Using environmental tracers to understand soil organic carbon and soil erosion on a steep slope hillslope in south-east Australia
G. R. Hancock
A School of Environmental and Life Sciences, The University of Newcastle, Callaghan, NSW 2308, Australia.
Soil Research 61(6) 616-625 https://doi.org/10.1071/SR22263
Submitted: 17 December 2022 Accepted: 25 April 2023 Published: 26 May 2023
© 2023 The Author(s) (or their employer(s)). Published by CSIRO Publishing. This is an open access article distributed under the Creative Commons Attribution-NonCommercial-NoDerivatives 4.0 International License (CC BY-NC-ND)
Abstract
Context: It is well recognised that soil organic carbon (SOC) can be transported and deposited along the same pathways as those of soil erosion and deposition.
Aims: To examine the viability of environmental tracers 137Cs and unsupported 210Pb (210Pbex) as tools to inform soil erosion and deposition patterns as well as that of the distribution of SOC.
Methods: Multiple soil cores were collected along two transects of similar length and aspect in a steep-slope soil mantled environment in south-east Australia.
Key results: Average SOC concentration was high for both transects (~6% and 4%). SOC decreased moving downslope suggesting loss of SOC by erosion. There were strong and significant positive relationships of SOC with 137Cs and 210Pbex (both r > 0.77, P < 0.0001). At this site, SOC concentration appears related to erosion and deposition patterns.
Conclusion: The hillslope distribution of 137Cs and 210Pbex were very similar, indicating that both tracers were viable in this environment (r = 0.9, P < 0.0001). The different origins and half-lives of 137Cs and 210Pbex also demonstrate that the patterns of erosion and deposition are consistent at decadal time scales.
Implications: The use of 210Pbex provides an alternative method for understanding erosion and deposition patterns as well as that of SOC, given that the viability of 137Cs (half-life of 30.1 years) is now questionable due to no new replenishment.
Keywords: 137Cs, 210Pb, 210Pbex, carbon sequestration, lead-210, sediment transport, SOC, soil erosion.
Introduction
Understanding the movement and fate of soil organic carbon (SOC) is essential for improved management of the soil–landscape system as well as C sequestration (Murphy 2015; Minasny et al. 2017; Lal 2019). The location and movement of SOC has been shown to be related to soil erosion and deposition. Therefore, quantifying SOC soil redistribution requires an understanding of erosion and deposition processes at multiple spatial and temporal scales (Moore et al. 1993; Knighton 1998; Lal 2001, 2003, 2004; Berhe et al. 2007; Hancock et al. 2010; Quinton et al. 2010; Ruiz Sinoga et al. 2012; Gaspar and Navas 2013; Berhe et al. 2014; Kirkels et al. 2014; Murphy 2015; Doetterl et al. 2016; Hoyle et al. 2016).
Here, environmental tracers (137Cs and unsupported 210Pb also known as 210Pbex) are used to provide insights to sediment transport and SOC. Environmental tracers, particularly 137Cs are well understood and well used to understanding erosion and deposition patterns and erosion rates (Ritchie and McHenry 1975; Longmore et al. 1983; McFarlane et al. 1992; Loughran 1994; Loughran et al. 2002, 2004; Zapata et al. 2002; Walling et al. 2003; Zapata 2003; Li et al. 2006; Zhang et al. 2006; Fukuyama et al. 2008; Olley et al. 2013; Özden et al. 2013; Teramage et al. 2013; Mabit et al. 2014; Fissore et al. 2017).
Using both 137Cs and 210Pbex provides information over different time periods (He and Walling 1997; He et al. 2002; Li et al. 2003; Fukuyama et al. 2008; Mabit et al. 2009, 2014; Kato et al. 2010; Teramage et al. 2013). While 137Cs has been extensively used to determine soil erosion and deposition patterns, 210Pbex has been less commonly used (Lewis 1977; Walling et al. 2003; Walling et al. 2011). Further, it was shown that 137Cs and 210Pbex can be bound to soil organic and inorganic materials and be used as tracers (Lewis 1977; Dorr 1995). Teramage et al. (2013) found a stronger affinity between SOC and 210Pbex than for SOC and 137Cs. Few studies have examined the relationship between these tracers and SOC, particularly 210Pbex in the Australian environment.
As the last atmospheric nuclear test was 1972, 137Cs provides information on erosion and deposition patterns over an approximate 50-year period. In contrast, 210Pb is derived from naturally occurring 222Rn (Bunzl et al. 1995) and provides information over an approximate 100–200 year period (Walling and He 1999; Zapata et al. 2002; Walling et al. 2003; Zapata 2003; Zhang et al. 2006; Mabit et al. 2014). Both tracers therefore provide information at two different temporal scales (Wallbrink et al. 1994; Walling and He 2001; Li et al. 2003; Walling et al. 2003; Kato et al. 2010).
Here, both 137Cs and 210Pbex are used to understand SOC and soil erosion and deposition patterns on a relatively steep slope (~30%) in south-east Australia. This study forms part of a long-term investigation of hillslope geomorphology in the south-east region of Australia (Rüdiger et al. 2007; Martinez et al. 2009, 2010; Hancock et al. 2010, 2015; Wells et al. 2012; Wells and Hancock 2014; Chen et al. 2015; Kunkel et al. 2016, 2019; Hancock and Wells 2021). There appears to be few studies where the two tracers have been directly used and compared in this way.
The aims are (1) to report and understand the patterns of the environmental tracers (137Cs and 210Pbex) and SOC in a relatively high rainfall steep-slope environment and (2) assess the viability of the environmental tracers as tools to provide insights concerning soil erosion and deposition patterns as well as hillslope SOC concentration.
Study site
The study site (Springhills) is located in the headwaters of the Krui River catchment (~562 km2), New South Wales (NSW), Australia (Fig. 1), a tributary of the Goulburn River which joins the Hunter River and discharges at Newcastle. The site is underlain with Tertiary basalt of the Liverpool Range beds and forms part of the Merriwa Plateau. The basaltic soils are highly fertile. The study site is located on the property ‘Springhills’ and has undulating to steep topography typical of the local headwaters with relief at the site of approximately 500 m.
Original vegetation has been mostly cleared over the past 150 years and replaced with improved and natural pasture. While steep, landuse is well suited to cattle and sheep and cattle grazing (Story et al. 1963; Kovac and Lawrie 1991). Vegetation species include Austrostipa aristiglumis (plains grass) and various Poa (tussock) species (Fig. 2).
View from the Upper transect looking south down the Krui valley (top) and looking upslope (bottom). The white tape is the location of the sampling.
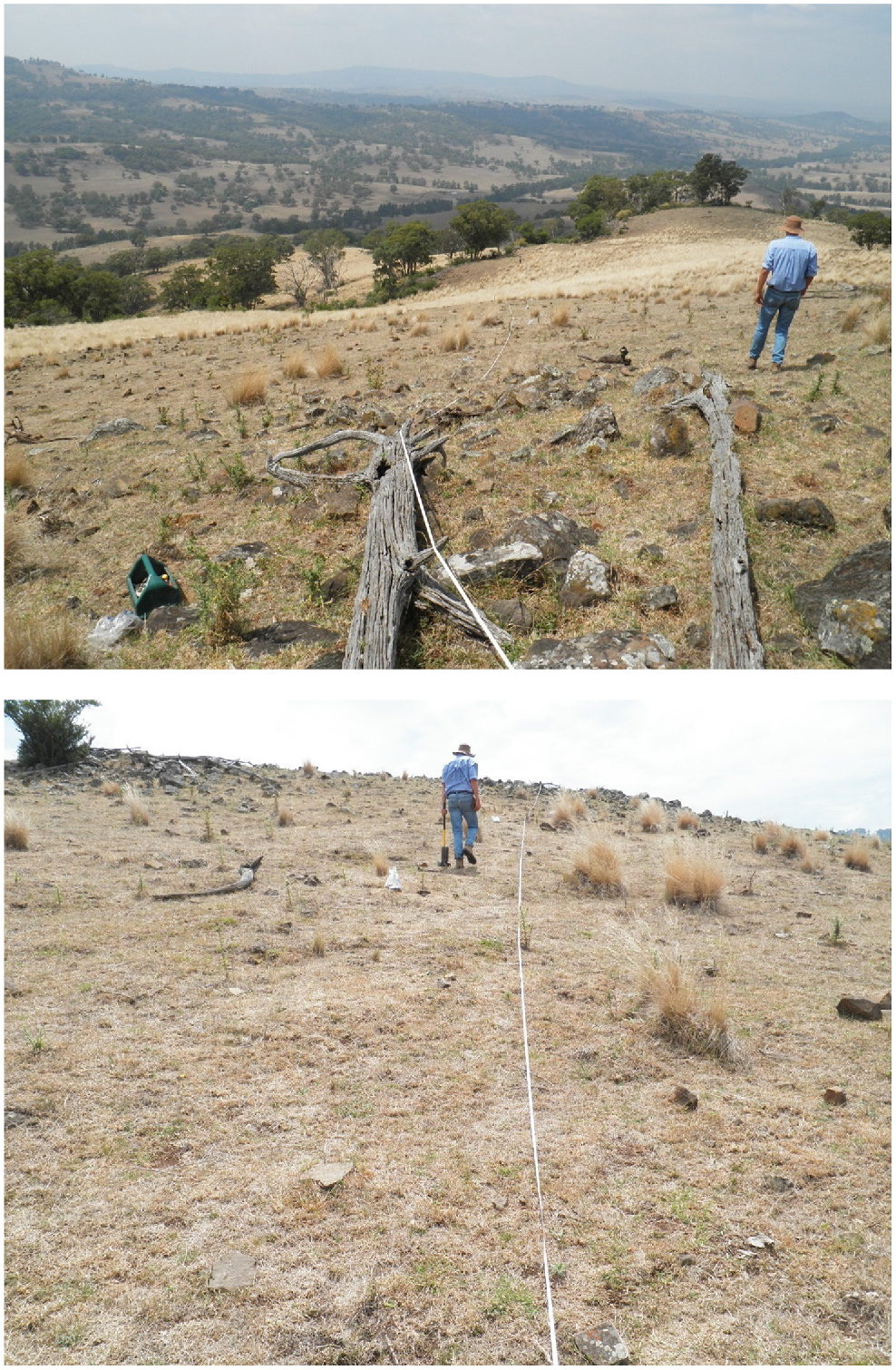
Climate is classified as temperate dominated by continental influence (Kovac and Lawrie 1991). Annual average rainfall is approximately 1000 mm. Mean monthly minimum and maximum air temperatures are 3°C (winter) and 16°C (summer), and 17°C (winter) and 30°C (summer), respectively (www.bom.gov.au).
Here two sites with approximately the same easterly aspect, relief, slope and slope lengths and management (pasture and grazing) but at two different elevations were examined (Figs 2, 3). A transect-based sampling methodology was employed. The two transects were named ‘Upper’ and ‘Lower’ transects (Table 1). The Upper transect at an elevation of 830 m was 150 m long and ran a ridgeline to a zero-order drainage line at its base (Fig. 2; Table 1). The 160-m-long Lower transect (highest elevation approximately 535 m) ran from ridgeline to a creek flat (Fig. 3). Both sites had similar vegetation cover (native and improved pasture), uniform soil type and consistent landuse (grazing).
Methods
Sampling and laboratory methodology
Soil samples were collected at regular intervals using a transect approach ensuring that the whole toposequence was sampled (Pennock and Appleby 2002). Steel cores were used for sampling (95 mm internal diameter and length 210 mm). For insertion of the core, a cap or ‘dolly’ was placed on top of the steel core and a hammer manually used to insert the core to the core maximum depth or point of refusal. Cores were extracted using vice-grips to twist the core and lift it from the ground. Each soil sample was double-bagged and labelled for transport.
Soil samples were processed at the University of Newcastle Soils Laboratory. Soil samples were first weighed and dried in a 40°C oven for at least 7 days. Each sample was disaggregated mechanically by hand using a mortar and pestle and passed through a 2 mm sieve to separate fine and coarse fractions and mass was recorded. Sand, silt and clay contents of the <2 mm fraction were determined by the hydrometer method (Smith and Atkinson 1975). Rock fraction here is defined as the >2 mm size fraction. Subsamples for total C assessment were sent to the Environmental Analysis Laboratory at Southern Cross University, Lismore, NSW (LECO dry combustion method).
Environmental tracers
Environmental radionuclides such as 137Cs and 210Pbex are useful for providing details on erosion and deposition across multiple spatial and temporal scales (Olley et al. 2013). The 137Cs can be used to understand medium-term (~50 year old) soil erosion and deposition patterns (Ritchie and McHenry 1975; Longmore et al. 1983; Campbell et al. 1988; McFarlane et al. 1992; Loughran 1994; Loughran et al. 2002, 2004; Krause et al. 2003; Zapata 2003; Martinez et al. 2009; Gaspar and Navas 2013; Hancock et al. 2015). 137Cs in soil was supplied as fallout from atmospheric testing of nuclear weapons and nuclear accidents (Teramage et al. 2013, 2015) and is adsorbed to clay after atmospheric fallout. There has been no input of 137Cs to the Australian environment since atmospheric testing of nuclear weapons stopped in the early 1970s. The half-life of 137Cs is relatively short (30.1 years); however, it is still detectable in many parts of Australia (including this site). Several studies have used this approach in the general study area (Martinez et al. 2009; Hancock et al. 2015; Hancock and Wells 2021).
Derived from the decay of gaseous 222Rn, 210Pbex is a naturally occurring radionuclide from the 238U decay series. Some 222Rn in soil diffuses into the atmosphere and decays to 210Pb, and subsequent fallout of 210Pb to the landscape surface provides an input that is not in equilibrium (excess) with its parent 226Ra (Walling et al. 2003; Zapata 2003; Gaspar et al. 2017). Fallout 210Pb is commonly termed unsupported or excess 210Pb, when incorporated into soils or sediments in order to distinguish it from the 210Pb produced in situ by the decay of 226Ra. Given its continuous fallout, 210Pbex can provide information over an approximate 100-year period (Zapata et al. 2002; Zapata 2003; Walling et al. 2003; Zhang et al. 2006; Mabit et al. 2014).
For the 137Cs and 210Pbex analysis, soil core samples (<2 mm) of mass 400–1000 g were stored for a minimum of 28 days to ensure equilibrium between 226Ra and its daughter 222Rn (an inert gas with half-life 3.8 days) was established. The 137Cs and 210Pbex concentrations were then measured using a hyper-pure coaxial Ge detector coupled to a multi-channel analyser. The 210Pbex concentrations were calculated by subtracting 226Ra-supported 210Pb concentration from the total 210Pb concentration. The concentrations of 137Cs were determined using peak at 662 keV, of 210Pb at 46.5 keV and of 226Ra at 609.3 keV. The counting time in all cases was over 80 000 s, which provided an analytical precision of ±6% for 137Cs and ±10% for 210Pbex.
Topographic data
A digital elevation model for the Upper and Lower hillslopes was created by systematic traverse using a Trimble 4700 base station and rover (Differential Global Positioning System or DGPS). Coordinates were automatically recorded every 2 m. Additional points were collected along and around each hillslope transect. These ungridded data were gridded using ordinary kriging to a regular 1 m grid. System accuracy was approximately 20 mm in the X and Y (horizontal) and 25 mm in the Z (vertical) directions by comparison with local fixed survey points. Topographic attributes such as slope, hillslope distance, upslope area and the topographic wetness index (TWI) (Beven and Kirkby 1979) were derived from these data. Sample points were recorded using the DGPS also.
Results
Hillslope and soil properties
The transects both had linear hillslope profiles with approximately the same slope (~30%) and length (~150 m) (Fig. 4, Table 1). At the time of sampling vegetation consisted of low grass cover (Figs 2, 3).
Soil texture (sand, silt and clay), in particular clay, can influence SOC concentration. Clay for the Upper transect (average = 35%, s.d. = 10) was significantly lower (P < 0.05) compared to the Lower transect (average = 49%, s.d. = 12). There was no distinct observable pattern in silt and sand along either transect nor with elevation or distance from the divide (Fig. 4). However, clay was negatively (non-significantly) (P > 0.05) correlated with elevation or distance from the catchment divide. That is, clay increased down the hillslope, but being non-significant may be due to chance. For both transects, SOC was significantly and negatively correlated with clay (P < 0.005).
For both transects, SOC concentration was ‘high’ (3.0–5.15%) to ‘very high’ (>5.15%) (Hazelton and Murphy 2007), being significantly higher (P < 0.05) for the Upper than the Lower transect (Table 2). The SOC for both transects was significantly negatively correlated (P < 0.05) with elevation and distance from the divide (Table 3). Combining both data sets also showed a significant correlation. There was no relationship of SOC with slope, and of SOC with upslope area or TWI for any of the data sets.
No. of samples | Average | Standard deviation | Minimum | Maximum | |
---|---|---|---|---|---|
Upper | 21 | 5.53 | 1.39 | 3.15 | 8.26 |
Lower | 22 | 4.09 | 1.44 | 1.35 | 6.71 |
Elevation (m) | Distance (m) | SOC (%) | Clay (%) | |
---|---|---|---|---|
Lower | ||||
137Cs | 0.6*** | −0.61*** | 0.83*** | −0.57* |
210Pbex | 0.62** | −0.63** | 0.82*** | −0.58** |
Upper | ||||
137Cs | 0.49* | −0.48* | 0.80* | −0.64** |
210Pbex | 0.45* | −0.42* | 0.82*** | −0.61** |
Lower and Upper data sets combined | ||||
137Cs | 0.54**,A | −0.54** | 0.80*** | −0.59*** |
210Pbex | 0.51**,A | −0.50** | 0.78*** | −0.56*** |
***P < 0.0001; **P < 0.005; *P < 0.05.
AElevation has been normalised for comparison.
Environmental tracers
For both transects, the concentrations of 137Cs, 210Pbex and SOC followed similar patterns (Figs 5, 6). The Upper and Lower transects both had the highest SOC and 137Cs concentrations at the top of the transect, which rapidly reduced at 20–50 m distance from the divide. A similar pattern was observed for 210Pbex.
The 137Cs was positively and significantly correlated with 210Pbex for both the Upper (r = 0.92, P < 0.0001) and Lower (r = 0.94, P < 0.0001) data sets (Fig. 7). For all data sets, SOC was significantly positively correlated with 137Cs and 210Pbex (Table 3, Fig. 8). This suggests that a lower SOC concentration is related to erosion while a higher SOC concentration is related to areas of deposition. Both 137Cs and 210Pbex were positively correlated with elevation and negatively correlated with distance from the catchment divide for both the Upper and Lower data sets (Table 3).
Combining both data sets, 137Cs and 210Pbex were positively correlated with normalised elevation (elevation for both Upper and Lower transects was scaled between 0 and 1 for comparison) and negatively correlated with distance from the divide (Table 3). Both 137Cs and 210Pbex were significantly positively correlated with SOC (%) and negatively correlated with clay.
Discussion
SOC and topography
Both transects demonstrated a landscape with high SOC concentrations suggesting good structural stability (Hazelton and Murphy 2007). Here SOC was significantly higher for the Upper transect yet clay was significantly lower. Combining both data sets demonstrated that the relationship with elevation was consistent across the varying elevation regimes of the two transects. We can only speculate that more rainfall is received at higher altitudes and together with lower temperatures (the Upper transect is ~250 m higher than the Lower transect), decay rates are slower and more SOC can be stored. Given the high SOC concentration, it is possible that the soil is saturated and that there is an export of SOC as suggested by the pattern of environmental tracers. On uncultivated hillslopes (albeit at lower slopes and lower rainfall but with similar management), no relationship was observed between SOC and environmental tracers over a number of years (Martinez et al. 2010; Hancock et al. 2015). Whether the soil at Springhills (and other sites) can increase SOC is open to question (Minasny et al. 2017).
We also found that despite a significantly higher SOC concentration for the Upper transect, clay percentage was significantly lower. This inverse relationship contrasts to much of the literature, where it is generally accepted that clays inhibit microbial and physical oxidation of SOC (Oades 1988; Arrouays et al. 1995; Grigal and Berguson 1998; Percival et al. 2000; Müller and Höper 2004; Wei et al. 2014; O’Brien et al. 2015; Singh et al. 2016). However, this understanding is not clear for all environments. Other studies in the area examining the Krui and Merriwa catchments also found that SOC decreased with increasing clay (Kunkel et al. 2019). We are currently examining this issue at other areas in the catchment with lower rainfall and different soils and texture (Martinez et al. 2009).
SOC and environmental tracers
Both transects had decreasing SOC down the transect (Table 3). There was a significant negative relationship of SOC with elevation as well as with distance down the hillslope (Table 3). This reduction is likely a result of the erosion and deposition processes occurring as demonstrated by 137Cs and 210Pbex (discussed further below). Therefore, SOC at this site is being lost from the hillslope at rates commensurate with the erosional loss.
Many studies have speculated or proposed a relationship between SOC and soil erosion and deposition (Lewis 1977; Dorr 1995; Kuhn et al. 2009; Martinez et al. 2009; Gaspar and Navas 2013; Gaspar et al. 2019). Several have employed 137Cs as a surrogate for erosion and deposition (Mabit et al. 2008). Here, a consistent and robust relationship between SOC and the environmental tracers 137Cs and 210Pbex was demonstrated (Walling et al. 2003; Fukuyama et al. 2008; Teramage et al. 2013, 2015; Özden et al. 2013).
The 137Cs and 210Pbex concentrations observed here represent erosion and deposition patterns during the past (approximately) 50 and 100 years, respectively (Zapata et al. 2002; Walling et al. 2003; Zhang et al. 2006; Teramage et al. 2013; Mabit et al. 2014; Gaspar et al. 2019). The author believes that this is the first report of 210Pbex used to examine the spatial and temporal distribution of SOC in a grazing pasture environment in Australia. The consistency of patterns between the two tracers suggests that the same erosion and deposition patterns occur across different time scales (Walling et al. 2003). The advantage of 210Pbex is that it is a naturally occurring product that is continually being replenished, and with a half-life of 22.3 years it appears to be a viable alternative to 137Cs. Further, given that 137Cs fallout terminated in the early 1970s and 210Pbex is continually replenished, the findings here suggest that SOC and its relationship with erosion and deposition is consistent at decadal time scales (Walling et al. 2003). With the consistent replenishment of 210Pbex, the patterns could be considered to be a function of the present hillslope behaviour, not an average of the last 50 years as provided by the 137Cs method. Therefore, the tracers have the ability for assessing and detecting change in SOC transport patterns. However, Kato et al. (2010) found that the use of 210Pbex may be problematic for soil erosion understanding in semi-arid areas (although not the environment examined here). This needs to be explored further in Australia. This suggests that the SOC patterns in relation to erosion and deposition are robust and consistent at longer than the 50-year history of 137Cs and 210Pbex in this environment (Walling et al. 2003; Mabit et al. 2009; Gaspar et al. 2019).
Study limitations and further work
Understanding SOC movement can be a complex exercise as SOC can be transported by a variety of surface and subsurface processes at different rates (Hassink 1997; Oades 1988; Rumpel and Kögel-Knabner 2011; Schwanghart and Jarmer 2011; Ruiz Sinoga et al. 2012; Gaspar and Navas 2013; Jandl et al. 2014). The environmental tracers here are well-accepted and understood (Zapata et al. 2002; Zapata 2003). These tracers provide information on erosion and deposition at decadal time scales and therefore reflect longer-term trends.
The use of the two different (and unrelated) tracers suggest that the observed patterns are robust. The method can be applied at a site after a major storm event to assess any change (Hancock et al. 2015, 2019). Interestingly, Hancock et al. (2015, 2019) found significant differences in 137Cs concentration between 2006 and 2014 in a subcatchment of the Krui as well as the entire Krui catchment. This difference was attributed to a major storm event in the area (Mills et al. 2010). However, it is recognised that the information provides little insight into short-term or storm-event time scale processes. Short-term tracers such as 7Be would also provide insight into storm-scale events (Zapata et al. 2002; Zapata 2003; Taylor et al. 2013). The 210Pbex is continually replenished and offers the opportunity to determine decadal-scale erosion rates. While there are conversion models available to determine erosion rates, these models have not been fully developed and evaluated, particularly for Australia. This is a major undertaking and the focus of ongoing work by the research team.
Conclusion
We examined a relatively high slope and high rainfall soil mantled environment for the spatial and temporal movement of SOC along two hillslope transects with similar lengths, slopes and aspect. Environmental tracers (137Cs and 210Pbex) were used to understand the movement of SOC. The SOC concentrations were high, indicating good structural stability, soil productivity and health. We found that the movement of SOC was related to soil erosion and deposition patterns. In particular, 210Pbex was shown to be a viable tracer to understand SOC patterns and this appears to be the first time that 210Pbex has been used in this way.
Importantly, the results demonstrate that soil C concentration is related to erosion and deposition processes at decadal time scales, suggesting a continuity of sediment transport and deposition processes despite several major droughts, different land ownership (over 150 years) and likely differences in land management. The approach demonstrated here using environmental tracers can be readily applied at other sites to further enhance our knowledge of SOC distribution and transport.
Declaration of funding
This research was largely supported by Australian Research Council Discovery Grants (DP 476 0556941: ‘Carbon, nutrient and sediment dynamics in a semi-arid catchment’ and 477 DP110101216: ‘A next generation spatially distributed model for soil profile dynamics and pedogenesis incorporating soil geochemistry and organic matter’).
Acknowledgements
The Cameron family is thanked for access to their property and their ongoing support.
References
Arrouays D, Vion I, Kicin JL (1995) Spatial analysis and modeling of topsoil carbon storage in temperate forest humic loamy soils of France. Soil Science 159(3), 191-198.
| Crossref | Google Scholar |
Berhe AA, Harte J, Harden JW, Torn MS (2007) The significance of the erosion-induced terrestrial carbon sink. BioScience 57, 337-346.
| Crossref | Google Scholar |
Berhe AA, Arnold C, Stacy E, Lever R, McCorkle E, Araya SN (2014) Soil erosion controls on biogeochemical cycling of carbon and nitrogen. Nature Education Knowledge 5(8), 2.
| Google Scholar |
Beven KJ, Kirkby MJ (1979) A physically based, variable contributing area model of basin hydrology. Hydrological Sciences Bulletin 24(1), 43-69.
| Crossref | Google Scholar |
Bunzl K, Kretner R, Schramel P, Szeles M, Winkler R (1995) Speciation of 238U, 226Ra, 210Pb, 228Ra, and stable Pb in the soil near an exhaust ventilating shaft of a uranium mine. Geoderma 67, 45-53.
| Crossref | Google Scholar |
Campbell BL, Loughran RJ, Elliott GL (1988) A method for determining sediment budgets using caesium-137. Paper presented at Sediment budgets, Porto Alegre Symposium (December 1988)’. International Association of Hydrological Sciences (IAHS) Publ. No. 174 In ‘ pp. 171-179 (IAHS).
| Google Scholar |
Chen M, Willgoose GR, Saco PM (2015) Evaluation of the hydrology of the IBIS land surface model in a semi-arid catchment. Hydrological Processes 29, 653-670.
| Crossref | Google Scholar |
Doetterl S, Berhe AA, Nadeu E, Wang Z, Sommer M, Fiener P (2016) Erosion, deposition and soil carbon: a review of process-level controls, experimental tools and models to address C cycling in dynamic landscapes. Earth-Science Reviews 154, 102-122.
| Crossref | Google Scholar |
Dorr H (1995) Application of 210Pb in soils. Journal of Paleolimnology 13, 157-168.
| Crossref | Google Scholar |
Fissore C, Dalzell BJ, Berhe AA, Voegtle M, Evans M, Wu A (2017) Influence of topography on soil organic carbon dynamics in a Southern California grassland. CATENA 149, 140-149.
| Crossref | Google Scholar |
Fukuyama T, Onda Y, Takenaka C, Walling DE (2008) Investigating erosion rates within a Japanese cypress plantation using Cs-137 and Pb-210ex measurements. Journal of Geophysical Research 113, F02007.
| Crossref | Google Scholar |
Gaspar L, Navas A (2013) Vertical and lateral distributions of 137Cs in cultivated and uncultivated soils on Mediterranean hillslopes. Geoderma 207–208, 131-143.
| Crossref | Google Scholar |
Gaspar L, Webster R, Navas A (2017) Fate of 210Pbex fallout in soil under forest and scrub of the central Spanish Pre-Pyrenees. European Journal of Soil Science 68, 259-269.
| Crossref | Google Scholar |
Gaspar L, Quijano L, Lizaga I, Navas A (2019) Effects of land use on soil organic and inorganic C and N at 137Cs traced erosional and depositional sites in mountain agroecosystems. CATENA 181, 104058.
| Crossref | Google Scholar |
Grigal DF, Berguson WE (1998) Soil carbon changes associated with short-rotation systems. Biomass and Bioenergy 14(4), 371-377.
| Crossref | Google Scholar |
Hancock GR, Wells T (2021) Predicting soil organic carbon movement and concentration using a soil erosion and landscape evolution model. Geoderma 382, 114759.
| Crossref | Google Scholar |
Hancock GR, Murphy D, Evans KG (2010) Hillslope and catchment scale soil organic carbon concentration: an assessment of the role of geomorphology and soil erosion in an undisturbed environment. Geoderma 155, 36-45.
| Crossref | Google Scholar |
Hancock GR, Wells T, Martinez C, Dever C (2015) Soil erosion and tolerable soil loss: insights into erosion rates for a well-managed grassland catchment. Geoderma 237–238, 256-265.
| Crossref | Google Scholar |
Hancock GR, Kunkel V, Wells T, Martinez C (2019) Soil organic carbon and soil erosion – understanding change at the large catchment scale. Geoderma 343, 60-71.
| Crossref | Google Scholar |
Hassink J (1997) The capacity of soils to preserve organic C and N by their association with clay and silt particles. Plant and Soil 191(1), 77-87.
| Crossref | Google Scholar |
He Q, Walling DE (1997) The distribution of fallout 137Cs and 210Pb in undisturbed and cultivated soils. Applied Radiation and Isotopes 48(5), 677-690.
| Crossref | Google Scholar |
He Q, Walling DE, Wallbrink PJ (2002) Alternative methods and radionuclides for use in soil-erosion and sedimentation investigations. In ‘Handbook for the assessment of soil erosion and sedimentation using environmental radionuclides’. (Ed. F. Zapata) pp. 185–215. (Kluwer Academic Publishers: Dordrecht, Netherlands)
Hoyle FC, O’Leary RA, Murphy DV (2016) Spatially governed climate factors dominate management in determining the quantity and distribution of soil organic carbon in dryland agricultural systems. Scientific Reports 6, 31468.
| Crossref | Google Scholar |
Jandl R, Rodeghiero M, Martinez C, Cotrufo MF, Bampa F, Van Wesemael B, Harrison RB, Guerrini IA, Richter Dd, Jr, Rustad L, Lorenz K, Chabbi A, Miglietta F (2014) Current status, uncertainty and future needs in soil organic carbon monitoring. Science of The Total Environment 468–469, 376-383.
| Crossref | Google Scholar |
Kato H, Onda Y, Tanaka Y (2010) Using 137Cs and 210Pbex measurements to estimate soil redistribution rates on semi-arid grassland in Mongolia. Geomorphology 114(4), 508-519.
| Crossref | Google Scholar |
Kirkels FMSA, Cammeraat LH, Kuhn NJ (2014) The fate of soil organic carbon upon erosion, transport and deposition in agricultural landscapes – a review of different concepts. Geomorphology 226, 94-105.
| Crossref | Google Scholar |
Krause AK, Loughran RJ, Kalma JD (2003) The use of caesium-137 to assess surface soil erosion status in a water-supply catchment in the Hunter Valley, New South Wales, Australia. Australian Geographical Studies 41(1), 73-84.
| Crossref | Google Scholar |
Kuhn NJ, Hoffmann T, Schwanghart W, Dotterweich M (2009) Agricultural soil erosion and global carbon cycle: controversy over? Earth Surface Processes and Landforms 34, 1033-1038.
| Crossref | Google Scholar |
Kunkel V, Wells T, Hancock GR (2016) Soil temperature dynamics at the catchment scale. Geoderma 273(2016), 32-44.
| Crossref | Google Scholar |
Kunkel V, Hancock GR, Wells T (2019) Large catchment-scale spatiotemporal distribution of soil organic carbon. Geoderma 334, 175-185.
| Crossref | Google Scholar |
Lal R (2001) Soil degradation by erosion. Land Degradation & Development 12(6), 519-539.
| Crossref | Google Scholar |
Lal R (2003) Soil erosion and the global carbon budget. Environment International 29, 437-450.
| Crossref | Google Scholar |
Lal R (2004) Soil carbon sequestration to mitigate climate change. Geoderma 123(1–2), 1-22.
| Crossref | Google Scholar |
Lal R (2019) Accelerated soil erosion as a source of atmospheric CO2. Soil and Tillage Research 188, 35-40.
| Crossref | Google Scholar |
Lewis DM (1977) The use of 210Pb as a heavy metal tracer in the Susquehanna River system. Geochimica et Cosmochimica Acta 41(11), 1557-1564.
| Crossref | Google Scholar |
Li Y, Poesen J, Yang JC, Fu B, Zhang JH (2003) Evaluating gully erosion using 137Cs and 210Pb/137Cs ratio in a reservoir catchment. Soil and Tillage Research 69, 107-115.
| Crossref | Google Scholar |
Li Y, Zhang QW, Reicosky DC, Bai LY, Lindstrom MJ, Li L (2006) Using 137Cs and 210Pbex for quantifying soil organic carbon redistribution affected by intensive tillage on steep slopes. Soil and Tillage Research 86, 176-184.
| Crossref | Google Scholar |
Longmore ME, O’Leary BM, Rose CW, Chandica AL (1983) Mapping soil erosion and accumulation with the fallout isotope caesium-137. Soil Research 21, 373-385.
| Crossref | Google Scholar |
Loughran RJ (1994) The use of the environmental isotope caesium-137 for soil erosion and sedimentation studies. Trends in Hydrology 1, 149-167.
| Google Scholar |
Loughran RJ, Elliott GL, McFarlane DJ, Campbell BL (2004) A survey of soil erosion in Australia using caesium-137. Australian Geographical Studies 42(2), 221-233.
| Crossref | Google Scholar |
Mabit L, Bernard C, Makhlouf M, Laverdière MR (2008) Spatial variability of erosion and soil organic matter content estimated from 137Cs measurements and geostatistics. Geoderma 145, 245-251.
| Crossref | Google Scholar |
Mabit L, Klik A, Benmansour M, Toloza A, Geisler A, Gerstmann UC (2009) Assessment of erosion and deposition rates within an Austrian agricultural watershed by combining 137Cs, 210Pbex and conventional measurements. Geoderma 150, 231-239.
| Crossref | Google Scholar |
Mabit L, Benmansour M, Abril JM, Walling DE, Meusburger K, Iurian AR, Bernard C, Tarján S, Owens PN, Blake WH, Alewell C (2014) Fallout 210Pb as a soil and sediment tracer in catchment sediment budget investigations: a review. Earth-Science Reviews 138, 335-351.
| Crossref | Google Scholar |
Martinez C, Hancock GR, Kalma JD (2009) Comparison of fallout radionuclide (caesium-137) and modelling approaches for the assessment of soil erosion rates for an uncultivated site in south-eastern Australia. Geoderma 151, 128-140.
| Crossref | Google Scholar |
Martinez C, Hancock GR, Kalma JD (2010) Relationships between 137Cs and soil organic carbon (SOC) in cultivated and never-cultivated soils: an Australian example. Geoderma 158, 137-147.
| Crossref | Google Scholar |
Mcfarlane DJ, Loughran RJ, Campbell BL (1992) Soil erosion of agricultural land in Western Australia estimated by cesium-137. Soil Research 30, 533-546.
| Crossref | Google Scholar |
Minasny B, Malone BP, McBratney AB, Angers DA, Arrouays D, Chambers A, Chaplot V, Chen Z-S, Cheng K, Das BS, Field DJ, Gimona A, Hedley CB, Hong SY, Mandal B, Marchant BP, Martin M, McConkey BG, Mulder VL, O’Rourke S, Richer-de-Forges AC, Odeh I, Padarian J, Paustian K, Pan G, Poggio L, Savin I, Stolbovoy V, Stockmann U, Sulaeman Y, Tsui C-C, Vågen T-G, van Wesemael B, Winowiecki L (2017) Soil carbon 4 per mille. Geoderma 292(15), 59-86.
| Crossref | Google Scholar |
Moore ID, Gessler PE, Nielsen GA, Peterson GA (1993) Soil attribute prediction using terrain analysis. Soil Science Society of America Journal 57, 443-452.
| Crossref | Google Scholar |
Murphy BW (2015) Impact of soil organic matter on soil properties – a review with emphasis on Australian soils. Soil Research 53(6), 605-635.
| Crossref | Google Scholar |
Müller T, Höper H (2004) Soil organic matter turnover as a function of the soil clay content: consequences for model applications. Soil Biology and Biochemistry 36(6), 877-888.
| Crossref | Google Scholar |
Oades JM (1988) The retention of organic matter in soils. Biogeochemistry 5(1), 35-70.
| Crossref | Google Scholar |
Olley J, Burton J, Smolders K, Pantus F, Pietsch T (2013) The application of fallout radionuclides to determine the dominant erosion process in water supply catchments of subtropical South-east Queensland, Australia. Hydrological Processes 27, 885-895.
| Crossref | Google Scholar |
O’Brien SL, Jastrow JD, Grimley DA, Gonzalez-Meler MA (2015) Edaphic controls on soil organic carbon stocks in restored grasslands. Geoderma 251–252, 117-123.
| Crossref | Google Scholar |
Özden B, Uğur A, Esetlili T, Esetlili BC, Kurucu Y (2013) Assessment of the effects of physical–chemical parameters on 210Po and 210Pb concentrations in cultivated and uncultivated soil from different areas. Geoderma 192, 7-11.
| Crossref | Google Scholar |
Percival HJ, Parfitt RL, Scott NA (2000) Factors controlling soil carbon levels in New Zealand grasslands is clay content important? Soil Science Society of America Journal 64, 1623-1630.
| Crossref | Google Scholar |
Quinton JN, Govers G, Van Oost K, Bardgett RD (2010) The impact of agricultural soil erosion on biogeochemical cycling. Nature Geoscience 3, 311-314.
| Crossref | Google Scholar |
Ritchie JC, McHenry JR (1975) Fallout Cs-137: a tool in conservation research. Journal of Soil and Water Conservation 30(341), 283-286.
| Google Scholar |
Ruiz Sinoga JD, Pariente S, Diaz AR, Martinez Murillo JF (2012) Variability of relationships between soil organic carbon and some soil properties in Mediterranean rangelands under different climatic conditions (South of Spain). CATENA 94, 17-25.
| Crossref | Google Scholar |
Rumpel C, Kögel-Knabner I (2011) Deep soil organic matter – a key but poorly understood component of terrestrial C cycle. Plant and Soil 338, 143-158.
| Crossref | Google Scholar |
Rüdiger C, Hancock G, Hemakumara HM, Jacobs B, Kalma JD, Martinez C, Thyer M, Walker JP, Wells T, Willgoose GR (2007) Goulburn River experimental catchment data set. Water Resources Research 43, W10403.
| Crossref | Google Scholar |
Schwanghart W, Jarmer T (2011) Linking spatial patterns of soil organic carbon to topography – A case study from south-eastern Spain. Geomorphology 126(1–2), 252-263.
| Crossref | Google Scholar |
Singh M, Sarkar B, Biswas B, Churchman J, Bolan NS (2016) Adsorption-desorption behavior of dissolved organic carbon by soil clay fractions of varying mineralogy. Geoderma 280, 47-56.
| Crossref | Google Scholar |
Taylor A, Blake WH, Smith HG, Mabit L, Keith-Roach MJ (2013) Assumptions and challenges in the use of fallout beryllium-7 as a soil and sediment tracer in river basins. Earth-Science Reviews 126, 85-95.
| Crossref | Google Scholar |
Teramage MT, Onda Y, Kato H, Wakiyama Y, Mizugaki S, Hiramatsu S (2013) The relationship of soil organic carbon to 210Pbex and 137Cs during surface soil erosion in a hillslope forested environment. Geoderma 192, 59-67.
| Crossref | Google Scholar |
Teramage MT, Onda Y, Wakiyama Y, Kato H, Kanda T, Tamura K (2015) Atmospheric 210Pb as a tracer for soil organic carbon transport in a coniferous forest. Environmental Science: Processes & Impacts 17, 110.
| Crossref | Google Scholar |
Walling DE, He Q (1999) Improved models for estimating soil erosion rates from cesium-137 measurements. Journal of Environmental Quality 28(2), 611-622.
| Crossref | Google Scholar |
Walling DE, He Q (2001) Models for converting 137Cs measurements to estimates of soil redistribution rates on cultivated and uncultivated soils, estimating bomb-derived 137Cs reference inventories (including software for model implementation). A contribution to the IAEA Coordinated Research Programmes on Soil Erosion (D1.50.05) and Sedimentation (F3.10.01). p. 32. University of Exeter, UK.
Walling DE, Collins AL, Sichingabula HM (2003) Using unsupported lead-210 measurements to investigate soil erosion and sediment delivery in a small Zambian catchment. Geomorphology 52, 193-213.
| Crossref | Google Scholar |
Walling DE, Zhang Y, He Q (2011) Models for deriving estimates of erosion and deposition rates from fallout radionuclide (caesium-137, excess lead-201, and beryllium-7) measurements and the development of user friendly software for model implementation. In ‘Impact of soil conservation measures on erosion control and soil quality’. IAEA-TECDOC-1665. pp. 11–33. (International Atomic Energy Agency: Vienna, Austria)
Wei H, Guenet B, Vicca S, Nunan N, Asard H, AbdElgawad H, Shen W, Janssens IA (2014) High clay content accelerates the decomposition of fresh organic matter in artificial soils. Soil Biology and Biochemistry 77, 100-108.
| Crossref | Google Scholar |
Wells T, Hancock G (2014) Comparison of vertical transport of 137Cs and organic carbon in agricultural cracking soils. Geoderma 214–215, 228-238.
| Crossref | Google Scholar |
Wells T, Hancock GR, Dever C, Murphy D (2012) Prediction of vertical soil organic carbon profiles using soil properties and environmental tracer data at an untilled site. Geoderma 170, 337-346.
| Crossref | Google Scholar |
Zapata F (2003) Introduction. Soil and Tillage Research 69(2003), 1-2.
| Crossref | Google Scholar |
Zhang X, Qi Y, Walling DE, He X, Wen A, Fu J (2006) A preliminary assessment of the potential for using 210Pbex measurement to estimate soil redistribution rates on cultivated slopes in the Sichuan Hilly Basin of China. CATENA 68, 1-9.
| Crossref | Google Scholar |