Managing grazing to increase ground cover in rangelands: using remote sensing to detect change
Sarah E. McDonald A * , Aaron T. Simmons B C , Steven Harden D , Susan E. Orgill E , Juan Guerschman F and Craig Strong GA
B
C
D
E
F
G
Abstract
Practices that improve the quantity, composition, and persistence of ground cover can contribute to a range of ecosystem services that support agricultural production, regulate climate, reduce erosion and support nutrient cycling. In rangeland grazing systems, incorporating periods of rest and matching stocking rates to feed availability is commonly used with the goal of improving land condition and productivity at a property scale. Understanding and quantifying differences in ground cover associated with changes to grazing management can provide livestock producers with greater confidence in the outcomes associated with their management. It can also demonstrate their nature positive activities which may be valued in emerging markets. This study sought to quantify any changes in ground cover resulting from changed grazing management (strategically managing the timing, intensity and duration of grazing events to maintain or improve land condition) across seven mixed grazing (cattle, sheep and/or goats) study sites in the semi-arid rangelands of western New South Wales, Australia. Time-series estimates of ground cover derived from Landsat imagery for each study site were compared with biophysically similar regional benchmark areas as controls. Overall, ground cover was found to have increased significantly (2–7%) following change in grazing management at four of the seven study sites, relative to control benchmark areas. It was apparent different land units varied in their response to the management change, and that the preceding 12 months rainfall (such as wet, intermediate or dry rainfall years) did not have a consistently significant effect on the relative response. Results of this study highlight that improvements in ground cover and land condition may be achieved through changes to grazing management, but also that there are complexities in both achieving and measuring any change. This study demonstrates the practical application of remotely sensed cover data and dynamic regional comparison techniques to document environmental outcomes at the property scale from grazing management in low input, extensive rangeland grazing systems.
Keywords: ecosystem services, grazing management, grazing system, livestock management, rangeland management, rotational grazing, satellite imagery, stocking rate.
Introduction
With the introduction and expansion of pastoralism throughout the Australian rangelands, significant landscape degradation occurred, primarily a result of overgrazing by both livestock and introduced rabbits, in combination with extended periods of drought (Greene and Tongway 1989; McKeon 2004). Degradation includes soil erosion (wind, sheet and gully), growth of invasive native shrubs, and an overall decline in perennial grass content and pasture quality. This degradation reduced the productive capacity of grazing land and the provision and support of important ecosystem services including air and water quality, biodiversity, and climate mitigation (Pickup 1996; MacLeod and McIvor 2006; Stafford Smith et al. 2007). Furthermore, recent research indicates that in the absence of widespread adoption of land management practices that promote soil carbon sequestration and retention in the rangelands, rangelands will become a considerable source of greenhouse gas emissions, and landscape function will continue to be significantly and irreversibly constrained in the future (Chappell et al. 2019; Viscarra Rossel et al. 2024).
Given rangelands occupy 81% of Australia’s land mass and support approximately 30% of Australia’s livestock production (Bell et al. 2014; Foran et al. 2019), improving grazing management and reversing degradation in these areas can have important economic and ecological impacts (Bell et al. 2014). In recent years, there has been a shift in societal preferences, public policy and industry values towards multifunctional landscapes that deliver agricultural productivity, alongside environmental and societal outcomes (Briske et al. 2020; Witt et al. 2021). For example, environmental stewardship targets are now embedded in many industry frameworks, including the Australian Beef Sustainability Framework (ABSF 2024), Sheep Sustainability Framework (SSF 2024), National Farmers Federation 2030 roadmap (NFF 2018), and are also an essential component of Australia’s commitment to meeting the United Nations Sustainable Development Goals (UN SDGs).
To meet national and global sustainability goals and maximise access to emerging ecosystem service markets, there remains an ongoing need to identify management practices that can achieve improvements in land condition while maintaining agricultural production. Improving management of livestock grazing by matching stocking rates to feed availability (carrying capacity), providing periods of rest and recovery from grazing, and adapting grazing systems to seasonal conditions has been demonstrated to increase ground cover, productivity and plant diversity, and support ecosystem service provision including carbon sequestration in rangelands throughout the world (Byrnes et al. 2018; di Virgilio et al. 2019; McDonald et al. 2019a; Teague and Kreuter 2020). However, in southern Australian semi-arid rangelands, there have been only a small number of quantitative studies assessing the impact of grazing management systems, and in particular, few that have assessed multi-paddock grazing systems (Hacker and McDonald 2021). Those that have been undertaken were primarily one-off, fence line comparison studies across relatively small scales (e.g. Alemseged et al. 2011; Waters et al. 2017; McDonald et al. 2018, 2019b), the limitations of which are well recognised (Landsberg et al. 2003; Teague and Barnes 2017). To meet industry goals and demonstrate environmental stewardship, a robust, scalable, repeatable and management-sensitive method is required (Briske et al. 2020).
Ground cover (in the context of this paper, defined as the cover of the soil surface by living and non-living organic material including plants, litter, cryptogam, coarse woody debris and dung, for example) is an important indicator of land condition in the arid and semi-arid Australian rangelands (Beutel et al. 2021), and is correlated with many other indicators of ecosystem condition including biodiversity (Ludwig et al. 2004; Ward and Kutt 2009; McDonald et al. 2018), higher soil carbon stocks (Orgill et al. 2017; Wang et al. 2018; Gray et al. 2022), reduced runoff and water erosion (Greene et al. 1994), reduced wind erosion (Leys et al. 2023), landscape function (Tongway and Hindley 2004) and productivity (Pickup 1996). However, detecting changes in ground cover associated with changed management can be challenging because of the large scale of rangeland grazing properties, low rainfall and variable climate, the variability in soil type, vegetation and grazing patterns across management areas, and slow timeframes over which changes occur (Bastin et al. 2024). Remote sensing has the potential to overcome some of these challenges and to provide cost-effective, objective data across larger spatial and temporal scales (Beutel et al. 2019; Retallack et al. 2023), thus supporting producer decision making and enabling adaptive management by monitoring key indicators of landscape condition (Jansen et al. 2022).
In monitoring the impact of management change on ground cover, particularly in rangeland environments where climate variability is a primary driver of change (Pickup 1996), it is important to separate the impact of management from landscape and climatic drivers (Bastin et al. 2012; Barnetson et al. 2017). One approach to achieve this is by comparing ‘treatment’ areas with dynamic benchmark areas (‘controls’) of comparable land types, and that are subject to similar climatic factors (e.g. Bastin et al. 2012; Zhang and Carter 2018; Donohue et al. 2022). This approach has been successfully used to demonstrate improvement in ground cover from management change (e.g. Beutel et al. 2021; Bartley et al. 2023; Bastin et al. 2024). However, no studies have examined effects of intensive multi-paddock grazing management with flexible stocking rates matched to carrying capacity at property-scale, across multiple properties and using this dynamic benchmark remote sensing approach in southern Australian rangelands.
This study aimed to quantify any change in ground cover and assess the potential to achieve environmental outcomes through changed grazing management (adoption of multi-paddock grazing and flexible stocking rates matched to carrying capacity) in NSW semi-arid rangelands. In addition, the study aimed to identify which land types and in what preceding rainfall conditions changes in ground cover were most apparent.
Methods
Study location
This study was undertaken on seven extensive grazing properties (7100–17,000 ha) in NSW semi-arid rangelands in three key bioregions – the Darling Riverine Floodplain, Cobar Peneplain and Murray Darling Depression (Fig. 1, IBRA7 2012). Average annual rainfall across the region ranges from 500 mm in the east to less than 200 mm in the west (BOM 2024). Average temperatures across the region range from 24—33°C in January to 9–15°C in July (BOM 2024). Vegetation across the seven properties is predominantly native open grassland, chenopod shrubland, and Acacia, Casuarina, Eucalyptus or Callitris woodland, with some clearing of invasive native shrubs and annual cropping (winter cereal and fodder crops) on the Cobar Peneplain.
Location of study sites (S1–S7) in the NSW rangelands and the Interim Biogeographic Regions of Australia (IBRA7 2012). The red outline indicates the boundary of the Australian rangelands.
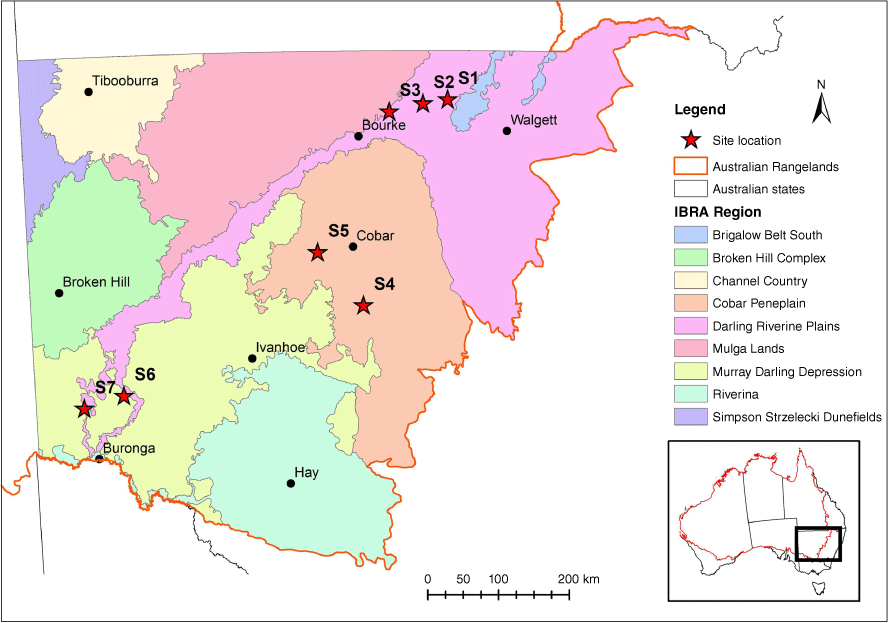
Grazing management
For this study, a study site was either the entire property or a portion of the property that had undergone a change in grazing management. At each site, the livestock management was flexible and highly variable. The manager of each site reported that livestock grazing (comprised of cattle, sheep and/or goats) was managed by moving livestock through multiple (19–120) paddocks, which were grazed for relatively short periods (approximately 1 day–2 weeks) followed by periods of long rest (often more than 6 months), strategically managing the timing of grazing and adjusting stocking rates depending on feed availability, pasture condition, animal needs and the time of year. Managers of each study site had the aim of managing their grazing systems to maintain or improve ground cover, pasture species diversity, pasture quality and productivity of both pastures and livestock. All study sites had been managed according to these principles for at least 10 years prior to this study. Prior to adopting this management approach and establishment of infrastructure (e.g. subdivisional fencing, waterpoint development) to support this change, all managers reported paddocks were predominantly continuously grazed and set stocked across fewer (one to eight) and larger paddocks. Study site and management information is provided in Table 1.
Site identifier | Location | Average annual rainfall (mm) | Vegetation & soil | Study site area (ha) | Number of paddocks | Average graze & rest time A | Average stocking rate B (DSE/ha) | Year of management change | Dominant livestock type | |
---|---|---|---|---|---|---|---|---|---|---|
S1 | Brewarrina | 400 | Floodplain, Mitchell grass grassland, chenopod shrubland, Coolabah open woodland; Vertosols. | 7800 | 62 | 4–10 days graze, 3 month rest | 0.41 | 2007 | Cattle, some sheep | |
S2 | Brewarrina | 385 | Floodplain, Mitchell grass grassland, chenopod shrubland, Coolabah open woodland; Vertosols. | 7100 | 120 | 1–2 day graze, 200 day rest | 0.45 | 2001 | Cattle, some sheep | |
S3 | Bourke | 370 | Sandplain and floodplains, grassland, chenopod shrubland, open woodland and shrublands; Vertosols & Sodosols. | 9900 | 52 | 7–20 day graze, 12 month rest | 0.27 | 2010 | Sheep, cattle | |
S4 | Cobar | 400 | Bimble Box & Pine woodland, shrubland; Kandosols. | 10,000 | 85 | Variable grazing length, 6 month rest | 0.83 | 2009 | Sheep, goats, cattle | |
S5 | Cobar | 340 | Mulga woodland, bimblebox woodland, shrubland; Kandosols, Rudosols & Tenosols. | 15,900 | 19 | Variable, 3–6 month rest | 0.44 | 2010 | Sheep, goats | |
S6 | Pooncarie | 264 | Belah & bluebush; Calcarosols & Tenosols. | 17,000 | 60 | 10–14 day graze, 4–6 month rest | 0.30 | 1999 | Sheep | |
S7 | Wentworth | 262 | Belah & Bluebush. Calcarosols, Vertosols, & Chromosols. | 11,700 | 34 | 10 days graze, 6 months rest | 0.33 | 2002 | Sheep, Cattle |
Dry Sheep Equivalent (DSE) is a standardised unit related to the amount of feed required to maintain a 50 kg wether at constant weight.
For each study site, a 10 km buffer area around the site boundary was selected as a reference point used for comparisons, referred to in this paper as the benchmark area. This distance was chosen to cover a similar size area and have similar landscape and climatic properties to that of the study site. Specific information on stocking rates, grazing approach and management goals for the benchmark area was not collected. The managers of the study sites reported that, generally, management within the benchmark areas was continuous grazing and/or set stocking of larger paddocks. The stocking rates of the study sites and benchmark areas were estimated to be similar by the site managers.
Data collection and analysis
The impact of grazing management on total ground cover was examined using an adaptation of the regional comparison method (Zhang and Carter 2018), and the principles of the Dynamic Reference Cover Method (Bastin et al. 2012) and Compere (Donohue et al. 2022), whereby cover within the study site was compared with biophysically similar areas within 10 km of the study site to control for variability associated with climate and land type.
The landscapes of each study site and the associated benchmark area (combined) were classified using Cibo Lab’s Land Response Units (LRUs). The LRUs consist of pixel groups that share similar values in their vegetation dynamics and landscape properties. They were created using long time series (1987–2022) of satellite-derived vegetation cover, variables derived from the 30-m Digital Elevation Model (including slope and aspect), the surface reflectance of the barest state (Roberts et al. 2019), and the Radiometric Grid of Australia (Radmap), among others. A full description of this product is described in Guerschman et al. (2023). In each property and benchmark area, the LRU segments were grouped into five land types using a k-means clustering approach with an unsupervised classification. These units were compared against satellite imagery, Land Systems (Walker 1991), Plant Community Types (OEH 2023), and validated by the site managers to confirm their suitability. Characteristics of the five land types for each property are summarised in Supplementary Table S1.
At Site 2, approximately 700 ha of scalded areas had been restored with mechanical intervention (ponding and ripping) during the study period. These areas were considered to potentially confound the impacts of the changed grazing management and therefore were excluded from the data and analysis.
Ground cover data were obtained from the Landsat Seasonal Ground Cover version 3 (Department of Environment and Science, Queensland Government 2022). This data product provides an estimate of the ground cover (i.e. the understory, excluding the trees) for every 30-m pixel in Australia, with a seasonal time step and is available from 1989 to present. For each land type in the study site and benchmark area, we derived the median ground cover in each season between 1989 and 2023. Rainfall data were also obtained from the Bureau of Meteorology and summarised as seasonal means for each property.
All data analysis was performed in R (R Core Team 2021). For each study site, the trend in ground cover associated with changed management was assessed by examining the difference in ground cover (GC) between the study site (S) and benchmark (B) area (GCS−B) for each land type. The trend in GCS−B for the whole site was calculated by weighting the means by the area (ha) of each land type within the study site. The trend of GCS−B was generated using the decompose function from the R package stats, which splits the data into seasonal, trend and irregular components. Sen’s slope is the median of all the pairwise slopes between all pairs of points in a data set. The sens.slope command from R package trend (Pohlert 2023) was used to estimate the linear trend before and after management change for the whole site and each land type within each site.
Impact of land type characteristics
To determine if different attributes of each land type affected the direction and magnitude of the Sen’s slope, correlations of the linear trends before and after management change with some of the attributes used to classify the land types were determined, including slope of land (slope), perennial ground cover (PG2020), soil texture (clay), barest earth reflectance in the red, near-infrared and shortwave infrared bands (barestE1, barestE2, barestE3), soil moisture (NDWI50th), plant biomass (tsdmM5), greenness (green M1) and soil reflectance (Th).
Preceding 12 month rainfall trends
We hypothesised that the rainfall preceding the time at which monitoring occurs may affect the magnitude of change observed between the study site and benchmark areas. To test this hypothesis, for each site we classified each seasonal timepoint into three categories denoted ‘dry’ (where the preceding 12 month rainfall was in the lowest tercile), ‘intermediate (where the preceding 12 month rainfall was in the middle tercile), and ‘wet’ (where the preceding 12 month rainfall was in the highest tercile of rainfall years during the study period). The values of GCS−B and the slope of the trend in GCS−B at each seasonal sample time were split into pre- and post-management change for each rainfall category (dry/intermediate/wet), with a t-test used to determine significant differences between the two periods (P < 0.05). Boxplots were used to show the distributions of the data.
Results
Average median ground cover across the seven study sites between 1989 and 2023 ranged from 43% (S2) to 72% (S4), with significant temporal variability (depending on site, a difference of approximately 50–80% between maximum and minimum cover over this period) precluding the ability to detect any obvious trends over this period (Table 2, Fig. 2). Overall, ground cover was approximately 15% higher in winter than summer (Table 2).
Site | Autumn | Winter | Spring | Summer | Annual | |
---|---|---|---|---|---|---|
S1 | 60.3 | 68.4 | 53.7 | 51.7 | 58.5 | |
S2 | 44.3 | 52.1 | 38.2 | 37.3 | 42.9 | |
S3 | 54.7 | 60.9 | 47.9 | 47.6 | 52.8 | |
S4 | 69.6 | 79.5 | 71.4 | 67.0 | 71.8 | |
S5 | 64.5 | 75.8 | 65.4 | 62.2 | 66.9 | |
S6 | 53.1 | 65.3 | 55.8 | 49.8 | 56.0 | |
S7 | 55.1 | 67.6 | 54.4 | 49.6 | 56.6 | |
Overall site average | 57.3 | 67.0 | 55.4 | 52.2 | 57.9 |
Median seasonal ground cover (%) across each site and associated benchmark area between 1989 and 2023. The vertical dotted line represents the date at which grazing management began to change across each site.
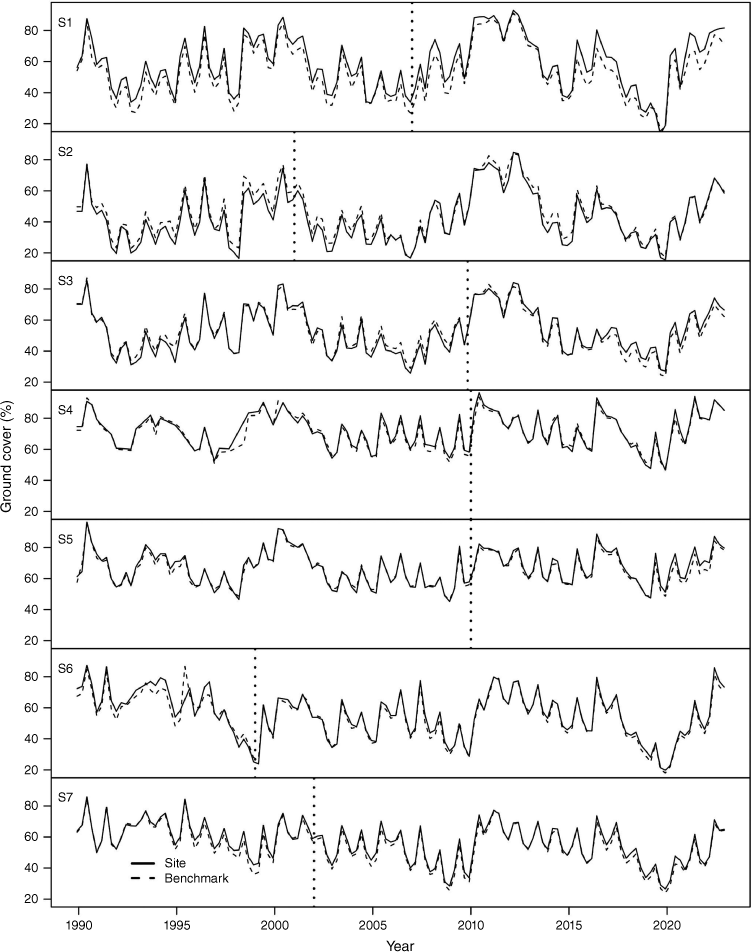
Prior to implementation of management changes, ground cover across five of the seven study sites was decreasing relative to that of the benchmark areas (Fig. 3). Following the management change of flexible stocking rates and introducing rest in the grazing system, a significant positive change in ground cover (ranging from 2 to 7% from the time of management change, P < 0.05), as determined by a significantly different slope between the pre- and post-management periods, was observed at four of the seven study sites (S2, S3, S5, S6; Figs 3 and 4). A nonsignificant positive change was observed at a fifth site (S1, P = 0.45; Fig. 4). At S4, there was no significant difference overall, although this site had confounding impacts of a clearing and an occasional cropping cycle and had the highest average ground cover of all the sites. At S7, there was no significant change in ground cover in the 20 years from management change relative to the benchmark area.
The trend of difference in total ground cover (%) between the study site and benchmark areas (GCS−B). The straight lines represent the median slope of the trend pre- and post-management change (Pohlert 2023). The vertical dotted line represents the date at which grazing management began to change across each site. Seasonal (3 monthly) rainfall totals are represented by blue bars on secondary axis.
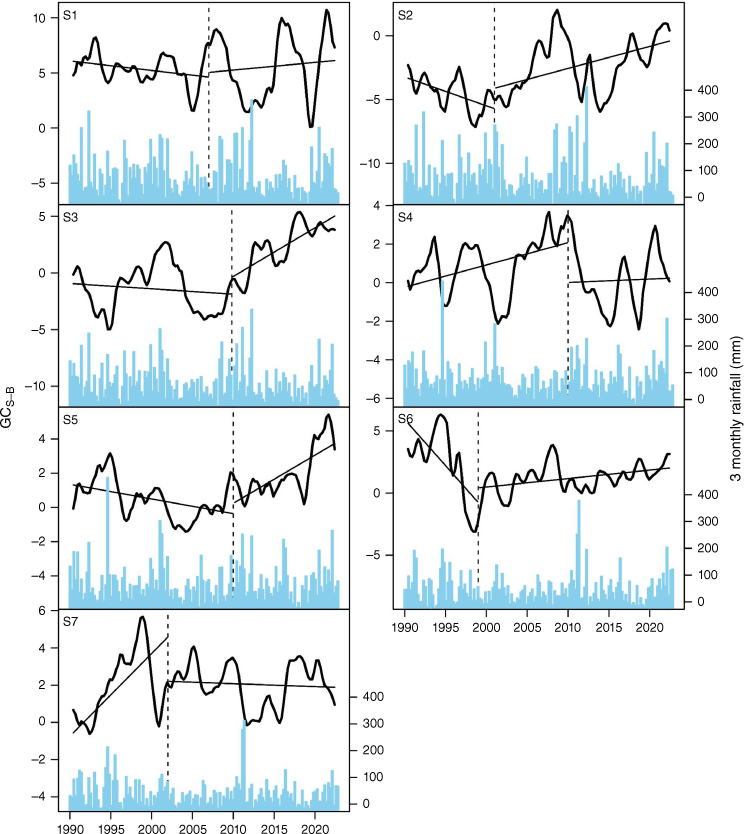
The median (±95% confidence intervals) slope (analysed as Sen’s slope, Pohlert 2023) of the trend of the difference between the study site (S1–S7) and benchmark areas pre- and post-management change. Positive values represent increasing cover on the property relative to the benchmark and negative values vice versa. Where the confidence interval overlaps zero, this indicates no significant trend in the change in ground cover between site and benchmark areas.
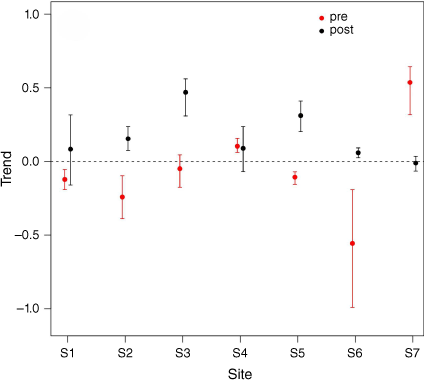
Trends reported here are a simplified representation of temporally dynamic time series. When examined at a finer temporal resolution, trends over time are nonlinear and at times are comprised of shorter-term reversals in trends. Assessment of the linear trends for each land type within a property also indicate that the direction and magnitude of change was dependent on land type (Supplementary Fig. S1). There was a weak negative association between the trend in GCS−B post-management change with the normalised difference water index (NDWI), total standing dry matter (TSDM) and green reflectance (r2 = −0.36, −0.15 and −0.16 respectively), potentially indicating that less productive land types had the greatest positive response to the management change (Supplementary Fig. S2).
Analysis of the effect of preceding 12 month rainfall on GCS−B and the trend of GCS−B pre- and post-management change (Fig. 5a) showed that in wet years the trend in GCS−B was negative across all sites (significant at S1 and S7; Fig. 5b). This indicates that ground cover in the benchmark areas increased more (or decreased less), than the study sites during these periods. There was no significant difference in the trend in GCS−B pre- and post-management change at any study site during dry periods, but in intermediate rainfall years the trend in GCS−B was significantly more positive post-management change at S2 (Fig. 5a).
Comparison of (a) the trend of the slope of difference in ground cover between the study site and benchmark (GCS−B) and (b) median ground cover GCS−B between pre- (yellow) and post- (blue) management change for sampling periods where preceding 12 month rainfall was in the lowest (Dry), middle (Intermediate) and highest (Wet) tercile of rainfall years during the analysis period. Black dots indicate individual data points for the slope (a) and median difference (b) for each sampling period, and the boxes represent the inter quartile range of data. Significant differences (P < 0.05) of the mean between pre- and post-management change are indicated by an asterisk. (same as previous caption)
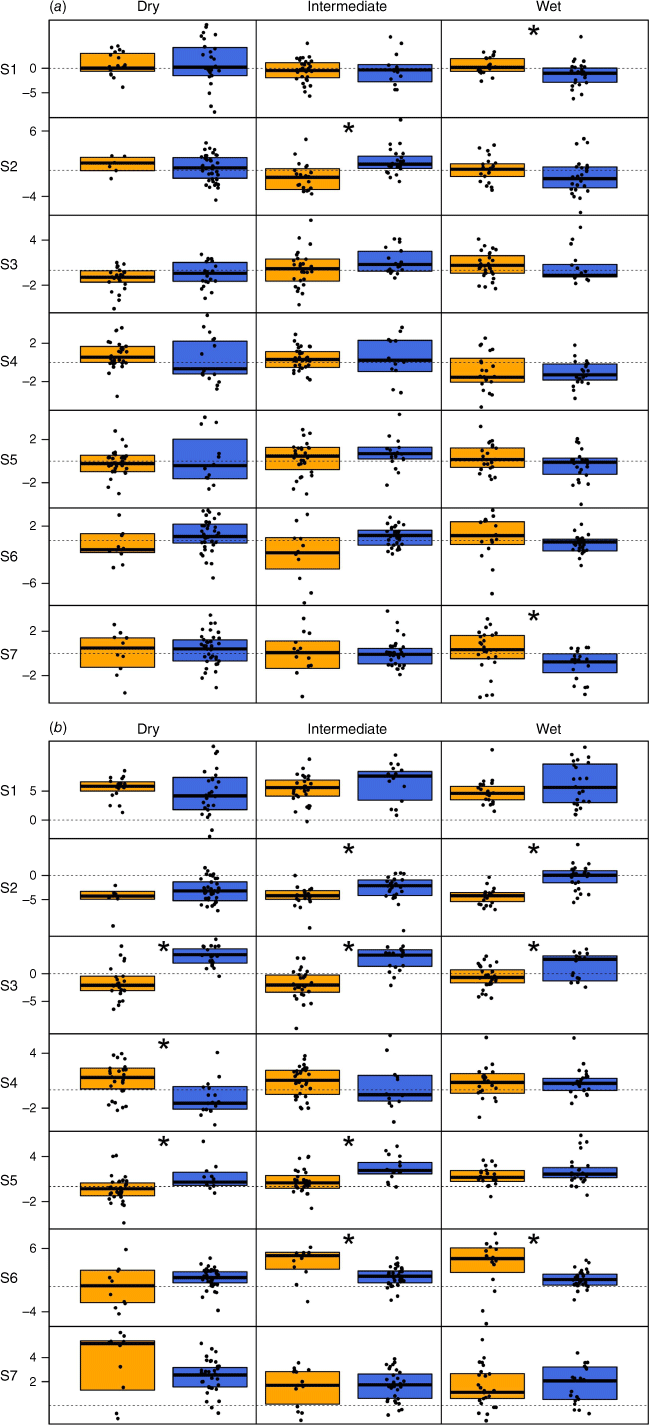
Discussion
This study demonstrated the capacity for improved grazing management (matching stocking rate to carrying capacity and providing regular periods of long rest from grazing) to increase ground cover and associated ecosystem services across four of the seven heterogeneous, dynamic rangeland grazing sites examined in this study. These results are supported by findings elsewhere in Australian rangelands. Using remote sensing in the Great Barrier Reef Catchment, Queensland, Bartley et al. (2023) demonstrated a ground cover increase at some, but not all sites that had adopted regenerative grazing management (i.e. planned rotational grazing). The potential for high-intensity rotational or multi-paddock grazing systems (>20 paddocks) in conjunction with flexible stocking rates to improve ground cover has rarely been tested in Australia, at scale, and under ‘real world’ conditions where the practical implications and seasonal adaptations that land managers face are incorporated. Results of previous smaller scale, cross fence studies comparing ground cover between two or more grazing management treatments in the southern Australian rangelands are also consistent with our findings, demonstrating potential improvements in ground cover in these managed grazing systems (Alemseged et al. 2011; Waters et al. 2017; McDonald et al. 2019b). However, in other areas of Australia, benefits of intensive rotational grazing systems are less clear. For example, in Queensland, Hall et al. (2014) found no difference in total ground cover between intensive rotational grazing, extensive rotational grazing and continuous grazing systems. Similarly, a synthesis of evidence related to sustainable grazing management practices for northern Australia found little benefit of complex multi-paddock grazing systems (O’Reagain et al. 2014). Across Australia, a recent meta-analysis of grazing intensity and strategy comparisons revealed significantly higher ground cover under lower intensity grazing and in grazing systems that incorporate periods of rest (e.g. rotational grazing) compared with continuous grazing systems (McDonald et al. 2023), however this review did not examine the nuances of different grazing systems and the interaction of grazing intensity and strategy. Across the four sites where a significant improvement in ground cover relative to that of the benchmark sites was observed from the time of management change, the increase was up to 7%. This increase is consistent with the reported increase in cover from the ‘Enterprise Based Conservation’ project in western NSW (which reported change in ground over ranging from 0.63 to 2.69% after 5 years of destocking or managing grazing to maintain cover; Moss et al. 2012).
Each site, and each land type within each site, experienced differing patterns in the response of total ground cover following management change, likely reflecting the complexity and considerable variability in land types, rainfall, climate patterns and grazing management approaches across properties in western NSW. The variable response across sites and land types is consistent with previous multi-site studies in NSW rangelands (e.g. Waters et al. 2017). This inconsistency in the observed response highlights the challenges of detecting and measuring change and recommending best management to achieve environmental outcomes in the rangelands, and may account for the often variable and conflicting results reported in grazing management studies (Briske et al. 2008). We were not able to confidently predict where (i.e. land type) and when (i.e. preceding 12 month rainfall) the changes (if any) would be most apparent with the approach and landscape variables used in this study, although there was some indication that increased ground cover in response to the grazing management change was greatest in areas of lower productivity (i.e. lower NDWI, TSDM and greenness). Alternative analysis methods that explored additional predictor variables or increased spatial resolution may reduce the variability and provide better prediction of the key landscape determiners of change. The considerable variability in the GCS−B and the trend of GCS−B over time at individual sites (Figs 2 and 3), demonstrates the value and importance of longer term temporal studies to understand impacts of management changes in this environment. To better understand and report change, for example for use in environmental accounting, we recommend frequent measurements (at least seasonal to capture the temporal variability), at property scale and where possible minimising the impact of climatic variability by comparing against dynamic benchmarks that are subject to similar land type and climate constraints.
Response times are often slow in rangeland environments and are driven by rainfall events. Previous studies have indicated it can take over 20 years for arid and semiarid regions of Australia to respond to management changes (e.g. Bartley et al. 2023). It is also pertinent to recognise that in this study, the year of management change represents when these property managers started their new grazing management approach. In reality it takes years to fully implement and refine the grazing management strategy at the property scale, for example, installing fencing, water points and logistics of bringing together livestock (and associated classes) in large groups to rotationally graze. Across all properties, management changes over the 10–20 years from initial change were incremental and were continuing at the time of this study. Therefore, it is possible that the benefits of the changed grazing management are not yet fully apparent. It is also important to consider that with overall low productivity and high variability in ground cover for this region, we are unable to determine if the change in ground cover that has been achieved, and the rate of change, is able to be sustained over longer timeframes. A reference ecosystem or site able to act as a benchmark, like those used in the National Restoration Standards (Standards Reference Group SERA 2021), could potentially provide an indication of maximum attainable ground cover, however to the best of our knowledge these are not documented for the semi-arid rangelands of NSW. The benefit of the approach taken with this study, is that this low-cost temporal evaluation can be undertaken regularly, and in the future at a meaningful scale in these extensively grazed rangeland systems. With the emergence of online tools and platforms to access remotely sensed ground cover data, the approach taken in this study has the potential to be integrated and automated to provide producers real-time information to aid their decision making in managing their livestock to maintain or improve ground cover and demonstrate environmental outcomes. There is a continued drive for ecosystem service markets, supported by robust, repeatable, transparent and accurate methods of monitoring and measuring change in response to management actions. This is of particular importance in rangeland environments where distance, scale, variability in season and landscapes across properties adds additional challenges to monitoring and measuring change. Importantly, for environmental accounting, this approach is management sensitive, outcome driven and reflects the current climatic conditions, thereby meeting integrity standards and minimising the impact of any metrics primarily associated with patterns of extreme dry (e.g. El Nino and associated droughts) or wet (e.g. La Nina). With continued development of remote sensing technologies, including the use of different sensors, Light Detection and Ranging (LiDAR) and Unmanned Aerial Vehicles (UAVs), and additional indicators of condition, any response of ground cover and other ecosystem services to grazing management can be quantified and demonstrated with greater precision, potentially increasing access to environmental markets in rangeland grazing systems.
Study limitations, assumptions and future research recommendations
There are a number of potential limitations and assumptions behind the approach used in this study, which may have limited the ability to detect small, incremental changes and influenced the precision of our conclusions. These are summarised below:
We did not gather detailed information on management within the 10 km benchmark area surrounding each study site. Our approach was to trial a less intrusive, cost-effective, automatable and scalable approach to evaluating and documenting changes in ground cover attributable to management. Thus there are multiple assumptions associated with management within the benchmark area, including that the grazing management has not changed substantially over time and that it is reflective of more conventional management (predominantly continuous grazing at set stocking rates) like that of the sites prior to management change.
Across all study sites, grazing pressure by unmanaged herbivores (predominantly goats and kangaroos) was at times considerable, resulting in an inability to achieve true rest of pastures from grazing and exaggerating stocking rates. Although producers attempt to manage this pressure as best they can, and in recent years some have invested in infrastructure (e.g. fencing and controlling access to water points) to reduce this impact, it may have masked some of the potential benefits of short graze periods, resting paddocks from livestock grazing and matching stocking rate to feed availability. Further research to understand this impact and demonstrate the benefits of total grazing pressure management is required and may be achieved using a similar approach to this study.
Information presented in this study is representative only of total ground cover, and it is important to recognise there are other important indicators of land condition (including botanical composition, soil health, pasture biomass, landscape function, for example). We were not able to assess changes and improvements in these indicators through the approach used; changes in which were reported anecdotally by many of the study site managers. Measurement of other indicators such as vegetation structure (i.e. the height and distribution of ground cover) would also enable estimation of exposure to wind erosion (Webb et al. 2020) and potential quantification of the ecosystem service provided by improved grazing management. As technology advances this is something to revisit in the future, and on-ground data collection of these variables at smaller scales in targeted locations would complement the ground cover results of this study.
Due to the nature of this study, it was not possible to separate effects of managing the timing and duration of grazing events and managing stocking rates to match feed availability. Rather, the study was designed to evaluate the potential (and constraints) to achieve increases in ground cover through strategic grazing management of livestock in southern Australian rangeland systems. To provide more specific management recommendations to achieve change, understanding management nuances (e.g. grazing intensity, timing and duration of rest/graze) or better predicting which land types might achieve significant improvement and identifying where to invest for biggest returns, more study sites representing the range of grazing management approaches or a higher resolution of grazing management impacts within each property is required.
In some regions of western NSW, clearing, cropping or high tree and shrub cover may limit the application of this approach or confound the effects of grazing management being evaluated. These factors limit our confidence in the results reported in this study for Site 4 in particular, and further research is required to improve confidence in this approach and to more reliably understand changes in response to grazing when confounded with other land management activities.
Conclusion
The study has highlighted that livestock producers can potentially achieve improvements in ground cover and associated ecosystem services through changes in grazing management (management of stocking rates and introduction of planned rest into grazing systems). However, the magnitude and direction of response observed may be dependent on specific grazing management decisions, the timing of monitoring, land type and preceding rainfall during which the management change is evaluated. With increasing pressure for livestock producers to demonstrate sustainable management, and emergence of environmental markets rewarding producers for provision of ecosystem services, this study supports the used of remote sensing using biophysically similar, dynamic benchmarks (Bastin et al. 2012; Zhang and Carter 2018; Donohue et al. 2022) to detect and measure change and support the adoption of management of the timing and intensity of livestock grazing in variable semiarid rangeland environments.
Data availability
The data that support this study will be shared upon reasonable request to the corresponding author.
Conflicts of interest
Sarah McDonald is an Associate Editor of The Rangeland Journal. To mitigate this potential conflict of interest she had no editor-level access to this manuscript during peer review. The authors have no further conflicts of interest to declare.
Acknowledgements
The authors would like to thank the producers on whose properties this study was undertaken and who provided information regarding their management and management history. We are also grateful for the review of this manuscript by Warwick Badgery and Lachy Ingram and the anonymous reviewers who significantly improved the quality of this paper.
References
ABSF (2024) ‘Australian Beef Sustainability Framework.’ (Meat & Livestock Australia Limited) Retrieved from https://www.sustainableaustralianbeef.com.au/ [accessed on 16 April 2024]
Alemseged Y, Hacker RB, Smith WJ, Melville GJ (2011) Temporary cropping in semi-arid shrublands increases native perennial grasses. The Rangeland Journal 33, 67-78.
| Crossref | Google Scholar |
Barnetson J, Phinn S, Scarth P, Denham R (2017) Assessing Landsat fractional ground-cover time series across Australia’s arid rangelands: separating grazing impacts from climate variability. The International Archives of the Photogrammetry, Remote Sensing and Spatial Information Sciences 42, 15-26.
| Crossref | Google Scholar |
Bartley R, Abbott BN, Ghahramani A, Ali A, Kerr R, Roth CH, Kinsey-Henderson A (2023) Do regenerative grazing management practices improve vegetation and soil health in grazed rangelands? Preliminary insights from a space-for-time study in the Great Barrier Reef catchments, Australia. The Rangeland Journal 44, 221-246.
| Crossref | Google Scholar |
Bastin G, Scarth P, Chewings V, Sparrow A, Denham R, Schmidt M, O’Reagain P, Shepherd R, Abbott B (2012) Separating grazing and rainfall effects at regional scale using remote sensing imagery: a dynamic reference-cover method. Remote Sensing of Environment 121, 443-457.
| Crossref | Google Scholar |
Bastin G, Cowley R, Friedel M, Materne C (2024) Applying two remotely-sensed methods for monitoring grazing impacts in the Australian arid zone. The Rangeland Journal 45, 141-159.
| Crossref | Google Scholar |
Bell LW, Hayes RC, Pembleton KG, Waters CM (2014) Opportunities and challenges in Australian grasslands: pathways to achieve future sustainability and productivity imperatives. Crop & Pasture Science 65, 489-507.
| Crossref | Google Scholar |
Beutel TS, Trevithick R, Scarth P, Tindall D (2019) VegMachine.net. online land cover analysis for the Australian rangelands. The Rangeland Journal 41, 355-362.
| Crossref | Google Scholar |
Beutel TS, Shepherd R, Karfs RA, Abbott BN, Eyre T, Hall TJ, Barbi E (2021) Is ground cover a useful indicator of grazing land condition? The Rangeland Journal 43, 55-64.
| Crossref | Google Scholar |
BOM (2024) ‘Maps of average conditions.’ (Bureau of Meteorology, Australian Government) Accessed from http://www.bom.gov.au/climate/maps/averages/ [accessed on 7 February 2024]
Briske DD, Derner JD, Brown JR, Fuhlendorf SD, Teague WR, Havstad KM, Gillen RL, Ash AJ, Willms WD (2008) Rotational grazing on rangelands: reconciliation of perception and experimental evidence. Rangeland Ecology & Management 61, 3-17.
| Google Scholar |
Briske DD, Coppock DL, Illius AW, Fuhlendorf SD, Niu K (2020) Strategies for global rangeland stewardship: assessment through the lens of the equilibrium–non‐equilibrium debate. Journal of Applied Ecology 57, 1056-1067.
| Crossref | Google Scholar |
Byrnes RC, Eastburn DJ, Tate KW, Roche LM (2018) A global meta-analysis of grazing impacts on soil health indicators. Journal of Environmental Quality 47, 758-765.
| Crossref | Google Scholar | PubMed |
Chappell A, Webb NP, Leys JF, Waters CM, Orgill S, Eyres MJ (2019) Minimising soil organic carbon erosion by wind is critical for land degradation neutrality. Environmental Science & Policy 93, 43-52.
| Google Scholar |
Department of Environment and Science, Queensland Government (2022) Seasonal ground cover - Landsat, JRSRP algorithm Version 3.0, Australia Coverage. Version 1.0. Terrestrial Ecosystem Research Network (Dataset). Available at https://portal.tern.org.au/metadata/TERN/fe9d86e1-54e8-4866-a61c-0422aee8c699
di Virgilio A, Lambertucci SA, Morales JM (2019) Sustainable grazing management in rangelands: over a century searching for a silver bullet. Agriculture, Ecosystems & Environment 283, 106561.
| Google Scholar |
Donohue RJ, Mokany K, McVicar TR, O’Grady AP (2022) Identifying management‐driven dynamics in vegetation cover: applying the Compere framework to Cooper Creek, Australia. Ecosphere 13, e4006.
| Crossref | Google Scholar |
Foran B, Smith MS, Burnside D, Andrew M, Blesing D, Forrest K, Taylor J (2019) Australian rangeland futures: time now for systemic responses to interconnected challenges. The Rangeland Journal 41, 271-292.
| Crossref | Google Scholar |
Gray JM, Wang B, Waters CM, Orgill SE, Cowie AL, Ng EL (2022) Digital mapping of soil carbon sequestration potential with enhanced vegetation cover over New South Wales, Australia. Soil Use and Management 38, 229-247.
| Crossref | Google Scholar |
Greene R, Tongway DJ (1989) The significance of (surface) physical and chemical properties in determining soil surface condition of red earths in rangelands. Soil Research 27, 213-225.
| Crossref | Google Scholar |
Greene RSB, Kinnell PIA, Wood JT (1994) Role of plant cover and stock trampling on runoff and soil-erosion from semi-arid wooded rangelands. Soil Research 32, 953-973.
| Crossref | Google Scholar |
Guerschman JP, Scarth P, Tickle P (2023) ‘Accelerating the Adoption of Satellite Assisted Forage Budgeting Across Northern Beef Businesses.’ (Meat and Livestock Australia Limited) Retrieved from https://www.mla.com.au/contentassets/9d148c6d96b344de8cff0ab0ac6b2c6a/p.psh.1286-cibo-finalreport_feb2024_final.pdf [accessed on 3 May 2024]
Hacker RB, McDonald SE (2021) Prospects for sustainable use of the pastoral areas of Australia’s southern rangelands: a synthesis. The Rangeland Journal 43, 185-209.
| Crossref | Google Scholar |
Hall TJ, McIvor JG, Reid DJ, Jones P, MacLeod ND, McDonald CK, Smith DR (2014) A comparison of stocking methods for beef production in northern Australia: pasture and soil surface condition responses. The Rangeland Journal 36, 161-174.
| Crossref | Google Scholar |
Jansen V, Traynor ACE, Karl JW, Lepak N, Sprinkle J (2022) Monitoring grazing use: strategies for leveraging technology and adapting to variability. Rangelands 44, 64-77.
| Crossref | Google Scholar |
Landsberg J, James CD, Morton SR, Müller WJ, Stol J (2003) Abundance and composition of plant species along grazing gradients in Australian rangelands. Journal of Applied Ecology 40, 1008-1024.
| Crossref | Google Scholar |
Leys J, Heidenreich S, White S, Guerschaman J, Strong C (2023) Dust-storm frequencies, community attitudes, government policy and land management practices during three major droughts in New South Wales, Australia. The Rangeland Journal 44, 343-355.
| Crossref | Google Scholar |
Ludwig JA, Tongway DJ, Bastin GN, James CD (2004) Monitoring ecological indicators of rangeland functional integrity and their relation to biodiversity at local to regional scales. Austral ecology 29, 108-120.
| Crossref | Google Scholar |
MacLeod ND, McIvor JG (2006) Reconciling economic and ecological conflicts for sustained management of grazing lands. Ecological Economics 56, 386-401.
| Crossref | Google Scholar |
McDonald SE, Reid N, Waters CM, Smith R, Hunter J (2018) Improving ground cover and landscape function in a semi-arid rangeland through alternative grazing management. Agriculture, Ecosystems & Environment 268, 8-14.
| Google Scholar |
McDonald SE, Lawrence R, Kendall L, Rader R (2019a) Ecological, biophysical and production effects of incorporating rest into grazing regimes: a global meta‐analysis. Journal of Applied Ecology 56, 2723-2731.
| Crossref | Google Scholar |
McDonald SE, Reid N, Smith R, Waters CM, Hunter J, Rader R (2019b) Rotational grazing management achieves similar plant diversity outcomes to areas managed for conservation in a semi-arid rangeland. The Rangeland Journal 41, 135-145.
| Crossref | Google Scholar |
McDonald SE, Badgery W, Clarendon S, Orgill S, Sinclair K, Meyer R, Butchart DB, Eckard R, Rowlings D, Grace P, Doran-Browne N (2023) Grazing management for soil carbon in Australia: a review. Journal of Environmental Management 347, 119146.
| Crossref | Google Scholar | PubMed |
Moss J, Sinden J, Stayner R (2012) Estimating the cost of protecting groundcover on privately managed properties in the Australian rangelands: the case of the West 2000 Plus Enterprise-Based Conservation scheme. The Rangeland Journal 34, 75-87.
| Crossref | Google Scholar |
NFF (2018) 2030 Roadmap. (National Farmers Federation) Retrieved from https://nff.org.au/policies/roadmap/ [accessed on 16 April 2024]
OEH (2023) NSW Plant Community Type Classification. (Environment and Heritage, NSW Government). Retrieved from https://www.environment.nsw.gov.au/topics/animals-and-plants/biodiversity/nsw-bionet/nsw-plant-community-type-classification [accessed on 16 April 4 2024]
O’Reagain P, Scanlan J, Hunt L, Cowley R, Walsh D (2014) Sustainable grazing management for temporal and spatial variability in north Australian rangelands – a synthesis of the latest evidence and recommendations. The Rangeland Journal 36, 223-232.
| Crossref | Google Scholar |
Orgill SE, Waters CM, Melville G, Toole I, Alemseged Y, Smith W (2017) Sensitivity of soil organic carbon to grazing management in the semi-arid rangelands of south-eastern Australia. The Rangeland Journal 39, 153-167.
| Crossref | Google Scholar |
Pickup G (1996) Estimating the effects of land degradation and rainfall variation on productivity in rangelands: an approach using remote sensing and models of grazing and herbage dynamics. Journal of Applied Ecology 33, 819-832.
| Crossref | Google Scholar |
Pohlert T (2023) ‘Trend: Non-Parametric Trend Tests and Change-Point Detection.’ (R package version 1.1.6) Available at https://CRAN.R-project.org/package=trend
R Core Team (2021) ‘R: A language and environment for statistical computing.’ (R Foundation for Statistical Computing: Vienna, Austria) Available at https://www.R-project.org/
Retallack A, Finlayson G, Ostendorf B, Clarke K, Lewis M (2023) Remote sensing for monitoring rangeland condition: current status and development of methods. Environmental and Sustainability Indicators 19, 100285.
| Crossref | Google Scholar |
Roberts D, Wilford J, Ghattas O (2019) Exposed soil and mineral map of the Australian continent revealing the land at its barest. Nature Communications 10, 5297.
| Crossref | Google Scholar | PubMed |
SSF (2024) ‘Sheep Sustainability Framework.’ (Meat & Livestock Australia Limited) Retrieved from https://www.sheepsustainabilityframework.com.au/ [accessed on 16 April 2024]
Stafford Smith DM, McKeon GM, Watson IW, Henry BK, Stone GS, Hall WB, Howden SM (2007) Learning from episodes of degradation and recovery in variable Australian rangelands. Proceedings of the National Academy of Sciences 104, 20690-20695.
| Crossref | Google Scholar | PubMed |
Teague R, Barnes M (2017) Grazing management that regenerates ecosystem function and grazingland livelihoods. African Journal of Range & Forage Science 34, 77-86.
| Google Scholar |
Teague R, Kreuter U (2020) Managing grazing to restore soil health, ecosystem function, and ecosystem services. Frontiers in Sustainable Food Systems 4, 534187.
| Crossref | Google Scholar |
Tongway D, Hindley N (2004) Landscape function analysis: a system for monitoring rangeland function. African Journal of Range and Forage Science 21, 109-113.
| Crossref | Google Scholar |
Wang B, Waters C, Orgill S, Cowie A, Clark A, Li Liu D, Simpson M, McGowen I, Sides T (2018) Estimating soil organic carbon stocks using different modelling techniques in the semi-arid rangelands of eastern Australia. Ecological Indicators 88, 425-438.
| Crossref | Google Scholar |
Ward DP, Kutt AS (2009) Rangeland biodiversity assessment using fine scale on-ground survey, time series of remotely sensed ground cover and climate data: an Australian savanna case study. Landscape Ecology 24, 495-507.
| Crossref | Google Scholar |
Waters CM, Orgill SE, Melville GJ, Toole ID, Smith WJ (2017) Management of grazing intensity in the semi-arid rangelands of Southern Australia: effects on soil and biodiversity. Land Degradation & Development 28, 1363-1375.
| Google Scholar |
Webb NP, Kachergis E, Miller SW, McCord SE, Bestelmeyer BT, Brown JR, Chappell A, Edwards BL, Herrick JE, Karl JW, Leys JF, Metz LJ, Smarik S, Tatarko J, Van Zee JW, Zwicke G (2020) Indicators and benchmarks for wind erosion monitoring, assessment and management. Ecological Indicators 110, 105881.
| Crossref | Google Scholar |
Witt GB, Althor G, Colvin RM, Witt KJ, Gillespie N, McCrea R, Lacey J, Faulkner T (2021) How environmental values influence trust and beliefs about societal oversight and need for regulation of the Australian cattle industry. Environmental Research Letters 16, 034006.
| Crossref | Google Scholar |
Viscarra Rossel RA, Zhang M, Behrens T, Webster R (2024) A warming climate will make Australian soil a net emitter of atmospheric CO2. npj Climate and Atmospheric Science 7, 79.
| Crossref | Google Scholar |
Zhang B, Carter J (2018) FORAGE – An online system for generating and delivering property-scale decision support information for grazing land and environmental management. Computers and Electronics in Agriculture 150, 302-311.
| Crossref | Google Scholar |