Carbon sequestration uncertainty: is grazing-induced soil organic carbon accrual offset by inorganic carbon loss?
Kurt O. Reinhart
A
B
Abstract
In drylands, soil inorganic carbon (SIC) represents the largest terrestrial carbon sink, and observational studies indicate a negative relationship and possible trade-off between SIC (e.g. calcium carbonate [CaCO3]) and soil organic carbon (SOC). Some rangeland managers aim to increase SOC stocks to help decarbonise the atmosphere. Unfortunately, the fate of SIC (and SOC) is uncertain, and grazing-induced SOC accrual may correspond with CaCO3 dissolution, which can produce CO2 emissions. An added concern is whether carbon sequestration schemes focused on SOC stocks need to be discounted for putative CO2 emissions due to CaCO3 dissolution. We used data from a 5-year grazing experiment in the Northern Great Plains of the US. We tested whether grazing management treatments affect SIC, and whether grazing-induced SOC accrual was potentially offset by SIC loss. The experiment had a randomised complete block design and pretreatment data. Response variables were SOC and SIC stocks (0–60 cm depth). Moderate summer grazing (control) is regionally common and treatments that may alter soil stocks included: no grazing, severe summer grazing, moderate autumn grazing, and severe autumn grazing. We also tested for a negative relationship between SOC and SIC across all soil cores (n = 244). Severe grazing (summer and autumn) increased SOC by 0.83 and 0.88 kg × m−2 relative to moderate summer grazing, respectively. However, no treatments affected SIC. Conversely, we found an overall weak but significant (r2 = 0.04, P = 0.002), near one-to-one negative relationship between SIC and SOC stocks of soil cores. Our findings suggest severe grazing can increase SOC without affecting SIC, at least over the short term (5 years). This finding mirrors results from an observational study elsewhere in the Northern Great Plains that also failed to detect grazing effects on SIC. Long-term grazing experiments (>5 years) with pretreatment data may be required to detect grazing effects on SIC.
Keywords: carbon dioxide removal, carbon ranching, dryland, grassland, livestock grazing, natural climate solution, soil carbon sequestration, soil inorganic carbon, soil organic carbon.
Introduction
Researchers are interested in identifying good grazing and soil organic carbon (SOC) outcomes to help decarbonise the atmosphere (Bai and Cotrufo 2022; Reinhart et al. 2022b). Some in the enterprise of ‘regenerative agriculture’ have claimed that good grazing, conducted at scale, is a simple remedy to prevent climate change and decarbonise the atmosphere (Savory 2013; Moyer et al. 2020). Researchers like Stanley et al. (2024) echoed these claims and stated, ‘[good] grazing (sic) management is… scalable across millions of globally grazed acres, low cost, and has high technical potential’ to decarbonise the atmosphere. Others are less optimistic (Hawkins et al. 2022; Reinhart et al. 2022b). Henderson et al. (2015) estimated the achievable SOC sequestration potential of scaled good grazing in global grazing lands is 148–699 megatons of atmospheric CO2 × year−1, which according to United Nations Environment Program (2022) is <2% of CO2 emissions (37,900 megatons) in 2021. Although a <2% reduction in annual anthropogenic emissions is helpful, claims that scaled good grazing can stabilise Earth’s CO2 concentration are overblown and reinforce the dangerous narrative that scaled good grazing can sequester a majority of CO2 from annual fossil fuel emissions (Hawkins et al. 2022).
Soil inorganic carbon (SIC) is another major terrestrial carbon sink, especially in drylands such as grasslands, shrublands, and savannahs (Zamanian et al. 2016, 2021). SIC is synonymous with carbonate carbon (e.g. calcium carbonate [CaCO3]). Even though calcareous soils have appreciable SIC (Fig. 1) and cover >30% of Earth’s land surface (Chen and Barak 1982), 96% of research on soil carbon sequestration overlooks SIC (Raza et al. 2024). Similarly, carbon dioxide removal schemes of voluntary carbon markets often require monitoring SOC but not SIC (or total soil carbon) (Pudasaini et al. 2024). A concern is whether SOC myopia may inflate estimates of carbon sequestration by not discounting for dissolution of SIC and other sources of CO2 equivalent emissions (e.g. Hawkins et al. 2022; Wang et al. 2023a).
Average (±95% confidence interval) root and soil (rock- and root-free fine soil) carbon stock of a northern mixed prairie dominated by C3 graminoids in eastern Montana, USA. Stocks were derived from 120 cores collected pretreatment (2013) by Reinhart et al. (2022a). Root carbon was estimated (root biomass × 0.53 [±0.002]), and the conversion factor was derived from a nearby experiment (Haferkamp and MacNeil 2004).
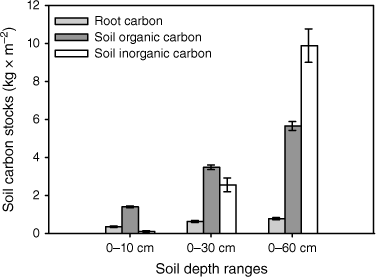
Complex processes govern SIC in dryland systems (Monger and Martinez-Rios 2001; Schlesinger 2017; Ding et al. 2022; Zhong and Zhou 2022), and a number of factors can influence their concentration. For example, carbonates are likely to precipitate where water is ephemeral and in aqueous areas around roots (Monger and Martinez-Rios 2001). SIC stocks can also be affected by plant invasions (Wilsey et al. 2020) and land management (Denef et al. 2008; Wang et al. 2015, 2023b; Kim et al. 2020; Zamanian et al. 2021; Ball et al. 2023). Dryland irrigation may increase soil calcium levels, which may increase SIC stocks (Monger and Martinez-Rios 2001; Sanderman 2012; Ball et al. 2023).
In non-irrigated rangelands, observational studies report a negative correlation (and possible trade-off) between SOC and SIC stocks (Pan and Guo 2000; Deane McKenna et al. 2022) but the biogeochemical drivers underlying this negative relationship remain unclear. If the supposed SOC–SIC trade-off exists, then grazing practices that increase SOC will decrease SIC, which may have unintended impacts on carbon sequestration. In drylands, the best grazing-SOC outcome may degrade SIC stocks by a biogeochemical pathway (i.e. complete [or congruent] calcium carbonate dissolution) that is a potential source of CO2 (Monger and Martinez-Rios 2001). Good grazing SOC outcomes may increase dissolution of CaCO3 by increasing natural acidification processes such as root and microbial respiration, carboxylic acids in root exudates, and decomposition of organic matter (Sanderman 2012; Zhong and Zhou 2022). Livestock management may also impact SIC by affecting water infiltration (Gifford and Hawkins 1978), plant biomass and plant species composition (Monger and Martinez-Rios 2001; Sanderman 2012; Deane McKenna et al. 2022), and microbial community composition and functioning (Liu et al. 2020).
Grazing experiments indicate varying effects of grazing on SIC. An unreplicated and long-term grazing study (without pre-treatment data) suggested that, compared to no grazing, moderate and severe grazing can increase SIC in the Central Great Plains of North America (Reeder et al. 2004). In other drylands, SIC has shown evidence of sometimes decreasing (Niu et al. 2011) and other times being unaffected (Cao et al. 2013) by grazing. Our overall understanding of grazing and soil carbon is limited by the quantity (McSherry and Ritchie 2013) and quality of data (e.g. little replication, no pretreatment data, incomplete description of grazing pattern). Data quality has likely suffered because grazing experiments require a lot of land, meaningful scale introduces heterogeneity among replicates, and preexisting differences are rarely taken into account (Reinhart et al. 2022b).
We previously reported SOC responses to 5 years of bovine grazing treatments (Reinhart et al. 2022a) and that grazing-induced shifts in vegetation were not predictive of SOC accrual (Reinhart et al. 2024) in a grassland in the Northern Great Plains of North America. Here, we tested whether grazing practices that generate the greatest SOC accrual are counterbalanced by SIC depletion. We hypothesised SOC accrual processes will increase acidification processes (e.g. increased soil respiration and decomposition of organic matter) that may degrade SIC. Specifically, we hypothesised paddocks with severe summer and autumn grazing would have more SOC (Reinhart et al. 2024) and less SIC than controls (moderate summer grazing). Across all soil cores, we also tested for a negative relationship between SOC and SIC stocks (Deane McKenna et al. 2022) because the two are indicators of (but inversely associated with) soil depth and development.
Materials and methods
Study area
This study was conducted at the Fort Keogh Livestock and Range Research Laboratory (105°57′59.1″W, 46°24′24.8″N, Montana, USA). The study site was a calcareous grassland (Fig. 1) with northern mixed prairie, a Pinehill loam soil (fine, smectitic, frigid Aridic Haplustalfs, or Alfisols), and 4% south-west slope. This temperate, semiarid location has a mean annual temperature and precipitation of 8.8°C and 327 mm, respectively.
Experimental design
Details of the grazing experiment were described previously (Reinhart et al. 2022a, 2024). Moderate and severe summer grazing treatments (June/July) and moderate and severe autumn grazing treatments (October/November) were applied to paddocks (60 × 30 m) arranged in a randomised complete block design with five replications (4 × 5 = 20 paddocks) and blocks aligned perpendicular to the slope. Summer grazing occurred during late June, and autumn grazing during late October or early November, after a killing frost (−2°C). Senescence of the perennial species is generally initiated by summer heat and dryness, and autumn precipitation is insufficient to stimulate new autumn growth. In contrast, annual bromes (Japanese brome and cheatgrass) germinate primarily during late summer and early autumn and may remain active through autumn. Typically, the late June period used for the summer grazing treatment is characterised as being 2–3 weeks before peak plant biomass, with active plant growth and high forage quality. Stocking rates varied among years to target 600 and 300 kg × ha−1 post-grazing residual standing crop for moderate and severe grazing, respectively. Under average forage production, the residual mass levels correspond to stocking rates of 1 and 1.5 animal unit month (AUM) × ha−1 and utilisation levels of 50 and 75%, respectively. Prescribed residual standing crop was typically achieved with 2–5 days of cattle (i.e. cow and cow-calf pairs [Bos taurus]) grazing per paddock. Grazing treatments were applied annually from 2013 to 2018. Additionally, five plots (4.8 × 4.8-m) were established 20–48 m from the western edge of the grazing treatment paddocks and were rested from grazing (i.e. ungrazed) from 2013 to 2018.
Soil sampling and analysis
With a percussion corer, we cored soil from 5 of 25 possible points of a microsite (4 × 4-m grid) in each paddock and exclosure pre- (2013) and post-treatment (2018) (Reinhart et al. 2022a). Bulk density, root biomass, SIC, and SOC were measured in three depth increments (0–10, 10–30, and 30–60 cm) of each core (Reinhart et al. 2024). Soil samples were broken up, air-dried to constant weight, and weighed. Then a 5-g subsample from each soil sample was further dried (105°C, 24 h) then weighed to determine % dry matter. We used a sieving approach to separate fine soil (<2 mm), rocks, and roots as described by Reinhart and Vermeire (2017). To measure root biomass, roots were dried to constant mass (105°C, 24 h), weighed, combusted at 550°C for 16 h, and reweighed to determine the % ash. During the sieving process, rocks (>2 mm) were also collected by hand and weighed.
To determine SOC stocks of fine soil, we measured each core increment’s dry weight and total carbon and SIC concentrations using methods of Reinhart and Vermeire (2017). In brief, from each 10-g root-free subsample, we removed and ground 1.5 g of a soil sample on a roller-milling device (Arnold and Schepers 2004). The SOC concentration (%) of ground samples was determined by measuring total carbon concentration with the dry combustion method (Flash 2000 Series Carbon-Nitrogen Thermo Combustion Elemental Analysis system, Thermo Scientific, MA, USA) and then subtracting the SIC, which represents the carbonate carbon. The SIC content of ground samples was determined by the modified pressure-calcimeter method in 2013 samples (Sherrod et al. 2002), and a modification of this method was used to assay the 2018 samples (Stetson and Osborne 2015). The two approaches give very similar estimates, and the approach we used in 2018 is less labour-intensive (Stetson and Osborne 2015). Total carbon and SIC (rock- and root-free fine soil) were adjusted for % dry matter (105°C, 24 h). Soil dry weight and SIC concentration (per core depth increment) were used to estimate SIC stocks (kg × m−2) of the 0–60 cm depth increment following routine data conversion and summation steps (FAO 2019). The same approach was used to estimate SOC stocks. This resulted in 250 SIC (or SOC) stock estimates of rock- and root-free fine soil (5 grazing treatments × 5 treatment replications × 5 cores per experimental unit × 2 years = 250). The full soil dataset is available (Reinhart et al. 2022c).
Statistical analysis
We analysed the effects of grazing treatments on SIC and SOC jointly with a multivariate regression model (Reinhart et al. 2024). Data were averaged (per paddock per year) before analysis (Hurlbert 1984). The model had terms for block, treatment, and pre-treatment SIC and SOC and was fit using a Fortran program (Intel Corporation 2023). All inferences were based on 95% confidence intervals comparing other treatments to the typical summer moderate grazing treatment. We also tested for a negative relationship between SOC and SIC stocks of soil cores using simple linear regression (R Development Core Team 2020). We used Rosner’s test to identify outliers in EnvStats, and we removed 0 SIC and 6 SOC outliers prior to analysis.
Results
At our northern mixed-grass prairie study site, SIC (i.e. carbonate carbon) was the largest fraction of soil carbon to 60 cm depth (Fig. 1). We found no evidence grazing influenced SIC (and soil total carbon) (Fig. 2). Conversely, severe grazing (summer, autumn) increased SOC by 0.83 and 0.88 kg × m−2 relative to moderate summer grazing, respectively (Fig. 2). We found a weak but significant, near one-to-one negative relationship between SIC and SOC stocks of all cores (Fig. 3) suggesting a possible trade-off between SOC and SIC.
Effect of grazing treatments (average ± 95% confidence interval) on (a) soil organic carbon (SOC) and (b) soil inorganic carbon (SIC) relative to the common practice of moderate summer grazing. Confidence intervals that fail to overlap zero indicate significant differences (P < 0.05). Inset shows results for soil total carbon, and treatment order follows main panels.
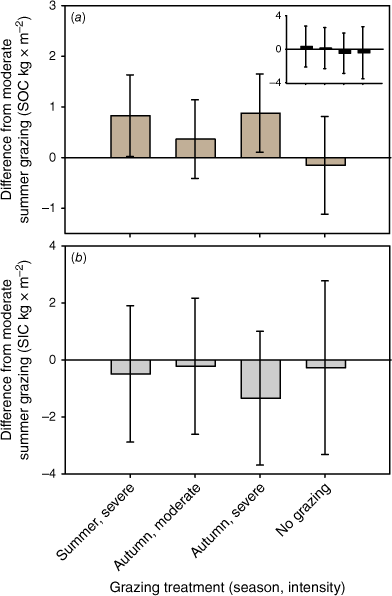
Discussion
We found no effect of grazing treatments on SIC and no evidence that grazing-induced SOC accrual was associated with SIC depletion. Our findings support the interpretation that SIC stocks are stable and unaffected by grazing and grazing-induced SOC accrual over 5 years. A natural experiment also investigated effects of grazing on SIC in the Northern Great Plains and reported no effect of grazing (Bork et al. 2020). Conversely, an unreplicated study has suggested that, compared to no grazing, 56 years of moderate and severe grazing can increase SIC in the Central Great Plains (Reeder et al. 2004). If the grazing treatment period is sufficiently long, then grazing treatments may appreciably affect SIC stocks.
In other drylands, grazing has sometimes reduced SIC (Niu et al. 2011) or not affected SIC (Cao et al. 2013). Our approach and those used by others serve as a first approximation of grazing effects on SIC, SOC–SIC interactions, and whether SIC is an active source (or sink) of atmospheric CO2. Expanded soil measurements (e.g. bicarbonate content, calcium content, pH) are likely necessary to interpret biogeochemical reactions (i.e. carbonate precipitation, dissolution, and re-precipitation reactions). It is worth noting that there are three types of carbonate dissolution pathways and only ‘complete (or congruent) dissolution’ is a putative source of CO2 emissions (Monger and Martinez-Rios 2001). Other dissolution pathways may be associated with SIC losses in specific soil depth increments (e.g. carbonates in surface soils dissolve and reprecipitate deeper in soil horizon) but may be neutral in terms of CO2 emission.
Some have questioned (1) whether the soil carbon sequestration of grazing lands is sufficient for the entire grazing management system to be a net carbon sink (Wang et al. 2023a), and (2) validity of the voluntary carbon market (Davidson 2022; Reinhart et al. 2022b). As we previously reported (Reinhart et al. 2022a), severe grazing in summer and autumn increased SOC accrual relative to the control (moderate summer grazing) over 5 years. Another study in the same ecoregion measured net sources and sinks for greenhouse gases of a long-term (unreplicated) grazing experiment and reported areas with heavy and moderate grazing were net carbon sinks (Liebig et al. 2010).
Similar to our findings, Deane McKenna et al. (2022) and Pan and Guo (2000) identified a negative relationship between SOC and SIC in drylands. Others have noted that rainfall and plant composition treatments may have opposite and offsetting effects on SIC and SOC (Monger and Martinez-Rios 2001; Huber et al. 2019), which may partly explain the observed negative SIC–SOC relationship. Grazing practices may also alter SIC by increasing (or decreasing) natural acidification and carbonate dissolution processes, such as root and microbial respiration and decomposition of organic matter, which were not measured in our study (Wilsey et al. 2020). Alternatively, grazing may alter calcium carbonate formation processes. For example, ungulate grazers may disrupt microbial-induced calcium carbonate precipitation (Mitchell and Ferris 2006; Chu et al. 2012; Wen et al. 2019). In drylands, plant root exudation (e.g. carboxylate) used to solubilise calcium-bound phosphorus may also affect calcium carbonate formation (Zhong and Zhou 2022). Grazing may alter root-mining activity, which may increase (or decrease) calcium carbonate formation. Very few grazing studies have assessed soil carbon accrual and to our knowledge no prior study has tested for grazing effects on SOC and SIC jointly.
Carbon dioxide removal (CDR) schemes and related research tend to focus on SOC and ignore SIC (Pudasaini et al. 2024; Raza et al. 2024). Carbon ranching is one type of natural CDR scheme (Pudasaini et al. 2024) where some livestock ranchers agree to alter their grazing management with the aim of increasing SOC (National Academies of Sciences, Engineering, and Medicine 2019; Reinhart et al. 2022b). Because SIC and SOC are likely to have inverse relationships in drylands, measurement of total carbon may be preferred for estimation of soil carbon sequestration (Fig. 2) (Huber et al. 2019). Despite such improvements, scaled good grazing is likely to have only a small effect (e.g. <2% of 2021 emissions) on annual anthropogenic greenhouse gas emissions.
Declaration of funding
Funded by USDA appropriated funds (CRIS # 5434-21630-003-00D) and a Climate, Food and Farming Network-Global Research Alliance Development Scholarship (CLIFF-GRADS). This research was supported by Mitigate+: Research for Low Emissions Food Systems and the Global Research Alliance on Agricultural Greenhouse Gases (GRA) through their CLIFF-GRADS program. Funding for Mitigate+ comes from the Consortium of International Agricultural Research Centers Trust (CGIAR) Fund. Mention of trade names or commercial products in this publication is solely for the purpose of providing specific information and does not imply recommendation or endorsement by the USDA. USDA is an equal opportunity provider and employer.
References
Arnold SL, Schepers JS (2004) A simple roller-mill grinding procedure for plant and soil samples. Communications in Soil Science and Plant Analysis 35(3–4), 537-545.
| Crossref | Google Scholar |
Bai Y, Cotrufo MF (2022) Grassland soil carbon sequestration: current understanding, challenges, and solutions. Science 377(6606), 603-608.
| Crossref | Google Scholar | PubMed |
Ball KR, Malik AA, Muscarella C, Blankinship JC (2023) Irrigation alters biogeochemical processes to increase both inorganic and organic carbon in arid-calcic cropland soils. Soil Biology and Biochemistry 187, 109189.
| Crossref | Google Scholar |
Bork EW, Raatz LL, Carlyle CN, Hewins DB, Thompson KA (2020) Soil carbon increases with long-term cattle stocking in northern temperate grasslands. Soil Use and Management 36(3), 387-399.
| Crossref | Google Scholar |
Cao J, Wang X, Sun X, Zhang L, Tian Y (2013) Effects of grazing intensity on soil labile organic carbon fractions in a desert steppe area in Inner Mongolia. SpringerPlus 2(Suppl 1), S1.
| Crossref | Google Scholar | PubMed |
Chu J, Stabnikov V, Ivanov V (2012) Microbially induced calcium carbonate precipitation on surface or in the bulk of soil. Geomicrobiology Journal 29(6), 544-549.
| Crossref | Google Scholar |
Davidson EA (2022) Is the transactional carbon credit tail wagging the virtuous soil organic matter dog? Biogeochemistry 161(1), 1-8.
| Crossref | Google Scholar |
Deane McKenna M, Grams SE, Barasha M, Antoninka AJ, Johnson NC (2022) Organic and inorganic soil carbon in a semi-arid rangeland is primarily related to abiotic factors and not livestock grazing. Geoderma 419, 115844.
| Crossref | Google Scholar |
Denef K, Stewart CE, Brenner J, Paustian K (2008) Does long-term center-pivot irrigation increase soil carbon stocks in semi-arid agro-ecosystems? Geoderma 145(1), 121-129.
| Crossref | Google Scholar |
Ding W, Cong W-F, Lambers H (2022) Response to Zhong and Zhou: P-acquisition strategies and total soil C sequestration. Trends in Ecology & Evolution 37(1), 14-15.
| Crossref | Google Scholar | PubMed |
FAO (2019) Measuring and modelling soil carbon stocks and stock changes in livestock production systems: Guidelines for assessment (Version 1). Livestock Environmental Assessment and Performance (LEAP) Partnership. 170 pp. (Food and Agriculture Organization of the United Nations) Available at https://openknowledge.fao.org/server/api/core/bitstreams/3573cc7c-f8b0-42cc-bead-ea56c502647e/conten
Gifford GF, Hawkins RH (1978) Hydrologic impact of grazing on infiltration: a critical review. Water Resources Research 14(2), 305-313.
| Crossref | Google Scholar |
Haferkamp MR, MacNeil MD (2004) Grazing effects on carbon dynamics in the northern mixed-grass prairie. Earth and Environmental Science 33(1), S462-S474.
| Crossref | Google Scholar |
Hawkins HJ, Venter ZS, Cramer MD (2022) A holistic view of Holistic Management: what do farm-scale, carbon, and social studies tell us? Agriculture, Ecosystems & Environment 323, 107702.
| Crossref | Google Scholar |
Henderson BB, Gerber PJ, Hilinski TE, Falcucci A, Ojima DS, Salvatore M, Conant RT (2015) Greenhouse gas mitigation potential of the world’s grazing lands: modeling soil carbon and nitrogen fluxes of mitigation practices. Agriculture, Ecosystems & Environment 207, 91-100.
| Crossref | Google Scholar |
Huber DP, Lohse KA, Commendador A, Joy S, Aho K, Finney B, Germino MJ (2019) Vegetation and precipitation shifts interact to alter organic and inorganic carbon storage in cold desert soils. Ecosphere 10(3), e02655.
| Crossref | Google Scholar |
Hurlbert SH (1984) Pseudoreplication and the design of ecological field experiments. Ecological Monographs 54(2), 187-211.
| Crossref | Google Scholar |
Intel Corporation (2023) Intel Fortran Compiler for one API. 2023.1 edn. (Santa Clara, CA) Available at https://www.intel.com/content/www/us/en/developer/tools/oneapi/fortran-compiler.html#gs.clgbcc
Kim JH, Jobbágy EG, Richter DD, Trumbore SE, Jackson RB (2020) Agricultural acceleration of soil carbonate weathering. Global Change Biology 26(10), 5988-6002.
| Crossref | Google Scholar | PubMed |
Liebig MA, Gross JR, Kronberg SL, Phillips RL (2010) Grazing management contributions to net global warming potential: a long-term evaluation in the Northern Great Plains. Journal of Environmental Quality 39(3), 799-809.
| Crossref | Google Scholar | PubMed |
Liu Z, Sun Y, Zhang Y, Qin S, Sun Y, Mao H, Miao L (2020) Desert soil sequesters atmospheric CO2 by microbial mineral formation. Geoderma 361, 114104.
| Crossref | Google Scholar |
McSherry ME, Ritchie ME (2013) Effects of grazing on grassland soil carbon: a global review. Global Change Biology 19(5), 1347-1357.
| Crossref | Google Scholar | PubMed |
Mitchell AC, Ferris FG (2006) The influence of Bacillus pasteurii on the nucleation and growth of calcium carbonate. Geomicrobiology Journal 23(3–4), 213-226.
| Crossref | Google Scholar |
Moyer J, Smith A, Rui Y, Hayden J (2020) Regenerative agriculture and the soil carbon solution. Report. (Kutztown, PA) Available at https://rodaleinstitute.org/wp-content/uploads/Rodale-Soil-Carbon-White-Paper_v11-compressed.pdf
Niu D, Hall SJ, Fu H, Kang J, Qin Y, Elser JJ (2011) Grazing exclusion alters ecosystem carbon pools in Alxa desert steppe. New Zealand Journal of Agricultural Research 54(3), 127-142.
| Crossref | Google Scholar |
Pudasaini K, Rolfe J, Bhattarai T, Walsh K (2024) Comparison of major carbon offset standards for soil carbon projects in Australian grazing lands. Carbon Management 15(1), 2298725.
| Crossref | Google Scholar |
Raza S, Irshad A, Margenot A, Zamanian K, Li N, Ullah S, Mehmood K, Ajmal Khan M, Siddique N, Zhou J, Mooney SJ, Kurganova I, Zhao X, Kuzyakov Y (2024) Inorganic carbon is overlooked in global soil carbon research: a bibliometric analysis. Geoderma 443, 116831.
| Crossref | Google Scholar | PubMed |
Reeder JD, Schuman GE, Morgan JA, LeCain DR (2004) Response of organic and inorganic carbon and nitrogen to long-term grazing of the shortgrass steppe. Environmental Management 33(4), 485-495.
| Crossref | Google Scholar | PubMed |
Reinhart KO, Vermeire LT (2017) Power and limitation of soil properties as predictors of variation in peak plant biomass in a northern mixed-grass prairie. Ecological Indicators 80, 268-274.
| Crossref | Google Scholar |
Reinhart KO, Rinella MJ, Sanni Worogo HS, Waterman RC, Vermeire LT (2022a) Lessons from a next generation carbon ranching experiment. Geoderma 425(1), 116061.
| Crossref | Google Scholar |
Reinhart KO, Sanni Worogo HS, Rinella MJ (2022b) Ruminating on the science of carbon ranching. Journal of Applied Ecology 59(3), 642-648.
| Crossref | Google Scholar |
Reinhart KO, Rinella MJ, Waterman RC, Sanni Worogo HS, Vermeire LT (2024) Above-ground plant properties are not leading indicators of grazing-induced soil carbon accrual in the Northern Great Plains. Ecological Indicators 158, 111509.
| Crossref | Google Scholar |
Sanderman J (2012) Can management induced changes in the carbonate system drive soil carbon sequestration? A review with particular focus on Australia. Agriculture, Ecosystems & Environment 155, 70-77.
| Crossref | Google Scholar |
Savory A (2013) How to green the world’s deserts and reverse climate change. In ‘Technology, Entertainment, Design (TED)’, Long Beach, CA, USA. Available at https://savory.global/science_library/ted/
Schlesinger WH (2017) An evaluation of abiotic carbon sinks in deserts. Global Change Biology 23(1), 25-27.
| Crossref | Google Scholar | PubMed |
Sherrod LA, Dunn G, Peterson GA, Kolberg RL (2002) Inorganic carbon analysis by modified pressure-calcimeter method. Soil Science Society of America Journal 66, 299-305.
| Crossref | Google Scholar |
Stanley PL, Wilson C, Patterson E, Machmuller MB, Cotrufo MF (2024) Ruminating on soil carbon: applying current understanding to inform grazing management. Global Change Biology 30(3), e17223.
| Crossref | Google Scholar | PubMed |
Stetson SJ, Osborne SL (2015) Further modification of pressure-calcimeter method for soil inorganic carbon analysis. Communications in Soil Science and Plant Analysis 46(17), 2162-2167.
| Crossref | Google Scholar |
United Nations Environment Program (2022) Emissions gap report 2022: The closing window-Climate crisis calls for rapid transformation of societies. Report number 9280738127. (Nairobi, Kenya) Available at https://www.unep.org/emissions-gap-report-2022
Wang X, Wang J, Xu M, Zhang W, Fan T, Zhang J (2015) Carbon accumulation in arid croplands of northwest China: pedogenic carbonate exceeding organic carbon. Scientific Reports 5(1), 11439.
| Crossref | Google Scholar | PubMed |
Wang Y, de Boer IJM, Persson UM, Ripoll-Bosch R, Cederberg C, Gerber PJ, Smith P, van Middelaar CE (2023a) Risk to rely on soil carbon sequestration to offset global ruminant emissions. Nature Communications 14(1), 7625.
| Crossref | Google Scholar | PubMed |
Wang Z, Wang Y, Feng W (2023b) Change rates of soil inorganic carbon vary with depth and duration after land conversion across drylands in North China. Chemosphere 325, 138319.
| Crossref | Google Scholar | PubMed |
Wen K, Li L, Zhang R, Li Y, Amini F (2019) Micro-scale analysis of microbial-induced calcite precipitation in sandy soil through SEM/FIB imaging. Microscopy Today 27(1), 24-29.
| Crossref | Google Scholar |
Wilsey B, Xu X, Polley HW, Hofmockel K, Hall SJ (2020) Lower soil carbon stocks in exotic vs. native grasslands are driven by carbonate losses. Ecology 101(7), e03039.
| Crossref | Google Scholar | PubMed |
Zamanian K, Pustovoytov K, Kuzyakov Y (2016) Pedogenic carbonates: forms and formation processes. Earth-Science Reviews 157, 1-17.
| Crossref | Google Scholar |
Zamanian K, Zhou J, Kuzyakov Y (2021) Soil carbonates: the unaccounted, irrecoverable carbon source. Geoderma 384, 114817.
| Crossref | Google Scholar |
Zhong H, Zhou J (2022) Response to Ding et al.: Carboxylate exudation promotes C sequestration in dryland ecosystems. Trends in Ecology & Evolution 37(1), 12-13.
| Crossref | Google Scholar | PubMed |