Climate change must be factored into savanna carbon- management projects to avoid maladaptation: the case of worsening air pollution in western Top End of the Northern Territory, Australia
David M. J. S. Bowman
A
B
C
Abstract
Savanna fires are a major source of greenhouse gas (GHG) and particulate pollution globally. Since mid-2006, an Australian Government carbon offset program has incentivised Northern Territory land managers to undertake early dry-season savanna burning with the aim of reducing late dry-season wildfires and associated GHG emissions. The focus of this study is addressing concern that savanna burning carbon abatement projects are causing worsening air pollution in the city of Darwin. Reconstructed concentrations of daily particulate matter of <2.5 μm (PM2.5) since the 1960s showed since 2000s a worsening in PM2.5 in the early dry season (May, June, July), some improvement in the late dry season (August, September, October) with little overall difference for the whole dry season. Remote-sensing PM2.5 estimates in Darwin were correlated with region-wide PM2.5 estimates during the early dry season. Remote-sensing analysis of area burned and intensity of fires since 2002 showed that savanna carbon projects have shifted burning to the early dry season and caused increases in fire intensity compared with non-project areas. Increased fire intensity appears to follow sharply declining fuel moisture, as well as management effects on carbon project areas, which have possibly undermined the efficacy of savanna burning projects in reducing GHG emissions. More thorough evaluation of underlying assumption of savanna burning carbon abatement in Australia and elsewhere in the world is required to avoid maladaptation, such as over-crediting, smoke pollution, and other environmental harms.
Keywords: climate change, fire intensity, fire management, fuel moisture, greenhouse gas, MODIS, remote sensing, tropical savanna, wildfire.
Introduction
An urgent global challenge is arresting anthropogenic climate change. This demands decarbonisation of energy and industrial processes and careful management of terrestrial carbon stocks (Keenan and Williams 2018). Landscape fires are a particularly important component in carbon management because of their potential to either increase or decrease greenhouse gas (GHG) emissions (Bowman et al. 2023). A prime example of this concerns tropical savannas, a highly fire-prone vegetation type that accounts for approximately 65% of the global carbon emissions from landscape fires (Van Der Werf et al. 2017). It has been argued that stewardship of savanna fire regimes globally presents an opportunity to reduce GHG emissions by reducing fire intensity and area burned (Lipsett-Moore et al. 2018). This proposal is based on the development of carbon-abatement projects in northern Australian tropical savannas (Edwards et al. 2021). These savanna carbon projects are now regulated under the Australian Government’s carbon offset scheme (Carbon Credits (Carbon Farming Initiative) Act 2011 (Cth)) and earn Australian carbon-credit units (ACCUs) under a statutory savanna-burning carbon-offset method. Each ACCU issued under the scheme is supposed to represent real and additional abatement of GHG emissions equivalent to 1 tonne of carbon dioxide equivalent (CO2e). The ACCUs are a tradable financial instrument, with the primary source of demand now being large polluting facilities that can use the ACCUs to meet statutory obligations to keep their net emissions below prescribed emission limits.
In brief, the premise of the savanna carbon projects is that intentionally setting fires in the early dry season produces less GHG emissions because most fires are of lower intensity, consume less biomass per hectare, and are less extensive and more spatially patchy, reflecting higher fuel moisture when compared to late dry season wildfires that were prevalent before the projects began (Edwards et al. 2021). Operationally, the Australian approach rewards landowners and land managers who burn before 1 August, the date used to define the start of the late dry season (Australian Government 2018a, 2018b; Department of Climate Change 2023). The savanna carbon projects were first established in the Northern Territory in 2006 on Aboriginal land in western Arnhem Land and have been steadily expanded throughout this region (Fig. 1).
Savanna burning projects in the top part (latitudes north of 16°S) of the Northern Territory, Australia. (a) The geographic location of the Top End of the Northern Territory. (b) Spatial extent of the projects is shown. (c) The number of Australian carbon-credit units (ACCU) for year (since 2012), broken down by project, where a ACCU represents 1 tonne of carbon dioxide equivalent (CO2e) emissions stored or avoided by a project. We selected only savanna-burning projects that had not been revoked, and were located within the top half of the Northern Territory (Australian Government Clean Energy Regulator 2023). Date of registration each project is shown in parentheses.
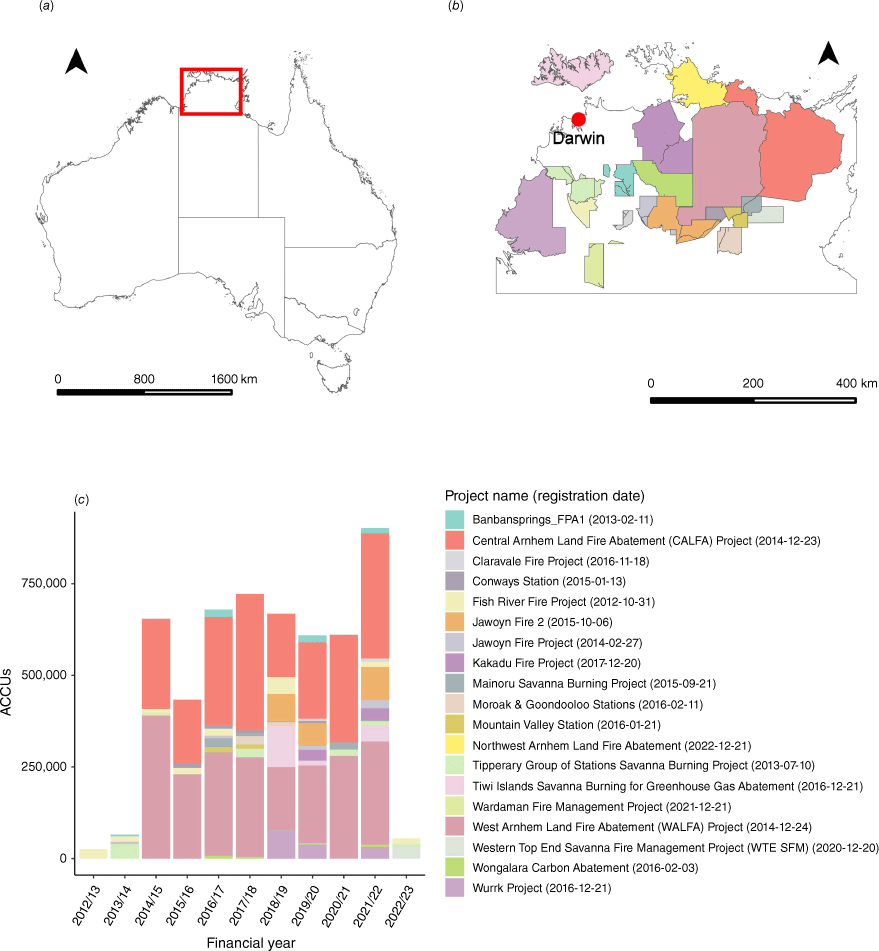
As of 1 October 2023, there were 79 registered savanna- burning projects across Australia. Since the ACCU scheme commenced, savanna burning projects have received 12.5 million ACCUs, just over 9% of total ACCU issuances, making them the fourth largest project type under the ACCU scheme by credit issuances (Australian Government Clean Energy Regulator 2023). The Northern Territory currently has 24 registered projects that cover ~48% of the northern part of the Northern Territory, above the latitude of 16°S (Fig. 1) (Australian Government Clean Energy Regulator 2023). These projects have received a total of 6.7 million ACCUs to October 2023, worth more than AU$200 million at prevailing market prices.
There is substantial public health concern that the tropical city of Darwin has the worst air quality of any Australian capital city because of wildfire smoke pollution (Jones et al. 2022; Tham et al. 2022). Particulate matter, especially the smaller size class particles of less than 2.5 μm in diameter (PM2.5), in wildfire smoke are known to cause significant ill health and death, with no safe lower threshold, albeit air quality standards are set to limit exposures above 25 μg m−3, measured over a 24 h period (Vardoulakis et al. 2020). Greenhouse gases and particulate matter in wildfire smoke are positively correlated (Reisen et al. 2018), so the recent declining air quality in Darwin despite all the concurrent activity in the Top End to reduce carbon emissions, is counterintuitive. It would be expected that air quality in Darwin should be benefiting from the savanna burning schemes in the Top End of the Northern Territory (Fig. 1). Jones et al. (2022) investigated Darwin’s worsening air quality, finding a statistical association with area burned up to 500 km from Darwin in the early, but not late, dry season. These authors suggested that the worsening air quality in Darwin may be linked to the savanna carbon projects.
Here, we extend the work of Jones et al. (2022) to better investigate the seeming paradox of GHG savanna burning being correlated with worsening air quality in Darwin. Our approach is multipronged, using available data to provide a robust spatial–temporal context of Darwin air quality and changes in landscape burning. Specifically, we reconstruct PM2.5 in Darwin air shed in the early, late, and whole dry season by using airport visibility records, building on the approach of Bowman et al. (2007a), and determine the correlation of Darwin PM2.5 with region-wide air quality by using remote sensing-based estimates of air pollution (Van Donkelaar et al. 2021). We then evaluate trends in the area burned and the fire intensity in the early, late, and whole dry season, and model monthly changes in fire intensity and fuel moisture derived from gridded meteorological data. We discuss our findings in terms of their public health significance and the implications for effective savanna burning GHG management. We also briefly discuss the implications of our research for potential maladaptive (Schipper 2020, 2022) effects of landscape carbon abatement programs that are being widely promoted in savanna landscapes across northern Australia and elsewhere in the world. All analyses presented were done using the R statistical software (v.4.2.2, https://www.r-project.org/).
Methods
Darwin airshed pollution: instrumental trends and historical reconstruction
Daily fine particulate matter (PM2.5) observations for Darwin were sourced from the Northern Territory Environment Protection Agency for four monitoring sites (Casuarina, Palmerston, Stokes Hill, Winnellie) between 2004 and 2022 (Northern Territory Environment Protection Authority 2023). Daily visibility data for Darwin Airport from 1960 to 2023 was sourced from the Bureau of Meteorology, with corresponding daily temperature (minimum, mean, and maximum) and rainfall for the Darwin Airport station (Australian Bureau of Meteorology 2023). We also sourced the following gridded (0.25° × 0.25°) ERA5 climate data (Hersbach et al. 2023): hourly temperature, dewpoint temperature, surface pressure, longitude vector (u) and latitude vector (v) components of wind, boundary layer height, and surface net solar radiation. We used the u and v components of wind to calculate wind speed. We then calculated daily mean values for all variables, and daily mean temperature and dewpoint temperature were used to calculate daily mean relative humidity.
We trained a random forest model in R (Breiman 2001) to predict daily PM2.5 for Darwin Airport by using meteorological variables, visibility, and month of the year as predictors. We assumed that PM2.5 observations for Darwin Airport were equivalent to the mean PM2.5 across the four monitoring sites in Darwin. Our modelling spanned 18 years (7 April 2004 to 30 December 2022), with a total of 6098 daily data points. We randomly split our data into training (80% of data) and testing (20% of data) sets, trained a model using the training set, and found an R-squared of 66.65% on the testing set. We used the random forest model to predict daily PM2.5 between 1 January 1960 and 30 December 2022. Given that we had some missing values for that period, we used the missRanger package in R to impute for missing values (Mayer 2023). Finally, we correlated PM2.5 concentrations in Darwin with the rest of the Top End of the Northern Territory, by using aerosol optical depth (AOD) remote-sensing estimates of gridded monthly PM2.5 from Van Donkelaar et al. (2021) aggregated at a 0.05° spatial resolution between January 1998 and December 2021. Statistically significant correlations in the early, late, and whole dry season were then mapped to understand the geographical patterns of air pollution across the Top End of the Northern Territory.
Fire-activity data
We obtained data for active fires and area burned from the Moderate Resolution Imaging Spectroradiometer (MODIS) sensors from the Terra and Aqua satellites (Giglio et al. 2021, 2022) from 2001 to 2022. We classified these data into project and non-project areas by using the geographic polygons defining carbon projects provided by the Australian Government (Australian Government Clean Energy Regulator 2023) (Fig. 1). Although strategic early dry-season fire management started at different times across the project areas, with the first commencing in 2006, for the purposes of the analyses, we pragmatically deemed the projects to have commenced in 2012 when the first ACCU projects were registered (Fig. 1c). This approach was necessary because no data are published on when the project activities commenced or even the formal project commencement date, only project registration dates. Project registration dates indicate only when the projects were registered under the legislation, not when they formally commenced or when the project activities commenced (projects were allowed to backdate their commencement dates until January 2011 and even the original methods were backdated until 2010). In the absence of better data, we assumed that all projects commenced in 2012, when the savanna fire-management methodology was fully operational (Meyer et al. 2012).
We analysed trends in area burned and the intensity of fire per hectare by using the MODIS Fire Radiative Power (FRP) product, available from the MODIS active fires data. We then used a generalised linear modelling (glm in R) to test to differences in fire intensity (measured by the sum of FRP) per hectare before and after the putative 2012 establishment year of the carbon projects for project and non-project areas and used the ggpredict function from the ggeffects package in R to generate predictions (Lüdecke 2018).
Fuel moisture
Fuel moisture index (FMI) was calculated following the method of Sharples et al. (2009) and Bowman et al. (2022a), by using gridded meteorological data from the Australian Gridded Climate Data (Evans et al. 2020) between 1 January 1990 and 31 December 2022, for the Top End region bounded by a box from a longitude 128°E to 137°E, and a latitude 16°S to 9°S. Daily maximum temperature and precipitation rasters were downloaded and resampled to 0.1° × 0.1° resolution, and FMI was calculated on a daily basis in each cell. We then used generalised linear modelling (glm function in R) to test for differences in FMI before and after the establishment of the carbon projects for project and non-project areas, and used the ggpredict function from the ggeffects package in R to generate predictions (Lüdecke 2018).
Results
Air pollution
The historically reconstructed monthly PM2.5 data in Darwin are closely correlated with both the instrumental record (r = 0.96) and the aerosol optical depth (AOD) derived estimates (r = 0.72) for the entire dry season (Fig. 2a), as well as the early dry season (r = 0.77) (Fig. 2b) and late dry season (r = 0.71) (Fig. 2c). For the historical record, there is no trend for whole dry season since 1960, but since the commencement of strategic early dry-season fire management activities in 2006 and the registration of the first ACCU projects in 2012 (Fig. 2a–c), there has been a slight increase in PM2.5 pollution in the early dry season and a corresponding decrease in late dry-season PM2.5 pollution.
Trends in PM2.5 in the Darwin airshed on the basis of instrumental, aerosol optical depth (AOD), and historical reconstruction for (a) whole dry season (May to October), (b) early dry season (May to July), and (c) late dry season (August to October). The trendline and 95th percentile confidence bounds (grey shading) are indicated, the commencement of strategic early dry-season fire-management activities in 2006 is indicated by a dashed red line, and the registration of the first ACCU projects in 2012 is indicated by a dashed green line.
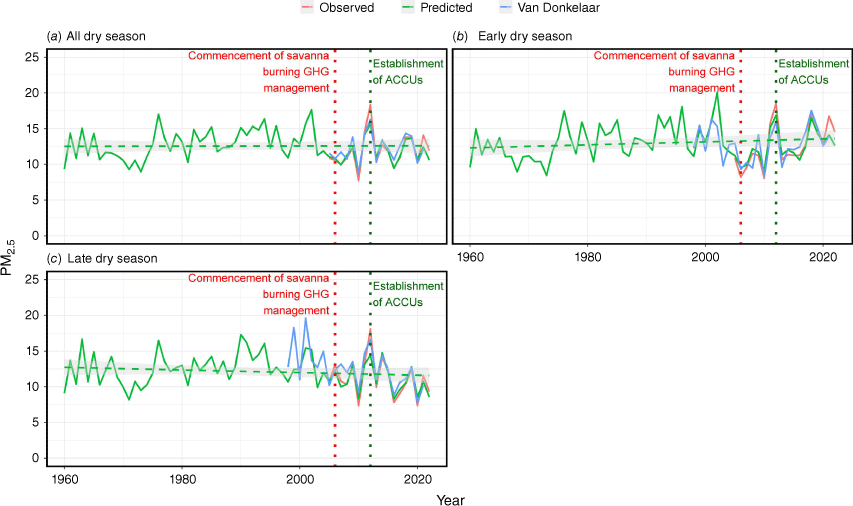
Dry-season particulate pollution is correlated with the pollution in the surrounding hinterland areas (Fig. 3a). In the early dry season, there was the strongest positive correlation (P < 0.01) between the mean monthly values of AOD-derived PM2.5 for Darwin and those for all 0.05° grid cells across the Top End (Fig. 3b). The distribution of grid cells with a significant correlation with Darwin’s PM2.5 was more geographically restricted to the region immediately surrounding Darwin in the late dry season (Fig. 3c).
Area burned and fire intensity
Within the available MODIS satellite record, the savanna-burning projects have reduced the the area burned in the dry season (Fig. 4a), with the clearest decline in late dry season and a corresponding, albeit smaller, increase in the early dry season (Fig. 4b, c). Notably, these trends are not apparent in areas that are outside the savanna carbon projects, which were subject to less burning both prior to and after the commencement of strategic early dry-season fire management activities (Fig. 4). Nonetheless, the mean fire intensity (FRP ha−1) was higher within carbon burning projects for the whole dry season, and the early and late dry seasons (Fig. 5a–c). Generalised linear modelling confirmed these differences, showing comparable fire intensity before the assumed establishment of the ACCU projects in 2012, with a marked increase thereafter, particularly on the project areas (Fig. 5d). Fig. 6 shows how fire intensity increases by month, and how the intensity curves are higher post-establishment of the ACCU projects compared to pre-establishment, with no differences between project and non-project areas.
Trends in percentage area burned within and outside carbon burning project areas in the Top End of the Northern Territory (10.5 million ha vs 12.6 million ha respectively). Burned area (ha) for (a) whole of dry-season, (b) burned area in early dry-season, and (c) burned area in late dry-season year, for project and non-project areas. The commencement of strategic early dry-season fire management activities in 2006 is indicated by a dashed red line, the registration of the first ACCU projects in 2012 is indicated by a dashed green line, and grey shading denotes the 95% confidence interval of the trend lines.
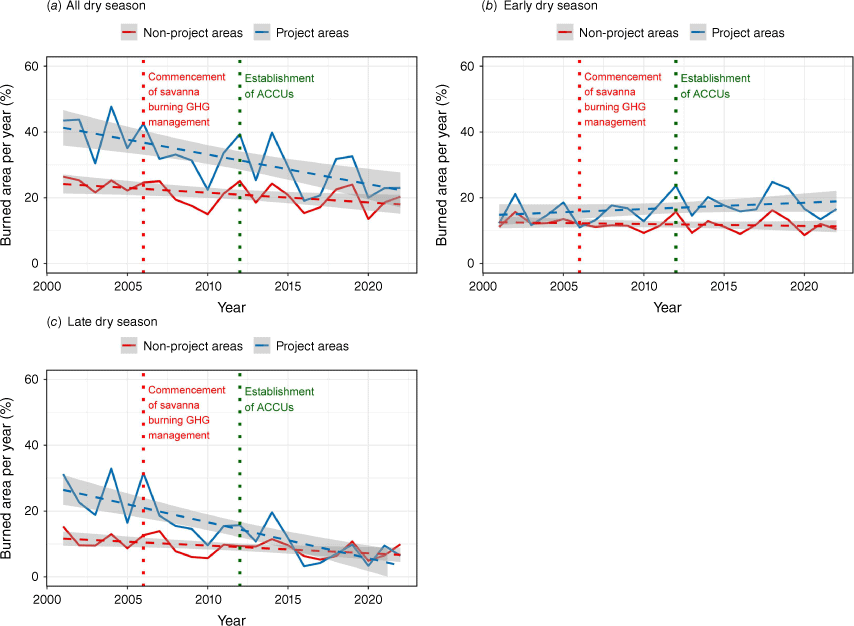
Yearly trends in mean fire intensity (FRP ha−1) in the Top End of the Northern Territory. Fire intensity (a) in whole of dry-season, (b) in early dry-season, (c) in late dry-season year for project and non-project areas, where the commencement of strategic early dry-season fire-management activities in 2006 is indicated by a dashed red line and grey shading denotes the 95% confidence interval of the trend lines. (d) Mean (and ±standard error) monthly fire intensity (FRP ha−1) for pre- and post establishment of ACCU projects (2012) between project and non-project areas on the basis of generalised linear model: fire intensity ~ pre/post × project area/non-project area + month with family = Gaussian(link = ‘log’). (e) Mean (and ± standard error) monthly fuel moisture index (FMI) for pre- and post-registration of ACCU projects (2012) between project and non-project areas on the basis of generalised linear models: FMI ~ pre/post × project area/non-project area + month.
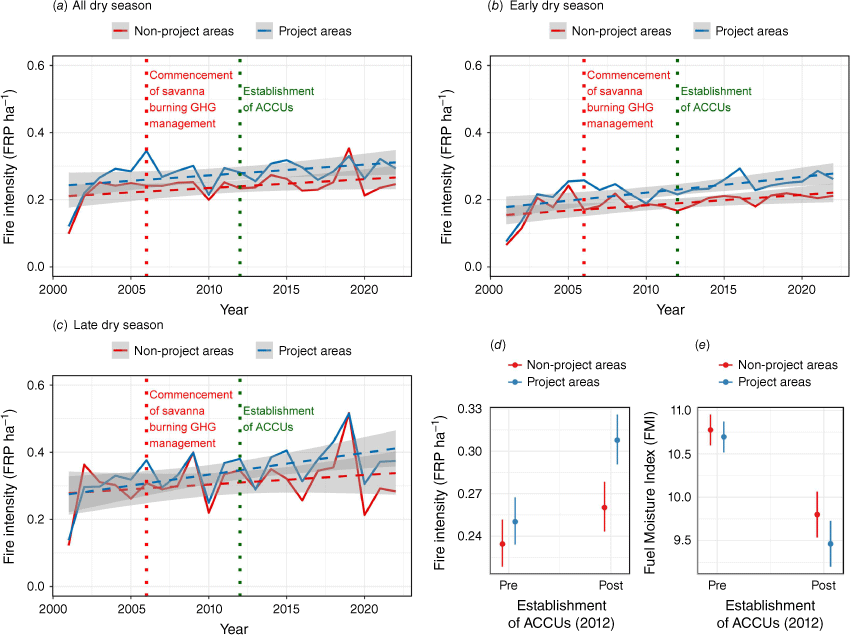
Monthly trends in fire intensity (FRP ha−1) and fuel moisture index (FMI) during the dry season (May to October) in the Top End of the Northern Territory. Fire intensity (a) before and (b) after 2012; fuel moisture index (c) before and (d) after 2012.
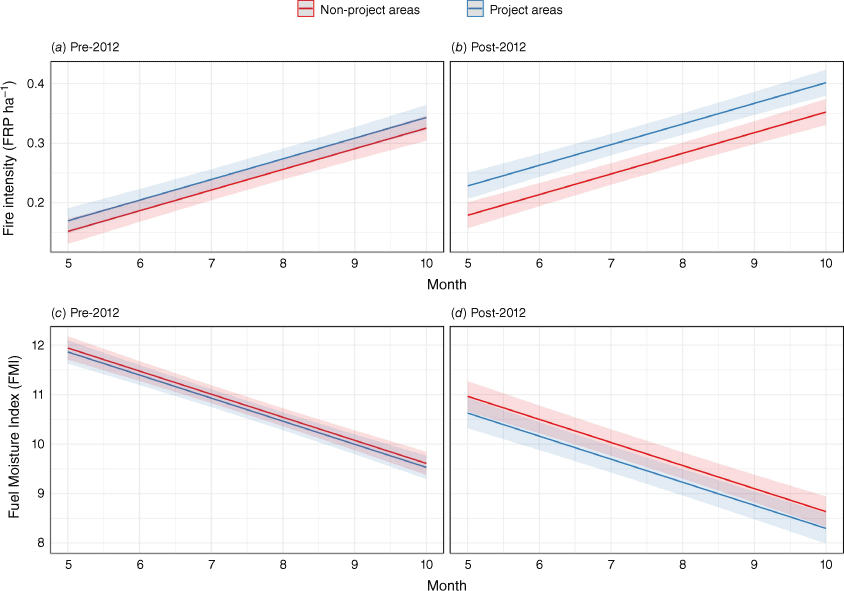
The divergence in the fire intensity on savanna carbon-project and non-project areas is apparent in Fig. 6a, which shows no difference prior to the registration of the ACCU projects (pragmatically assumed to be 2012) and a marked increase after their registration, with highest intensities on project areas. The increase in fire intensity means that fire intensities in August after the ACCU projects were first registered in 2012 were equivalent to the fire intensities in October before projects were first registered in 2012 (Fig. 6a, b). These monthly patterns are apparent, albeit in the opposite direction, for estimated fuel moisture (Fig. 6c, d), with a statistically significant inverse correlation between monthly fire intensity and monthly fuel moisture before and after the first registration of the ACCU projects in 2012 for savanna carbon project areas (−0.69 and −0.77 respectively) and for non-project areas (−0.55 and −0.64 respectively).
Discussion
This research was primarily motivated by a desire to understand the worsening air quality in Darwin, particularly in the early dry season, over the past two decades, coincident with the landscape-wide prescribed burning in the early dry season designed to reduce GHG emissions that commenced in 2006. Poor air quality in Darwin has manifest adverse impacts on human health demonstrated by numerous studies (Johnston et al. 2002, 2007; Hanigan et al. 2008; Campbell et al. 2022).
The savanna-burning carbon projects in the Top End of the Northern Territory have shifted burning to the early dry season, with a marked reduction in area burned in the late dry season (Fig. 4). However, this change is not evident on areas that are not managed for carbon abatement (Fig. 4). While we have shown that the proportion of the area burned in the late dry season has declined since the early 2000s, it is not clear that this period is representative of pre-project late dry-season fire activity. According to the NOAA Fire History Mapping dataset (Landgate 2023), used by the Australian Government to estimate savanna fire emissions in the national greenhouse accounts (Bowman et al. 2023), the beginning of the 21st century was characterised by high late dry-season fire activity in Northern Territory savannas that was significantly greater than the 1990 average. Such variability is likely to affect assumptions about the effectiveness of savanna burning projects in reducing dry-season fire extent, including our finding of a decline in area burned since the early 2000s (Fig. 4). Analysis of longer fire history mapping would help evaluate whether savanna burning projects have reduced dry-season fire extent and potentially explain the negligible change in the smoke pollution in Darwin since 1960 (Fig. 2).
A previous study by Jones et al. (2022) found that early dry-season burning in a 500 km radius from Darwin affected air pollution in Darwin between 2004 and 2019, and suggested a link with savanna-burning projects. Our study has extended the research of Jones et al. (2022) by providing a historical context to understand trends in particulate pollution in Darwin in the early, late, and whole dry season since 1960. We expected that air quality in Darwin would have improved following the implementation of the savanna-burning projects. However, we found that Darwin PM pollution in the early, but not late, dry season has worsened since the implementation of the savanna-burning projects (Fig. 2). Our geospatial analysis confirmed that Darwin air pollution seasonal patterns are a regional rather than local phenomenon, by demonstrating a strong correlation between Darwin particulate pollution with estimates of air pollution across the Top End (latitudes north of 16°S) of the Northern Territory (Fig. 3). The cause for the strong correlation is most likely to relate to the consistent prevailing south-eastern winds in the early dry season that would drive smoke towards Darwin (Jones et al. 2022).
Our analysis also identified that areas managed for carbon abatement have higher fire intensity compared to those that are not managed for carbon. Interestingly, this difference was marginal and statistically non-significant prior to the first registration of the ACCU projects in 2012, but has subsequently increased in magnitude and has become significantly different after their registration (Fig. 5d, e). The cause of the difference following the registration of the ACCU projects in 2012 remains unclear, but may reflect different patterns of burning. For instance, because financial benefit is derived from early dry-season fires, it is possible that the fixed date to define the end of the early dry season incentivises managers to burn as late as possible to increase the extent of ‘early’ dry-season fires and thereby reduce the risk of fires occurring in the late dry season. Frequent burning is known to increase the abundance of flammable grasses in Northern Territory savannas (Bowman et al. 2007b, 2014), creating fuel beds that burn intensely (Prior et al. 2017). Hence, repeated burning associated with carbon projects may have changed vegetation characteristics and fuel load, leading to more intense fires, including initiating a grass-fire cycle (D’Antonio and Vitousek 1992).
Our research also found a credible linkage between fire intensity and fuel dryness that can also contribute to worsening air quality in the early dry season (Fig. 6). There is a global pattern of declining fuel moisture caused by hotter and drier conditions associated with anthropogenic climate change (Ellis et al. 2022). Drier fuels are causing larger and more intense fires nationally and globally (Canadell et al. 2021; Ellis et al. 2022). A trend toward drier fuels highlights the vulnerability to climate change of fire management interventions designed to reduce GHG pollution (Bowman et al. 2023). The current savanna burning carbon method assumes fixed emissions per unit area, rigidly defined by early or late dry-season periods on the basis of historical observational data from different vegetation types (Meyer et al. 2012; Australian Government 2018a, 2018b; Department of Climate Change 2023). Our analysis has shown that the assumption of an early and late dry-season dichotomy in emissions owing to differing fuel consumption is likely to be flawed, given the strong decline in dry-season monthly fuel moisture that influences fire intensity.
From the data produced in this study, it is not possible to reach definitive conclusions on whether, and the extent to which, the savanna burning projects in the Northern Territory have reduced GHG emissions. The study was intended to explore the causes of observed worsening air quality in Darwin, not to evaluate the efficacy of savanna burning projects in abating emissions. Nonetheless, the negligible overall trend in Darwin PM2.5 pollution since the 1960s, albeit with a shift from late to early dry season, suggests that the emission reductions generated by the projects may be small and that projects may have been over-credited (i.e. the number of carbon credits may significantly exceed the actual emission reductions).
Further research is warranted to better understand the effects of changing dry-season fuel moisture on GHG emissions associated with changing fuel loads, flaming versus smouldering combustion and fuel consumption, particularly in coarse woody debris (Volkova et al. 2019). Such research should specifically test the finding that there is negligible difference in emissions factors between early and the late dry season in Australia’s savannas, which was reported by Meyer et al. (2012), and which is relied on in the savanna burning carbon method to estimate abatement (Australian Government 2018a, 2018b; Department of Climate Change 2023). Also required is an improved understanding of the proportion of available fuel that is burned at a landscape scale, as a function of fuel moisture (e.g. the patchiness of fires, the consumption of different fuel components and associated emissions) (Desservettaz et al. 2017). Such further research necessarily demands a mix of further field, laboratory, and fine-resolution remote-sensing studies. In this context, it is important to note that current quantitative assessments of GHG benefits from savanna burning projects hinge on broadscale remote-sensing assessment with limited field validation (Edwards et al. 2021).
The current savanna carbon projects appear to be maladaptive to climate change, being rigid in their application and unable to accommodate natural variability in dry-season onset and length, as well as the effects of climate change on fuel moisture and fire intensity. The legislative focus on changing the season of burning is likely to have diverted attention away from determining whether GHG emission have been reduced by the savanna burning programs. Furthermore, the projects may have other maladaptive aspects such as causing unintended environmental and social adverse effects (Schipper 2020, 2022), including worsening early dry-season air pollution and human health impacts (Jones et al. 2022), loss of biodiversity (Corey et al. 2020) and cultural disruptions (Petty et al. 2015). To be more adaptive, the savanna burning projects should have greater flexibility in the definition of the end of the dry season, for example, by measuring changes in fuel moisture. An additional maladaptive aspect of the current savanna carbon projects is that they are not restoring traditional Aboriginal patch burning. For example, Bowman et al. (2022b) found that the commencement of strategic early dry-season fire management activities in central Arnhem Land in 2006 was associated with a population decline of the comparatively fire-sensitive savanna conifer Callitris intratropica. Arguably, a more adaptative approach to reducing GHG emissions from savannas would be to increase the amount of long unburned vegetation through careful patch burning more closely aligned with traditional Aboriginal practices, an approach that would most likely have benefits for biodiversity (Trauernicht et al. 2015).
More broadly, our study has highlighted the need to carefully evaluate the likely positive and negative social and environmental effects of applying extending savanna burning carbon abatement projects to savanna landscapes outside Australia (Lipsett-Moore et al. 2018; Laris 2021). Key issues that must be addressed include the feasibility of defining early and late dry season for burning (Laris et al. 2017), understanding how particulate and GHG emission factors vary among seasons and among fuel types (Laris et al. 2023), and integrating Indigenous knowledge and burning practices into project design (Laris et al. 2017). Proponents of savanna burning carbon abatement projects must demonstrate that there are viable ways of ensuring that GHG abatement is not over-credited and of mitigating their potentially maladaptive effects, including adverse ecological impacts and environmental harms such as smoke pollution that we have demonstrated in this study (Jones et al. 2022).
Conclusions
We have shown that the current approach to GHG mitigation using the early dry-season savanna burning method has caused increased smoke pollution in the Northern Territory capital Darwin, especially in the early dry season, when south-easterly winds transport smoke from savannas that surround Darwin. The cause of the worsening smoke pollution is most likely a combination of climate change making fuels drier in the early dry season and, hence, causing more intense fires, as well as possible management effects associated with the implementation of savanna burning projects. We suggest the ACCU scheme savanna burning carbon method is maladaptive, given it relies on a fixed definition of the end of the early dry season and an assumption that fires are less intense and polluting at this time. To be more effective, and truly adaptive to climate change, savanna carbon schemes must be flexible and responsive to environmental changes, particularly those caused by climate change such as fuel moisture and fire intensity.
Data availability
The data that support the findings of this study were derived from various online remote-sensing, climatological, meteorological and Australian Government databases. The data used in the analyses are available from the corresponding author, DMJSB, upon reasonable request.
Declaration of funding
This research was partly funded by the Australian Research Council Laureate Fellowship, Grant number FL220100099 awarded to DMJSB.
Acknowledgements
We acknowledge the diligence of numerous workers assembling and maintaining invaluable data sets upon which this paper is based and the helpful comments from two anonymous reviewers.
References
Australian Bureau of Meteorology (2023) Climate Data Online. Available at http://www.bom.gov.au/climate/data/ [accessed 30 October 2023]
Australian Government Clean Energy Regulator (2023) About the ACCU Scheme. Available at https://www.cleanenergyregulator.gov.au/ERF/About-the-Emissions-Reduction-Fund [accessed 30 October 2023]
Bowman DMJS, Dingle JK, Johnston FH, Parry D, Foley M (2007a) Seasonal patterns in biomass smoke pollution and the mid 20th‐century transition from Aboriginal to European fire management in northern Australia. Global Ecology and Biogeography 16(2), 246-256.
| Crossref | Google Scholar |
Bowman DMJS, Franklin DC, Price OF, Brook BW (2007b) Land management affects grass biomass in the Eucalyptus tetrodonta savannas of monsoonal Australia. Austral Ecology 32(4), 446-452.
| Crossref | Google Scholar |
Bowman DMJS, MacDermott HJ, Nichols SC, Murphy BP (2014) A grass-fire cycle eliminates an obligate‐seeding tree in a tropical savanna. Ecology and Evolution 4(21), 4185-4194.
| Crossref | Google Scholar | PubMed |
Bowman DMJS, Furlaud JM, Porter M, Williamson GJ (2022a) The fuel moisture index based on understorey Hygrochron iButton humidity and temperature measurements reliably predicts fine fuel moisture content in Tasmanian Eucalyptus forests. Fire 5(5), 130.
| Crossref | Google Scholar |
Bowman DMJS, Williamson GJ, Johnston FH, Bowman C, Murphy BP, Roos CI, Trauernicht C, Rostron J, Prior LD (2022b) Population collapse of a Gondwanan conifer follows the loss of Indigenous fire regimes in a northern Australian savanna. Scientific Reports 12(1), 9081.
| Crossref | Google Scholar | PubMed |
Bowman DMJS, Williamson GJ, Ndalila M, Roxburgh SH, Suitor S, Keenan RJ (2023) Wildfire national carbon accounting: how natural and anthropogenic landscape fires emissions are treated in the 2020 Australian government greenhouse gas accounts report to the UNFCCC. Carbon Balance and Management 18(1), 14.
| Crossref | Google Scholar | PubMed |
Breiman L (2001) Random forests. Machine Learning 45(1), 5-32.
| Crossref | Google Scholar |
Campbell SL, Anderson CC, Wheeler AJ, Cook S, Muster T, Johnston FH (2022) Managing extreme heat and smoke: a focus group study of vulnerable people in Darwin, Australia. Sustainability 14(21), 13805.
| Crossref | Google Scholar |
Canadell JG, Meyer C, Cook GD, Dowdy A, Briggs PR, Knauer J, Pepler A, Haverd V (2021) Multi-decadal increase of forest burned area in Australia is linked to climate change. Nature Communications 12(1), 6921.
| Crossref | Google Scholar | PubMed |
Corey B, Andersen AN, Legge S, Woinarski JCZ, Radford IJ, Perry JJ (2020) Better biodiversity accounting is needed to prevent bioperversity and maximize co‐benefits from savanna burning. Conservation Letters 13(1), e12685.
| Crossref | Google Scholar |
D’Antonio CM, Vitousek PM (1992) Biological invasions by exotic grasses, the grass/fire cycle, and global change. Annual Review of Ecology and Systematics 23(1), 63-87.
| Crossref | Google Scholar |
Desservettaz M, Paton‐Walsh C, Griffith DWT, Kettlewell G, Keywood MD, Vanderschoot MV, Ward J, Mallet MD, Milic A, Miljevic B, Ristovski ZD, Howard D, Edwards GC, Atkinson B (2017) Emission factors of trace gases and particles from tropical savanna fires in Australia. Journal of Geophysical Research: Atmospheres 122(11), 6059-6074.
| Crossref | Google Scholar |
Edwards A, Archer R, De Bruyn P, Evans J, Lewis B, Vigilante T, Whyte S, Russell-Smith J (2021) Transforming fire management in northern Australia through successful implementation of savanna burning emissions reductions projects. Journal of Environmental Management 290, 112568.
| Crossref | Google Scholar | PubMed |
Ellis TM, Bowman DMJS, Jain P, Flannigan MD, Williamson GJ (2022) Global increase in wildfire risk due to climate‐driven declines in fuel moisture. Global Change Biology 28(4), 1544-1559.
| Crossref | Google Scholar | PubMed |
Giglio L, Schroeder W, Hall JV (2021) MODIS Collection 6 and Collection 6.1. Active Fire Product User’s Guide. Report. (NASA: Washington, DC, USA) https://modis‐fire.umd.edu/files/MODIS_C6_C6.1_Fire_User_Guide_1.0.pdf
Giglio L, Humber M, Hall JV, Argueta F, Boschetti L, Roy D (2022) Collection 6.1 MODIS Burned Area Product User’s Guide. Version 1. Report. (NASA: Washington, DC, USA) https://modis‐fire.umd.edu/files/MODIS_C61_BA_User_Guide_1.1.pdf
Hanigan IC, Johnston FH, Morgan GG (2008) Vegetation fire smoke, indigenous status and cardio-respiratory hospital admissions in Darwin, Australia, 1996–2005: a time-series study. Environmental Health 7, 42.
| Crossref | Google Scholar | PubMed |
Johnston FH, Kavanagh AM, Bowman DMJS, Scott RK (2002) Exposure to bushfire smoke and asthma: an ecological study. Medical Journal of Australia 176(11), 535-538.
| Crossref | Google Scholar | PubMed |
Johnston FH, Bailie RS, Pilotto LS, Hanigan IC (2007) Ambient biomass smoke and cardio-respiratory hospital admissions in Darwin, Australia. BMC Public Health 7, 240.
| Crossref | Google Scholar | PubMed |
Jones PJ, Furlaud JM, Williamson GJ, Johnston FH, Bowman DMJS (2022) Smoke pollution must be part of the savanna fire management equation: a case study from Darwin, Australia. Ambio 51(11), 2214-2226.
| Crossref | Google Scholar | PubMed |
Keenan TF, Williams CA (2018) The terrestrial carbon sink. Annual Review of Environment and Resources 43, 219-243.
| Crossref | Google Scholar |
Landgate (2023) NOAA Fire History Mapping. Available at https://catalogue.data.wa.gov.au/dataset/noaa-fire-history-mapping
Laris P (2021) On the problems and promises of savanna fire regime change. Nature Communications 12(1), 4891.
| Crossref | Google Scholar | PubMed |
Laris P, Koné M, Dadashi S, Dembele F (2017) The early/late fire dichotomy: time for a reassessment of Aubréville’s savanna fire experiments. Progress in Physical Geography 41(1), 68-94.
| Crossref | Google Scholar |
Laris P, Koné M, Dembélé F, Rodrigue CM, Yang L, Jacobs R, Laris Q, Camara F (2023) The pyrogeography of methane emissions from seasonal mosaic burning regimes in a West African landscape. Fire 6(2), 52.
| Crossref | Google Scholar |
Lipsett-Moore GJ, Wolff NH, Game ET (2018) Emissions mitigation opportunities for savanna countries from early dry season fire management. Nature Communications 9(1), 2247.
| Crossref | Google Scholar | PubMed |
Lüdecke D (2018) ggeffects: tidy data frames of marginal effects from regression models. Journal of Open Source Software 3(26), 772.
| Crossref | Google Scholar |
Mayer M (2023) missRanger: Fast Imputation of Missing Values. R package version 2.3.0. Available at https://CRAN.R-project.org/package=missRanger [accessed 30 October 2023]
Meyer CP, Cook GD, Reisen F, Smith TEL, Tattaris M, Russell‐Smith J, Maier SW, Yates CP, Wooster MJ (2012) Direct measurements of the seasonality of emission factors from savanna fires in northern Australia. Journal of Geophysical Research: Atmospheres 117(D20), D20305.
| Crossref | Google Scholar |
Northern Territory Environment Protection Authority (2023) AQI Summary. Available at http://ntepa.webhop.net/NTEPA/Default.ltr.aspx [accessed 30 October 2023]
Petty AM, DeKoninck V, Orlove B (2015) Cleaning, protecting, or abating? Making indigenous fire management ‘work’ in northern Australia. Journal of Ethnobiology 35(1), 140-162.
| Crossref | Google Scholar |
Prior LD, Murphy BP, Williamson GJ, Cochrane MA, Jolly WM, Bowman DMJS (2017) Does inherent flammability of grass and litter fuels contribute to continental patterns of landscape fire activity? Journal of Biogeography 44(6), 1225-1238.
| Crossref | Google Scholar |
Reisen F, Meyer CP, Weston CJ, Volkova L (2018) Ground‐based field measurements of PM2. 5 emission factors from flaming and smoldering combustion in eucalypt forests. Journal of Geophysical Research: Atmospheres 123(15), 8301-8314.
| Crossref | Google Scholar |
Schipper ELF (2020) Maladaptation: when adaptation to climate change goes very wrong. One Earth 3(4), 409-414.
| Crossref | Google Scholar |
Schipper ELF (2022) Catching maladaptation before it happens. Nature Climate Change 12(7), 617-618.
| Crossref | Google Scholar |
Sharples JJ, McRae RH, Weber R, Gill AM (2009) A simple index for assessing fuel moisture content. Environmental Modelling & Software 24(5), 637-646.
| Crossref | Google Scholar |
Trauernicht C, Brook BW, Murphy BP, Williamson GJ, Bowman DMJS (2015) Local and global pyrogeographic evidence that indigenous fire management creates pyrodiversity. Ecology and Evolution 5(9), 1908-1918.
| Crossref | Google Scholar | PubMed |
Van Der Werf GR, Randerson JT, Giglio L, Van Leeuwen TT, Chen Y, Rogers BM, Mu M, Van Marle MJE, Morton DC, Collatz GJ, Yokelson RJ, Kasibhatla PS (2017) Global fire emissions estimates during 1997–2016. Earth System Science Data 9(2), 697-720.
| Crossref | Google Scholar |
Van Donkelaar A, Hammer MS, Bindle L, Brauer M, Brook JR, Garay MJ, Hsu NC, Kalashnikova OV, Kahn RA, Lee C, Levy RC, Lyapustin A, Sayer AM, Martin RV (2021) Monthly global estimates of fine particulate matter and their uncertainty. Environmental Science & Technology 55(22), 15287-15300.
| Crossref | Google Scholar | PubMed |
Vardoulakis S, Jalaludin BB, Morgan GG, Hanigan IC, Johnston FH (2020) Bushfire smoke: urgent need for a national health protection strategy. Medical Journal of Australia 212(8), 349-353.e1.
| Crossref | Google Scholar | PubMed |
Volkova L, Roxburgh SH, Surawski NC, Meyer CM, Weston CJ (2019) Improving reporting of national greenhouse gas emissions from forest fires for emission reduction benefits: an example from Australia. Environmental Science & Policy 94, 49-62.
| Crossref | Google Scholar |