Coastal geomorphology and geology of the Gippsland Lakes region: a review and future directions
David M. Kennedy
A
B
C
D
Abstract
The Gippsland Lakes region is located on Gunaikurnai Country and is principally within the territories of the Tatungalung and Krauatungalung clans. It contains arguably the best-preserved barrier island sequence in Australia that represents deposition during sea level highstands dating back to at least Marine Isotope Stage (MIS) 7. The beach barrier and enclosed estuarine sequences are also a significant economic and social asset for Victoria. This review brings together and integrates the geomorphic research on the barriers initiated with the doctoral studies of the late Eric Bird in the mid-20th century. The Gippsland Lakes form within the depressions between three barrier sequences – the Prior Barrier (MIS 7), Inner Barrier (MIS 5) and Outer Barrier (MIS 1). Ninety Mile Beach occurs on the seaward edge of the Outer Barrier and represents a littoral sediment transport system that extends from Corner Inlet, north through the region, and eventually into New South Wales. The Outer Barrier and Ninety Mile Beach are highly dynamic environments with major phases of reworking occurring in the past 200 years related to changing land management practices. In the past few decades it appears that the system is now trending towards erosion, with instances of wave overwash and landward dune rollover becoming more apparent. To understand whether the system has reached a tipping point towards long-term erosion (barrier transgression) it is urgent for the current sedimentary system to be understood in much greater detail and modelling undertaking on future trajectories of shoreline change. This research is needed to understand the resilience of the system to climate change at the spatial scale needed to properly ‘care for Country’.
Keywords: barrier islands, beach, climate change, dunes, geomorphology, Gippsland, Ninety Mile Beach, sea level.
Introduction
The Gippsland Lakes is one of the most diverse coastal environments in Australia. It contains extensive RAMSAR-listed wetlands, beaches, dunes, fluvial and tidal deltas and a variety of estuarine systems. It is also the only active barrier-island system in Australia. The diversity of physical geography in the region, all being based on deposition in relation to sea level, means the region is considered the most vulnerable in Australia to future climate change (DCC 2009).
The region is located on Gunaikurnai Country. The area is principally within the territories of the Tatungalung and Krauatungalung clans, and bordered by the Brataualung, Brayakaulung and Brabralung clan areas. Gippsland is of great cultural significance, providing important story places that relate to all aspects of Gunaikurnai life and world views, both past and present (Birkett-Rees et al. 2023). Their occupation of the land extends back to over 30,000 years (David et al. 2021), and is evidenced by middens, artifact scatters and burial sites. The impacts of climate change are of great concern to traditional owners with >80% of recorded archaeological sites being impacted by environmental change associated with a warming climate (Birkett-Rees et al. 2023).
The coastal landforms and marine geology are also an important economic resource for a range of sectors. The offshore Gippsland Basin continues to be a significant hydrocarbon province. Gas and oil are piped onshore at Loch Grange, Paradise Beach and Seaspray for processing then distributed in pipelines northward to Sydney in New South Wales and also to Victoria and South Australia (AEMO 2023). The Gippsland Lakes are central to tourism in the region which generates an estimated $1.57 billion for the local economy from 5.63 million visitors annually (Destination Gippsland 2023). The region is home to a large agribusiness sector which occupies 1.64 million ha and produces $1.16 billion in commodities. Dairy production accounts for about a third of Victoria’s production and 19% of Australia’s. A significant grazing industry is based on beef production of which a major proportion is exported. Wool and prime lamb production is also important as is vegetable production and fishing (Destination Gippsland 2023).
This paper aims to review the geology of the Gippsland Lakes region with a focus on coastal geomorphology in the late Pleistocene and Holocene epochs. It seeks to understand how the coast has behaved during past and current climatic conditions in order to identify the existing knowledge gaps which prevent researchers and managers from caring for Country in a warmer world.
Gippsland Basin
The Gippsland Basin underlies the region forming the continental shelf basement of eastern Victoria and extending into the Latrobe Valley onshore. The basin was formed during the Mesozoic period as a result of rifting associated with the breakup of the eastern Gondwana and creation of the Tasman Sea. It is infilled with up to 7.5 km of sediments (James and Evans 1971; Baillie et al. 2019) and the basin is most well-known for its petroleum resources. Sediments of the Seaspray Group, a cool-water carbonate shelf depositional environment, form a seal for the oil and gas resources (Holdgate et al. 2003) and in places this unit is exposed at the surface.
The southern end of the basin is defined by the Darriman and Foster Fault systems which mark the Southern Terrace zone. The study area is framed within this zone with the Foster Fault system extending into the middle of Corner Inlet (James and Evans 1971; Baillie et al. 2019). Vertical movement on these fault systems has led to the creation of vertical space in which sediments can accumulate on the coast as well as in the large depositional basin of Corner Inlet (Bird 1993). These major fault systems within the basement geology, while providing the geological boundaries for landform development, are considered inactive during the Holocene (Cupper et al. 2003) and therefore can be assumed not to affect present-day sediment transport or the region’s response to climate change.
Surface geology
Contemporary shoreline
The oldest units found exposed on the modern coast are the Middle Devonian (380 Ma) Mount Singapore Granite on Wilsons Promontory (Fig. 1). These lithologies are an S-type monzogranite (Rossiter 2003) which are relatively high in Rb and low in Ba and Sr with occasional concentrations of garnet (Chappell et al. 1988). Weathering of the granite during the Mesozoic occurred to depths of over 300 m with much of this regolith being subsequently eroded, especially at the coast (Hill and Joyce 1995; Hill et al. 1995). The topography of Wilsons Promontory is strongly influenced by tectonic activity dating after the early-Cretaceous with vertical fault movement contributing to the elevation of palaeoerosion surfaces (Hill et al. 1995).
A simplified surficial geological map of the Gippsland Basin Bioregion, located in the southeastern corner of mainland Australia (Data sourced from Bioregional Assessments 2015).
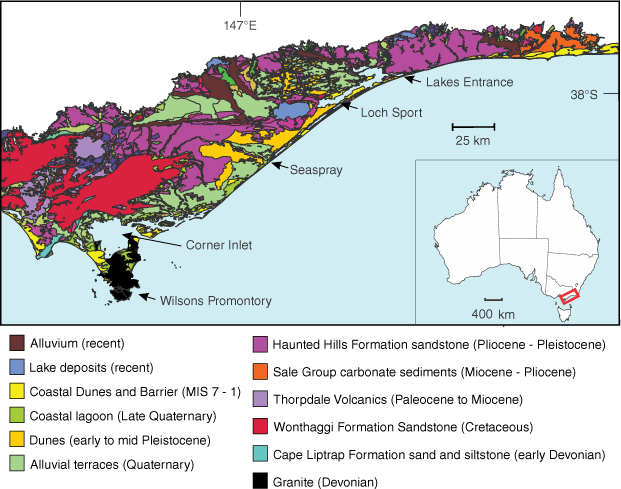
The next oldest sediments to be exposed on the open-ocean coast are older limestones observed to outcrop on the beachface and surf zone at McGaurans Beach following storms (N Rosengren unpubl. data). This represents the uppermost and younger parts of the Seaspray Group of carbonates described by Holdgate et al. (2003) elsewhere in the basin.
The remaining units, which account for nearly all of the Ninety Mile Beach coast are composed of late Neogene deposits which form a coastal plain up to tens of kilometres wide, behind which low-energy swamp deposits are found. The width of the Holocene sequences is determined by the presence of Tertiary age terraces which are closest to the modern-day coast at McGaurans Beach. Where the coastal plain is wider, from approximately Seaspray north to Lakes Entrance, the lacustrine environment of the Gippsland Lakes region has formed where beach-barrier sequences of Last Interglacial and Penultimate Interglacial age are also found (Bird 1961, 1965; Thom 1984; Oliver et al. 2018; Kennedy et al. 2020).
Shelf environment
The southern extent of the contemporary offshore sedimentary environment is the Bassian Rise which extends from Wilsons Promontory to Flinders Island in Bass Strait. This rise separates the Gippsland and Bass basins and forms a morphological divide between the study area and the contemporary sedimentary environments further south. The sea floor of the inner shelf, adjacent to the study area, is broadly composed of gravelly sand with isolated patches of sand and gravelly muddy sand (James et al. 2008) with a mean grain size of 1–2 ɸ (ɸ units = −Log2(mm units)) coarsening to greater than −1 ɸ offshore in an easterly direction (Blom and Alsop 1988) (Fig. 2). The surface sediments of the inner shelf are broadly Holocene in age and are composed of biogenic gravel, sand and mud (James et al. 2008), with a mineralogy of over 70% carbonate (Blom and Alsop 1988). Closer to shore in the upper shoreface, in the zone of principal sediment transport, the carbonate content is lower (<50%) (Blom and Alsop 1988). In some locations, such as the bedrock defined bays of Wilsons Promontory, the sandy sediments are observed to be entirely quartzose, representing a local source of sand from the surrounding granitic hills (Ierodiaconou et al. 2018). On the inner shelf, bryozoan-derived sediment dominate the carbonate fraction with this relict biogenic material becoming dominant at the shoreface offshore of Ninety Mile Beach (James et al. 2008) and also coinciding with the observed outcrops of carbonate rocks of the Seaspray Group in the surf zone.
The different grain sizes of surface sediment on the continental shelf and in Bass Strait (left map) and the distribution of surface sediment facies, based on study of samples indicated, on the continental shelf off Tasmania and in Bass Strait (right map) (Source: James et al. (2008); reproduced with permission – Elsevier licence # 5659610806113).
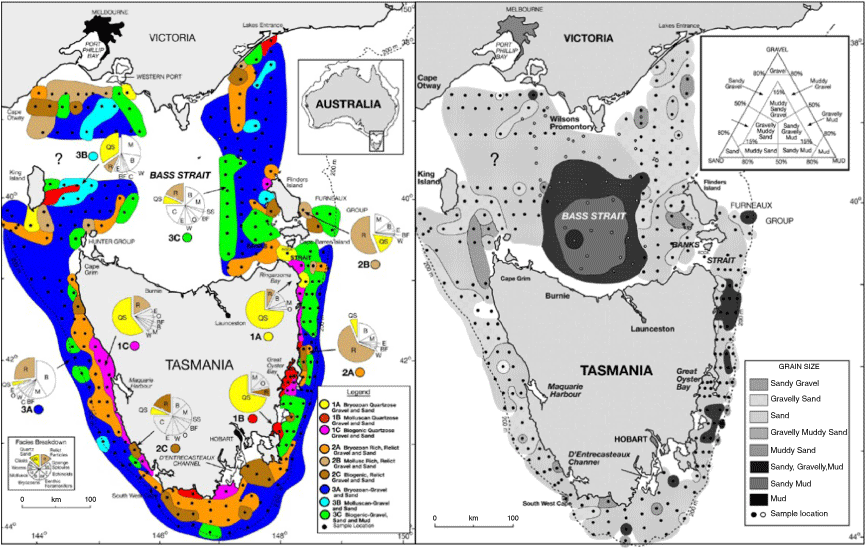
The contemporary inner shelf environment is not a major sediment source for the shorelines of Ninety Mile Beach north of Corner Inlet. It may have contributed some sediment to onshore barrier construction as sea level transgressed across its surface, drowned beach-barrier sequences are present on its outer edges (Brooke et al. 2017), but is considered to be a ravinement surface (Holdgate et al. 2003) with little connection to the modern coast. During the early to mid-Holocene, as sea level stabilised at modern elevations after rising from its lowstand during the last Glacial Maximum, the beach barriers of the contemporary coast were initially built from sediment transported from the inner shelf (Bird 1961, 1965, 1971; Thom 1984; Kennedy et al. 2020); however, by the late Holocene this source appears to have been exhausted with sediment supply being primarily sourced longshore from Corner Inlet in the south (Kennedy et al. 2020). There is currently a dearth of information on the degree of connectiveness between the modern littoral and shallow marine sedimentary systems. Investigations of the degree to which the modern shelf is a source or sink for the contemporary beaches is critical for understanding the resilience of the coast to future sea level rise and changed wave conditions in a warmer world.
Broad coastal geomorphology of the Gippsland Lake barrier sequences
The coastal geomorphology of the Gippsland region has been studied since the mid 20th century. The late Prof. Eric Bird (Bird 1961, 1965, 1971) provided some of the early discussions of landform evolution, with a focus on the Lakes region. This was closely followed by Jenkin (Jenkin 1968) who debated some of Bird’s interpretations while also extending landform analysis to the southern extent of the region in Corner Inlet. A renewal of interest in the evolution of the Gippsland Lakes occurred in the mid-1980s and 1990s, principally by Prof. Bruce Thom who undertook a drilling program to better understand the morphostratigraphy of the region (Thom 1984; Thom et al. 1994). He and Prof. Ted Bryant (Bryant and Price 1997) were the first to systematically apply quantitative dating techniques (luminescence and C14) to the area. The most recent exploration of the geomorphology has been led by this author (Oliver et al. 2018; Kennedy et al. 2020), through the application of the latest aerial surveying and dating techniques, bringing together much of the previous work and more recent research.
The following description summarises this work. There are three principal periods of dune and beach development in the Gippsland Lakes, each occurring during highstand sea level periods. These are:
Prior Barrier (~220,000 years in age)
Inner Barrier (80,000–125,000 years in age)
Outer Barrier (<8000 years in age).
Bird first defined the broad range of barrier ages based on their geographical position relative to the modern shore. Their elevation, and subsequent quantitative dating of sediments, has confirmed they are indeed three distinct units and relate to interglacial warmer climatic periods, such as occurs today. Each barrier therefore represents a period of high sea level. They are each geographically separated by a trough containing the modern lakes and temporally by a long cold glacial period. During each phase of sea level rise, sand is swept off the continental shelf to be deposited as a new barrier sequence seaward of the previous one (Fig. 3).
A picture of the changes to the Gippsland Lakes landforms during the past three sea level highstands going back to 220,000 years ago. Each period of higher sea level leads to the creation of a beach-dune barrier with a lake separating each sequence. The lakes are formed in troughs that develop between the successive barriers which form seaward of the former beach sequences (Modified from Oliver et al. 2018).
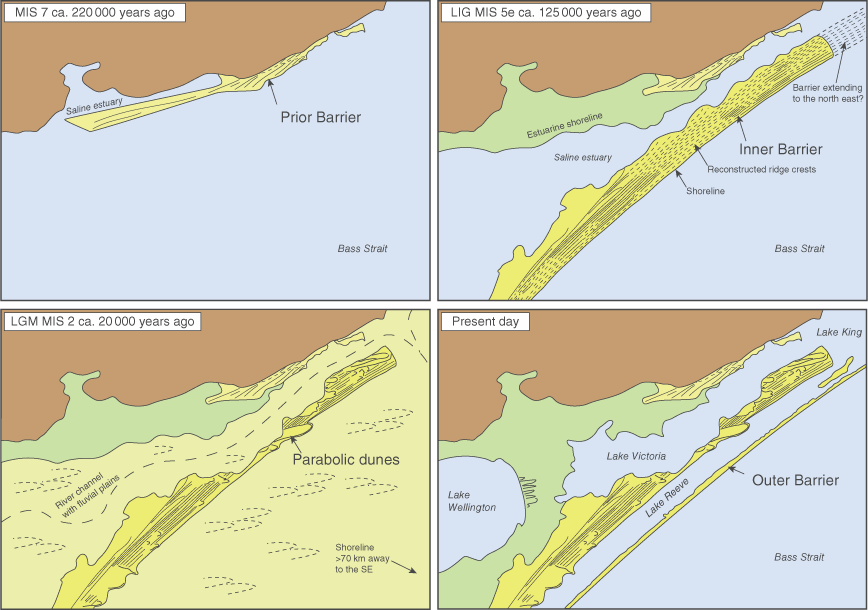
The following describes the current understanding of the evolution of the beach-barrier sequences.
Penultimate Interglacial (Prior Barrier), ~220,000 years ago (MIS 7)
This earliest phase of consolidated beach-barrier development is found in the Prior Barrier sequences (Fig. 3). These are located at the inland limit of the Gippsland Lakes, and the sequence is best represented at Banksia Peninsula. The sediments are fine to medium grained and white, with the colour representing bleaching that has occurred in the extended period between their deposition and today.
Apart from the descriptive work of Bird and Jenkin, there has been little detailed investigation of this sequence. At present there is no direct evidence of its age, and this remains a significant gap in the understanding of how the coast of Gippsland behaves during periods of high sea level such as today and into the future. The age of ~220,000 years ago is an estimate based on the assumption that sea level at that time was at or near present elevations in this region. It is also estimated based on its geomorphology and position relative to the well-dated sequences of younger age located seaward (see below).
Last Interglacial (Inner Barrier), 80,000–125,000 years ago (MIS 5a–5e)
The Inner Barrier is typified by the sand sequence between Lake Victoria and Lake Reeve, on which Loch Sport is located (Fig. 4). The sequence was described by Jenkin (1968) who classified it as Phase Iva age under his stratigraphic timeline. The dating in Oliver et al. (2018) provides the most accurate and precise age of the sequence. This most recent work, using optically stimulated luminescence dating, confirms and refines many of the previous qualitative inferences and finalises the pre-existing debates on sea level change and landform response in the region. It is a composite feature involving deposition of marine, estuarine and aeolian deposits.
Topographic LiDAR images of the three barrier sequences in which the Gippsland Lakes are formed. The luminescence ages on the Inner Barrier and adjacent to the Prior Barrier are derived from Oliver et al. (2018).
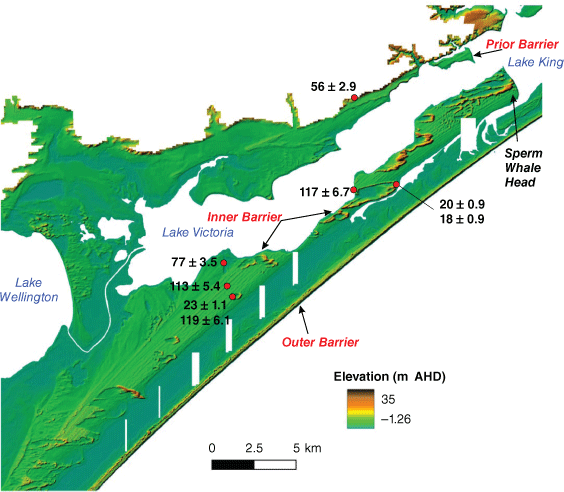
The Inner Barrier was deposited primarily around 120,000–130,000 years ago (Fig. 3). This period is known as the Last Interglacial (MIS 5e) when global sea level was 3–5 m higher than present elevations (Murray-Wallace and Woodroffe 2014). The barrier has formed as a series of parallel ridges which are elevated around 7 m above present sea level. The ridges are former foredunes formed on top of beach deposits. The size and shape of the dunes, as well as the sediment they contain, are very similar to the modern beach (Oliver et al. 2018) (Fig. 4). We can therefore assume that this sequence formed, and behaved, in a similar manner to the modern-day beach.
One enigmatic age (77 ± 3.5 thousand years, MIS 5a) (Oliver et al. 2018) has been recorded at its inland edge near Loch Sport (Fig. 4). This area has a distinctly different morphological character to the rest of the dune ridges and occurs on a ‘terrace’ 2 m lower in elevation (at 5.5 m AHD) than the main foredune ridges. The area contains less distinct ridges which appear to spread out in a fan shape in a northerly direction. The timing of deposition of this sequence occurs at the end of the Last Interglacial when sea level was 20‒30 m lower than the peak of the highstand. It has been preliminarily interpreted to be an estuarine shoreline forming in a lake environment. This phase of deposition has only recently been discovered and not previously described elsewhere in the Gippsland Lakes.
Dune reworking during the Last Glacial Period, 60,000–20,000 years ago (MIS 3–2)
Between the interglacial periods, the climate of Gippsland was colder, drier and windier than today. Winds blew from a mostly westerly direction. As sea level was up to 120 m lower than present – during the coldest peak of the Last Ice Age – a land bridge connected the Gippsland region to Tasmania with Bass Strait being dry and above sea level (Fig. 3).
The beaches and dunes of the Prior and Inner Barrier were stranded above sea level during this time and subjected to reworking by wind. A lower cover of vegetation occurred due to the harsher climate (Hill and Bowler 1995). This meant the dunes formed previously at the coast could now be remobilised. This led to the creation of blow-outs and parabolic dunes, U-shaped dunes features that are oriented along the average wind direction (generally westerly). These dunes are now stable and are most prominent along the Inner Barrier at Sperm Whale Head (Fig. 4). These dunes were most active around 18,000–20,000 years ago, with additional evidence of dune activity in the Prior Barrier occurring at around 40,000–50,000 years ago (Bryant and Price 1997; Oliver et al. 2018).
The period of dune reworking is not unique to the Loch Sport region of the Gippsland Lakes. Dunes of this orientation have been described around the margins of Corner Inlet and along the Yanakie Peninsula (Hill and Bowler 1995). These have all been inferred to be active during the peak of the last Ice Age and therefore indicate regional aeolian reworking around this time. This phase of reworking ended when the climate began to warm, and sea level began to rise to present elevations (Oliver et al. 2018; Kennedy et al. 2020).
Holocene (Outer Barrier), 10,000 years ago – today (MIS 1)
The Outer Barrier encompasses Ninety Mile Beach and the dune systems immediately behind it (Fig. 3). It is part of a continuous littoral drift system that extends from the entrance to Corner Inlet northeast of Wilson’s Promontory all the way to Mallacoota near the New South Wales border. This discussion will, however, focus on that part of the barrier where dating has been undertaken and therefore the areas where the evolutionary history is most well constrained (Kennedy et al. 2020). This area extends from McLoughlins Beach to Lakes Entrance.
During the Holocene, sea level rose quickly from its lowest elevation of c. −120 m around 18,000 years ago reaching modern elevations by around 7000 years ago. Sea level history in the Gippsland region since then is best defined by an envelope as indicators of higher or lower positions can reflect a range of geomorphic processes (Kennedy et al. 2020). In other parts of Victoria there are indications that it may have been higher, e.g. estuarine muds (Kennedy et al. 2021) and raised notches (Baker et al. 2001; Gardner et al. 2006). Quantification of the presence, extent, and height of sea level within the Holocene remains an unanswered but fundamentally critical question to be solved for understanding the response of Victoria’s coast to global warming.
The first part of the outer barrier to form began as islands around 9500 years ago at Rotamah Island (Fig. 5). This represents the earliest known deposition on the coast during the Holocene. As sea level had not yet stabilised, the island was likely transgressive in nature, moving landward as the shoreline migrated inland. It likely looked similar to the active islands at the mouth of modern-day Corner Inlet. Soon after sea level stabilisation, further offshore islands formed in what is today the Golden Beach area. The source of sand for these offshore islands was likely the shallow marine area immediately seaward.
A schematic model for the evolution of the Holocene barrier. The first phase of evolution saw island accretion in the northern part followed by a long barrier island fronting the pre-Holocene barriers. A final phase of evolution was related to an input of sediment from the southwestern end of the barrier which infilled the tidal entrance in the north and created the Bunga Arm enclosing the earliest Holocene beach barrier within the Gippsland Lakes (Sourced from Kennedy et al. 2020 and reproduced with permission – Elsevier licence # 5660121063809).
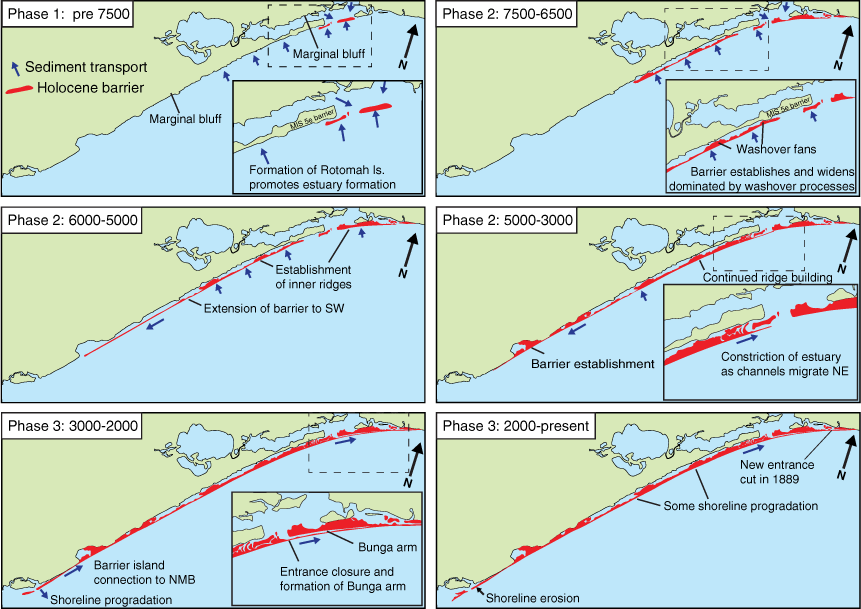
These offshore islands continued to build vertically until around 6500 years ago, before starting to extend in a southerly direction until 3000 years ago. This extension led to the formation of the lake system seaward of Loch Sport. The lakes remained open at this time, with a free connection to the open ocean through a large tidal channel located at the present day Loch Grange. Rotomah Island and others in the vicinity were still isolated sandy islands exposed to the open ocean (Fig. 5).
The next major change occurred after 3000 years ago, it appears that infill of Corner Inlet (details of which are unknown) reached a stage where sand could be transported northwards along the Outer Barrier (Fig. 5). The beach ridges at McLoughlins Beach rapidly built in a 500-year window between 2000 and 2500 years ago. Sand moved quickly along Ninety Mile Beach, infilling the entrance at Loch Grange and then forming another barrier seaward of Rotomah Island leading to the creation of the Bunga Arm. This resulted in a natural entrance, near where Lakes Entrance is today, as the only connection between the Gippsland Lakes and the open ocean.
A final phase of dune mobility has occurred in the last 200 years. Reworking appears to have occurred along the seaward edge of the entire beach system with the barrier rolling over in a landward direction. Fresh sand is observed to bury older soil surfaces along the entire front section of the Outer Barrier. The timing of this phase of mobility, in the last 200 years, suggests it is a result of changed land management from 1850s up to the 1980s. All these areas are now broadly stable due to the removal of stock and introduction of Marram Grass (Ammophila sp.), as well as changed fire patterns.
Modern day coastal dynamics
The decadal-scale dynamics of Ninety Mile Beach is well represented in the analysis of Landsat Satellite imagery available from 1988. Using a machine-learning approach (Bishop-Taylor et al. 2021) this imagery is used to track the position of the wetted-shoreline, thereby providing a measure of the planform position of mean sea level (i.e. the shoreline) through time (DEA 2022). Based on this analysis (Fig. 6), the current open-ocean coastline of the Gippsland Lakes is stable to eroding, with significant accretion only occurring around the engineered mouth to Lakes Entrance and on the islands that mark the seaward boundary of Corner Inlet (Fig. 6).
Rates of shoreline retreat along Ninety Mile Beach as calculated through shoreline analysis of LandSat imagery. The Bunga Arm (a), McGaurans Beach (b) and McLoughlins Beach (c) are the areas undergoing the most significant erosion along the shoreline. The majority of the beach is stable with minor areas of accretion occurring at Corner Inlet and adjacent to the current estuary mouth (Source: DEA 2023).
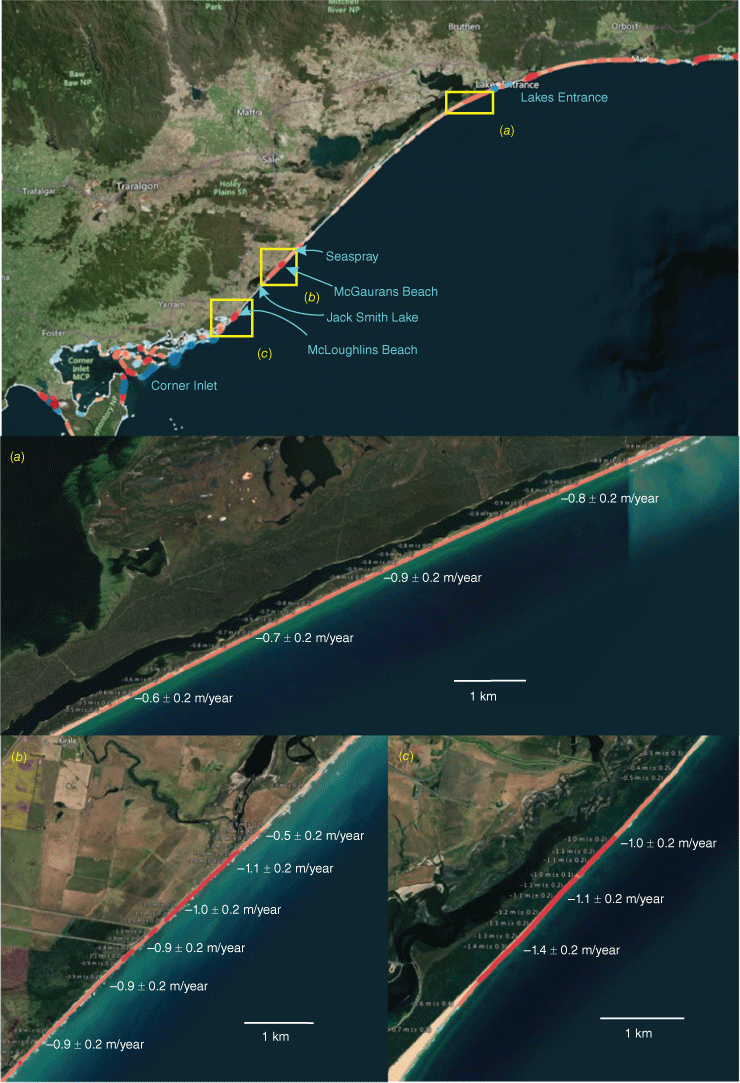
The areas of greatest erosion (>50 m since 1988) are found at the northern end of the Bunga Arm (Fig. 6a), McGaurans (Fig. 6b) and McLoughlins Beach (Fig. 6c), where shoreline retreat (measured at c. MSL elevation) of 30 and 40 m has occurred in the past 35 years. Each of these sites represents a unique geomorphic environment on the Outer Barrier. Along the Bunga Arm, the barrier is almost certainly young (though undated, likely <2000 years in age) and represents the last period of dune-formation in the Outer Barrier complex. At McLoughlins Beach the current shoreline is oriented several degrees away from the foredune ridges indicating a long-term shift in the direction of wave approach (Kennedy et al. 2020). At McGaurans Beach, the dunes are narrow, formed only through the presence of Marram Grass with washover deposits common in the back-dune areas. The beach sequences are also thin with the underlying peat and Tertiary Carbonates exposed in the surf zone (N Rosengren unpubl. data; Kennedy et al. 2020) (Fig. 7a). In addition, low-energy back-barrier swamp deposits have been described by local communities to occur underneath the modern foredune. This area also experiences the highest rate of retreat for Ninety Mile Beach at 1.3 ± 0.2 m/year. It is also observed that the current shoreline is highly dynamic around estuary entrances such as at Jake Smith Lake and Merriman Creek (Seaspray). For the latter, erosion of the foredune in 2007 led to exposure of back-barrier peats deposits on the beachface (Fig. 7b). This indicates the dune sequence here, like McGaurans Beach, is very thin and likely undergoing long-term retreat.
Peat outcrops exposed in 2007 on the rear of the beach at (a) McGaurans Beach (2007) and (b) at Seaspray. Exposure of low-energy back barrier sediments is generally considered an indicator of a transgressive barrier, that is, a beach-dune system undergoing retreat. Photographs by N Rosengren.
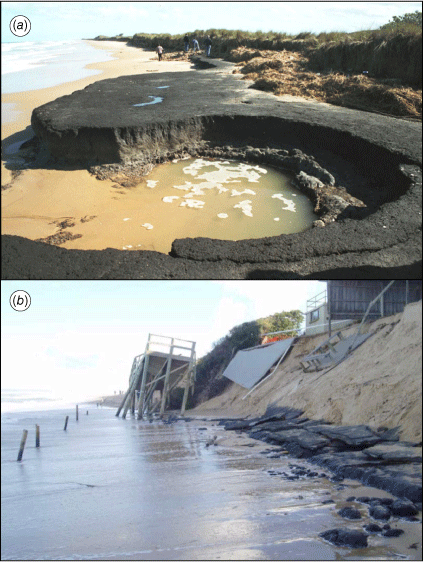
The future trajectory for Ninety Mile Beach and the dune systems of the Outer Barrier are highly uncertain, but almost certainly the entire system is at significant risk of major change including beach-dune erosion and landward retreat of the shoreline. While the broad rates of sea level rise are understood (see McInnes et al. – this volume), the ability of the landforms to respond will depend on the available sediment supply which is poorly understood. Barrier retreat can occur as sediment is transferred offshore (Bruun-style response) or moved landward as material eroded from the dunes is moved inland (Davidson-Arnott-style response) through wave overwash or aeolian processes (Davidson-Arnott 2005). Overwash has been observed in 2007 in the McGaurans Beach ‒ Jake Smith Lake Region, in 1979 at Eastern Beach near Lakes Entrance and potentially around the 1950s along the Bunga Arm (N Rosengren unpubl. data). This is similar to the processes of barrier retreat inferred to have occurred on the barrier islands systems in the eastern USA during the period of rising sea levels during the Holocene (Kraft et al. 1987). The wind-transfer of sand from the dunes inland is also observed along the entire barrier sequence. This is seen in the subsurface sampling and ground penetrating radar surveys of Kennedy et al. (2020), where several metres of sand have been deposited over old land surfaces within the past 200 years. Whether these changes are an indication of the system moving into a net erosional phase or being part of the natural dynamic is uncertain, but it is worrying that all are indicators of retreat rather than progradation of the coast. Detailed numerical modelling using hybrid-numerical models such as ShoreTrans (McCarroll et al. 2021) is seen as a critical next stage in understanding the future trajectories of shoreline movement in the region.
Conclusions and future research directions
The Gippsland Lakes region is home to a diverse range of coastal landforms containing arguably Australia’s most dynamic barrier islands systems dating back to the Penultimate Interglacial Period 220,000 years ago. The beaches are intimately connected to the marine environment, especially the wave climate and sea level, with their response to sea level rise being modulated by sediment supply. The current beach and dune system of Ninety Mile Beach is highly dynamic, while a large proportion of the coast is currently stable, but there are now areas where significant retreat has been observed over the past 35 years. Evidence of wave overwash and inland dune movement is apparent along its entire length, but it is difficult to conclusively assign climate change as a driver of these changes. It is clear, however, that the general trend observed along the current coast is towards erosion with accretion only occurring in isolated areas of significant positive sediment supply such as at the entrance to Corner Inlet and in the dredge spoil areas adjacent to Lakes Entrance.
Despite the geomorphic uniqueness of the region and its socio-economic importance to Victoria and Australia, there remains many fundamental gaps in scientific knowledge. Refinement and expansion of our knowledge of the boundary conditions to the system are critical for future management. This includes quantification of sediment dynamics (e.g. surf zone longshore drift, sediment supply) onto the open-ocean beach as well as into the modern foredunes. The age structure of the Outer Barrier needs to be further refined in order to assess the degree of natural resilience at the scale of individual towns. Recent work has now established the broad principles of change; the next stage is to refine our knowledge to the small scale. That is, the size of individual locations of interest (e.g. midden sites and individual towns) and temporal scales (e.g. individual storms to decades) which will enable both traditional and non-traditional owners to manage the coastal estate for the future. The region has significant natural resilience due to the relatively low level of urban development on the coast. To properly manage human population growth in the current dynamic landform zone and properly ‘care for Country’, a thorough understanding of the current and future landform dynamics is essential.
References
AEMO (2023) Victorian Gas Planning Report: Gas transmission network planning for Victoria. Available at https://aemo.com.au/-/media/files/gas/national_planning_and_forecasting/vgpr/2023/2023-victorian-gas-planning-report.pdf?la=en
Baillie P, Carter P, Grahame J, Zhou J, Mudge N (2019) The Gippsland Basin: reprocessing reveals new opportunities in a ‘mature’ basin. The APPEA Journal 59(1), 394-409.
| Crossref | Google Scholar |
Baker RGV, Haworth RJ, Flood PG (2001) Inter-tidal fixed indicators of former Holocene sea levels in Australia: a summary of sites and a review of methods and models. Quaternary International 83–85, 257-273.
| Crossref | Google Scholar |
Bioregional Assessments (2015) Bioregional Assessment Programme, Definition of the basin, basin extent and regional geological context. Available at https://www.bioregionalassessments.gov.au/assessments/11-context-statement-gippsland-basin-bioregion/1131-geological-structural-framework [retrieved from 12 October 2023]
Bird ECF (1961) The coastal barriers of east Gippsland, Australia. The Geographical Journal 127(4), 460-468.
| Crossref | Google Scholar |
Bird ECF (1965) The evolution of sandy barrier formations on the East Gippsland coast. Proceedings of the Royal Society of Victoria 79, 75-88.
| Google Scholar |
Bishop-Taylor R, Nanson R, Sagar S, Lymburner L (2021) Mapping Australia’s dynamic coastline at mean sea level using three decades of Landsat imagery. Remote Sensing of Environment 267, 112734.
| Crossref | Google Scholar |
Blom WM, Alsop DB (1988) Carbonate mud sedimentation on a temperate shelf: Bass Basin, southeastern Australia. Sedimentary Geology 60(1), 269-280.
| Crossref | Google Scholar |
Brooke BP, Nichol SL, Huang Z, Beaman RJ (2017) Palaeoshorelines on the Australian continental shelf: Morphology, sea-level relationship and applications to environmental management and archaeology. Continental Shelf Research 134, 26-38.
| Crossref | Google Scholar |
Bryant EA, Price DM (1997) Late Pleistocene marine chronology of the Gippsland Lakes region, Australia. Physical Geography 18(4), 318-334.
| Crossref | Google Scholar |
Chappell BW, White AJR, Hine R (1988) Granite provinces and basement terranes in the Lachlan Fold Belt, southeastern Australia. Australian Journal of Earth Sciences 35, 505-521.
| Crossref | Google Scholar |
David B, Arnold LJ, Delannoy J-J, Fresløv J, Urwin C, Petchey F, McDowell MC, Mullett R, Mialanes J, Wood R, Crouch J, Berthet J, Wong VNL, Green H, Hellstrom J (2021) Late survival of megafauna refuted for Cloggs Cave, SE Australia: implications for the Australian Late Pleistocene megafauna extinction debate. Quaternary Science Reviews 253, 106781.
| Crossref | Google Scholar |
Davidson-Arnott RGD (2005) Conceptual model of the effects of sea level rise on sandy coasts. Journal of Coastal Research 216, 1166-1172.
| Crossref | Google Scholar |
DCC (2009) ‘Climate change risks to Australia’s coast: a first pass national assessment’. (Department of Climate Change) Available at https://www.dcceew.gov.au/climate-change/policy/adaptation/publications/climate-change-risks-australias-coasts
DEA (2023) ‘Digital Earth Australia Coastlines.’ (Geoscience Australia) Available at https://maps.dea.ga.gov.au/#share=s-DEACoastlines&playStory=1[retrieved August 2023]
Gardner TW, Webb J, Davis AG, Cassel EJ, Pezzia C, Merritts DJ, Smith B (2006) Late Pleistocene landscape response to climate change: eolian and alluvial fan deposition, Cape Liptrap, southeastern Australia. Quaternary Science Reviews 25(13–14), 1552-1569.
| Crossref | Google Scholar |
Destination Gippsland (2023) Strategies, plans and resources [accessed 2023]. Available at https://www.visitgippsland.com.au/destination-gippsland/about/industry-resources
Hill SM, Bowler JM (1995) Linear dunes at Wilson’s promontory and South-East Gippsland, Victoria: relict landforms from periods of past aridity. Proceedings of the Royal Society of Victoria 107, 73-81.
| Google Scholar |
Hill SM, Joyce EB (1995) Granitic regolith and landscape evolution of Wilsons Promontory, Victoria. Proceedings of the Royal Society of Victoria 107, 1-10.
| Google Scholar |
Hill SM, Ollier CD, Joyce EB (1995) Mesozoic deep weathering and erosion: an example from Wilsons Promontory, Australia. Zeitschrift für Geomorphologie 39, 331-339.
| Crossref | Google Scholar |
Holdgate GR, Wallace MW, Gallagher SJ, Smith AJ, Keene JB, Moore D, Shafik S (2003) Plio‐Pleistocene tectonics and eustasy in the Gippsland Basin, southeast Australia: Evidence from magnetic imagery and marine geological data. Australian Journal of Earth Sciences 50(3), 403-426.
| Crossref | Google Scholar |
Ierodiaconou D, Schimel ACG, Kennedy D, Monk J, Gaylard G, Young M, Diesing M, Rattray A (2018) Combining pixel and object based image analysis of ultra-high resolution multibeam bathymetry and backscatter for habitat mapping in shallow marine waters. Marine Geophysical Research 39(1), 271-288.
| Crossref | Google Scholar |
James EA, Evans PR (1971) The stratigraphy of the offshore Gippsland Basin. The APPEA Journal 11(1), 71-74.
| Crossref | Google Scholar |
James NP, Martindale RC, Malcolm I, Bone Y, Marshall J (2008) Surficial sediments on the continental shelf of Tasmania, Australia. Sedimentary Geology 211(1), 33-52.
| Crossref | Google Scholar |
Kennedy DM, Oliver TSN, Tamura T, Murray-Wallace CV, Thom BG, Rosengren NJ, Ierodiaconou D, Augustinus P, Leach C, Gao J, McSweeney SL, Konlechner T, Woodroffe CD (2020) Holocene evolution of the Ninety Mile Beach sand barrier, Victoria, Australia: The role of sea level, sediment supply and climate. Marine Geology 430, 106366.
| Crossref | Google Scholar |
Kennedy DM, Wong VNL, Jacobsen GE (2021) Holocene infill of the Anglesea Estuary, Victoria: a keep-up estuary in a geologically constrained environment. Australian Journal of Earth Sciences 68, 839-851.
| Crossref | Google Scholar |
Kraft JC, Chrzastowski MJ, Belknap DF, Toscano MA, Fletcher CH (1987) The transgressive barrier-lagoon coast of Delaware: Morphostratigraphy, sedimentary sequences and responses to relative rise in sea level. In ‘Sea-level fluctuation and coastal evolution. Vol. Special Publication No. 41’. (Eds D Nummedal, OH Pilkey, JD Howard) pp. 129–143. (SEPM)
McCarroll RJ, Masselink G, Valiente NG, Scott T, Wiggins M, Kirby J-A, Davidson M (2021) A rules-based shoreface translation and sediment budgeting tool for estimating coastal change: ShoreTrans. Marine Geology 435, 106466.
| Crossref | Google Scholar |
Oliver TSN, Kennedy DM, Tamura T, Murray-Wallace CV, Konlechner TM, Augustinus PC, Woodroffe CD (2018) Interglacial-glacial climatic signatures preserved in a regressive coastal barrier, southeastern Australia. Palaeogeography, Palaeoclimatology, Palaeoecology 501, 124-135.
| Crossref | Google Scholar |
Thom B, Hesp P, Bryant E (1994) Last glacial “coastal” dunes in Eastern Australia and implications for landscape stability during the Last Glacial Maximum. Palaeogeography, Palaeoclimatology, Palaeoecology 111(3), 229-248.
| Crossref | Google Scholar |