Sheep rotational grazing strategy to improve soil organic carbon and reduce carbon dioxide emission from spring wheat in an arid region
Irshad Ahmad

A
Abstract
Cultivating forage crops is crucial to improve feed production, and grazing is an important utilisation method to improve soil fertility.
Improving soil organic carbon (SOC) content and reducing carbon dioxide (CO2) emission through grazing management from a spring wheat field.
We compared sheep rotational grazing and control, and studied their effects on SOC and CO2 emission from a spring wheat field.
Sheep rotational grazing improved SOC content (by 23.5%) and soil easily oxidised organic carbon (EOC) content (by 7.7%) and reduces soil microbial biomass carbon (MBC) content (by 35.8%) compared with the control. Sheep rotational grazing reduced CO2 emission compared with the control. Sheep grazing reduced cumulative CO2 emission by 28.9% and 33.0% in May and June compared with the control.
Sheep grazing improved SOC content and reduce CO2 emission from a spring wheat field.
Based on our short-term study, sheep rotational grazing has a significant effect on SOC, EOC and MBC contents and CO2 emission from spring wheat fields in arid regions. For a large-scale assessment of sheep grazing on soil fertility and CO2 emission, more investigation for different soils and climates is necessary. Furthermore, a long-term study is also necessary to better understand the effect of sheep rotational grazing on soil fertility and CO2 emission from spring wheat fields in arid regions.
Keywords: arid regions, carbon dioxide emission, microbial biomass carbon, sheep grazing, soil organic carbon, soil fertility, wheat.
Introduction
Natural grasslands cover an area of about 25% of earth terrestrial ecosystems and provide forages for ruminants, carbon sequestration, water conservation, sand-fixing function and other important ecosystem services (Hopkins and Wilkins 2006; Chen et al. 2017; Hu et al. 2019; Zhao et al. 2020). However, natural grasslands are degrading due to over grazing, climate change and poor management and it is challenging to provide quality feed for livestock production (Wang et al. 2019a; Huang et al. 2021). China has 400 million ha of grassland (Sui and Zhou 2013; Li 2017) and a survey by the Ministry of Environmental Protection, China, suggested that about 31.8% of the grasslands are in a degraded state (Li et al. 2018). Overgrazing due to high stocking rates and static grazing management is an important cause of grassland degradation (Akiyama and Kawamura 2007). Feed shortage in China is increasing due to rapid development of livestock husbandry and therefore it is crucial to improve feed production (Li et al. 2015; Kamran et al. 2022). Cultivating forage crops, especially wheat, is important to cope with feed shortage and improve livestock production and food security (Zeleke 2019; Yang et al. 2022). Cultivating forage crops can not only provide high-quality feed for livestock but also increase soil nutrient storage and improve soil fertility and productivity (Dong and Shen 2000; Sun et al. 2019; Ghani et al. 2022). In China, the total grassland area accounts for 13% of the global grassland, and cultivated grassland represents 1.9% of total grassland (Sui and Zhou 2013; Li 2017). Considering the feed shortage it is important to cultivate forage crops for livestock production in arid regions.
Grazing is an effective strategy to regulate natural grasslands and sown pastures in which animals feed on forage, trampling the soil and returning their excrement to pastures (Wang et al. 2019b; Wei et al. 2021). Grazing maintains healthy development of the ecosystem (Hou et al. 2016). Integrated crop and livestock systems reduce fertiliser usage, improve forage yield and enhance nutrient circulation in the ecosystem (Hatfield et al. 2007; Ferreira et al. 2015). Grazing regulates the interspecific competition between crops and weeds, which hinders the growth and reproduction of weeds in the field, thereby reducing the damage to vegetation by pests and diseases, reducing the use of pesticides and protecting the environment in a healthy manner (Carr et al. 2005). Grazing significantly improved water use efficiency, light use efficiency, carbon and nitrogen content in alpine grasslands (Shen et al. 2019). Livestock feeding on the above-ground parts of vegetation can increase the branching of forage and increase the growth rate, thereby stimulating compensatory growth of forage and increasing the yield (Che et al. 2019). Grazing affects physiological and morphological characteristics of plants as well as soil physico-chemical properties (Zheng et al. 2011; Yang et al. 2019). Therefore, sheep grazing is an effective method to improve soil fertility and forage productivity.
Global climatic change and human activities affect the carbon and nitrogen cycles of terrestrial ecosystems (Yan et al. 2014). The global mean temperature will rise 2–7°C by the end of the century due to increasing greenhouse gas emissions (Allison et al. 2009). China will have a CO2 emission peak before 2030 and China plans to achieve carbon neutrality before 2060 (Xi 2020). According to IPCC (2013), minute changes in the soil organic carbon (SOC) pool affect carbon dioxide (CO2) emission. Sequestration of SOC plays a crucial role in mitigating anthropogenic increase in atmospheric concentration of CO2 (Chen et al. 2018). The SOC is part of the carbon cycle and enhancing SOC stock through sustainable soil carbon sequestration practices can help mitigate climate change (Amelung et al. 2020). The CO2 emission from soil depends on soil respiration and the combination of roots and heterotrophic respiration, and therefore changes in roots and soil microorganisms affect CO2 emission (Hanson et al. 2000). Research suggests that grazing lands are a major sink of carbon; however, improper use of grazing land degrades soil carbon storage capacity and soil health and appropriate grazing results in higher SOC content and improves hydrological function and the soil ecosystem (Kim et al. 2023). Grazing plays a crucial role in the carbon cycle of ecosystems (Zhao et al. 2017; Chen and Frank 2020). Grazing affects the SOC storage by three mechanisms: defoliation, excrement return and trampling (Mikola et al. 2009; Liu et al. 2015). Livestock trampling effects seed germination, seed dispersal, plant cover, plant composition, nutrient cycling and soil physical conditions (Drewry et al. 2008; Horn et al. 2013; Schrama et al. 2013; Ludvíková et al. 2014; Heggenes et al. 2017). Previous research suggested that livestock trampling could increase SOC storage (Liu et al. 2015). The influence of grazing on the soluble SOC content is closely related with the growth season and soil depth (Luo et al. 2009). Grazing can increase the distribution of photosynthetic products in the roots of alpine meadow vegetation and promote the input of photosynthetic products into the soil thereby increasing carbon storage (Hafner et al. 2012). In the grasslands of southern Australia, compared with continuous grazing, long-term grazing rotation increased SOC storage by increasing the vegetation productivity and root turnover rate (Sanderman et al. 2015). Additionally, studies in alpine meadows have proved that grazing can increase carbon storage by stimulating the net ecosystem exchange, and grazing can also reduce CO2 emission by reducing the sensitivity of soil respiration to climate factors (Chen et al. 2015). Thus, sheep rotational grazing is an important utilisation method to improve SOC storage and reduce CO2 emission.
Earlier experiments indicated a limited effect of sheep rotational grazing on SOC content, soil microbial biomass carbon (MBC) content, soil easily oxidised organic carbon (EOC) content and CO2 emission. The objectives of our experiment were to determine the effect of sheep rotational grazing and control treatment on SOC content and CO2 emission in arid regions. The experimental results provide important insights regarding reducing CO2 emission from wheat fields and improvement of soil fertility using sheep rotational grazing.
Materials and methods
Experimental site, experimental design and field management
A field experiment was conducted at the Linze Grassland Agricultural Experimental Station of Lanzhou University, China (100°02′E, 39°15′N; 1390 a.s.l.) in the 2018 growth season. The experimental station is located in the core area of the Hexi corridor Hei-he Oasis in the inland arid area of northwest China, with the Qilian Mountains in the south and the Alxa Desert in the north. The experimental area is a salinised meadow area. The soil of the experimental site was classified as Aquisalids according to United States Department of Agriculture soil taxonomy (Ning et al. 2020). Soil pH of the experiment site was 8.5 and salt contents were 0.6–0.9% (Ghani et al. 2022). The soil electrical conductivity was 4.2 dS m−1. The experimental site is an arid area having deficit and erratic precipitation (Fig. 1). The annual precipitation of the site was 118.4 mm. The precipitation is concentrated in May–September and accounts for more than 70% of total annual precipitation. Evaporation of the experimental site was 1830.4 mm. The annual average temperature is 7.7°C, annual sunshine hours are 3042 and the frost-free period is about 170 days. Monthly precipitation and monthly average temperature during the growth season were measured using an automatic weather station (Fig. 1). The main agricultural systems in the study area are specialised intensive crop production and extensive integrated crop–livestock production (Hou et al. 2008). The nutrient status of the top 0–20 cm soil layer of the site comprised 10.2 g kg−1 SOC, 4.8 g kg−1 EOC, 208.3 mg kg−1 MBC, 0.80 g kg−1 total soil nitrogen, 0.85 g kg−1 soil total phosphorus and 0.50 g kg−1 soil total potassium.
Mean temperature (°C) and precipitation (mm) for January–December 2018 at the experimental site.
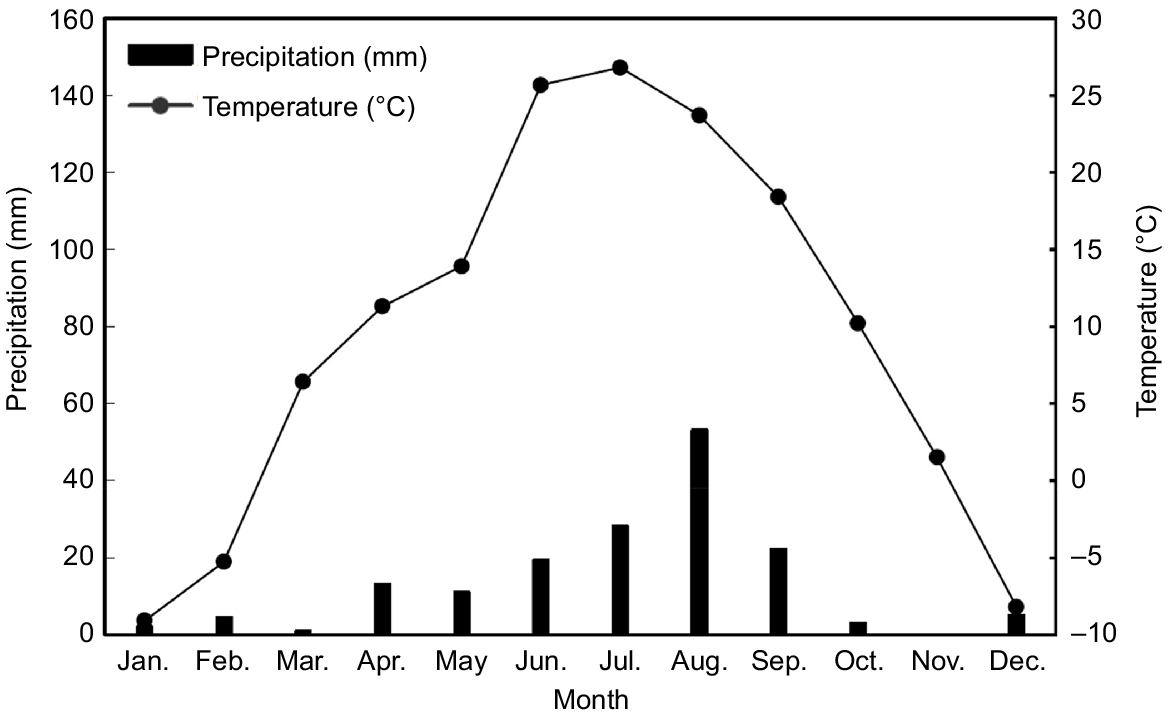
The field experiment had a randomized complete block design with three replications. There were two treatments: sheep grazing (RG) and control (CK). Spring wheat (Yong-Liang No. 15), which is commonly grown for forage production in arid regions, was sown in March 2018 at a seed rate of 300 kg ha−1. Each single plot had an area of 1 ha. The inter row spacing was 10 cm. Nitrogen (urea, 46% N) was applied at the time of sowing at a rate of 225 kg N ha−1 (Guo et al. 2023) and phosphorus (calcium superphosphate, 16% P2O5) at 75 kg ha−1. These are the recommended rates of nitrogen and phosphorus for wheat in this area. Sheep grazing was carried out at a stocking rate of 80 adult sheep ha−1 at stem elongation stage (BBCH-30). Sheep grazing was carried out at two times. The grazing time in the wheat field was from May (1–5 May) to June (1–5 June). Each grazing time was 5 days, and the grazing time interval was about 25 days. In the middle of each plot we constructed a wire fence to isolate a 10 m × 10 m plot as CK. The field was irrigated (120 mm) once in April.
Sampling and measurements
Dry matter yield (g m−2) was measured in May (20 days after first sheep grazing), June (20 days after second sheep grazing) and July (40 days after second sheep grazing) in RG and CK treatments in 2018. At three different locations in each replication, a 1 m2 area was cut at ground level. The samples were oven dried at 75°C to a constant weight. An electronic balance was used to measure the dry matter yield.
The SOC, MBC and EOC contents were measured in May (20 days after first sheep grazing), June (20 days after second grazing) and in July (40 days after second grazing) during the 2018 growth season. Soil samples from 0–10, 10–20 and 20–30 cm soil depths at three different locations in each replication were taken with an auger. The SOC content was determined using the potassium dichromate sulfuric acid oxidation method (Wang et al. 2014a); MBC content was assayed as described by Zeng et al. (2015); and EOC content was assayed by potassium permanganate oxidation method (Wang et al. 2014b).
The CO2 emission was measured during May and June. The measurements were carried out during 6–10 May, 11–20 May, 21–30 May, 6–10 June, 11– 20 June and 21–June from 0 to 24 h. The 8150 Multiplexer Soil Carbon Flux Automatic Monitoring System 8100-104 (LI-COR USA), a long-term CO2 emission measurement system, was used to monitor the CO2 emission fluxes. Taking 0–24 h as a measurement cycle, the air chamber was automatically closed once every 30 min, and the closing time was 1.5 min. The system automatically recorded the changes in CO2 fluxes and we used the Li-8100-201 temperature sensor and Li-8100-204 ML 2x soil moisture sensor to monitor the 5 cm soil-depth temperature and moisture. One day before each measurement, in order to avoid the impact of installation of the air chamber on soil respiration, a polyvinyl chloride respiration measurement ring was inserted in the soil in advance. The inner diameter and height of the ring were 10 and 12 cm, respectively. The height of the measuring ring exposed to the ground was adjusted by artificially adjusting the height of the air chamber, which was generally about 5–8 cm.
Statistical analysis
Analysis of variance was determined with SPSS 19.0 software (SPSS, Inc., Armonk, USA). The data obtained from each sampling event were analysed separately. Mean comparison was carried out with Tukey’s test at P ≤ 0.05. Pearson’s correlations were calculated to determine the relationship of CO2 fluxes with soil temperature and soil moisture.
Results
Dry matter yield
Dry matter yield of spring wheat significantly differed for the RG and CK treatments (Fig. 2). The dry matter yield in RG and CK treatments increased from May (20 days after first sheep grazing) to July (40 days after second grazing), and maximum dry matter yield was in July (40 days after second grazing). The dry matter yield was higher in the CK than the RG treatment. The RG treatment decreased dry matter yield in May (20 days after first grazing) by 49.4 g m−2, in June (20 days after second grazing) by 79.7 g m−2 and in July (40 days after second grazing) by 91.4 g m−2 compared with the CK treatment.
SOC and its components
Sheep grazing significantly improved SOC content compared with the CK treatment (Fig. 3). The SOC content was high at 0–10 cm soil depth and then reduced with greater soil depth. The SOC content increased from May (20 days after first grazing), reached to its maximum in June (20 days after second grazing) and then decreased in July (40 days after second grazing). Means based on three stages’ data showed that sheep grazing significantly increased SOC content at 0–10 cm soil depth by 23.5%, at 10–20 cm depth by 21.4% and at 20–30 cm depth by 25.6% compared with CK treatment.
Effects of sheep rotational grazing (RG) and control treatment (CK) on the soil organic carbon content at 0–10, 10–20 and 20–30 cm soil depths of spring wheat at 20 days after first sheep grazing and 20 and 40 days after second sheep grazing. Vertical bars represent means ± s.d. (n = 3). Analyses were done separately for different grazing intervals and different soil depths.
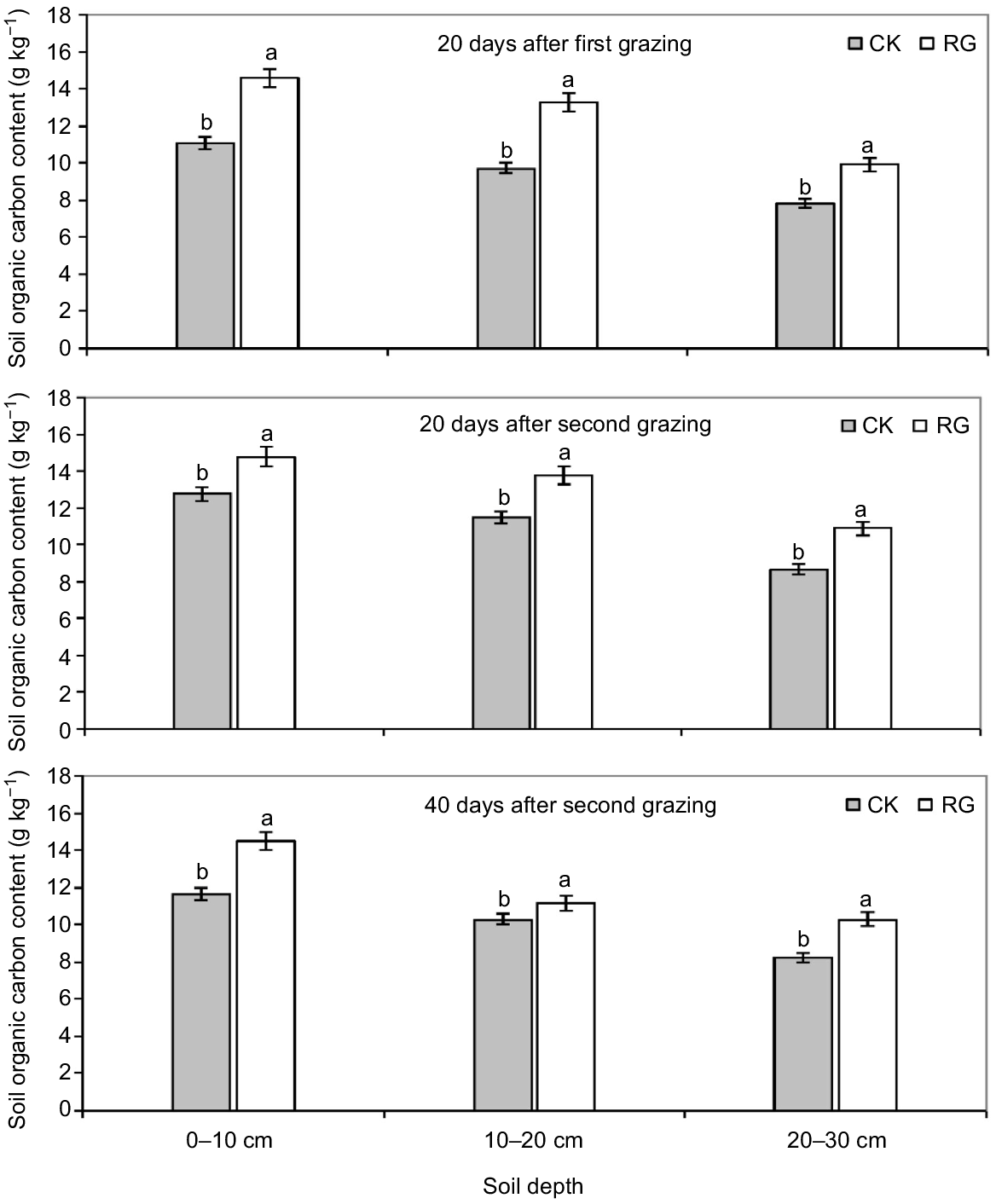
Sheep grazing significantly reduced the MBC content compared with CK treatment (Fig. 4). The MBC content was high at 0–10 cm soil depth. The MBC content was higher in May (20 days after first grazing), decreased in June (20 days after second grazing) and then increased in July (40 days after second grazing). Means based on May, June and July data showed that RG significantly reduced MBC content at 0–10 cm soil depth by 35.9%, at 10–20 cm by 33.0% and at 20–30 cm by 38.5% compared with CK treatment.
Effects of sheep rotational grazing (RG) and control treatment (CK) on the soil microbial biomass carbon content at 0–10, 10–20 and 20–30 cm soil depth of spring wheat at 20 days after first sheep grazing and 20 and 40 days after second sheep grazing. Vertical bars represent means ± s.d. (n = 3). Analyses were done separately for different grazing intervals and different soil depths.
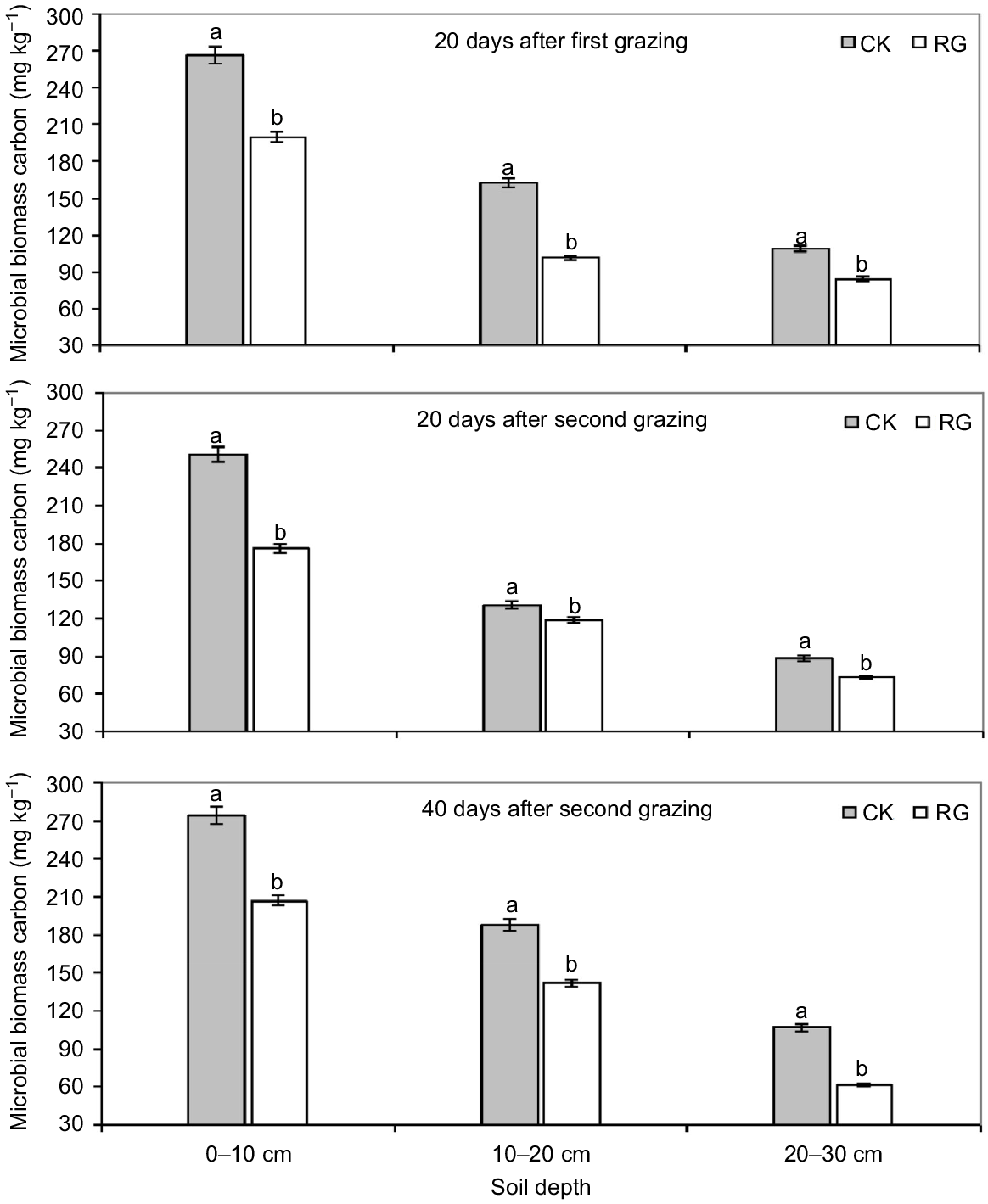
The EOC content was higher in RG compared with CK treatment (Fig. 5). The EOC content was higher in the 0–10 cm soil layer, followed by 10–20 cm and then 20–30 cm. Means based on May, June and July data showed that RG increased EOC content at 0–10 cm soil layer by 4.9%, at 10–20 cm by 13.6% and at 20–30 cm by 4.5% compared with CK treatment.
Effects of sheep rotational grazing (RG) and control treatment (CK) on the soil easily oxidised organic carbon content at 0–10, 10–20 and 20–30 cm soil depth of spring wheat at 20 days after first sheep grazing and 20 and 40 days after second sheep grazing. Vertical bars represent means ± s.d. (n = 3). Analyses were done separately for different grazing intervals and different soil depths.
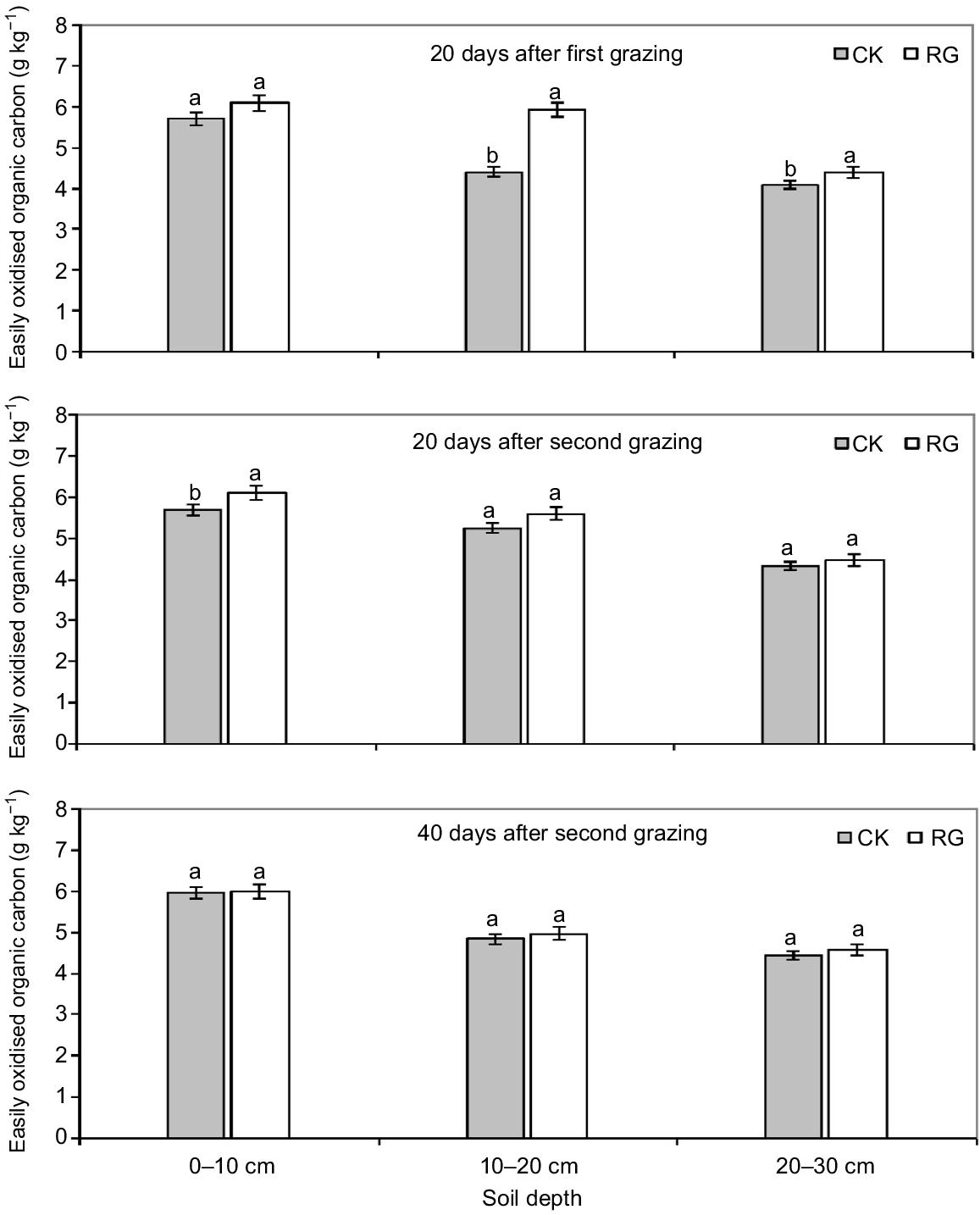
Sheep grazing significantly reduced CO2 emission from the spring wheat field compared with CK treatment (Fig. 6). The CO2 emission fluxes peaks all appeared around 2:00 PM. The daily dynamics of CO2 emission in RG and CK treatments showed a trend of simultaneous change. The average CO2 emission in RG treatment was 4.9 (6–10 May), 5.7 (11–20 May) and 6.5 (21–30 May) μmol m−2 s−1 and correspondingly in CK was 6.4, 7.4 and 8.1 μmol m−2 s−1. The average CO2 emission in RG treatment was 5.6 (6–10 June), 5.2 (11–20 June) and 5.1 (21–30 June) μmol m−2 s−1 and correspondingly in CK was 7.0, 8.0 and 6.9 μmol m−2 s−1.
Effects of sheep rotational grazing (RG) and control treatment (CK) on carbon dioxide emission from a spring wheat field for 6–10 May (May, a), 11–20 May (May, b), 21–30 May (May, c), 6–10 June (June, a), 11–20 June (June, b) and 21–30 June (June, c) from 0 to 24 h. Vertical bars represent means ± s.d. (n = 3). Data of May (a) and June (a) are means of 5 days whereas data of May (b), June (b), May (c) and June (c) are means of 10 days.
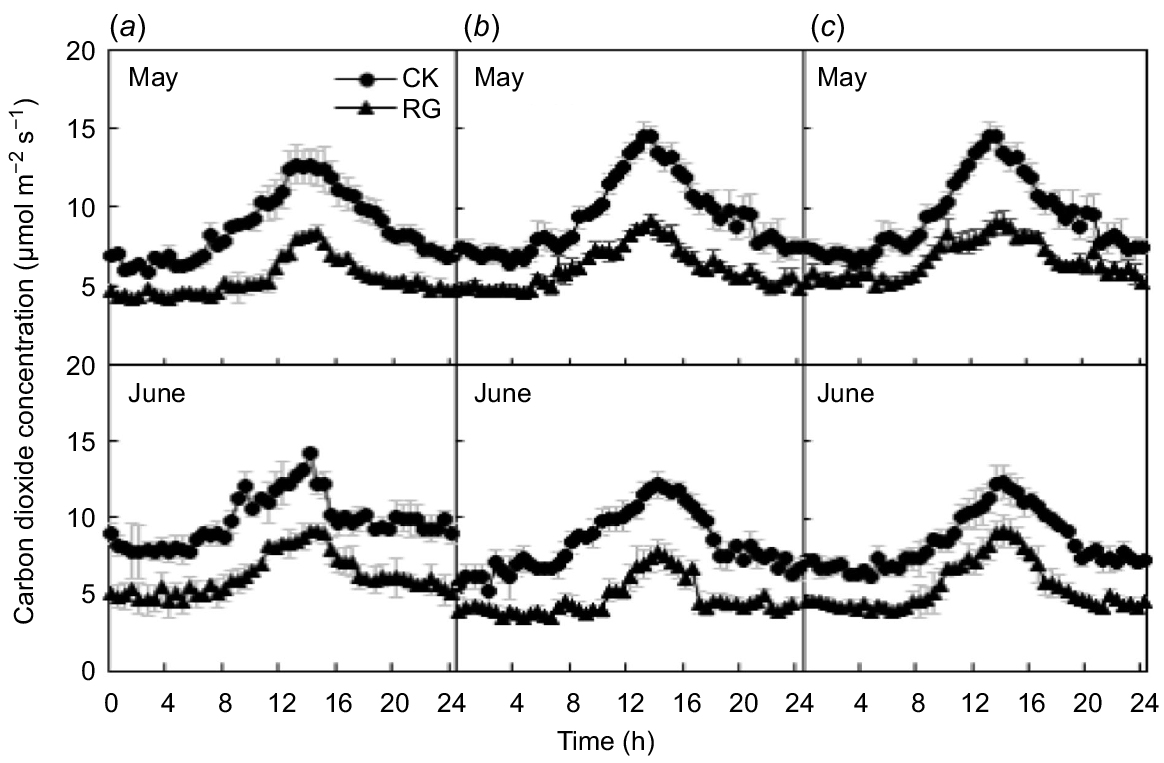
The RG and CK treatments showed significantly different cumulative CO2 emission from the spring wheat field (Fig. 7). The CK treatment had higher cumulative CO2 emission in May and June compared with RG treatment. The cumulative CO2 emission in May and June in CK treatment was 228.1 and 217.9 g CO2 m−2 month−1, respectively, and correspondingly in RG treatment was 177.0 and 163.7 g CO2 m−2 month−1. Sheep grazing reduced the cumulative CO2 emission in May by 28.9% and in June by 33.0% compared with CK treatment.
During the spring wheat growth season, the CO2 fluxes showed an exponential increase with increases in soil temperature and moisture (Fig. 8). Soil temperature and moisture were significantly positively correlated with CO2 fluxes. In May and June, during the monitoring period of the two treatments of wheat field, the R2 value of CO2 flux against soil temperature was higher than that for soil moisture, but the influence of soil moisture on CO2 flux in May was higher than that of soil temperature (P < 0.001 vs P < 0.05).
Discussion
Effect of sheep rotational grazing on SOC content and its components
Soil is the biggest carbon pool of grassland ecosystem and stores 28–37% of the carbon in the terrestrial ecosystem (Lal 2004a; Tang et al. 2018; Zhang et al. 2018). The increase of storage or sequestration of SOC content is important to improve soil fertility and crop productivity as well as mitigate the CO2 increase in the atmosphere (Lal 2004b; Wiesmeier et al. 2019). The SOC content reflects the long-term stability between accumulation and loss of organic carbon and is affected by human activities, land use, precipitation and microbial metabolism (Piao et al. 2009; Yang et al. 2012). Grazing is an important strategy to utilise cultivated grasslands by improving soil fertility and environmental quality (Šimek and Čuhel 2009; Ehsanul 2016). Grazing significantly influences SOC storage in grasslands (Hao et al. 2024). Long-term grazing rotation can increase SOC storage by increasing vegetation productivity and root turnover rate (Sanderman et al. 2015). In our study, the RG treatment significantly increased SOC content compared with CK. A possible reason is that sheep trampling enhanced the decomposition of litter and converted it into soil organic matter (Ball et al. 2012). An other possible reason is that livestock feeding on forage changes the pattern of vegetation carbon allocation, promoting the transfer of photosynthetic products to the root system and providing a rich substrate for the formation of soil organic matter (Piñeiro et al. 2010; Hafner et al. 2012). A third possible reason is that the sheep excrement return during grazing improved the SOC content. Research suggests that grazing increases the SOC content (Han et al. 2014). The MBC is an index indicating any SOC content changes due to land use or management measures, and its content generally accounts for 1–4% of the soil organic matter (Allen et al. 2010). The MBC is a crucial indicator of changes of soil quality as well as management practices and is sensitive to environmental changes (Holt 1997; Wang and Long 2008). Liu et al. (2012) showed that grazing increased SOC, MBC and total nitrogen contents in typical steppe and desert steppe ecosystems in China. However, our results suggested that SOC content was increased and MBC content decreased in RG compared with CK treatment. The possible reason for lower MBC content in sheep grazing treatment is that sheep trampling increases the soil compactness and decreases soil porosity, soil permeability and water-stable aggregates and thus changes the living environment of microorganisms, which in turn disturbs microorganism metabolism and reproduction, reducing their activity and ultimately leading to lower MBC content (Chai et al. 2016; Tong et al. 2018). Xu et al. (2023) showed that the soil microbial biomass and enzyme activities reduced with increases in grazing intensity and duration. In our study, MBC content of the surface soil was higher compared with deeper soil layers, and surface soil contains more microorganisms than deeper soil layers. The SOC content was also higher in the surface soil compared with deeper soil layers, and the soil porosity is high and the oxygen sufficient, which are conducive for survival of microorganisms (Niu et al. 2013). Rui et al. (2011) suggested that Tibetan sheep grazing increased the organic matter of surface soil of alpine meadow but reduced the MBC content at 20–30 cm soil depth. The EOC content under RG treatment was not much higher than for CK. A possible reason is that EOC content is an unstable part of the SOC, and the effect of grazing on EOC content is not obvious; it may also be related to the quality of litter entering the soil (Wen et al. 2016). The higher EOC content in the top 0–10 cm soil depth in RG treatment was attributed to sheep excrement return during grazing.
Effects of sheep rotational grazing on CO2 emission and its relation with soil moisture and temperature
Soil respiration is an important process in the global carbon cycle and plays an important role in altering CO2 levels in the atmosphere (Li et al. 2024). Soil respiration is composed of three parts – root respiration, microorganism respiration and soil animal respiration – with the first two accounting for about 90% of soil respiration (Chen et al. 2011). Grazing animals feeding, trampling and their excreta return directly or indirectly result in greenhouse gas emissions and global warming (IPCC 2007; Treweek et al. 2016). The RG treatment significantly reduced CO2 emission and cumulative CO2 emission compared with CK. Previous study showed that grazing reduced soil respiration rate compared with no-grazing treatment (Jia et al. 2007); furthermore, the mean soil respiration rate in no-grazing and grazing treatments was 247.9 and 108.3 mg CO2 m−2 h−1, respectively. Moreover, the higher soil respiration in no-grazing treatment was attributed to higher biomass and soil moisture content. Cui et al. (2000) reported that grazing reduced CO2 emission in Stipa grandis grasslands. Previous research suggested that summer grazing decreased CO2 concentration in desert and typical temperate steppe ecosystems, whereas it increased in meadow (Shi et al. 2017). Furthermore, N2O emission increased in meadow but was not significantly higher than for desert and typical temperate steppe ecosystems. Our results are consistent with those of Jia et al. (2007), who found that grazing significantly reduced CO2 emission in typical Leymus chinensis steppes in Inner Mongolia. The possible reason for low CO2 emission in sheep grazing treatment is that long-term sheep trampling compacts the soil and reduces its porosity, which in turn reduces soil hydraulic conductivity, and thus the decrease in soil water content reduces respiration rate and CO2 emission (Ball et al. 2012; Chen et al. 2013). Another possible reason for low CO2 emission is that RG treatment had lower vegetation coverage and the soil was more exposed, which accelerated the evaporation and loss of soil water; at the same time the increase in the area of grassland exposed to light makes the change in soil temperature more obvious and the CO2 emission is significantly reduced (Jia et al. 2007). Furthermore, grazing reduces the existing stock on the ground, reduces the photosynthetic rate and supplies more photosynthetic products to the above-ground parts, which reduces root biomass, weakens root respiration and reduces CO2 emission (Gong et al. 2014). Our findings are inconsistent with those of Liu et al. (2016), likely because of differences in the grazing system, forage type and soil moisture status. Chen et al. (2015) suggested that in alpine meadow, grazing can increase carbon storage by stimulating net ecosystem exchange, and also reduce system CO2 emission by reducing the sensitivity of soil respiration to climate factors. In western Canadian grasslands, grazing reduced CO2 emissions from sandy soil (Thomas et al. 2018). Our study showed significantly reduced CO2 emission for RG compared with CK treatment. The CO2 fluxes were affected by soil temperature and moisture. The CO2 fluxes increased exponentially with increased soil temperature and moisture, consistent with results of Zhao et al. (2016). The daily dynamics of CO2 fluxes are related to the daily changes in soil temperature. During the day, solar radiation reaches its maximum at about 2:00 PM and at this time the soil temperature is almost at its peak. The increase in temperature can promote plant root respiration, speed up metabolism of related microorganisms in the soil, stimulate enzyme activity and thus cause more CO2 emission (Huang et al. 2005; Johnston and Sibly 2018; Nissan et al. 2023). Many studies have shown a quadratic, exponential or linear relationship of soil moisture and soil temperature with CO2 fluxes (Zhou et al. 2013; Gong et al. 2014). Our findings indicated that sheep grazing is an important strategy to improve soil fertility and reduce CO2 emission in arid regions.
Conclusion
In this experiment, sheep grazing affected SOC, EOC and MBC contents, CO2 emission and dry matter yield of wheat. Sheep grazing resulted in higher SOC and EOC contents but lower MBC content. The CO2 emission and cumulative CO2 emission were also lower for the sheep grazing treatment. The results are important for improving the SOC, EOC and MBC contents and reducing CO2 emission from spring wheat in arid regions. In our short-term study, sheep rotational grazing had a significant effect on SOC, EOC and MBC contents and CO2 emission from a spring wheat field in an arid region. More investigation using different soils and climates is necessary for a large-scale assessment of effects of sheep grazing on soil fertility and CO2 emission. Furthermore, a long-term study is required to better understand the sheep grazing impact on soil physico-chemical properties, greenhouse gas emissions and soil microbial community structure in arid regions.
Data availability
The datasets used and/or analysed during the current study are available from the corresponding author on reasonable request.
Declaration of funding
This research work was supported by the Program for Innovative Research Team of Ministry of Education (IRT_17R50), the Program of National Science and Technology Assistance (KY202002011), the China Postdoctoral Science Foundation (2021M701521) and Lanzhou City’s Scientific Research Funding Subsidy to Lanzhou University.
References
Akiyama T, Kawamura K (2007) Grassland degradation in China: methods of monitoring, management and restoration. Grassland Science 53(1), 1-17.
| Crossref | Google Scholar |
Allen DE, Pringle MJ, Page KL, Dalal RC (2010) A review of sampling designs for the measurement of soil organic carbon in Australian grazing lands. The Rangeland Journal 32(2), 227-246.
| Crossref | Google Scholar |
Allison I, Bindoff NL, Bindschadler R, Cox P, de Noblet-Ducoudre N, England M, Francis J, Gruber N, Haywood A, Karoly D, Kaser G, Le Quere C, Lenton T, Mann M, McNeil B, Pitman A, Rahmstorf S, Rignot E, Schellnhuber HJ, Schneider A, Sherwood S, Somerville R, Steffen K, Steig E, Visbeck M, Weaver A (2009) ‘The Copenhagen diagnosis 2009 updating the world on the latest climate science.’ (The University of New South Wales Climate Change Research Centre (CCRC): Sydney, Australia)
Amelung W, Bossio D, de Vries W, Kögel-Knabner I, Lehmann J, Amundson R, Bol R, Collins C, Lal R, Leifeld J, Minasny B, Pan G, Paustian K, Rumpel C, Sanderman J, van Groenigen JW, Mooney S, van Wesemael B, Wander M, Chabbi A (2020) Towards a global-scale soil climate mitigation strategy. Nature Communications 11, 5427.
| Crossref | Google Scholar |
Ball BC, Cameron KC, Di HJ, Moore S (2012) Effects of trampling of a wet dairy pasture soil on soil porosity and on mitigation of nitrous oxide emissions by a nitrification inhibitor, dicyandiamide. Soil Use and Management 28(2), 194-201.
| Crossref | Google Scholar |
Carr PM, Poland WW, Tisor LJ (2005) Natural reseeding by forage legumes following wheat in Western North Dakota. Agronomy Journal 97(4), 1270-1277.
| Crossref | Google Scholar |
Chai JL, Xu CL, Zhang JW, Yang HL, Xiao H, Pan TT, Wang Y, Yu XJ (2016) Response of soil physical properties and microbial quantities to simulated trampling on Alpine meadow. Acta Agrestia Sinica 24(6), 1234-1240 [in Chinese with English abstract].
| Google Scholar |
Che ZJ, Li Y, Quan L, Xiang M, Che ZQ (2019) The effect of grazing methods on the characteristics of grassland vegetation. Protection Forest Science and Technology 6, 58-60 [in Chinese with English abstract].
| Google Scholar |
Chen J, Frank DA (2020) Herbivores stimulate respiration from labile and recalcitrant soil carbon pools in grasslands of Yellowstone National Park. Land Degradation & Development 31, 2620-2634.
| Crossref | Google Scholar |
Chen XJ, Wang YR, Hou FJ (2011) Mechanisms and controlling factors of grassland ecosystem greenhouse gas emissions. Pratacultural Science 28(5), 722-728 [in Chinese with English abstract].
| Google Scholar |
Chen J, Wang Q, Li M, Liu F, Li W, Yin L (2013) Effects of deer disturbance on soil respiration in a subtropical floodplain wetland of the Yangtze River. European Journal of Soil Biology 56(1), 65-71.
| Crossref | Google Scholar |
Chen J, Shi W, Cao J (2015) Effects of grazing on ecosystem CO2 exchange in a meadow grassland on the Tibetan Plateau during the growing season. Environmental Management 55(2), 347-359.
| Crossref | Google Scholar | PubMed |
Chen H, Shao L, Zhao M, Zhang X, Zhang D (2017) Grassland conservation programs, vegetation rehabilitation and spatial dependency in Inner Mongolia, China. Land Use Policy 64, 429-439.
| Crossref | Google Scholar |
Chen S, Wang W, Xu W, Wang Y, Wan H, Chen D, Tang Z, Tang X, Zhou G, Xie Z, Zhou D, Shangguan Z, Huang J, He J-S, Wang Y, Sheng J, Tang L, Li X, Dong M, Wu Y, Wang Q, Wang Z, Wu J, Chapin FS, III, Bai Y (2018) Plant diversity enhances productivity and soil carbon storage. Proceedings of the National Academy of Sciences 115(16), 4027-4032.
| Crossref | Google Scholar |
Cui XY, Chen SQ, Chen ZZ (2000) CO2 release from typical Stipa grandis grassland soil. The Journal of Applied Ecology 11, 390-394 [in Chinese with English abstract].
| Google Scholar |
Drewry JJ, Cameron KC, Buchan GD (2008) Pasture yield and soil physical property responses to soil compaction from treading and grazing – a review. Soil Research 46, 237-256.
| Crossref | Google Scholar |
Ehsanul HMD (2016) Suitable integrated crop-livestock production system in char area of Bangladesh. Journal of Fisheries & Livestock Production 4(1), 156.
| Crossref | Google Scholar |
Ferreira GA, Oliveira PSRd, Alves SJ, Costa ACTd (2015) Soybean productivity under different grazing heights of Brachiaria ruziziensis in an integrated crop-livestock system. Revista Ciencia Agronomica 46(4), 755-763.
| Crossref | Google Scholar |
Ghani MU, Kamran M, Ahmad I, Arshad A, Zhang C, Zhu W, Lou S, Hou F (2022) Alfalfa-grass mixtures reduce greenhouse gas emissions and net global warming potential while maintaining yield advantages over monocultures. Science of The Total Environment 849, 157765.
| Crossref | Google Scholar | PubMed |
Gong J-R, Wang Y, Liu M, Huang Y, Yan X, Zhang Z, Zhang W (2014) Effects of land use on soil respiration in the temperate steppe of Inner Mongolia, China. Soil and Tillage Research 144(5), 20-31.
| Crossref | Google Scholar |
Guo Y, Ning J, Lou S, Zhang C, Zhu W, Hou F (2023) Grazing regulates soil N cycling of annual pastures by enhancing soil N turnover in arid climate condition. Land Degradation & Development 34(18), 5733-5743.
| Crossref | Google Scholar |
Hafner S, Unteregelsbacher S, Seeber E, Lena B, Xu X, Li X, Guggenberger G, Miehe G, Kuzyakov Y (2012) Effect of grazing on carbon stocks and assimilate partitioning in a Tibetan montane pasture revealed by 13CO2 pulse labeling. Global Change Biology 18(2), 528-538.
| Crossref | Google Scholar |
Han Q, Luo G, Li C, Xu W (2014) Modeling the grazing effect on dry grassland carbon cycling with Biome-BGC model. Ecological Complexity 17, 149-157.
| Crossref | Google Scholar |
Hanson PJ, Edwards NT, Garten CT, Andrews JA (2000) Separating root and soil microbial contributions to soil respiration: a review of methods and observations. Biogeochemistry 48, 115-146.
| Crossref | Google Scholar |
Hao X, Yang J, Dong S, He F, Zhang Y (2024) The influence of grazing intensity on soil organic carbon storage in grassland of China: a meta-analysis. Science of The Total Environment 924, 171439.
| Crossref | Google Scholar | PubMed |
Hatfield PG, Blodgett SL, Spezzano TM, Goosey HB, Lenssen AW, Kott RW, Marlow CB (2007) Incorporating sheep into dryland grain production systems: I. Impact on over-wintering larva populations of wheat stem sawfly, Cephus cinctus Norton (Hymenoptera: Cephidae). Small Ruminant Research 67(2-3), 209-215.
| Crossref | Google Scholar |
Heggenes J, Odland A, Chevalier T, Ahlberg J, Berg A, Larsson H, Bjerketvedt DK (2017) Herbivore grazing-or trampling? Trampling effects by a large ungulate in cold high-latitude ecosystems. Ecology and Evolution 7(16), 6423-6431.
| Crossref | Google Scholar | PubMed |
Holt JA (1997) Grazing pressure and soil carbon, microbial biomass and enzyme activities in semi-arid northeastern Australia. Applied Soil Ecology 5, 143-149.
| Crossref | Google Scholar |
Hopkins A, Wilkins RJ (2006) Temperate grassland: key developments in the last century and future perspectives. The Journal of Agricultural Science 144(6), 503-523.
| Crossref | Google Scholar |
Horn A, Pachmann G, Poschlod P (2013) Can sheep replace indigenous antelope as seed dispersers in the Kalahari? Journal of Arid Environments 91, 69-78.
| Crossref | Google Scholar |
Hou FJ, Nan ZB, Xie YZ, Li XL, Lin HL, Ren JZ (2008) Integrated crop-livestock production systems in China. The Rangeland Journal 30(2), 221-231.
| Crossref | Google Scholar |
Hou FG, Ning J, Feng QS (2016) The type and productivity of grassland grazing system. Pratacultural Science 33(3), 353-367 [in Chinese with English abstract].
| Google Scholar |
Hu Z, Zhao Z, Zhang Y, Jing H, Gao S, Fang J (2019) Does ‘Forage-Livestock Balance’ policy impact ecological efficiency of grasslands in China? Journal of Cleaner Production 207, 343-349.
| Crossref | Google Scholar |
Huang X, Lakso AN, Eissenstat DM (2005) Interactive effects of soil temperature and moisture on Concord grape root respiration. Journal of Experimental Botany 56(420), 2651-2660.
| Crossref | Google Scholar | PubMed |
Huang L, Ning J, Zhu P, Zheng Y, Zhai J (2021) The conservation patterns of grassland ecosystem in response to the forage-livestock balance in North China. Journal of Geographical Sciences 31(4), 518-534.
| Crossref | Google Scholar |
IPCC (2013) Climate change 2013: the physical science basis. In ‘Contribution of Working Group I to the fifth assessment report of the intergovernmental panel on climate change’. (Eds TF Stocker, D Qin, G-K Plattner, M Tignor, SK Allen, J Boschung, A Nauels, Y Xia, V Bex, PM Midgley) pp. 659–740. (Cambridge University Press: Cambridge, UK)
Jia B, Zhou G, Wang F, Wang Y, Weng E (2007) Effects of grazing on soil respiration of Leymus chinensis steppe. Climatic Change 82, 211-223.
| Crossref | Google Scholar |
Johnston ASA, Sibly RM (2018) The influence of soil communities on the temperature sensitivity of soil respiration. Nature Ecology & Evolution 2, 1597-1602.
| Crossref | Google Scholar | PubMed |
Kamran M, Yan Z, Jia Q, Chang S, Ahmad I, Ghani MU, Hou F (2022) Irrigation and nitrogen fertilization influence on alfalfa yield, nutritive value, and resource use efficiency in an arid environment. Field Crops Research 284, 108587.
| Crossref | Google Scholar |
Kim JJ, Ale S, Kreuter UP, Richard Teague W, DelGrosso SJ, Dowhower SL (2023) Evaluating the impacts of alternative grazing management practices on soil carbon sequestration and soil health indicators. Agriculture, Ecosystems & Environment 342, 108234.
| Crossref | Google Scholar |
Lal R (2004a) Soil carbon sequestration to mitigate climate change. Geoderma 123, 1-22.
| Crossref | Google Scholar |
Lal R (2004b) Agricultural activities and the global carbon cycle. Nutrient Cycling in Agroecosystems 70, 103-116.
| Crossref | Google Scholar |
Li Z (2017) Grassland management in China: countermeasures to reverse degradation. China Economist 1, 98-117 [in Chinese with English abstract].
| Google Scholar |
Li SX, Wang ZH, Li SQ, Gao YJ (2015) Effect of nitrogen fertilization under plastic mulched and non-plastic mulched conditions on water use by maize plants in dryland areas of China. Agricultural Water Management 162, 15-32.
| Crossref | Google Scholar |
Li X, Huang Q, Mi X, Bai Y, Zhang M, Li X (2018) Grazing every month minimizes size but boosts photosynthesis in Stipa grandis in the steppe of Inner Mongolia, China. Journal of Arid Land 10, 601-611.
| Crossref | Google Scholar |
Li S, Xing T, Sa R, Zhang Y, Chen H, Jin K, Shao Q, Tang S, Wang C (2024) Effects of grazing on soil respiration in global grassland ecosystems. Soil and Tillage Research 238, 106033.
| Crossref | Google Scholar |
Liu N, Zhang Y, Chang S, Kan H, Lin L (2012) Impact of grazing on soil carbon and microbial biomass in typical steppe and desert steppe of Inner Mongolia. PLoS ONE 7(5), e36434.
| Crossref | Google Scholar | PubMed |
Liu N, Kan HM, Yang GW, Zhang YJ (2015) Changes in plant, soil, and microbes in a typical steppe from simulated grazing: explaining potential change in soil C. Ecological Monographs 85(2), 269-286.
| Crossref | Google Scholar |
Liu H, Zang R, Chen HYH (2016) Effects of grazing on photosynthetic features and soil respiration of rangelands in the Tianshan Mountains of Northwest China. Scientific Reports 6(1), 30087.
| Crossref | Google Scholar |
Ludvíková V, Pavlů VV, Gaisler J, Hejcman M, Pavlů L (2014) Long term defoliation by cattle grazing with and without trampling differently affects soil penetration resistance and plant species composition in Agrostis capillaris grassland. Agriculture, Ecosystems & Environment 197, 204-211.
| Crossref | Google Scholar |
Luo C, Xu G, Wang Y, Wang S, Lin X, Hu Y, Zhang Z, Chang X, Duan J, Su A (2009) Effects of grazing and experimental warming on DOC concentrations in the soil solution on the Qinghai-Tibet plateau. Soil Biology and Biochemistry 41(12), 2493-2500.
| Crossref | Google Scholar |
Mikola J, Setälä H, Virkajärvi P, Saarijärvi K, Ilmarinen K, Voigt W, Vestberg M (2009) Defoliation and patchy nutrient return drive grazing effects on plant and soil properties in a dairy cow pasture. Ecological Monographs 79(2), 221-244.
| Crossref | Google Scholar |
Ning J, He XZ, Hou F, Lou S, Chen X, Chang S, Zhang C, Zhu W (2020) Optimizing alfalfa productivity and persistence versus greenhouse gases fluxes in a continental arid region. PeerJ 8, e8738.
| Crossref | Google Scholar | PubMed |
Nissan A, Alcolombri U, Peleg N, Galili N, Jimenez-Martinez J, Molnar P, Holzner M (2023) Global warming accelerates soil heterotrophic respiration. Nature Communications 14, 3452.
| Crossref | Google Scholar | PubMed |
Niu DC, Jiang SG, Qin Y, Zhang BL, Cao GT, Fu H (2013) Effects of grazing and fencing on soil microorganisms and enzymes activities. Pratacultural Science 30(4), 528-534 [in Chinese with English abstract].
| Google Scholar |
Piao S, Fang J, Ciais P, Peylin P, Huang Y, Sitch S, Wang T (2009) The carbon balance of terrestrial ecosystems in China. Nature 458, 1009-1013.
| Crossref | Google Scholar | PubMed |
Piñeiro G, Paruelo JM, Oesterheld M, Jobbágy EG (2010) Pathways of grazing effects on soil organic carbon and nitrogen. Rangeland Ecology & Management 63(1), 109-119.
| Crossref | Google Scholar |
Rui Y, Wang S, Xu Z, Wang Y, Chen C, Zhou X, Kang X, Lu S, Hu Y, Lin Q, Luo C (2011) Warming and grazing affect soil labile carbon and nitrogen pools differently in an alpine meadow of the Qinghai-Tibet Plateau in China. Journal of Soils and Sediments 11(6), 903-914.
| Crossref | Google Scholar |
Sanderman J, Reseigh J, Wurst M, Young M-A, Austin J (2015) Impacts of rotational grazing on soil carbon in native grass-based pastures in Southern Australia. PLoS ONE 10(8), e0136157.
| Crossref | Google Scholar |
Schrama M, Heijning P, Bakker JP, van Wijnen HJ, Berg MP, Olff H (2013) Herbivore trampling as an alternative pathway for explaining differences in nitrogen mineralization in moist grasslands. Oecologia 172, 231-243.
| Crossref | Google Scholar | PubMed |
Shen H, Dong S, Li S, Xiao J, Han Y, Yang M, Zhang J, Gao X, Xu Y, Li Y, Zhi Y, Liu S, Dong Q, Zhou H, Yeomans JC (2019) Grazing enhances plant photosynthetic capacity by altering soil nitrogen in alpine grasslands on the Qinghai-Tibetan plateau. Agriculture, Ecosystems & Environment 280, 161-168.
| Crossref | Google Scholar |
Shi H, Hou L, Yang L, Wu D, Zhang L, Li L (2017) Effects of grazing on CO2, CH4, and N2O fluxes in three temperate steppe ecosystems. Ecosphere 8(4), e01760.
| Crossref | Google Scholar |
Šimek M, Čuhel J (2009) Effects of cattle grazing, trampling and excrement deposition on microbial nitrogen transformations in upland soil. In ‘Current research topics in applied microbiology and microbial biotechnology – II international conference on environmental, industrial and applied microbiology’. pp. 29–32. (World Scientific Publishing Co PTE: Singapore). doi:10.1142/9789812837554_0006
Sui X, Zhou G (2013) Carbon dynamics of temperate grassland ecosystems in China from 1951 to 2007: an analysis with a process-based biogeochemistry model. Environmental Earth Sciences 68, 521-533.
| Crossref | Google Scholar |
Sun J, Jiao T, Li Y, Wang F, Wang P, Yu X (2019) A study on the optimal allocation of the Alpine Pasture Grassland-Livestock – a case of a Herder in Maqin County, Qinghai Province. Acta Agrestia Sinica 27(3), 728-735.
| Google Scholar |
Tang X, Zhao X, Bai Y, Tang Z, Wang W, Zhao Y, Wan H, Xie Z, Shi X, Wu B, Wang G, Yan J, Ma K, Du S, Li S, Han S, Ma Y, Hu H, He N, Yang Y, Han W, He H, Yu G, Fang J, Zhou G (2018) Carbon pools in China’s terrestrial ecosystems: new estimates based on an intensive field survey. Proceedings of the National Academy of Sciences 115, 4021-4026.
| Crossref | Google Scholar |
Thomas BW, Gao X, Zhao M, Bork EW, Hao X (2018) Grazing altered carbon exchange in a dry mixed-grass prairie as a function of soil texture. Canadian Journal of Soil Science 98(1), 136-147.
| Google Scholar |
Tong L, Zhao B, Wu LM (2018) Effect of grazing on soil organic carbon fractions and soil physical-chemical properties in desert steppe in Inner Mongolia. Ecology and Environmental Sciences 27(9), 1602-1609 [in Chinese with English abstract].
| Google Scholar |
Treweek G, Di HJ, Cameron KC, Podolyan A, Goss M (2016) Simulated animal trampling of a free-draining stony soil stimulated denitrifier growth and increased nitrous oxide emissions. Soil Use and Management 32, 455-464.
| Crossref | Google Scholar |
Wang C, Long R (2008) Changes in soil organic carbon and microbial biomass carbon at different degradation successional stages of alpine meadows in the headwater region of three rivers in China. Journal of Applied & Environmental Biology 14, 225-230 [in Chinese with English abstract].
| Google Scholar |
Wang C-Y, Zhang J-J, Lv Y-L, Wang L, He N-P (2014a) Effects of long-term grazing exclusion on soil organic carbon fractions in the grasslands of Inner Mongolia. Acta Prataculturae Sinica 23(5), 31-39 [in Chinese with English abstract].
| Google Scholar |
Wang D, Geng ZC, She D, He WX, Hou L (2014b) Vertical distribution of soil active carbon and soil organic carbon storage under different forest types in the Qinling Mountains. Chinese Journal of Applied Ecology 25(6), 1569-1577.
| Google Scholar | PubMed |
Wang P, Deng X, Jiang S (2019a) Global warming, grain production and its efficiency: case study of major grain production region. Ecological Indicators 105, 563-570.
| Crossref | Google Scholar |
Wang Y, Yuan H, Zhang X, Sun Y, Chang S, Li G, Hou F (2019b) Tibetan sheep grazing modifies rodent density and their interactions effect on GHG emissions of alpine meadow. Scientific Reports 9, 17066.
| Crossref | Google Scholar | PubMed |
Wei Y, Zhang Y, Wilson GWT, Guo Y, Bi Y, Xiong X, Liu N (2021) Transformation of litter carbon to stable soil organic matter is facilitated by ungulate trampling. Geoderma 385, 114828.
| Crossref | Google Scholar |
Wen D, He N, Zhang J (2016) Dynamics of soil organic carbon and aggregate stability with grazing exclusion in the Inner Mongolian grasslands. PLoS ONE 11(1), e0146757.
| Crossref | Google Scholar |
Wiesmeier M, Urbanski L, Hobley E, Lang B, von Lützow M, Marin-Spiotta E, van Wesemael B, Rabot E, Ließ M, Garcia-Franco N, Wollschläger U, Vogel H-J, Kögel-Knabner I (2019) Soil organic carbon storage as a key function of soils – a review of drivers and indicators at various scales. Geoderma 333, 149-162.
| Crossref | Google Scholar |
Xu H, You C, Tan B, Xu L, Liu Y, Wang M, Xu Z, Sardans J, Peñuelas J (2023) Effects of livestock grazing on the relationships between soil microbial community and soil carbon in grassland ecosystems. Science of The Total Environment 881, 163416.
| Crossref | Google Scholar | PubMed |
Yan Z-Q, Qi Y-C, Dong Y-S, Peng Q, Sun L-J, Jia J-Q, Cao C-C, Guo S-F, He Y-L (2014) Nitrogen cycling in grassland ecosystems in response to climate change and human activities. Acta Prataculturae Sinica 6, 279-292.
| Crossref | Google Scholar |
Yang X, Ren W, Sun B, Zhang S (2012) Effects of contrasting soil management regimes on total and labile soil organic carbon fractions in a loess soil in China. Geoderma 177–178, 49-56.
| Crossref | Google Scholar |
Yang C, Zhang Y, Hou F, Millner JP, Wang Z, Chang S (2019) Grazing activity increases decomposition of yak dung and litter in an alpine meadow on the Qinghai-Tibet plateau. Plant and Soil 444, 239-250.
| Crossref | Google Scholar |
Yang J, Lai X, Shen Y (2022) Response of dual-purpose winter wheat yield and its components to sowing date and cutting timing in a semiarid region of China. Crop Science 62, 425-440.
| Crossref | Google Scholar |
Zeleke KT (2019) Effect of grazing time and intensity on growth and yield of spring wheat (Triticum aestivum L.). Journal of Integrative Agriculture 18(5), 1138-1147.
| Crossref | Google Scholar |
Zeng Q, Li X, Dong Y, Li Y, An S (2015) Soil microbial biomass nitrogen and carbon, water soluble nitrogen and carbon under different arbors forests on the Loess Plateau. Acta Ecologica Sinica 35(11), 3598-3605.
| Crossref | Google Scholar |
Zhang M, Li X, Wang H, Huang Q (2018) Comprehensive analysis of grazing intensity impacts soil organic carbon: a case study in typical steppe of Inner Mongolia, China. Applied Soil Ecology 129, 1-12.
| Crossref | Google Scholar |
Zhao J, Li X, Li R, Tian L, Zhang T (2016) Effect of grazing exclusion on ecosystem respiration among three different alpine grasslands on the central Tibetan Plateau. Ecological Engineering 94(1), 599-607.
| Crossref | Google Scholar |
Zhao F, Ren C, Shelton S, Wang Z, Pang G, Chen J, Wang J (2017) Grazing intensity influence soil microbial communities and their implications for soil respiration. Agriculture, Ecosystems & Environment 249, 50-56.
| Crossref | Google Scholar |
Zhao Z, Chen J, Bai Y, Wang P (2020) Assessing the sustainability of grass-based livestock husbandry in Hulun Buir, China. Physics and Chemistry of the Earth, Parts A/B/C 120, 102907.
| Crossref | Google Scholar |
Zheng S, Lan Z, Li W, Shao R, Shan Y, Wan H, Taube F, Bai Y (2011) Differential responses of plant functional trait to grazing between two contrasting dominant C3 and C4 species in a typical steppe of Inner Mongolia, China. Plant and Soil 340, 141-155.
| Crossref | Google Scholar |
Zhou X, Wang X, Zhang H, Pang J, Ren Y, Hou P, Liu W (2013) Seasonal and interannual variability in soil respiration in wheat field of the Loess Plateau, China. Acta Ecologica Sinica 33(23), 7525-7536.
| Crossref | Google Scholar |