Availability of phosphorus and nitrogen from modified mono-ammonium phosphate (MAP) fertiliser compounds
Jonathan W. McLachlan
A
Abstract
Moderating nutrient release to match plant requirements more closely can improve nutrient use efficiency. The formation of lower-solubility ammonium salts may be a simple and cost-effective way to slow the release of nitrogen (N) from fertiliser sources. Several modified mono-ammonium phosphate (MAP) fertiliser compounds were prepared by adding magnesium silicate to regular MAP fertiliser and reconstituting the granules. This process results in the formation of schertelite that may potentially slow the release of both N and phosphate to soil solution. These modified MAP fertiliser compounds include more citrate-soluble N and phosphorus (P) than standard MAP fertiliser. The fertiliser compounds were added to a P-responsive soil and barley plants were grown for 5 weeks to investigate shoot yield responses to nutrient availability of the modified MAP fertiliser compounds. Reverse dilution tracing techniques were also used to compare the fertiliser solubility and P availability of the modified MAP fertiliser compounds with regular MAP fertiliser. Barley (Hordeum vulgare) plants recovered P equally and efficiently over 5 weeks of growth in the P-responsive soil, suggesting that the fertiliser compounds were sufficiently soluble to meet plant requirements and that phosphate release was not slowed by the formation of schertelite. However, shoot yields were generally lower when the barley plants were grown with the modified MAP fertiliser compounds compared to standard MAP fertiliser. This reduced growth was likely due to decreased N availability through a slower release of N, thus further research is warranted to determine the potential for these products to improve N use efficiency.
Keywords: 32P-radioisotope tracer, barley (Hordeum vulgare), fertiliser recovery, magnesium silicate, nutrient acquisition, schertelite, silicon.
Introduction
Nutrient use efficiency is an important consideration in high-input agricultural systems. For example, nearly 100 years of research has aimed to slow the release of nitrogen (N) from fertiliser sources using a variety of novel methods ranging from the addition of compounds to slow enzymatic release to direct polymer coatings (Timilsena et al. 2015). Most research has focused on N due to the many loss pathways of this nutrient (Lassaletta et al. 2014). However, slowing N release to match plant demands is challenging because of the relatively high solubility of nitrate and ammonium salts. A chance observation when examining an X-ray diffraction spectra of interactions of magnesium silicate salts with ammonium compounds revealed the formation of schertelite, a lower solubility magnesium ammonium phosphate salt usually associated with biogenic formation (McConnell 1979). Schertelite formation may potentially slow the release of both N and phosphate to soil solution. Slower release of these nutrients would be beneficial for improving nutrient use efficiency, particularly during crop establishment when plant roots are still developing and cannot maximise the acquisition of nutrients that are readily available.
The objective of this experiment was to investigate the availability of N and phosphorus (P) from several modified mono-ammonium phosphate (MAP) fertiliser compounds that included a magnesium silicate additive. It was expected that the release of these nutrients from the modified MAP fertiliser compounds would be slower than from regular MAP fertiliser. The fertiliser compounds described in this paper include less water soluble and more citrate-soluble N and P than standard MAP fertiliser.
Materials and methods
Plant growth conditions
A P-responsive clay soil (Red Ferrosol; Isbell 1996) was collected from a field in Kingaroy, Qld, Australia. The soil had a Colwell extractable P concentration of 14 mg P kg−1 (as measured by the method of Colwell (1963)), a Phosphorus Buffering Index (PBI) of 385 (as measured by the modified method of Burkitt et al. (2008)), and a pH (1:5 w/v; 0.01 M CaCl2) of ~5.0. The soil was sieved (<5 mm) before 1.1 kg (oven-dry equivalent) of the unamended soil was weighed into plastic bags. A 1 mg P L−1 solution labelled with 32P-radioisotope tracer was added to the soil before the soil in the bags was mixed and incubated for 2 weeks. Reverse dilution tracing techniques were used to compare the fertiliser solubility and P availability of the fertiliser compounds. After 2 weeks, cylindrical PVC pots (87 mm internal diameter, 200 mm height) were filled with a subsoil layer of ~0.9 kg labelled soil. Basal nutrients were then added to the soil as a solution at rates equivalent to 20 kg N ha−1 (as NH4NO3), 15 kg K ha−1 and 7 kg S ha−1 (as K2SO4). Several modified mono-ammonium phosphate (MAP; NH4H2PO4) fertiliser compounds were prepared by adding magnesium-silicate (SIL) to regular MAP fertiliser and reconstituting the MAP granules (1.0–1.4 mm diameter). The compounds included regular MAP (10% N, 22% P), MAP + SIL #1 (9.5% N, 12.5% P), MAP + SIL #2 (8.2% N, 12.1% P), and MAP + SIL #3 (6.5% N, 12.4% P). The percentages of N and P were adjusted with small amounts of urea in MAP + SIL #1, ammonium sulfate in MAP + SIL #2, and ammonium sulfate and triple superphosphate in MAP + SIL #3. These compounds were used to prepare 10 fertiliser treatments:
nil MAP/MAP + SIL
MAP at 28 kg ha−1
MAP + SIL #1 at 49 kg ha−1
MAP + SIL #2 at 51 kg ha−1
MAP + SIL #3 at 50 kg ha−1
MAP at 56 kg ha−1
MAP + SIL #1 at 98 kg ha−1
MAP + SIL #2 at 102 kg ha−1
MAP + SIL #3 at 100 kg ha−1
MAP at 100 kg ha−1
These application rates are below industry standard but were expected to be adequate for the controlled-environment experiment and allow an N response curve to be fitted to the shoot yield data. After the basal nutrients and fertiliser treatments had been added, a further ~0.2 kg of labelled soil was added to the pots. The basal nutrient and fertiliser treatment application rates were based on the surface area of the pots and a row spacing of 0.2 m.
Barley (Hordeum vulgare cv. Gardiner) was established in the pots by sowing seed on the soil surface to achieve a population of four plants per pot. This population is higher than industry standard but was selected so that plants would draw down on available nutrients within the relatively small pots. A 10-mm layer of sand was placed above the seeds, to reduce both evaporation and radioactivity from the surface of the pots. Three replicates of each treatment were prepared. The pots were placed in a glasshouse (natural daylight, ~1800 μmol m−2 s−1 peak intensity; 23/15°C, day/night) in Armidale, NSW, Australia. Pots were arranged in a randomised complete block design (blocks comprised the different replicates) which was generated using DiGGer (Coombes 2006). Plants were grown between September and November 2021. Soil moisture was maintained between 80 and 100% field capacity by watering daily.
Harvest and analysis
Plants were harvested after 5 weeks’ growth. Shoots were cut at the soil surface, oven dried at 70°C for 72 h and weighed. Shoot samples were finely cut before a ~0.5-g subsample was pre-digested in a glass tube with 1 mL deionised water and 4 mL 70% (v/v) nitric acid for at least 16 h. Samples were then digested using a Milestone UltraWAVE 640 (Milestone Srl, Sorisole, Italy). The P concentration of the digested samples was determined using an Inductively Coupled Plasma-Optical Emission Spectrometer (ICP-OES) (Agilent, Mulgrave, Australia). Shoot P content was calculated by multiplying shoot P concentration and shoot dry mass. Digested shoot samples were also analysed for 32P-radioisotope activity using a PerkinElmer Tri-Carb 2810TR (PerkinElmer, Waltham, USA). A scintillation cocktail was prepared by mixing 3 mL of sample with 17 mL of scintillant (PerkinElmer UltimaGold AB). All samples were analysed by liquid scintillation counting (LSC) for 5 min and were corrected for radioactive decay and dilution. The 32P-radioisotope counts were used to calculate the specific activity of the shoot material, the amount of plant P derived from applied P, and the recovery of applied P as follows:
Shoot N concentration was also determined, by analysing 3 mg of the ground shoot samples using isotope ratio mass spectrometry (IRMS). Shoot N content was calculated by multiplying shoot N concentration and shoot dry mass.
Measured parameters were analysed in R ver. 4.0.2 (R Core Team 2020) by fitting linear models and using an analysis of variance with ‘treatment’ as the predictor variable. Means and standard errors were calculated from the fitted linear models (R package: emmeans) (Lenth 2020) and means were compared using least significant difference (l.s.d.). Shoot yield responses to N added by the application of MAP fertiliser were determined by fitting a self-starting logistic function (y = a/(1 + exp ((b – x)/c)), where x is the N added and y is the shoot dry mass) as described by Crawley (2013). Normal quantile-quantile plots and Shapiro–Wilkes tests were used to test the normality of the residuals for all fitted models. When required, these models were log-transformed to meet the assumptions of normality. A 5% level of significance was applied for all statistical tests.
Results and discussion
Shoot dry mass increased in response to fertiliser application (P < 0.001; Fig. 1). The shoot yields of the three MAP + SIL fertiliser compounds were similar when compared at each application rate (i.e. ~50 kg ha−1 and ~100 kg ha−1). Shoot P concentrations also increased in response to fertiliser application (P < 0.001; data not shown), although these concentrations were relatively low across the different treatments (0.08–0.16%). Shoot N concentrations were equivalent across the fertilised treatments (avg. 1.2%) but lower than the control (2.8%) that had received a small, basal application of N (P < 0.001; data not shown).
Shoot dry mass of Hordeum vulgare cv. Gardiner after 5 weeks’ growth in response to 10 fertiliser treatments. The treatments included multiple rates of a range of regular and modified (+ magnesium silicate) mono-ammonium phosphate fertiliser compounds (MAP and MAP + SIL, respectively). Values are mean ± s.e. (n = 3). Bar, l.s.d. (P = 0.05).
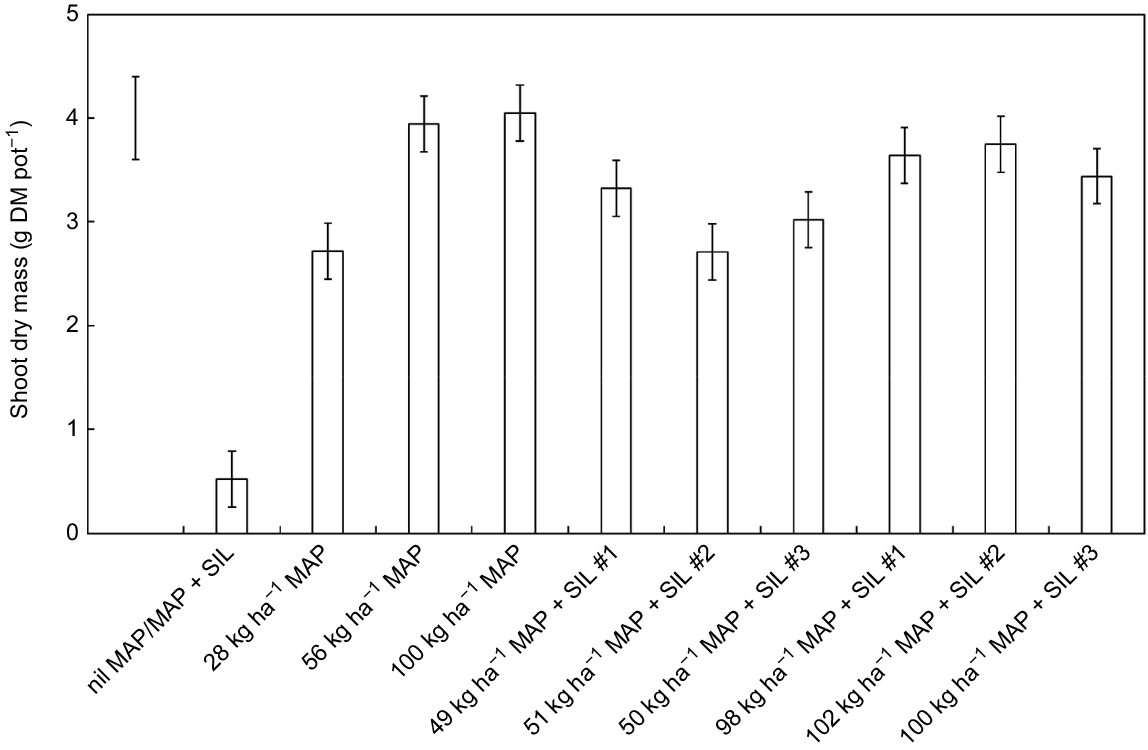
The proportion of P derived from fertiliser (Pdff) generally increased in response to the higher fertiliser application rates (P < 0.001; Fig. 2a). The relatively high Pdff values, even at low fertiliser application rates, indicate that the plants were highly P-stressed after growing for 5 weeks in the P-responsive soil. This is consistent with the low shoot P concentrations. Fertiliser P recovery was not significantly different among the fertiliser treatments (P = 0.670) (Fig. 2b). However, the fertiliser recoveries of 17–23% are relatively high considering the short period of growth (McBeath et al. 2012). These results suggest that the fertiliser compounds (including both the regular and modified MAP fertilisers) were sufficiently soluble and phosphate release was not slowed by the formation of schertelite.
Shoot phosphorus derived from fertiliser (a) and fertiliser phosphorus recovery (b) by Hordeum vulgare cv. Gardiner after 5 weeks’ growth in response to ten fertiliser treatments. The treatments included multiple rates of a range of regular and modified (+ magnesium silicate) mono-ammonium phosphate fertiliser compounds (MAP and MAP + SIL, respectively). Values are mean ± s.e. (n = 3). Bar, l.s.d. (P = 0.05).
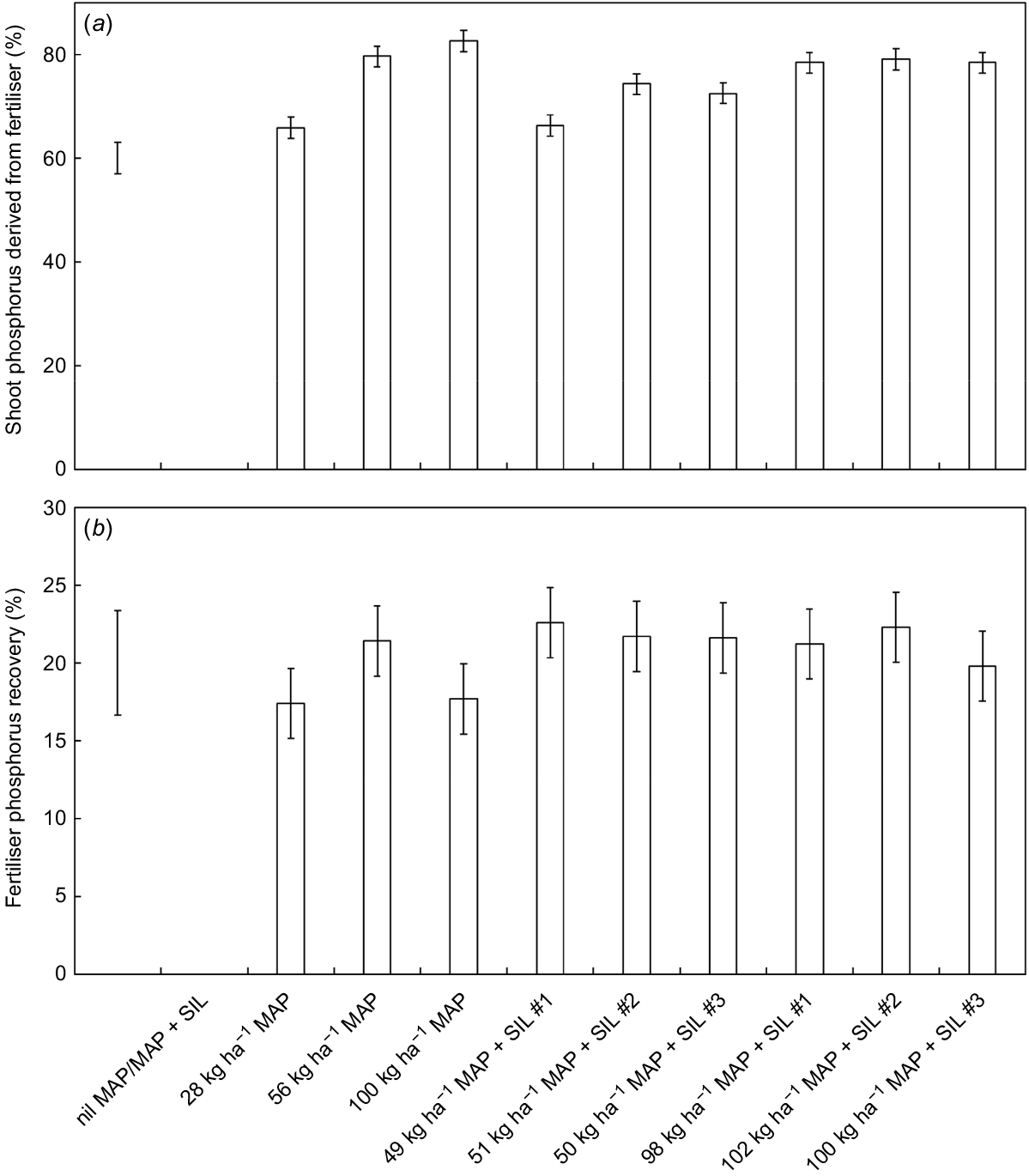
When plotted against the amount of N added (including N from both the basal application and the MAP/MAP + SIL treatments), shoot dry mass and shoot N content clearly increased in response to the addition of N across the four regular MAP fertiliser treatments (dashed lines, Fig. 3). The shoot yields of the MAP + SIL fertiliser compounds were either equivalent to, or lower than that of, the regular MAP fertiliser (Fig. 3a). Similarly, the shoot N contents of the MAP + SIL fertiliser compounds were lower than that of the regular MAP fertiliser (Fig. 3b). These results suggest that the MAP + SIL fertiliser compounds likely released N more slowly than the regular MAP fertiliser, although the addition of magnesium silicate could have also reduced N availability more permanently.
The relationship between the amount of nitrogen added and either the shoot dry mass (a) or shoot nitrogen (N) content (b) of Hordeum vulgare cv. Gardiner across ten fertiliser treatments. The treatments included multiple rates of a range of regular and modified (+ magnesium silicate) mono-ammonium phosphate fertiliser compounds (MAP and MAP + SIL, respectively). Values are the mean (n = 3) of each treatment. A self-starting logistic model (dashed line) was used to describe the shoot yield and shoot N content responses to increasing N across the four regular MAP treatments.
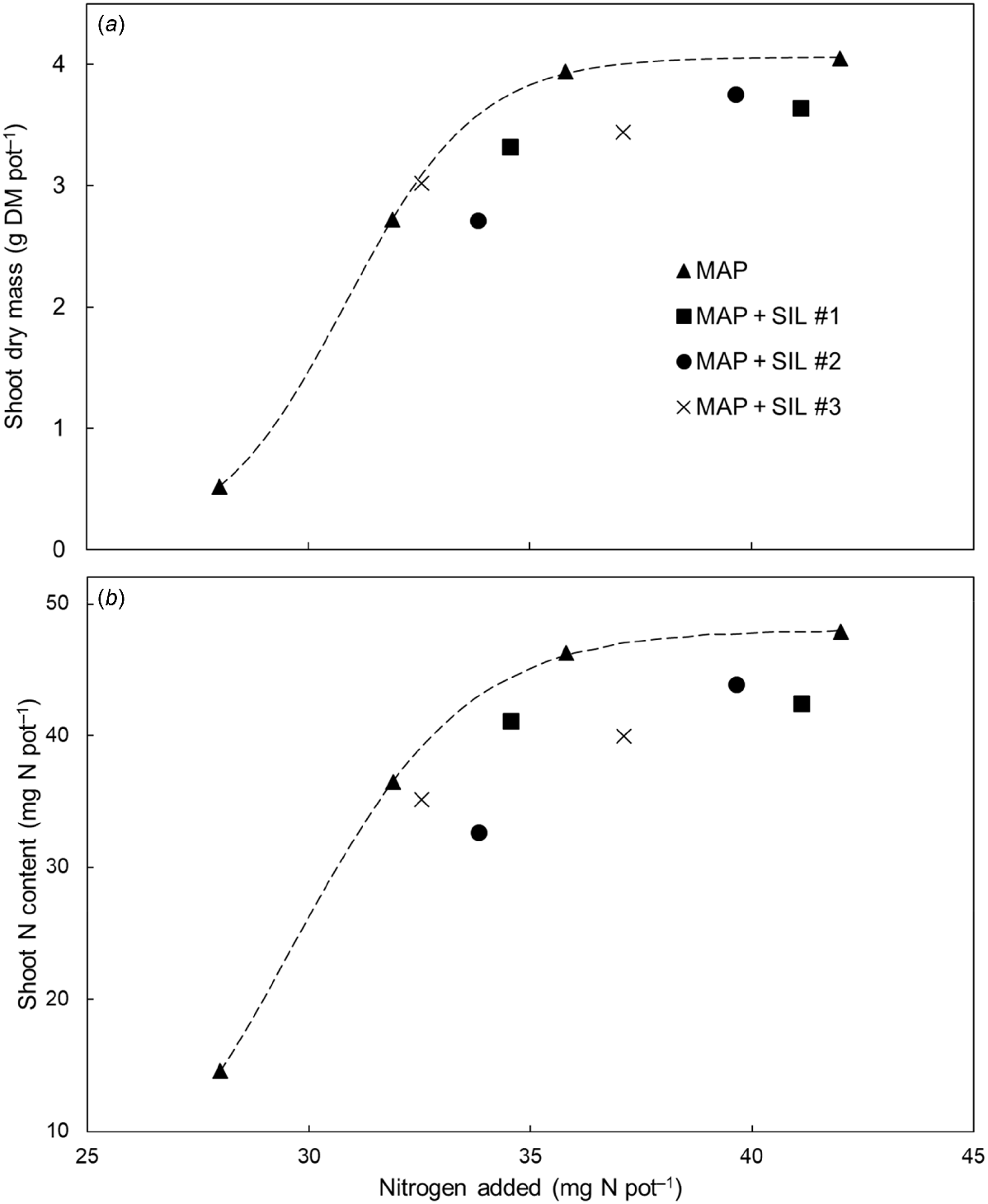
The combined results indicate that the different fertiliser compounds were sufficiently soluble, yet only the availability of N was reduced by the magnesium silicate additive. Since the total N requirements of these plants were substantially higher than that of the total P requirements, it is possible that addition of magnesium silicate decreased product availability in the short term but that this was observed as N constraint since plants had a higher requirement for N than P. In this sense it is also possible that the primary source of the nutrition was the unreacted MAP content rather than directly from schertelite. Irrespective, these fertiliser compounds may be beneficial at crop establishment during which root development is encouraged by available P while potential losses of N are reduced. As these modified MAP fertiliser compounds include more citrate-soluble N and P than standard MAP fertiliser, further research is warranted to understand the potential benefit of modifying MAP fertiliser for slowing the release of N and improving N use efficiency.
Data availability
The data presented in this study are available on request from the corresponding author.
References
Burkitt LL, Sale PWG, Gourley CJP (2008) Soil phosphorus buffering measures should not be adjusted for current phosphorus fertility. Australian Journal of Soil Research 46(8), 676-685.
| Crossref | Google Scholar |
Colwell JD (1963) The estimation of the phosphorus fertilizer requirements of wheat in southern New South Wales by soil analysis. Australian Journal of Experimental Agriculture 3(10), 190-197.
| Crossref | Google Scholar |
Coombes NE (2006) DiGGer, a design generator. Available at http://nswdpibiom.org/austatgen/software/
Lassaletta L, Billen G, Grizzetti B, Anglade J, Garnier J (2014) 50 year trends in nitrogen use efficiency of world cropping systems: the relationship between yield and nitrogen input to cropland. Environmental Research Letters 9(10), 105011.
| Crossref | Google Scholar |
Lenth R (2020) emmeans: estimated marginal means, aka least-squares means. R package version 1.5.0. Available at https://CRAN.R-project.org/package=emmeans
McBeath TM, McLaughlin MJ, Kirby JK, Armstrong RD (2012) The effect of soil water status on fertiliser, topsoil and subsoil phosphorus utilisation by wheat. Plant and Soil 358(1–2), 337-348.
| Crossref | Google Scholar |
Timilsena YP, Adhikari R, Casey P, Muster T, Gill H, Adhikari B (2015) Enhanced efficiency fertilisers: a review of formulation and nutrient release patterns. Journal of the Science of Food and Agriculture 95(6), 1131-1142.
| Crossref | Google Scholar | PubMed |