% mineralisation for F&V bag after 185 days of soil incubation. The small degradation of the F&V bag was also exhibited in SEM results, where no obvious surface changes were observed after 77 and 185 days of incubation. Similar to the straw plastic, an initial oxidative degradation followed by microbial degradation of the F&V bag has been suggested. An increase in the carbonyl group of the F&V bag was observed after 77 days of incubation, but this decreased in the sample taken after 185 days. In addition, the formation of alkoxy groups between 1000 and 1090 cm−1 was observed in the F&V bag spectrum after 77 days of incubation, and the intensity of these bands decreased with prolonged incubation.
Priming effect
We found an overall positive priming effect on SOC with the addition of all four plastics at the termination of incubation, consistent with observations in another two studies (Guliyev et al. 2023; Zhang et al. 2023). The positive priming could be due to co-metabolism resulting from increased microbial biomass and simultaneous increase in enzyme production. Specifically, the increased SOC mineralisation was probably due to an increased extracellular enzyme activity stimulated by plastics and/or the addition of energy brought by plastics catabolites for SOC decomposers to utilise. Conventional F&V bag and oxo-degradable straw are not readily available energy sources for microbial communities, thus a small positive priming effect was observed. However, PBAT and starch-based bin liner is hydrolysable through enzymes such as lipases, cutinases and esterases and biodegrades through microbial assimilation (Perz et al. 2016), which could lead to co-metabolic SOC mineralisation. Notably, the mulch film, mainly consisting of PBAT and starch, had a cumulative positive priming effect over the incubation period, but it was far less than for the bin liner. There was a negative priming effect for the mulch film during 23–77 days of incubation. The negative priming of mulch film could be a result of toxic additives present in the plastic, which may have reduced microbial activities and led to a negative priming effect on SOC. However, further research is needed to confirm this hypothesis.
Conclusions
Increasing usage of PE as mulch film has led to the accumulation of a large amount of plastic waste in soil environments. The low degradability of PE plastic and difficulties in collecting and recycling contribute to plastic contamination in soils. Biodegradable mulch films offer an advantage due to their relatively rapid degradation in soil environments. It has been estimated that demand for biodegradable mulch film will increase to USD 82.82 million by 2030 compared to USD 45.24 million in 2022 (Grand View Research 2022). However, MPs and NPs can be formed from degradation of biodegradable mulch film in soils (Sintim et al. 2020; Griffin-LaHue et al. 2022). Concerns have arisen over the generation of MPs and NPs because they may persist in soils for extended periods. Our results showed that the mineralisation of biodegradable mulch film MPs in soil is rather limited (only 4.1% after ~6 months) and this mineralisation was mainly due to the decomposition of starch in the film. Biodegradable MPs and NPs can pose environmental hazards to plant growth, microbial community and function and adversely affect soil functions and properties (Huo et al. 2022b). Therefore, the use of biodegradable mulch film should be carefully considered.
Carbon dioxide efflux from soils is one of earth’s largest fluxes of C to the atmosphere and any changes in soil CO2 flux would have crucial impacts on climate, food security and atmospheric CO2 concentration (Myers et al. 2017). Consequently, it is important to preserve and even increase SOC to mitigate greenhouse gas emissions and meet the food security challenge. As observed in this study, MP contamination can cause a positive priming to SOC, especially biodegradable bin liner blended with readily degradable starch. Therefore, the increased SOC mineralisation caused by plastics may affect climate change. Further research is needed on this aspect, particularly under field conditions and over a longer period to evaluate the effect of MPs on natural soil.
This study demonstrates that the δ13C technique is a powerful tool for investigating the rate of mineralisation of biodegradable, conventional and oxo-degradable MPs in soils. Not only is it capable of distinguishing between plastic and soil-derived C, but also allowed us to determine plastics’ priming effect on SOC. One limitation of this technique is the need to have contrasting δ13C values for soil and plastics, which may not be possible in all instances. However, with terrestrial plastic pollution becoming an increasing problem, understanding the mineralisation of plastics is crucial. Additionally, the effect of MP characteristics (e.g. size, type and thickness), soil characteristics (e.g. pH, texture and organic matter content), temperature (mesophilic and thermophilic) and moisture on plastic mineralisation should be the focus of future studies.
Conflicts of interest
Balwant Singh is a co-Editor-in-Chief of Soil Research, but played no role in the editorial handling or review process of this manuscript.
Acknowledgements
We are grateful to Professor Michael Bird from James Cook University for providing the soil sample and Scott Morton from BioBag for providing Mater-Bi mulch film. We also would like to thank Elizabeth Carter and Ashalatha Indiradevi Kamalasanan Pillai for FTIR and SEM training, respectively. We also thank Jennifer Whan from James Cook University for the δ13C analysis.
References
Abbasi S, Turner A, Hoseini M, Amiri H (2021) Microplastics in the Lut and Kavir Deserts, Iran. Environmental Science & Technology 55, 5993-6000.
| Crossref | Google Scholar | PubMed |
Accinelli C, Abbas HK, Shier WT, Vicari A, Little NS, Aloise MR, Giacomini S (2019) Degradation of microplastic seed film-coating fragments in soil. Chemosphere 226, 645-650.
| Crossref | Google Scholar | PubMed |
Albertsson A-C (1978) Biodegradation of synthetic polymers. II. A limited microbial conversion of 14C in polyethylene to 14CO2 by some soil fungi. Journal of Applied Polymer Science 22, 3419-3433.
| Crossref | Google Scholar |
Al Hosni AS, Pittman JK, Robson GD (2019) Microbial degradation of four biodegradable polymers in soil and compost demonstrating polycaprolactone as an ideal compostable plastic. Waste Management 97, 105-114.
| Crossref | Google Scholar | PubMed |
Al-Salem SM, Sultan HH, Karam HJ, Al-Dhafeeri AT (2019) Determination of biodegradation rate of commercial oxo-biodegradable polyethylene film products using ASTM D 5988. Journal of Polymer Research 26, 157.
| Crossref | Google Scholar |
Álvarez-Lopeztello J, Robles C, del Castillo RF (2021) Microplastic pollution in neotropical rainforest, savanna, pine plantations, and pasture soils in lowland areas of Oaxaca, Mexico: preliminary results. Ecological Indicators 121, 107084.
| Crossref | Google Scholar |
Ammala A, Bateman S, Dean K, Petinakis E, Sangwan P, Wong S, Yuan Q, Yu L, Patrick C, Leong KH (2011) An overview of degradable and biodegradable polyolefins. Progress in Polymer Science 36, 1015-1049.
| Crossref | Google Scholar |
Arkatkar A, Juwarkar AA, Bhaduri S, Uppara PV, Doble M (2010) Growth of Pseudomonas and Bacillus biofilms on pretreated polypropylene surface. International Biodeterioration & Biodegradation 64, 530-536.
| Crossref | Google Scholar |
Bandopadhyay S, Sintim HY, DeBruyn JM (2020) Effects of biodegradable plastic film mulching on soil microbial communities in two agroecosystems. PeerJ 8, e9015.
| Crossref | Google Scholar | PubMed |
Barron A, Sparks TD (2020) Commercial marine-degradable polymers for flexible packaging. iScience 23, 101353.
| Crossref | Google Scholar | PubMed |
Beltrán-Sanahuja A, Benito-Kaesbach A, Sánchez-García N, Sanz-Lázaro C (2021) Degradation of conventional and biobased plastics in soil under contrasting environmental conditions. Science of The Total Environment 787, 147678.
| Crossref | Google Scholar |
Berto D, Rampazzo F, Gion C, Noventa S, Ronchi F, Traldi U, Giorgi G, Cicero AM, Giovanardi O (2017) Preliminary study to characterize plastic polymers using elemental analyser/isotope ratio mass spectrometry (EA/IRMS). Chemosphere 176, 47-56.
| Crossref | Google Scholar | PubMed |
Berto D, Rampazzo F, Gion C, Noventa S, Formalewicz M, Ronchi F, Traldi U, Giorgi G (2019) Elemental Analyzer/Isotope Ratio Mass Spectrometry (EA/IRMS) as a tool to characterize plastic polymers in a marine environment. In ‘Plastics in the environment’. (Ed. A Gomiero) pp. 37–54. (IntechOpen: London)
Bettas Ardisson G, Tosin M, Barbale M, Degli-Innocenti F (2014) Biodegradation of plastics in soil and effects on nitrification activity. A laboratory approach. Frontiers in Microbiology 5, 710.
| Crossref | Google Scholar |
Chamas A, Moon H, Zheng J, Qiu Y, Tabassum T, Jang JH, Abu-Omar M, Scott SL, Suh S (2020) Degradation rates of plastics in the environment. ACS Sustainable Chemistry & Engineering 8, 3494-3511.
| Crossref | Google Scholar |
Chiellini E, Corti A, Swift G (2003) Biodegradation of thermally-oxidized, fragmented low-density polyethylenes. Polymer Degradation and Stability 81, 341-351.
| Crossref | Google Scholar |
Darwish MSA, Mostafa MH, Hussein LI, Abdaleem AH, Elsawy MA (2021) Preparation, characterization, mechanical and biodegradation behavior of polypropylene – chitosan/ZnO nanocomposite. Polymer-Plastics Technology and Materials 60, 1630-1640.
| Crossref | Google Scholar |
Das MP, Kumar S (2015) An approach to low-density polyethylene biodegradation by Bacillus amyloliquefaciens. 3 Biotech 5, 81-86.
| Crossref | Google Scholar | PubMed |
Das MP, Kumar S, Das J (2018) Fungal-mediated deterioration and biodegradation study of low-density polyethylene (LDPE) isolated from municipal dump yard in Chennai, India. Energy, Ecology and Environment 3, 229-236.
| Crossref | Google Scholar |
Datta D, Halder G (2019) Effect of media on degradability, physico-mechanical and optical properties of synthesized polyolefinic and PLA film in comparison with casted potato/corn starch biofilm. Process Safety and Environmental Protection 124, 39-62.
| Crossref | Google Scholar |
Elzhov TV, Mullen KM, Spiess A-N, Bolker B (2022) minpack.lm: R interface to the Levenberg-Marquardt nonlinear least-squares algorithm found in MINPACK, plus support for bounds. R Package Version 1.2-2. Available at https://cran.r-project.org/web/packages/minpack.lm/index.html [verified 11 October 2022].
Esmaeili A, Pourbabaee AA, Alikhani HA, Shabani F, Esmaeili E (2013) Biodegradation of low-density polyethylene (LDPE) by mixed culture of Lysinibacillus xylanilyticus and Aspergillus niger in soil. PLoS ONE 8, e71720.
| Crossref | Google Scholar | PubMed |
Fang Y, Singh B, Singh BP, Krull E (2014) Biochar carbon stability in four contrasting soils. European Journal of Soil Science 65, 60-71.
| Crossref | Google Scholar |
Ferreira-Filipe DA, Paço A, Natal-da-Luz T, Sousa JP, Saraiva JA, Duarte AC, Rocha-Santos T, Patrício Silva AL (2022) Are mulch biofilms used in agriculture an environmentally friendly solution? – an insight into their biodegradability and ecotoxicity using key organisms in soil ecosystems. Science of The Total Environment 828, 154269.
| Crossref | Google Scholar | PubMed |
Frias JPGL, Nash R (2019) Microplastics: finding a consensus on the definition. Marine Pollution Bulletin 138, 145-147.
| Crossref | Google Scholar | PubMed |
Geyer R, Jambeck JR, Law KL (2017) Production, use, and fate of all plastics ever made. Science Advances 3, e1700782.
| Crossref | Google Scholar | PubMed |
Giebel BM, Cime S, Rodgers L, Li T-D, Zhang S, Wang T (2022) Short-term exposure to soils and sludge induce changes to plastic morphology and 13C stable isotopic composition. Science of the Total Environment 821, 153375.
| Crossref | Google Scholar | PubMed |
Gigault J, Halle At, Baudrimont M, Pascal P-Y, Gauffre F, Phi T-L, El Hadri H, Grassl B, Reynaud S (2018) Current opinion: what is a nanoplastic? Environmental Pollution 235, 1030-1034.
| Crossref | Google Scholar | PubMed |
Gómez EF, Michel FC, Jr. (2013) Biodegradability of conventional and bio-based plastics and natural fiber composites during composting, anaerobic digestion and long-term soil incubation. Polymer Degradation and Stability 98, 2583-2591.
| Crossref | Google Scholar |
Grand View Research (2022) Biodegradable mulch films market size, share & trends analysis report by crop (grains & oilseeds, flowers & plants), by raw material (TPS, PLA, PHA, AAC), by region (APAC, North America), and segment forecasts, 2022 - 2030. Available at https://www.grandviewresearch.com/industry-analysis/biodegradable-mulch-films-market/methodology [verified 5 December 2022].
Griffin-LaHue D, Ghimire S, Yu Y, Scheenstra EJ, Miles CA, Flury M (2022) In-field degradation of soil-biodegradable plastic mulch films in a Mediterranean climate. Science of The Total Environment 806, 150238.
| Crossref | Google Scholar | PubMed |
Guliyev V, Tanunchai B, Udovenko M, Menyailo O, Glaser B, Purahong W, Buscot F, Blagodatskaya E (2023) Degradation of bio-based and biodegradable plastic and its contribution to soil organic carbon stock. Polymers 15, 660.
| Crossref | Google Scholar | PubMed |
Horton AA, Walton A, Spurgeon DJ, Lahive E, Svendsen C (2017) Microplastics in freshwater and terrestrial environments: evaluating the current understanding to identify the knowledge gaps and future research priorities. Science of The Total Environment 586, 127-141.
| Crossref | Google Scholar | PubMed |
Huo Y, Dijkstra FA, Possell M, Singh B (2022b) Ecotoxicological effects of plastics on plants, soil fauna and microorganisms: a meta-analysis. Environmental Pollution 310, 119892.
| Crossref | Google Scholar | PubMed |
Jain K, Bhunia H, Reddy MS (2022) Degradation of polypropylene-poly-L-lactide blends by Bacillus isolates: a microcosm and field evaluation. Bioremediation Journal 26, 64-75.
| Crossref | Google Scholar |
Jung MR, Horgen FD, Orski SV, Rodriguez C V, Beers KL, Balazs GH, Jones TT, Work TM, Brignac KC, Royer S-J, Hyrenbach KD, Jensen BA, Lynch JM (2018) Validation of ATR FT-IR to identify polymers of plastic marine debris, including those ingested by marine organisms. Marine Pollution Bulletin 127, 704-716.
| Crossref | Google Scholar | PubMed |
Keith A, Singh B, Singh BP (2011) Interactive priming of biochar and labile organic matter mineralization in a smectite-rich soil. Environmental Science & Technology 45, 9611-9618.
| Crossref | Google Scholar | PubMed |
Kérouani S, Djerboua F, Sadoun T (2018) Efficient enhancement in polyethylene biodegradation as a consequence of oxidative fragmentation promoted by pro-oxidant/pro-degradant metal stearate. Journal of Polymer Engineering 38, 625-634.
| Crossref | Google Scholar |
Kubowicz S, Booth AM (2017) Biodegradability of plastics: challenges and misconceptions. Environmental Science & Technology 51, 12058-12060.
| Crossref | Google Scholar | PubMed |
La Mantia FP, Ascione L, Mistretta MC, Rapisarda M, Rizzarelli P (2020) Comparative investigation on the soil burial degradation behaviour of polymer films for agriculture before and after photo-oxidation. Polymers 12, 753.
| Crossref | Google Scholar | PubMed |
Lehmann A, Leifheit EF, Gerdawischke M, Rillig MC (2021) Microplastics have shape- and polymer-dependent effects on soil aggregation and organic matter loss – an experimental and meta-analytical approach. Microplastics and Nanoplastics 1, 7.
| Crossref | Google Scholar |
Lin Y, Xie J, Xiang Q, Liu Y, Wang P, Wu Y, Zhou Y (2022) Effect of propiconazole on plastic film microplastic degradation: focusing on the change in microplastic morphology and heavy metal distribution. Science of The Total Environment 822, 153609.
| Crossref | Google Scholar | PubMed |
Mondal T, Mondal S, Ghosh SK, Pal P, Soren T, Pandey S, Maiti TK (2022) Phthalates – a family of plasticizers, their health risks, phytotoxic effects, and microbial bioaugmentation approaches. Environmental Research 214, 114059.
| Crossref | Google Scholar | PubMed |
Myers SS, Smith MR, Guth S, Golden CD, Vaitla B, Mueller ND, Dangour AD, Huybers P (2017) Climate change and global food systems: potential impacts on food security and undernutrition. Annual Review of Public Health 38, 259-277.
| Crossref | Google Scholar | PubMed |
Otake Y, Kobayashi T, Asabe H, Murakami N, Ono K (1995) Biodegradation of low-density polyethylene, polystyrene, polyvinyl chloride, and urea formaldehyde resin buried under soil for over 32 years. Journal of Applied Polymer Science 56, 1789-1796.
| Crossref | Google Scholar |
Palsikowski PA, Kuchnier CN, Pinheiro IF, Morales AR (2018) Biodegradation in soil of PLA/PBAT blends compatibilized with chain extender. Journal of Polymers and the Environment 26, 330-341.
| Crossref | Google Scholar |
Perz V, Baumschlager A, Bleymaier K, Zitzenbacher S, Hromic A, Steinkellner G, Pairitsch A, Łyskowski A, Gruber K, Sinkel C, Küper U, Ribitsch D, Guebitz GM (2016) Hydrolysis of synthetic polyesters by Clostridium botulinum esterases. Biotechnology and Bioengineering 113, 1024-1034.
| Crossref | Google Scholar | PubMed |
Pinheiro J, Bates D, DebRoy S, Sarkar D, R Core Team (2021) nlme: linear and nonlinear mixed effects models. R package version 3.1-160. Available at https://CRAN.R-project.org/package=nlme. [Verified 11 November 2022]
Pokhrel S, Sigdel A, Lach R, Slouf M, Sirc J, Katiyar V, Bhattarai DR, Adhikari R (2021) Starch-based biodegradable film with poly(butylene adipate-co-terephthalate): preparation, morphology, thermal and biodegradation properties. Journal of Macromolecular Science, Part A 58, 610-621.
| Crossref | Google Scholar |
Raddadi N, Fava F (2019) Biodegradation of oil-based plastics in the environment: existing knowledge and needs of research and innovation. Science of The Total Environment 679, 148-158.
| Crossref | Google Scholar | PubMed |
Rafique A, Irfan M, Mumtaz M, Qadir A (2020) Spatial distribution of microplastics in soil with context to human activities: a case study from the urban center. Environmental Monitoring and Assessment 192, 671.
| Crossref | Google Scholar | PubMed |
Rapisarda M, La Mantia FP, Ceraulo M, Mistretta MC, Giuffrè C, Pellegrino R, Valenti G, Rizzarelli P (2019) Photo-oxidative and soil burial degradation of irrigation tubes based on biodegradable polymer blends. Polymers 11, 1489.
| Crossref | Google Scholar |
Raslan HA, Fathy ES, Mohamed RM (2018) Effect of gamma irradiation and fiber surface treatment on the properties of bagasse fiber-reinforced waste polypropylene composites. International Journal of Polymer Analysis and Characterization 23, 181-192.
| Crossref | Google Scholar |
Restrepo-Flórez J-M, Bassi A, Thompson MR (2014) Microbial degradation and deterioration of polyethylene – a review. International Biodeterioration & Biodegradation 88, 83-90.
| Crossref | Google Scholar |
Rillig MC, Leifheit E, Lehmann J (2021) Microplastic effects on carbon cycling processes in soils. PLoS Biology 19, e3001130.
| Crossref | Google Scholar | PubMed |
Ruggero F, Onderwater RCA, Carretti E, Roosa S, Benali S, Raquez J-M, Gori R, Lubello C, Wattiez R (2021) Degradation of film and rigid bioplastics during the thermophilic phase and the maturation phase of simulated composting. Journal of Polymers and the Environment 29, 3015-3028.
| Crossref | Google Scholar |
Rujnić-Sokele M, Pilipović A (2017) Challenges and opportunities of biodegradable plastics: a mini review. Waste Management & Research: The Journal for a Sustainable Circular Economy 35, 132-140.
| Crossref | Google Scholar | PubMed |
Sable S, Mandal DK, Ahuja S, Bhunia H (2019) Biodegradation kinetic modeling of oxo-biodegradable polypropylene/polylactide/nanoclay blends and composites under controlled composting conditions. Journal of Environmental Management 249, 109186.
| Crossref | Google Scholar | PubMed |
Sable S, Ahuja S, Bhunia H (2020a) Effect of pro-oxidant concentration on characteristics of packaging films of cobalt stearate filled polypropylene. Journal of Polymer Engineering 40, 637-646.
| Crossref | Google Scholar |
Sable S, Ahuja S, Bhunia H (2020b) Studies on biodegradability of cobalt stearate filled polypropylene after abiotic treatment. Journal of Polymers and the Environment 28, 2236-2252.
| Crossref | Google Scholar |
Sable S, Ahuja S, Bhunia H (2021) Biodegradation kinetic modeling of pro-oxidant filled polypropylene composites under thermophilic composting conditions after abiotic treatment. Environmental Science and Pollution Research 28, 21231-21244.
| Crossref | Google Scholar | PubMed |
Schöpfer L, Schnepf U, Marhan S, Brümmer F, Kandeler E, Pagel H (2022) Hydrolyzable microplastics in soil—low biodegradation but formation of a specific microbial habitat? Biology and Fertility of Soils 58, 471-486.
| Crossref | Google Scholar |
Sintim HY, Bary AI, Hayes DG, Wadsworth LC, Anunciado MB, English ME, Bandopadhyay S, Schaeffer SM, DeBruyn JM, Miles CA, Reganold JP, Flury M (2020) In situ degradation of biodegradable plastic mulch films in compost and agricultural soils. Science of The Total Environment 727, 138668.
| Crossref | Google Scholar | PubMed |
Someya Y, Kondo N, Shibata M (2007) Biodegradation of poly(butylene adipate-co-butylene terephthalate)/layered-silicate nanocomposites. Journal of Applied Polymer Science 106, 730-736.
| Crossref | Google Scholar |
Tachibana K, Hashimoto K, Yoshikawa M, Okawa H (2010) Isolation and characterization of microorganisms degrading nylon 4 in the composted soil. Polymer Degradation and Stability 95, 912-917.
| Crossref | Google Scholar |
Tokiwa Y, Calabia BP, Ugwu CU, Aiba S (2009) Biodegradability of plastics. International Journal of Molecular Sciences 10, 3722-3742.
| Crossref | Google Scholar | PubMed |
Tosin M, Barbale M, Chinaglia S, Degli-Innocenti F (2020) Disintegration and mineralization of mulch films and leaf litter in soil. Polymer Degradation and Stability 179, 109309.
| Crossref | Google Scholar |
Wang H, Wei D, Zheng A, Xiao H (2015) Soil burial biodegradation of antimicrobial biodegradable PBAT films. Polymer Degradation and Stability 116, 14-22.
| Crossref | Google Scholar |
Xiao M, Shahbaz M, Liang Y, Yang J, Wang S, Chadwicka DR, Jones D, Chen J, Ge T (2021) Effect of microplastics on organic matter decomposition in paddy soil amended with crop residues and labile C: a three-source-partitioning study. Journal of Hazardous Materials 416, 126221.
| Crossref | Google Scholar | PubMed |
Yu Y, Griffin-LaHue DE, Miles CA, Hayes DG, Flury M (2021) Are micro- and nanoplastics from soil-biodegradable plastic mulches an environmental concern? Journal of Hazardous Materials Advances 4, 100024.
| Crossref | Google Scholar |
Yussuf AA, Al-Saleh MA, Al-Samhan MM, Al-Enezi ST, Al-Banna AH, Abraham G (2018) Investigation of polypropylene-montmorillonite clay nanocomposite films containing a pro-degradant additive. Journal of Polymers and the Environment 26, 275-290.
| Crossref | Google Scholar |
Zaborowska M, Wyszkowska J, Borowik A (2020) Soil microbiome response to contamination with bisphenol A, bisphenol F and bisphenol S. International Journal of Molecular Sciences 21, 3529.
| Crossref | Google Scholar | PubMed |
Zhang G, Liu D, Lin J, Kumar A, Jia K, Tian X, Yu Z, Zhu B (2023) Priming effects induced by degradable microplastics in agricultural soils. Soil Biology and Biochemistry 180, 109006.
| Crossref | Google Scholar |
Zhu Z, Fang Y, Liang Y, Li Y, Liu S, Li Y, Li B, Gao W, Yuan H, Kuzyakov Y, Wu J, Richter A, Ge T (2022) Stoichiometric regulation of priming effects and soil carbon balance by microbial life strategies. Soil Biology and Biochemistry 169, 108669.
| Crossref | Google Scholar |
Zumstein MT, Schintlmeister A, Nelson TF, Baumgartner R, Woebken D, Wagner M, Kohler H-PE, McNeill K, Sander M (2018) Biodegradation of synthetic polymers in soils: tracking carbon into CO2 and microbial biomass. Science Advances 4, eaas9024.
| Crossref | Google Scholar |