An economic analysis of cell-fencing in semi-arid rangelands
Stuart J. Dawson

A
B
C
D
E Present Address:
Abstract
In Australia, livestock predation by dingoes (Canis familiaris) has contributed to what some livestock producers consider a dire situation for rangeland pastoralism, driving demand for cooperative regional-scale exclusion (‘cell’) fencing (i.e. pest-proof fences that encompass one or more individual properties) and landscape-scale predator control. The present case study predicted the effect of four cell-fences in the state of Western Australia (WA) on the gross margin of sheep (for meat or meat and wool) and cattle pastoral enterprises. We modelled the potential effects of the following four key variables: (1) four levels of commodity prices, (2) five levels of livestock weaning rate (based on livestock records collected 1985–1995; weaning rate is defined as number of lambs or calves that are born and survive to weaning, expressed as percentage of total mated females), (3) three predicted levels of time required to remove dingoes from within the fenced area, and (4) five levels of macropod (mainly kangaroo) response as competitive grazers, with a total of 3600 scenarios representing all combinations of these factors. Each scenario was assessed for profitability (i.e. net present value (NPV) over 25 years) and benefit of fencing (i.e. NPV compared with an unfenced enterprise of the same livestock type, region, and commodity prices). Finally, the benefit–cost ratio (BCR) of investment in cell fencing was calculated for each fenced scenario. The majority (67%) of scenarios representing continuation of current management (i.e. no cell fencing) returned a negative NPV (i.e. livestock enterprises were projected to make a loss). However, only 37.4% of cell-fenced scenarios returned a positive NPV, meaning that even with a cell-fence and successful removal of dingoes, the enterprise was still unlikely to be profitable. Only 43.4% of cell-fenced scenarios returned a BCR of cell fencing greater than one. Weaning rate following dingo removal was the most important factor determining return on investment for cell-fencing. Survival and reproduction of small livestock, particularly wool sheep, benefit most from cell-fencing, whereas cell-fencing and dingo removal did not result in greater profits for cattle enterprises. Running sheep for wool and meat within cell fencing coupled with removal of dingoes would maximise the likelihood of achieving a positive return on investment in cell fencing (although the enterprise may remain unprofitable overall); otherwise, unfenced enterprises affected by dingoes should run cattle as this will be more profitable.
Keywords: cattle, cluster fencing, cost–benefit analysis, dingo, exclusion, pastoral, predator, sheep, wild dog.
Introduction
Globally, fencing is one of the most widely used methods to reduce livestock predation (Miller et al. 2016; Moreira-Arce et al. 2018; van Eeden et al. 2018a; Khorozyan and Waltert 2019). At the smallest scale, animals can be fenced together in a small area (‘corralled’) at night, when predators are most active (Bauer et al. 2010). Alternatively, predator-proof fences can be erected to exclude predators from entire grazing areas (Smith et al. 2020a). The success of fencing is contextually dependant, and must be targeted to the relevant livestock, predator species, and agricultural system (Eklund et al. 2017).
In Australia, dingoes (Canis familiaris) commonly prey on livestock in many areas (Fleming et al. 2022). Dingo predation can have catastrophic effects on sheep enterprises, killing lambs and adults, as well as causing abandonment of young (Thomson 1984; Allen and Sparkes 2001; Fleming et al. 2001). Consequently, sheep production is considered by many to be mutually exclusive with their presence (Thomson 1986; Corbett 1995; Allen and Sparkes 2001; Fleming et al. 2001; Newsome et al. 2001b; Allen and Fleming 2004). The effect of predation on cattle is less pronounced, with estimates of predation of calves ranging from 1% to 7% (Eldridge and Bryan 1995; Hewitt 2009), although in extreme cases, it may be as high as 32% (Allen 2014). Broadscale predator control through lethal and non-lethal methods remains commonplace throughout the pastoral rangelands of Australia (Van Eeden et al. 2018b); however, dingo control as applied is not always associated with reduced livestock losses (Allen 2014; Campbell et al. 2019).
Two fences across the south-east (the ‘dingo barrier fence’) and south-west (the ‘state barrier fence’; Fig. 1) of the Australian continent are intended to exclude dingoes from the agricultural regions (McKnight 1969; Newsome et al. 2001a; Pacioni et al. 2018). Although dingoes are present ‘inside’ these fenced areas, dingo density is lower than outside the fence, with genetic evidence suggesting that these fences are effective barriers to gene flow (Stephens et al. 2023). Further, biogeographic and agricultural management differences notwithstanding, the persistence of small livestock (i.e. sheep and goats) grazing enterprises within fenced areas in agricultural regions, compared with their decline in pastoral regions, suggests that these fences are important in protecting livestock industries. Consequently, livestock producers, many outside agricultural areas (i.e. the pastoral rangelands), have begun erecting predator-proof fences, known as ‘cluster fences’ or ‘cell fences’, which generally encompass a group of individual properties (Smith et al. 2020a; Pacioni et al. 2021a, 2021b). Cell-fences are intended to allow properties to manage vertebrate pests, usually dingoes, goats, or macropods, while excluding reinvading individuals (fencing is rarely completely impermeable, depending on the type of fence construction, age, and maintenance; Fig. 2). Because cell-fences ‘isolate’ a property or group of properties, effective management of any pests is reliant on coordinated and cooperative management between individual managers within the cell.
Map of the four Southern Rangelands cell-fences (green areas) in Western Australia, in the Gascoyne, Goldfields, and a large and nested small cell-fence in the Murchison region. The bold black line indicates the state barrier fence, and the grey areas indicate pastoral leases. Inset indicates the location of the map at the continental scale.
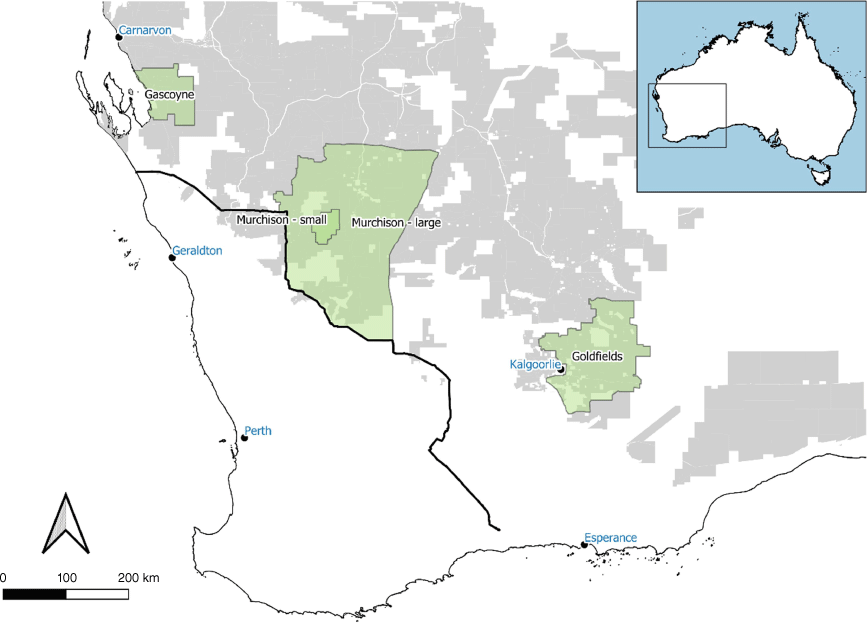
An example of the Goldfields cell fence, indicating the ‘lap wire’ used to prevent animals digging or pushing under the fence.
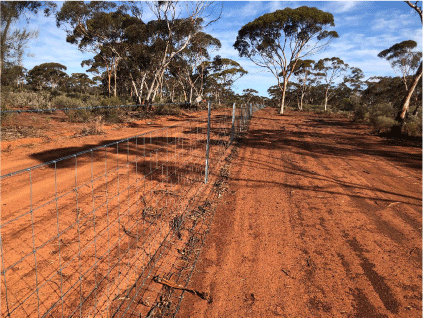
Fencing is expensive to erect and maintain and, depending on the fence type, can have unintended consequences that limit its utility (Kolowski and Holekamp 2006; Hayward and Kerley 2009; Bradby et al. 2014). For example, large macropods (Osphranter spp. and Macropus spp.) occur in all grazing regions of Australia, where they contribute to total grazing pressure to varying degrees (Pahl 2020b). Some studies have suggested that a reduction in dingo density results in increased macropod density (Caughley et al. 1980; Pople et al. 2000; Dawson et al. 2023), which increases competition with livestock for food and potentially reduces livestock productivity (Allen 2015; Prowse et al. 2015; Campbell et al. 2022).
In the Southern Rangelands of Western Australia (WA), four cell-fences are under construction or have recently been completed. Importantly, three of these four cell-fences encompass a larger area than any other cell-fences constructed in Australia. The demand for effective dingo control is high among many pastoralists; however, the economic benefits from cell fencing to pastoral enterprises are highly speculative (URS 2009; Petersen and Cooke 2016). The aim of the present study was to use a scenario-based modelling approach to investigate projected economic outcomes of these four cell-fence projects and identify the key factors that influence potential net benefits for pastoral enterprises. Although rarely investigated, quantifying the return on investment is fundamental to supporting wildlife management actions that are sustainable and effective (Choquenot and Hone 2000), and outcomes for this exercise could prove informative for proposed cell fencing elsewhere.
In the present study, we ran multiple scenarios to compare the economic state of a pastoral enterprise with a cell fence (fenced scenarios; Fig. 3) to a matched enterprise with no cell-fence (unfenced base-case scenarios; Fig. 3). We explored the variation among regions as well as differences among livestock types (cattle, meat sheep, wool sheep), and modelled the impact of key areas of uncertainty, namely, commodity prices, variation in livestock weaning rate (defined as number of lambs or calves that are born and survive to weaning, expressed as a percentage of total mated females), effectiveness of dingo control, and the response of macropods to dingo removal (Fig. 3). We compared different scenarios using two measures, namely (1) ‘profitability’, which is whether a given scenario returns a positive net present value (i.e. the income generated over 25 years greater than the cost, discounted at 5%), and (2) ‘benefit of fencing’, which is the difference in NPV between a pastoral enterprise with a cell fence and the matched enterprise without fencing (unfenced base-case scenario).
Methods
We modelled a pastoral enterprise for each of the four cell-fences (herein ‘regions’; Fig. 1). Models representing each of the four regions were parameterised using the present carrying capacity and property size averaged across all pastoral properties in the region, and estimated macropod density for that region (see details below). In the unfenced models, there was no cost for cell-fence construction or maintenance, investment in pest control remained constant at current levels, and the livestock metrics were modelled assuming current values (Fig. 3). The net present value (NPV), and benefit–cost ratio (BCR) were calculated over a 25-year period by using a 5% discount rate (New South Wales Treasury 2023). All historical values have been converted to 2020 Australian dollars, by using the consumer price index reported for June 2021 (Australian Bureau of Statistics 2021).
Models were created for each of the three livestock types (cattle, meat sheep, wool sheep); pastoral properties with a mix of livestock were not modelled. In addition, given the decline in goat density in WA (Bell 2015), goat production was also excluded from modelling. Livestock and macropods were assumed to be steady state (i.e. no seasonal or stochastic variation in macropod density and livestock production). As a result, input parameters were averaged over ‘good’ and ‘poor’ years to capture the temporal variation present.
The cell-fence projects described in the current study have received government financial support to varying degrees. Subsidisation of cell-fencing initiatives reduces the required investment by individual livestock enterprises, increasing the NPV and BCR achieved by individual stations. However, our analysis aimed to assess the benefits to livestock enterprises of investment in cell-fencing, not simply landholder investment in cell-fencing. Therefore, all investment, regardless of source, was considered together as an input, and the livestock produced was considered an output. Similarly, many pastoralists have additional sources of income, which were excluded from all analysis.
Modelling approach
Data used to parameterise models were derived from multiple sources, including published literature, landholder interviews, and a range of government, consultant, and private reports. The Pastoral Lands Board (PLB) is a statutory authority that administers pastoral leases in WA. Pastoral lease managers are required to provide an annual return to the PLB, detailing the number of livestock present, as well as pest species and damages caused by pests. De-identified data (1985–2021) were provided under licence by the PLB. To calculate regional averages, only pastoral enterprises situated inside or within 50 km of the four cell-fence areas were included. The 50 km buffer was included to gain the most representative regional average for livestock production and was particularly important for smaller cells containing only a few properties.
Average property size and present carrying capacity (averaged enterprises inside or within 50 km of the four cell-fence areas) were derived from PLB data based on the most recent assessment of lease condition (Table 1). The present carrying capacity is based on the land systems present and their range condition, and is assessed by pastoral lease inspectors at regular intervals (e.g. Hennig 2009). Of the properties for which data were included in the current study, 96% had their condition assessed and present carrying capacity revised after 2000. However, none of the assessments was more recent than 2009; the average time since the last inspection was 16 years.
Item | Gascoyne | Goldfields | Murchison – large | Murchison – small | Reference | |
---|---|---|---|---|---|---|
General | Incomplete | Incomplete | Completed 2021 | Completion due 2025 | ||
Total area of cell (km2) | 8454 | 24409 | 68,731 | 2188 | ||
Number of properties | 6 | 12 | 53 | 4 | ||
Average property size (km2) | 1395 | 1790 | 1217 | 1221 | PLB | |
Average carrying capacity per property (DSE) | 9351 | 9655 | 6479 | 6804 | DPIRD | |
Macropod density (individuals per km2) | 0.55 | 1.04 | 2.19 | 2.37 | DBCA; Dawson et al. (2023) | |
Macropod per property (DSE), @ 0.6 DSE per individual | 462 | 1118 | 1598 | 1736 | Pahl (2020a) | |
Dingo control | ||||||
Length of new cell-fence (km per property) | 60.1 | 79.2 | 5.4 | 57.5 | ||
Total cost of cell-fence ($ per km) | $6500 | $10,000 | $12,000 | $12,000 | RBG EOs | |
Cost of cell-fence per property ($) | $390,704 | $792,392 | $64,528 | $690,210 | ||
Length of fence to be maintained (km) | 360.7 | 950.9 | 869.2 | 230.1 | ||
Fence maintenance cost per property per year ($) | $4207 | $5546 | $1132 | $4026 | RBG EOs (@ $70/km/year) | |
Discounted total cost of fence construction and maintenance ($, over 25 years) | $428,577 | $829,111 | $76,651 | $711,386 | ||
Estimated time required to remove dingoes (years) | 5 | 7 | 7 | 5 | Adapted from Pacioni et al. (2021b) | |
Current baiting cost per property per year ($) | $1200 | $4004 | $445 | $445 | RBG EOs | |
Current LPMT cost per property per year ($) | $8466 | $4166 | $6385 | $6385 | RBG EOs | |
Current landholder dingo control input per property per year ($) | $6000 | $6000 | $6000 | $6000 | Targeted interviews | |
Cattle | ||||||
Initial herd size | 3206 | 870 | 666 | 666 | PLB return (2010–2019) | |
Branding rate – current (%) | 41 | 20 | 29 | 29 | PLB return (2015–2019) | |
Expected branding rate if dingoes are removed (%) | 52 | 37 | 32 | 32 | PLB return (1985–1995) | |
Adult mortality from dingo attack – current (%) | 0 | 0 | 0 | 0 | Targeted interviews | |
Meat sheep | ||||||
Initial herd size | 6289 | 238 | 4988 | 4988 | PLB return (2010–2019) | |
Marking rate – current (%) | 20 | 22 | 20 | 20 | PLB return (2015–2019) | |
Expected marking rate if dingoes are removed (%) | 45 | 45 | 45 | 45 | Experimental value, no existing data | |
Adult mortality from dingo attack – current (%) | 15 | 15 | 15 | 15 | Petersen and Cooke (2016), Thomson (1984) | |
Wool sheep | ||||||
Initial herd size | 3512 | 584 | 1587 | 3018 | Mean herd size 2010–2019 | |
Marking rate – current (%) | 17 | 22 | 12 | 12 | PLB return (2015–2019) | |
Expected marking rate if dingoes are removed (%) | 33 | 35 | 35 | 36 | PLB return (1985–1995) | |
Adult mortality from dingo attack – current (%) | 15 | 15 | 15 | 15 | Petersen and Cooke (2016) |
Abbreviations: DSE, dry sheep equivalent; LPMTs, licensed pest-management technicians; PLB, Pastoral Lands Board; DPIRD, Department of Primary Industries and Regional Development; DBCA, Department of Biodiversity, Conservation and Attractions; MRVC, Murchison Region Vermin Cell; RBG EOs, Recognised Biosecurity Group Executive Officers.
The four cell-fences are associated with three regional Recognised Biosecurity Groups (RBGs), community-led organisations funded by ratepayers and government to coordinate and deliver control of declared pests. The Executive Officers of each RBG provided input on dingo management and fencing costs. To quantify livestock practices and husbandry costs, 14 targeted interviews were conducted with managers of properties situated within, or in close proximity to, the four cell fences. Producers with cattle or sheep enterprises were surveyed; both production systems were not necessarily available in each region.
The livestock model used an age-structured herd matrix (based on the approach used by Wicks et al. 2014) and using PLB data supplemented with 14 targeted interviews with pastoralists (Tables 1, 2, Supplementary Table S1). The herd was split into the breeding herd (heifers and cows, maiden ewes and ewes), and the non-breeding herd (steers and breeding bulls, or wethers and breeding rams, Supplementary Table S1). We calculated the number of breeding females of Age 1 in the next year (t + 1), using the number of animals born, combined with re-stocker females (rs; heifers and ewes), less the animals turned off (sl), as follows:
where
t is the time period (years since fence completion)
number of animals of Type s in Cohort a
number of animals of Type s in Cohort a turned off
s is the livestock type, breeding (b) or non-breeding (nb)
rs number of re-stocker breeding females purchased
α is the weaning rate
Item | Unit | Cattle | Meat sheep | Wool sheep | Reference | |||
---|---|---|---|---|---|---|---|---|
Livestock sale price | ||||||||
Breeding herd <2 years | $ per head | $956.89 | $107.96 | $107.96 | MLA Saleyard data (mean 2015–2019) | |||
Breeding herd >2 years | $ per head | $982.66 | $88.11 | $88.11 | MLA Saleyard data (mean 2015–2019) | |||
Non-breeding herd <2 years | $ per head | $956.89 | $107.96 | $107.96 | MLA Saleyard data (mean 2015–2019) | |||
Non-breeding herd >2 years | $ per head | $1148.72 | $88.11 | $88.11 | MLA Saleyard data (mean 2015–2019) | |||
Purchase price | ||||||||
Replacement females (heifer or maiden ewe) | $ per head | $956.89 | $88.11 | $88.11 | MLA Saleyard data (average 2015–2019) | |||
Breeding bull/ram | $ per head | $2500.00 | $800.00 | $800.00 | Literature | |||
Transport costs | $ per head | $60.00 | $8.10 | $8.10 | Interviews | |||
Maintenance cost | ||||||||
Annual maintenance cost – breeding | $ per head | $70.00 | $5.07 | $5.07 | Interviews | |||
Annual maintenance cost – non-breeding | $ per head | $70.00 | $5.07 | $5.07 | Interviews | |||
Sale price ($) | ||||||||
Breeding herd <2 years | $ per head | $956.89 (300 kg) | $107.96 (18 kg) | $107.96 (18 kg) | MLA Saleyard data (mean 2015–2019) | |||
Breeding herd >2 years | $ per head | $982.66 (460 kg) | $88.11 (24 kg) | $88.11 (24 kg) | MLA Saleyard data (mean 2015–2019) | |||
Non-breeding herd <2 years | $ per head | $956.89 (300 kg) | $107.96 (18 kg) | $107.96 (18 kg) | MLA Saleyard data (mean 2015–2019) | |||
Non-breeding herd >2 years | $ per head | $1148.72 (380 kg) | $88.11 (24 kg) | $88.11 (24 kg) | MLA Saleyard data (mean 2015–2019) | |||
Wool | ||||||||
Average yield per head | Gascoyne | $ per head | $3.92 | PLB return (mean 1985–2019) | ||||
Goldfields | $ per head | $5.22 | PLB return (mean 1985–2019) | |||||
Murchison – large | $ per head | $4.96 | PLB return (mean 1985–2019) | |||||
Murchison – small | $ per head | $4.96 | PLB return (mean 1985–2019) | |||||
Wool price | $10.28 | AWI average 2015–2019 | ||||||
Purchase price | ||||||||
Replacement females (heifer or maiden ewe) | $ per head | $956.89 (300 kg) | $88.11 (24 kg) | $88.11 (24 kg) | MLA Saleyard data (mean 2015–2019) | |||
Breeding bull/ram | $ per head | $2500 | $800 | $800 | Literature | |||
Transport costs | $ per head | $60 | $8.1 | $8.1 | Interviews | |||
Shearing cost | $ per head | $8.15 | Interviews | |||||
Annual maintenance cost – breeding | $ per head | $70 | $5.07 1 | $5.07 | Interviews | |||
Annual maintenance cost – non-breeding | $ per head | $70 | $5.07 1 | $5.07 | Interviews |
Weights given in parentheses are dressed weights, and it was assumed that cattle dressed at 54%, and sheep at 45%.
The branding/marking rate (α) is the percentage of females that successfully raise a calf or lamb to be branded or marked/ear tagged (assumed to be at its first muster). This considers the calving/lambing rate (i.e. proportion of exposed cows that give birth) and postnatal mortality (i.e. proportion of young that die after birth but before being marked, including owing to dingo predation). An even sex ratio of calves and lambs was assumed.
The number of non-breeding animals of Age 1 was calculated using Eqn 1, but without the addition of re-stocker females.
The number of animals in both the breeding and non-breeding herds in Age cohorts 2–AS was calculated as the number of animals in the last time period less losses owing to mortality (µs) and animals turned off in the last time period, as follows:
It was assumed that all males were castrated and therefore all breeding bulls/rams were purchased externally, and that no females were spayed. The models also assumed that all cows were culled in their 9th year, steers in their 3rd year, ewes in their 6th year, and wethers in their 3rd year. Annual mortalities without predator losses were estimated at 6% for breeding cattle, 4% for non-breeding cattle, 10% for breeding sheep, and 8% for non-breeding sheep on the basis of pastoralist interviews and Wicks et al. (2014) (Table S2). The number of breeding bulls or rams in the herd was calculated as the number in the previous year with the annual mortality rate subtracted (Table S1), and including any new animals purchased. If the number of breeding bulls or rams in the herd fell below 3% of the herd, the enterprise would then respond by purchasing breeding males (3 bulls, or 10 rams). If the total livestock on the property was less than 80% of the carrying capacity (Table S1), re-stocker heifers or ewes would be purchased. It is unlikely that all re-stocker animals would be purchased in a single year, and therefore the number of re-stocker animals purchased was calculated as 10% of the difference in livestock between the carrying capacity and the total number held. The annual maintenance costs associated with running livestock were calculated on a per head basis by using values gained from targeted interviews with pastoralists; no wages, vehicle costs or other fixed costs were included.
The effect of livestock losses to dingo predation was incorporated into the livestock model by manipulating the branding/marking rate and the mortality of adult animals (Table S2). In our model, the mortality of adult cattle was not affected by dingoes. The current (i.e. dingo-affected) branding/marking rate was estimated using livestock return data (2015–2019 PLB returns; Table 1). The expected ‘predation-free’ marking/branding rate that could be achieved following dingo removal was the average rate between 1985 and 1995 (Table 1), during which dingo predation was relatively infrequent in the Southern Rangelands. As the density of dingoes would decrease in an exponential decay following effective control and fencing, the branding/marking rate was assumed to increase following a logistic curve from the current levels.
Removal of dingoes from inside finished cell fence is expected to require a significant increase in investment in control effort (Kennedy et al. 2021; Pacioni et al. 2021b). Investment in dingo control to achieve removal after completion of the cell fence, calculated on a per enterprise basis (Table 1), included a four-fold increase in the cost of predator baiting (primarily meat and poison costs), a two-fold increase in the cost of licensed pest-management technicians (LPMTs, or ‘doggers’), and a two-fold increase in the time required by each land manager performing dingo control (predominantly deployment of baits, trapping, and fence inspection). Based on interviews with pastoralists and conversations with pest managers, we assumed that that dingo control effort returned to current ‘preventative dingo control’ levels after dingo removal was achieved. We also tested three levels of time (number of years) required to achieve that removal.
Total expenditure on construction of the cell fence (consisting of labour, materials, and vegetation clearing) was calculated on a per enterprise basis, based on information provided by each RBG. The Goldfields, Gascoyne, and small Murchison cells required construction of an entirely new fence, whereas the large Murchison cell (the largest cell-fence in the present study) required construction of only a short new fence to connect two existing predator fences, and an upgrade of existing fences (Table 1). Regular fence maintenance costs were included on a per kilometre basis (Table 1). The cost of cell-fence construction was applied in Year 0, whereas the annual maintenance cost was applied from Year 1 to Year 25.
Macropods represent a significant contribution to total grazing pressure in the Southern Rangelands. The number of macropods (sum of all red kangaroos, Osphranter rufus, western grey kangaroos, Macropus fuliginosus, and euros, Osphranter robustus) present on the property was estimated using the average density of macropods within the area between 1996 and 2019, calculated from aerial survey data (Dawson et al. 2023; Table 1). The dry-sheep equivalent (DSE; Australian standard for comparing carrying capacity, representing the daily energy requirements to maintain condition in a 50 kg wether or dry ewe) for a 50 kg macropod is estimated to be 0.7–1 DSE (Pahl 2020a). Assuming an average mass of 30 kg for red kangaroos (~60% of the three macropod species recorded inside the cell-fenced areas, Dawson et al. 2023), we assumed an average DSE of 0.6 per individual macropod in the current study (Pahl 2020a). We then included five levels of macropod population size, assuming a steady state (i.e. no increase), or four increments in macropod numbers in response to removal of dingo predation.
The number of livestock turned off in each cohort was calculated based on the average turnoff values for each livestock type on a regional basis (to reflect regional differences in livestock practices; Table S1). Turnoff values were adjusted to ensure each model converged on a stable state (e.g. to avoid some models accumulating cattle indefinitely). The proportion of livestock turned off from each cohort was held constant for the duration of the study. The number of animals turned off in each cohort was restricted to not exceed the livestock available in that cohort.
If the total grazing pressure (all livestock and macropods combined; calculated as DSE) exceeded the carrying capacity of the property by 20%, the number of animals turned off was increased by a factor of four, representing a destocking response. If the stocking rate exceeded carrying capacity by 40%, turnoff was increased by a factor of 6, and if it exceeded the carrying capacity by 60%, turnoff was increased a factor of 8.
The price per head of animals was calculated using average saleyard values for the period 2015–2019 (available from https://www.mla.com.au/prices-markets/). The transport cost to market was calculated for sheep and cattle, but assumed to be consistent between cohorts and regions. For enterprises that ran wool sheep, it was assumed that the entire flock was shorn each year prior to any turnoff, with average wool production calculated by using the average wool production per head since 1985 (Table 2). The total value of wool produced was calculated as the total wool production multiplied by the value of wool, given as the average wool value between 2015 and 2019 (available from https://www.wool.com/market-intelligence/) minus shearing cost.
The NPV of cattle and meat sheep enterprises was calculated as the number of animals turned off in each cohort , multiplied by the value per head of livestock in that cohort , less transport costs (transc), livestock maintenance costs , cost of purchasing re-stocker animals (rs), and the cost of fencing (f) and dingo control (d), with a discount rate of () of 5%, as follows (Eqn 3):
The NPV of wool sheep enterprises was similarly calculated, with the inclusion of income from wool sales, calculated at the wool price (wp) multiplied by the yield (wy), multiplied by the number of sheep shorn , less the shearing cost (shearc), as follows (Eqn 4):
Analysis
To assess the benefits of cell-fencing, a model was run for each of the possible combinations of the input variables. In total, 48 unfenced scenarios were modelled (i.e. 4 regions × 3 livestock types × 4 commodity price levels), compared with 3600 fenced scenarios (i.e. 4 regions × 3 livestock types, × 4 commodity price levels × 5 livestock weaning rates × 3 dingo removal times × 5 macropod response levels). Regional and livestock differences were assessed by comparing estimated NPV for scenarios in which commodity prices, weaning rates, removal times, and macropod density were held at projected values (i.e. the values likely to occur, indicated in Fig. 3). Variation in the outcome was assessed by averaging estimated NPV across all scenarios by region and livestock type. The relative effects of each of the key variables was determined by individual analysis, while holding all other variables at their projected values.
We compared the outcomes of different scenarios using two measures, namely (1) ‘profitability’, which indicated whether a given scenario returned a positive NPV (i.e. the income generated over 25 years greater than the cost, discounted at 7%), and (2) ‘benefit of fencing’, which was visualised using two methods. First, the difference in NPV between a pastoral enterprise with a cell-fence and the matched enterprise without fencing (unfenced base-case scenario). And second, the BCR of investment in cell-fencing. The BCR was calculated by dividing the present value of the cost of cell-fencing and dingo control, by the present value of additional revenue from livestock sales. This was undertaken by comparing a cell-fenced scenario (with the associated construction and maintenance costs, dingo control costs, purchase of re-stocker animals, additional livestock maintenance costs, and additional livestock sales), with its corresponding unfenced scenario (i.e. with the same livestock type, region, and commodity prices). A sensitivity analysis was conducted to investigate the impact of changing the discount rate, with a low rate (3%) and a high rate (7%) being tested (New South Wales Treasury 2023).
In one suite of scenarios (i.e. enterprises in the large Murchison cell with a 30% or 40% increase in macropods following fencing), there were numerous outliers for the BCA. As a result, BCR values of >8 or <−8 were excluded (n = 30).
Results
The costs of building and maintaining (over 25 years) cell-fencing varied from $76,651 to $829,111 per property across the four regions (Table 1).
(1) Profitability
NPV ranged from –$1,305,258 to $4,509,006 over 25 years, reflecting the marked variability between current and predicted cell-fenced enterprises across the semi-arid Southern Rangelands of Western Australia. Of the 48 unfenced scenarios, 33.3% returned a positive NPV (i.e. were profitable over the 25-year timescale), compared with a similar proportion (37.4%) of the 3600 cell-fenced scenarios. Profitability was greatest for cattle enterprises in the Gascoyne region (which currently supports herd sizes 3–5 times those for the other regions; Table 1) and lowest for meat sheep enterprises in the Goldfields (which currently runs the smallest of sheep flocks, as low as 5% of those in other regions; Table 1).
(2) Benefit of fencing
In total, 43.4% of cell-fenced scenarios returned a BCR greater than one, being more profitable than the relevant unfenced scenario; the cost of fence construction, maintenance, and increased dingo control often was not outweighed by the increase in livestock production. Unsurprisingly, there was a marked variation in the relative benefit of cell-fencing among enterprises with different livestock in different regions (Fig. 4a). When considering the average NPV for each regional and livestock combination, cell-fenced enterprises outperformed the unfenced scenario in meat and wool sheep enterprises for two cells (Gascoyne and large Murchison), and wool sheep in two cells (Goldfields and small Murchison) (Fig. 4a). The NPV returned by cell-fenced scenarios for cattle enterprises in all regions was lower than the relevant unfenced scenario, suggesting that the investment in cell-fencing enterprises was rarely justified by an increase in cattle production (Fig. 4a). In all other livestock type–region combinations, the increased revenue resulting from cell-fencing did not outweigh the cost of investment in fence construction, fence maintenance, and dingo removal.
The net present value (NPV) of cell-fenced and unfenced pastoral enterprises across four regions in the Southern Rangelands of Western Australia, (a) summarising all scenarios examined (mean and standard deviation values), and (b), the mean benefit–cost ratio (BCR) and standard deviation of each of the combinations. For readability, the lower confidence interval of beef enterprises (−3.71) in the large Murchison cell was not shown.
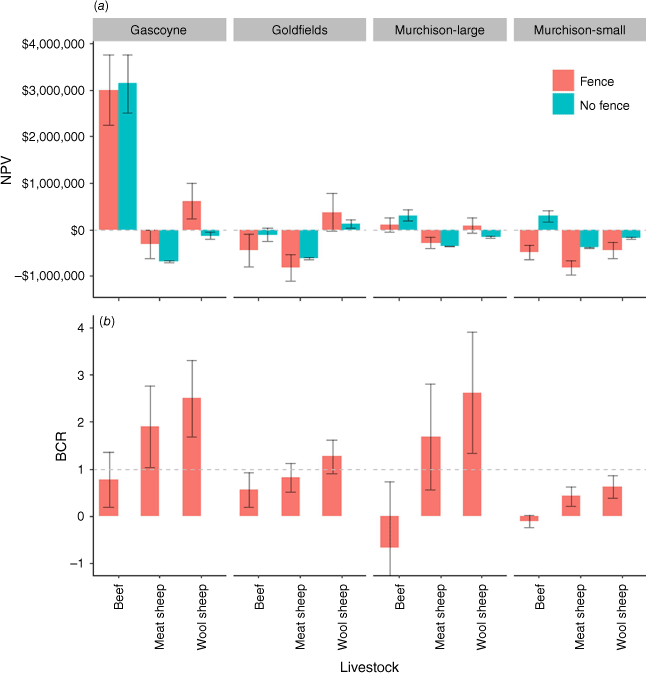
The BCR of cell-fencing was greater than one for sheep enterprises in the Gascoyne cell and all livestock in the large Murchison cell, and marginal or below one for all livestock types in the Goldfields and small Murchison cell (Fig. 4b).
Commodity prices
Changes in the prices for livestock sales, livestock purchases, and wool sales affected the return on investment for cell-fence construction, particularly for wool sheep enterprises. Broadly, shifting commodity prices influenced the profitability of each scenario, but not the overall conclusions about relative benefit of cell-fencing (Fig. 5a). Cell-fenced cattle enterprises in all four regions returned a lower NPV than did the unfenced scenario, with the BCR of cell-fencing cattle enterprises rarely greater than one. The BCR of cell-fenced meat sheep enterprises in the Gascoyne and large Murchison cells was greater than one (Fig. 5b), but was generally less than one in the Goldfields and small Murchison cell. The BCR of cell-fenced wool sheep enterprises was largely positive, except for the small Murchison cell.
The effect of commodity prices on (a) the net present value (NPV) of unfenced, and fenced scenarios, and (b) the benefit–cost ratio (BCR) of cell fencing. In this analysis, weaning rate was assumed to increase to expected ‘predation-free’ levels (assuming minimal dingo predation), removal of dingoes was achieved in the projected time, and there was no population increase by macropods. In (a), the dashed line represents NPV = $0, and in (b), it represents BCR = 1.
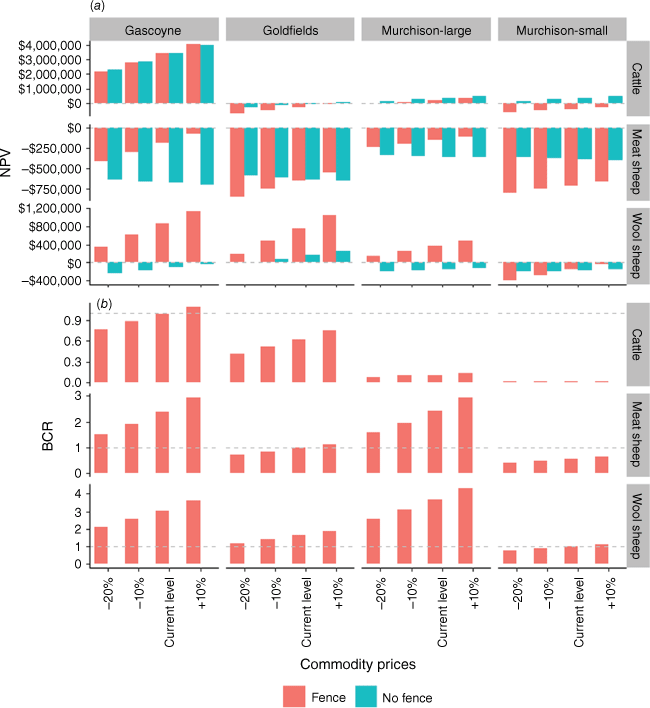
Weaning rate
On the basis of 2015–2019 PLB data, there was a two-fold difference in cattle branding rate among regions (20–41%), but all four regions had similar current weaning rates for meat sheep (20–22%) and wool sheep (12–17%) (Table 1). These values sit 20–35% below the weaning rates recorded between 1985 and 1995 (32–52%), when dingo predation was less common across the Southern Rangelands (expected ‘predation-free’ levels, Table 1). In the modelled scenarios based on these ‘ideal’ data, increased livestock weaning rate had a positive effect on the NPV of all cell-fenced enterprises, especially for wool sheep (Fig. 6a). The weaning rate, both current and expected, in the Gascoyne region is higher than in all other regions.
The effect of varying levels of response in livestock weaning rate on (a) the net present value (NPV) of cell-fenced scenarios, and (b) the benefit–cost ratio (BCR) of cell-fencing. In this analysis, commodity prices are assumed to remain at current levels, removal of dingoes was achieved in the projected time, and there was assumed to be no population increase by macropods. Values superimposed over the ‘Expected’ bar indicate the weaning rate that a cell-fenced property is expected to produce. In (a), the dashed line represents NPV = $0, and in (b), it represents BCR = 1.
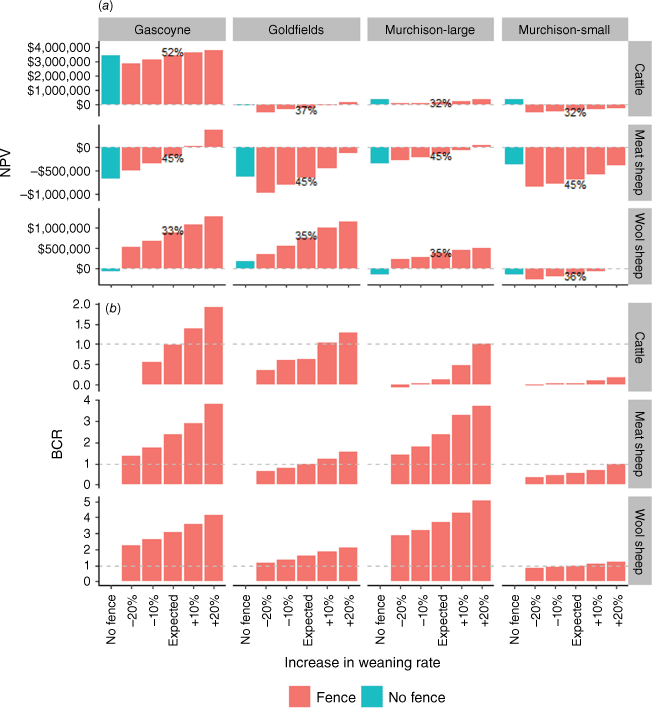
Cattle enterprises were unlikely to benefit greatly from cell-fencing unless weaning rates increased well beyond current rates, and even the expected ‘predation-free’ levels (Fig. 6b); the only cell-fenced cattle enterprises that outperformed the unfenced scenario were in the Gascoyne and Goldfields regions. In the Gascoyne region, for example, this would require that the weaning rates increased to at least 10% above expected ‘predation-free’ levels (52%), higher than the current weaning rates (41%). In the subset of results presented in Fig. 6a, meat sheep enterprises returned a negative NPV in nearly all situations. However, increasing weaning rate following cell-fencing had a positive effect on the BCR. In particular, the Goldfields and small Murchison cells were unlikely to return a BCR greater than one unless weaning rate following fence completion is very high (Fig. 6b). Cell-fenced wool sheep scenarios were more likely to return a greater NPV, and a better BCR, than were meat sheep scenarios (Fig. 6b).
Dingo control
Current data derived from RBGs and targeted interviewees indicated that managers currently faced similar costs for dingo control across the four regions ($12,830–$15,667 per property per year); however, greater investment would be required to remove dingoes within the cell-fences (Kennedy et al. 2021; Pacioni et al. 2021b). The length of time required to remove dingoes from inside cell-fenced areas would likely extend beyond the projected time required in the present modelling (we used 5 years to complete removal for the two smaller cells and 7 years to complete removal for the two larger cells), in which case the NPV of cell-fenced enterprises would be reduced (Fig. 7a). Notably, the time required for dingo removal has little impact on the return to cattle enterprises, whereas it has a relatively large effect on sheep (Fig. 7a, b). For sheep enterprises in the Gascoyne and large Murchison cells, cell-fenced enterprises outperformed the relevant unfenced scenario, and returned a BCR greater than one, even with a two-fold increase in dingo removal time (Fig. 7b).
The effect of increasing time required to remove dingoes from cell-fences on (a) the net present value (NPV) of cell-fenced scenarios, and (b) the benefit–cost ratio (BCR) of cell-fencing. In this analysis, weaning rate is assumed to increase to expected ‘predation-free’ levels, the commodity prices remain at current levels, and there is assumed to be no population increase by macropods. In (a), the dashed line represents NPV = $0, and in (b), it represents BCR = 1.
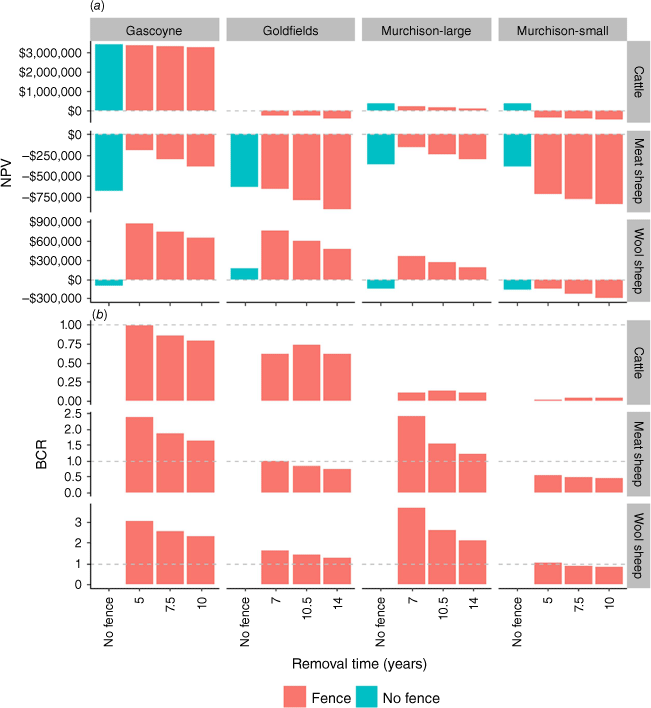
Macropod density
The macropod densities across the four regions were estimated to be between 0.55 and 2.37 kangaroos per km2, which equated to 462–1736 DSE per property. Dingo removal may lead to an increased macropod density by reducing predation, thus reducing available grazing for livestock. A projected 10–40% increase in macropod populations had particularly detrimental effects on wool sheep enterprises in both the small and large Murchison cells, which had the greatest macropod densities recorded. In all other scenarios, an increase in the density of macropods had little impact on the NPV of cell-fenced enterprises (Fig. 8a). The increasing BCR in the large Murchison cell with an increasing macropod response was due the reduction in livestock held in these scenarios, which reduced the maintenance cost of the cattle herd (Fig. 8b). In unfenced scenarios in the same region, if macropod numbers did not increase, then enterprises maintained a larger herd size, and therefore had great total livestock maintenance costs. Whereas BCR may be high, the NPV of these scenarios was low (Fig. 8a).
The effect of an increase in macropod density following dingo removal on (a) the net present value (NPV) of cell-fenced scenarios, and (b) the benefit–cost ratio (BCR) of cell-fencing. In this analysis, weaning is assumed to increase to expected ‘predation-free’ levels, the commodity prices remain at current levels, and removal of dingoes was achieved in the projected time. In (a), the dashed line represents NPV = $0, whereas in (b), it represents BCR = 1. The BCR value for cattle enterprises in the large Murchison cell with a 40% increase in macropods was excluded as an outlier.
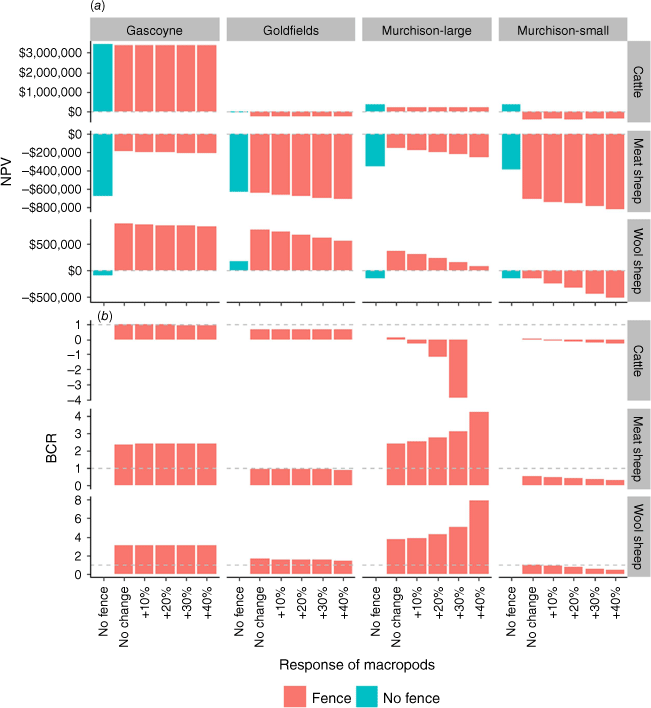
Discount rate
There was marked variation in the sensitivity of each region and livestock type to changes in the discount rate. Increasing the discount rate reduced the NPV and BCR in all instances (Fig. 9a, b). Of particular note are cattle enterprises in the Gascoyne cell, and sheep enterprises in the Goldfields and small Murchison cells, because increases in the discount rate caused the BCR of cell fencing to fall to less than one.
The effect of variation in the discount rate on the (a) the net present value (NPV) of cell-fenced scenarios, and (b) the benefit–cost ratio (BCR) of cell-fencing. In this analysis, weaning is assumed to increase to expected ‘predation-free’ levels, the commodity prices remain at current levels, removal of dingoes was achieved in the projected time, and there is assumed to be no population increase by macropods. In (a), the dashed line represents NPV = $0, and in (b), it represents BCR = 1.
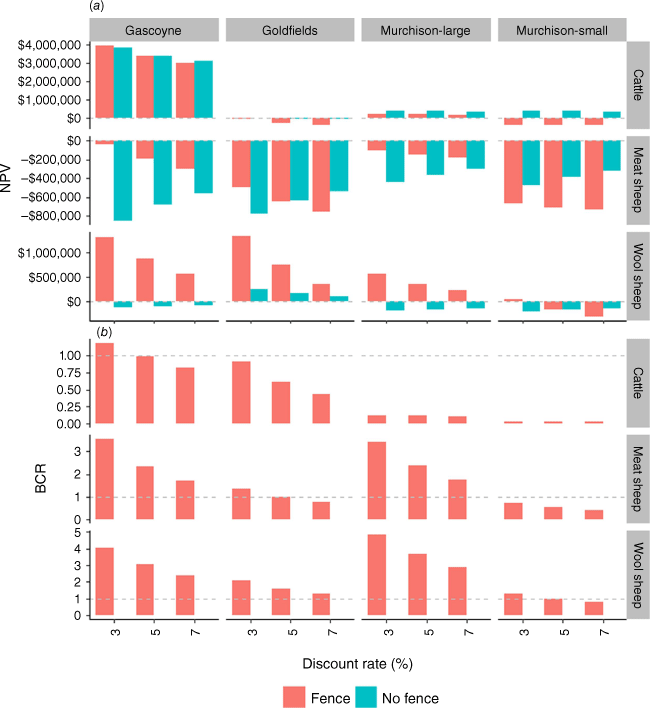
Discussion
Dingo predation coupled with declining land productivity in the Southern Rangelands of Western Australia has had a significant negative effect on pastoral enterprise profitability, particularly on those enterprises with small livestock (Foran et al. 2019). Consequently, there has been substantial investment in cell-fencing around groups of properties and more intensive dingo management. However, return on investment for a cell-fencing scenario in the Southern Rangelands is highly dependent on a range of variables and regional differences. The model-based approach used in the current study indicated that, in many cases, the cost of construction and maintenance of a cell-fence is not offset by increased productivity. Importantly, many components of the system described here interact, beyond the connections modelled in our analysis. Here, we discuss some of these interactions, keeping in mind the complexity inherent in such systems.
Region and livestock
The four cell-fenced regional scenarios examined vary markedly in size and productivity, and therefore suitability for different livestock types (indicated, in part, by the differences in average herd size between scenarios). Cattle enterprises in the Gascoyne cell were the most profitable scenario modelled, and pastoral enterprises in this region were likely to be highly profitable even without a cell-fence. This is likely to be a consequence of greater enterprise productivity within the Gascoyne region, suggesting that land productivity can substantially drive the relative benefit of cell-fencing. Whereas cell-fences are a relatively new concept in the WA Southern Rangelands, they have been employed extensively in the sheep rangelands of Queensland, Australia, for many years (Smith et al. 2020a), with positive returns on investment following dingo removal (Pahl 2022). The rangelands of northern Australia, including the cell-fenced regions of Queensland, with average stocking rates ranging from 0.25 DSE/ha in non-alluvial woodland (Kenny 2004) to 1.1 DSE/ha in Mitchell grass (Astrebla spp.) (Graham et al. 1991), are significantly more productive than are the Western Australian Southern Rangelands, where current stocking rate averages 0.06 DSE/ha (present study). Consequently, fewer livestock are protected by a fence of similar size and cost in Western Australia as in Queensland, limiting the relative increase in revenue. Therefore, generalising from the more productive rangelands of Queensland to the Southern Rangelands of Western Australia is likely to lead to unrealistic expectations.
Existing infrastructure and topography are also likely to influence profitability of cell-fencing. Both the Gascoyne and large Murchison cells in this study capitalise on existing features to reduce initial fencing costs. Our findings suggested that the relative benefit afforded by cell-fencing was highest in the Gascoyne cell, where the coastline acts as a western boundary. Similarly, the large Murchison cell uses two existing fences as western, southern and eastern borders. In other regions, the return on investment of cell-fencing exceeded the unfenced scenario only in the minority of cases.
A response to declining wool prices (Forsyth et al. 2014), in addition to high losses of livestock to dingoes (Allen and West 2013; Foran et al. 2019), had led many producers in the Southern Rangelands to move from small livestock to cattle. Consequently, many enterprises no longer have the appropriate infrastructure to run small livestock, and would require significant investment (or re-investment) in fences, yards, and shearing sheds if a change in livestock type was contemplated. The modelling approach used in the present study did not include the cost of changing to small livestock.
Small livestock are highly susceptible to dingo predation (Allen and Sparkes 2001; Fleming et al. 2001). Cattle are substantially less vulnerable, although predation can reach 32% (Allen 2014). Therefore, small livestock producers have more to gain from cell-fence construction, and cell-fenced scenarios outperformed the relevant unfenced scenario only for small livestock enterprises.
Commodity prices
Although not directly controllable by pastoralists, commodity prices have a profound impact on whether an enterprise is profitable, independent of fencing, and in turn may drive management decisions. For example, in response to declining wool prices many producers in Australia shifted from producing sheep to producing cattle (Forsyth et al. 2014). As cattle are less susceptible to dingo predation that are sheep, many land managers may have reduced active dingo control, which may have contributed to the increase in dingo density throughout pastoral areas, further reducing the viability of sheep enterprises (Forsyth et al. 2014).
The recent announcement of a planned cessation of live sheep export from Australia (DAFF 2024) is likely to reduce marketing options for sheep producers. Therefore, despite small livestock producers having the most to gain from cell-fencing, the lack of a live export market may see enterprises in the Southern Rangeland further favour cattle, which may, in turn, influence how producers prioritise dingo control.
Weaning rate
Reproductive output, quantified in the current study as weaning rate, is a primary driver of all livestock enterprise profitability (Fordyce et al. 2023). The increase in successful weaning of offspring is the primary avenue for a positive effect of dingo removal on pastoral enterprises, not to mention the animal welfare benefits of a low predation rate (Allen and Sparkes 2001; Allen and Hampton 2020). However, there is substantial uncertainty around expected weaning rate in the absence of dingo predation, as the Southern Rangelands have multiple other challenges that can reduce livestock productivity. These include large seasonal variations in forage supply and climatic (particularly precipitation) conditions (McKeon et al. 2009), year-round calving, which is out of synchrony with forage availability, increased metabolic expenditure in locomotion and thermoregulation, and extensive distances between grazing areas and available water for young stock to travel (Burns et al. 2010). Quantifying the impacts of isolated effects is problematic, and whereas increasing the weaning rate (reduced dingo predation) is the primary desired benefit of cell-fencing initiatives, the complexity of factors that influence weaning rate makes it likely that dingo removal may not necessarily trigger increased livestock reproductive output.
In many scenarios, increasing weaning rates beyond the expected weaning rates in the absence of dingo predation was required to achieve a positive return on investment in cell-fencing. In the current study, the average weaning rate between 1985 and 1995 was assumed to be representative of a ‘minimal predation environment’. The expected branding rate for cattle used in this study was 32–52%, depending on the region (Table 1), and was based on reported livestock returns. However, these values are considerably lower than the expected values (72–80%) used in previous economic analyses of cell-fencing projects in Western Australia (URS 2009; Petersen and Cooke 2016). Similarly, the expected marking rate for wool sheep used here was 32–36%, whereas the value used in previous economic analyses ranged from 54% to 78% (URS 2009; Petersen and Cooke 2016). The livestock returns used to calculate weaning rates used in the current study are self-reported, and may be subject to associated inaccuracies. To account for this uncertainty, we explored weaning rates higher than, and lower than, the ‘minimal predation environment’. If the weaning rate of livestock achieved following cell-fencing and removal of dingoes significantly exceeds the estimates used in the current analysis, the return on investment for cell-fenced projects is likely to be greater. In the current study, we have based our starting values on regionally relevant long-term datasets, and avoided speculative predictions of breeding potential. On removal of dingoes from within a completed cell-fence in the Southern Rangelands, further research quantifying the reproductive rate achieved by livestock should be completed.
Dingo control
Success of dingo control is dependent on numerous factors, including the starting density of dingoes, baiting intensity (Ballard et al. 2020), potential unavailability of baits to dingoes owing to uptake by other animals (Kreplins et al. 2018), unattractiveness of baits (Allsop et al. 2017; Kreplins et al. 2018), dingo movements in relation to control effort, seasonal availability of alternative foods, and, importantly, the size of the area over which control occurs. Further, the speed at which dingoes are removed has interactive effects with other components of the system. For example, once dingoes are removed, survival of livestock and weaning rates may increase, and annual predator control effort may reduce to ‘preventative levels’. The efficacy of dingo control, and the time required to remove them, is likely to be the most important variable determining the success of cell-fencing initiatives.
Considerable effort is currently invested in dingo control on pastoral leases throughout the Southern Rangelands, with total annual dingo control costs ranging from $12,830 to $15,667 per enterprise (this study). However, broadscale baiting strategies may not result in a measurable decline in dingo abundance sufficient to substantially decrease livestock predation (Kennedy et al. 2021).
Our modelled removal time (provisional) was based on a greatly increased control effort discussed by Pacioni et al. (2021b). Extension of the time required to remove dingoes did not completely negate the benefit of cell-fencing, but did reduce NPV. Conversely, reducing the timeframe required for dingo removal increased the NPV of cell-fenced scenarios. Recent coordinated dingo control in the small Murchison cell indicated that dingoes have been significantly reduced (Kreplins et al. 2022). Similarly, in two cell-fences in Queensland (3763 and 2265 km2), functional removal of dingoes was achieved in 3–5 years (Castle et al. 2021). However, the feasibility of dingo removal at a larger scale, and the time required to do so, is speculative (Pacioni et al. 2021b).
Macropod density
In recent years, there have been increasing calls for reducing active dingo control because of concern that this may have the perverse outcome of increasing macropod density, consequently reducing livestock productivity and increasing total grazing pressure within cell-fenced areas, potentially leading to overgrazing (e.g. Mills et al. 2020; Emmott 2021; Fisher et al. 2021; Pollock 2021). As such, there is concern that complete dingo removal from such areas could result in high macropod populations (see Prowse et al. 2015). However, uncertainty exists as to the degree to (and conditions under) which dingoes regulate macropod populations (Newsome et al. 2001a; Choquenot and Forsyth 2013).
We found little evidence that increased macropod density would negate the financial benefits of cell-fencing in Western Australia. Depending on the initial macropod density, macropod response to dingo removal had a negligible effect on the estimated NPV of cell-fencing (Table 1, Fig. 8a). The effect of increasing macropod numbers on NPV was relatively minor in the Goldfields cell, and negligible in the Gascoyne cell, and most pronounced for wool sheep enterprises, where a 40% increase in macropod density caused a significant reduction in NPV for both the small and large Murchison cells. The susceptibility of enterprises in the Murchison region to an increase in macropod density following cell-fencing is likely to be a function of high macropod density in the Murchison region, meaning that modelled populations started at a high density, coupled with the relatively small herd/flock size of enterprises in this region. The response of macropod populations to dingo removal is likely to have interactive effects with other components of our model. For example, we assumed that all landholders engage in dingo control. However, increased macropod populations may trigger some land managers to refrain from predator control (e.g. Emmott 2021) in the interest of reducing total grazing pressure, which in turn hampers the predator control efforts of neighbouring properties within the cell.
In our study, we did not model the possibility that macropod numbers would decrease following cell-fencing, as we considered it highly unlikely that pastoralists could invest enough control effort to cause such a decline. In the more productive rangelands of Queensland, where numerous cell-fences have been completed, cell-fencing combined with an increase in landholder control of macropods has resulted in a reported reduction in macropod density (Clark et al. 2018). However, we believe the Western Australian cell-fenced areas are too large, with too few land managers, for this to be likely. For example, the smallest cell in the current study encompasses four properties and covers 2188 km2, whereas the Tambo cell in Queensland (2265 km2) encompasses up to 50 properties (Castle et al. 2021). The relatively low density of macropods in the present Western Australian study compared with that in Queensland, and large average property size, mean that effective control of macropods by shooting is difficult to achieve. In this context, commercial macropod harvesters often struggle to remain financially viable.
Discount rate
The profitability of cell fenced enterprises and the benefit of cell-fencing was sensitive to changes in the discount rate. This is likely to be a reflection of the high costs incurred early in the 25-year timescale being compared against heavily discounted benefits achieved much later. In addition, many of the scenarios modelled in the current study produced marginal returns, and an increasing discount rate can easily negate the benefits of cell-fence projects. We considered all money spent on dingo control and cell-fencing at the property; however, we did not differentiate between externally funded and privately funded projects. Moreover, many of the cell-fences proposed in the Southern Rangelands are supported by government investment, generally in the form of provision of materials, reducing the significant initial construction costs. External investment in construction, reducing expenditure by individual enterprises, will therefore reduce the sensitivity of such enterprises to an increasing discount rate.
Limitations
We used a relatively simple, model-based approach to explore a range of possible outcomes of cell-fencing in the Western Australian Southern Rangelands. This approach was selected, in part, because there were no completed cell-fences in the region at the commencement of the study, limited primary data on livestock predation from the region, and limited understanding of the response of macropods to dingo control. These limitations have been addressed in a similar study by Prowse et al. (2015) using a modelling approach, combining multiple submodels based on theoretical relationships derived from well-described systems in eastern Australia. However, the modelling approach is limited in its ability to predict the outcome of the complex social, economic, biological, and environmental domains that affect rangeland pastoral enterprises.
The pastoral enterprises modelled in this approach were steady-state models, assuming no annual variation in carrying capacity (i.e. owing to rainfall or fire), macropod populations, livestock weaning rate, or dingo density. We accounted for stochastic variation by using long-term averages for all environmental variables, but cannot fully describe the good (and bad) years that will influence the year-to-year profitability of rangeland enterprises. In addition, the multitude of other variables experimentally manipulated in this study necessitated the simplification of other facets of the models to ensure accurate interpretation of trends. Even though the highly stochastic nature of the Southern Rangelands may make the use of a steady-state model unrealistic, we contend that the trends identified over time are informative, and do not believe that this approach systemically biased the reported findings in any significant way.
The current models assume that land managers will manipulate livestock numbers to avoid exceeding the current carrying capacity of the property. There are multiple reasons why this assumption is unrealistic, and in many cases, unnecessary. First, given the broad variation in the amount and timing of precipitation, adjusting stocking rates to match precipitation is largely impractical. Further, given the extensive nature of pastoral enterprises, with minimal handling of livestock and only rough estimates of macropod density, the total grazing pressure is unknown until the effects of overgrazing are observed. Second, many pastoralists interviewed in the current study believed that the current carrying capacity is unsustainably high and maintain a stocking rate below their current rating, whereas none held the view that it was too low. Third, the assumption that enterprises purchase re-stocker animals to increase the size of the herd in response to low livestock numbers may also be unsupported, because region-wide impacts such as drought and rain influence the costs of re-stockers, and many pastoral enterprises cannot afford to purchase breeders to increase livestock density (instead relying on intrinsic herd growth). However, these assumptions were required, to limit the livestock model and produce realistic outcomes.
The modelling approach used in the current study was parameterised using data from the annual PLB return. As pastoralists self-report in the annual report, it is likely that data provided have inherent inaccuracies, such as inaccurate estimate of livestock present, or even that some pastoralists deliberately under-report livestock numbers. In interviews, many pastoralists indicated their awareness of inaccurate reporting. However, given that the PLB dataset used in the current study represents 36 years of data from over 100 pastoral leases, we consider it represents the best data available and is an appropriate source of data to parameterise the models used. Indeed, given the speculative estimates of livestock reproduction used in other economic analyses of cell-fencing (see URS 2009; Petersen and Cooke 2016), the current analysis represents a more conservative estimate of livestock reproduction in the Southern Rangelands.
Finally, analyses of predation of livestock generally focus on the financial inputs and outcomes, ignoring indirect, non-economic impacts, such as livestock welfare, support for rural and remote communities, and mental health of primary producers (Wicks et al. 2014; Ecker et al. 2017). In the current study, we made no attempt to quantify these outcomes, although we expect their effects to be significant. Dingo control could also lead to an overall reduction in predation pressure applied to threatened species (Smith et al. 2020b), providing novel opportunities such as tourism, and increase community cohesiveness and involvement in ‘doing something positive’ for the environment. However, there are also potentially negative environmental effects of cell-fencing that could have wider community impact. For example, the construction of fences can create impermeable barriers that fragment populations of native species (Bradby et al. 2014; Jakes et al. 2018). Fencing can also divide properties, and cause issues among neighbours in terms of management practices.
Conclusions
In total, only 37.4% of cell-fenced scenarios returned a positive NPV, meaning that even with a cell-fence and successful dingo removal, most pastoral enterprises would be unlikely to be profitable.
However, 43.4% of cell-fenced scenarios returned a BCR greater than one, suggesting that in many cases the costs of investing in cell-fencing could provide a positive return on investment, independent of the overall enterprise profitability.
Profitability and relative benefits of cell-fence construction and subsequent dingo control efforts are largely contingent on increased weaning rates and effective and timely reduction in dingo numbers.
Estimates of reproduction rates were sourced from self-reported ‘livestock returns’, resulting in relatively conservative estimates of livestock reproduction without dingo predation; however, the validity of these data is discussed.
In general, unfenced cattle enterprises had the greatest profitability (highest NPV over the 25-year time frame), and there was little benefit to investment in cell-fencing.
The return on investment for fencing was greatest for small livestock enterprises, particularly wool sheep enterprises. Future changes, such as the phase-out of live sheep export announced in May 2024, are likely to have a significant effect on the willingness of pastoralists to run small stock.
Bioeconomic modelling can show scenarios where combinations of quantifiable variables are considered, but social and environmental impacts of cell-fencing are not easily taken into account in such models.
Conflicts of interest
Three of the authors (S. D., T. K., M. K.) are currently employed by, or have previously been employed by, the WA Department of Primary Industries and Regional Development, which supports the construction of cell-fences in WA by various mechanisms.
Declaration of funding
This research was supported by the Centre for Invasive Species Solutions (P01-L-006).
Acknowledgements
The authors thank Wayne Fletcher, Jim Miller, Greg Brennan and Liz Peterson for their input and advice throughout the design and conduct of the research. We acknowledge the contributions of the Carnarvon Rangelands Biosecurity Association, Meekatharra Rangelands Biosecurity Association, Goldfields–Nullarbor Rangelands Biosecurity Association, and the Central Wheatbelt Biosecurity Association, and appreciate the advice and support of the respective Executive Officers of these organisations. We sincerely appreciate the involvement of the pastoralists who were interviewed as part of this research, who welcomed us into their homes, often providing accommodation and food.
References
Allen LR (2014) Wild dog impacts on calf wastage in extensive beef cattle enterprises. Animal Production Science 54, 214-220.
| Crossref | Google Scholar |
Allen BL (2015) More buck for less bang: reconciling competing wildlife management interests in agricultural food webs. Food Webs 2, 1-9.
| Crossref | Google Scholar |
Allen BL, Fleming P (2004) Review of canid management in Australia for the protection of livestock and wildlife—potential application to coyote management. Sheep & Goat Research Journal 19, 97-104 Available at https://digitalcommons.unl.edu/icwdmsheepgoat/2.
| Google Scholar |
Allen BL, Hampton JO (2020) Minimizing animal welfare harms associated with predation management in agro‐ecosystems. Biological Reviews 95, 1097-1108.
| Crossref | Google Scholar | PubMed |
Allen L, Sparkes E (2001) The effect of dingo control on sheep and beef cattle in Queensland. Journal of Applied Ecology 38, 76-87.
| Crossref | Google Scholar |
Allen B, West P (2013) Influence of dingoes on sheep distribution in Australia. Australian Veterinary Journal 91, 261-267.
| Crossref | Google Scholar | PubMed |
Allsop SE, Dundas SJ, Adams PJ, Kreplins TL, Bateman PW, Fleming PA (2017) Reduced efficacy of baiting programs for invasive species: some mechanisms and management implications. Pacific Conservation Biology 23, 240-257.
| Crossref | Google Scholar |
Ballard G, Fleming PJS, Meek PD, Doak S (2020) Aerial baiting and wild dog mortality in south-eastern Australia. Wildlife Research 47, 99-105.
| Crossref | Google Scholar |
Bauer H, de Iongh H, Sogbohossou E (2010) Assessment and mitigation of human–lion conflict in West and Central Africa. Mammalia 74, 363-367.
| Crossref | Google Scholar |
Bradby K, Fitzsimons JA, Del Marco A, Driscoll DA, Ritchie EG, Lau J, Bradshaw CJA, Hobbs RJ (2014) Ecological connectivity or Barrier Fence? Critical choices on the agricultural margins of Western Australia. Ecological Management & Restoration 15, 180-190.
| Crossref | Google Scholar |
Burns B, Fordyce G, Holroyd R (2010) A review of factors that impact on the capacity of beef cattle females to conceive, maintain a pregnancy and wean a calf—Implications for reproductive efficiency in northern Australia. Animal Reproduction Science 122, 1-22.
| Crossref | Google Scholar | PubMed |
Campbell G, Coffey A, Miller H, Read JL, Brook A, Fleming PJ, Bird P, Eldridge S, Allen BL (2019) Dingo baiting did not reduce fetal/calf loss in beef cattle in northern South Australia. Animal Production Science 59, 319-330.
| Crossref | Google Scholar |
Campbell G, Emmott A, Pollock D, Traill BJ (2022) Can dingoes increase graziers’ profits and help maintain Australia’s rangelands? The Rangeland Journal 44, 129-135.
| Crossref | Google Scholar |
Castle G, Smith D, Allen LR, Allen BL (2021) Terrestrial mesopredators did not increase after top-predator removal in a large-scale experimental test of mesopredator release theory. Scientific Reports 11, 18205.
| Crossref | Google Scholar | PubMed |
Caughley G, Grigg G, Caughley J, Hill G (1980) Does dingo predation control the densities of kangaroos and emus? Wildlife Research 7, 1-12.
| Crossref | Google Scholar |
Choquenot D, Forsyth DM (2013) Exploitation ecosystems and trophic cascades in non‐equilibrium systems: pasture–red kangaroo–dingo interactions in arid Australia. Oikos 122, 1292-1306.
| Crossref | Google Scholar |
Choquenot D, Hone J (2000) Using bioeconomic models to maximize benefits from vertebrate pest control: lamb predation by feral pigs. In 'Human conflicts with wildlife: economic considerations. Proceedings of the Third NWRC Special Symposium'. (Eds L Clark, J Hone, JA Shivik, RA Watkins, KC VerCauteren, JK Yoder). Available at https://digitalcommons.unl.edu/nwrchumanconflicts/8
Dawson SJ, Kreplins TL, Kennedy MS, Renwick J, Cowan MA, Fleming PA (2023) Land use and dingo baiting are correlated with the density of kangaroos in rangeland systems. Integrative Zoology 18, 299-315.
| Crossref | Google Scholar | PubMed |
Ecker S, Please PM, Maybery DJ (2017) Constantly chasing dogs: assessing landholder stress from wild dog attacks on livestock using quantitative and qualitative methods. Australasian Journal of Environmental Management 24, 16-30.
| Crossref | Google Scholar |
Eklund A, López-Bao JV, Tourani M, Chapron G, Frank J (2017) Limited evidence on the effectiveness of interventions to reduce livestock predation by large carnivores. Scientific Reports 7, 2097.
| Crossref | Google Scholar | PubMed |
Emmott A (2021) The Dingo as a management tool on a beef cattle enterprise in western Queensland. Australian Zoologist 41, 459-466.
| Crossref | Google Scholar |
Fisher AG, Mills CH, Lyons M, Cornwell WK, Letnic M (2021) Remote sensing of trophic cascades: multi‐temporal landsat imagery reveals vegetation change driven by the removal of an apex predator. Landscape Ecology 36, 1341-1358.
| Crossref | Google Scholar |
Fleming PA, Stobo-Wilson AM, Crawford HM, Dawson SJ, Dickman CR, Doherty TS, Fleming P, Newsome TM, Palmer R, Thompson JA, Woinarski J (2022) Distinctive diets of eutherian predators in Australia. Royal Society Open Science 9, 220792.
| Crossref | Google Scholar | PubMed |
Foran B, Smith MS, Burnside D, Andrew M, Blesing D, Forrest K, Taylor J (2019) Australian rangeland futures: time now for systemic responses to interconnected challenges. The Rangeland Journal 41, 271-292.
| Crossref | Google Scholar |
Fordyce G, Shephard R, Moravek T, McGowan MR (2023) Australian cattle herd: a new perspective on structure, performance and production. Animal Production Science 63, 410-421.
| Crossref | Google Scholar |
Forsyth DM, Woolnough AP, Nimmo DG, Ritchie EG, Kennedy M, Pople A, Watson I (2014) A comment on the influence of dingoes on the Australian sheep flock. Australian Veterinary Journal 92, 461-462.
| Crossref | Google Scholar | PubMed |
Graham T, Clark R, Knights P, Lawrence D, Murphy R, Slater B, Warrian C (1991) Pasture management: current and recommended stocking rates in the Maranoa region, Queensland. Tropical Grasslands 25, 227-228.
| Google Scholar |
Hayward MW, Kerley GIH (2009) Fencing for conservation: restriction of evolutionary potential or a riposte to threatening processes? Biological Conservation 142, 1-13.
| Crossref | Google Scholar |
Jakes AF, Jones PF, Paige LC, Seidler RG, Huijser MP (2018) A fence runs through it: a call for greater attention to the influence of fences on wildlife and ecosystems. Biological Conservation 227, 310-318.
| Crossref | Google Scholar |
Kennedy MS, Kreplins TL, O’Leary RA, Fleming PA (2021) Responses of dingo (Canis familiaris) populations to landscape-scale baiting. Food Webs 27, e00195.
| Crossref | Google Scholar |
Kenny G (2004). Report to the productivity commission. Cost and stocking rate data for the Murweh Shire. Available at https://www.pc.gov.au/inquiries/completed/native-vegetation/murweh1/murweh1.pdf
Khorozyan I, Waltert M (2019) How long do anti-predator interventions remain effective? Patterns, thresholds and uncertainty. Royal Society Open Science 6, 190826.
| Crossref | Google Scholar | PubMed |
Kolowski JM, Holekamp KE (2006) Spatial, temporal, and physical characteristics of livestock depredations by large carnivores along a Kenyan reserve border. Biological Conservation 128, 529-541.
| Crossref | Google Scholar |
Kreplins T, Kennedy M, Adams P, Bateman P, Dundas S, Fleming P (2018) Fate of dried meat baits aimed at wild dog (Canis familiaris) control. Wildlife Research 45, 528-538.
| Crossref | Google Scholar |
Kreplins T, Kennedy M, Fleming T, Dawson S, Miller J, Barwick J, Macleay C, Omogbene M, O’Leary R, Renwick J (2022) ‘Assessment of the biodiversity, economic and productivity gains from exclusion fencing, Western Australia.’ Available at https://invasives.com.au/wp-content/uploads/2023/06/L006-Final-release.pdf
McKeon GM, Stone G, Syktus J, Carter J, Flood N, Ahrens D, Bruget D, Chilcott C, Cobon D, Cowley R (2009) Climate change impacts on northern Australian rangeland livestock carrying capacity: a review of issues. The Rangeland Journal 31, 1-29.
| Crossref | Google Scholar |
McKnight TL (1969) Barrier fencing for vermin control in Australia. Geographical Review 59, 330-347.
| Crossref | Google Scholar |
Miller JR, Stoner KJ, Cejtin MR, Meyer TK, Middleton AD, Schmitz OJ (2016) Effectiveness of contemporary techniques for reducing livestock depredations by large carnivores. Wildlife Society Bulletin 40, 806-815.
| Crossref | Google Scholar |
Mills CH, Waudby H, Finlayson G, Parker D, Cameron M, Letnic M (2020) Grazing by over-abundant native herbivores jeopardizes conservation goals in semi-arid reserves. Global Ecology and Conservation 24, e01384.
| Crossref | Google Scholar |
Moreira-Arce D, Ugarte CS, Zorondo-Rodríguez F, Simonetti JA (2018) Management tools to reduce carnivore–livestock conflicts: current gap and future challenges. Rangeland Ecology & Management 71, 389-394.
| Crossref | Google Scholar |
Newsome A, Catling P, Cooke BD, Smyth R (2001a) Two ecological universes separated by the dingo barrier fence in semi-arid Australia: interactions between landscapes, herbivory and carnivory, with and without dingoes. The Rangeland Journal 23, 71-98.
| Crossref | Google Scholar |
Pacioni C, Kennedy MS, Berry O, Stephens D, Schumaker NH (2018) Spatially-explicit model for assessing wild dog control strategies in Western Australia. Ecological Modelling 368, 246-256.
| Crossref | Google Scholar | PubMed |
Pacioni C, Kennedy MS, Ramsey DSL (2021a) When do predator exclusion fences work best? A spatially explicit modelling approach. Wildlife Research 48, 209-217.
| Crossref | Google Scholar |
Pacioni C, Ramsey DSL, Schumaker NH, Kreplins T, Kennedy MS (2021b) A novel modelling framework to explicitly simulate predator interaction with poison baits. Wildlife Research 48, 64-75.
| Crossref | Google Scholar |
Pahl L (2020a) Macropods, feral goats, sheep and cattle. 1. Equivalency in how much they eat. The Rangeland Journal 41, 497-518.
| Crossref | Google Scholar |
Pahl L (2020b) Macropods, feral goats, sheep and cattle. 2. Equivalency in what and where they eat. The Rangeland Journal 41, 519-533.
| Crossref | Google Scholar |
Petersen E, Cooke P (2016) Benefit Cost Analysis of Wild Dog Management Options in Regional Western Australia. (Report and model prepared by Agknowledge and Advanced Choice Economics Pty Ltd for the Department of Agriculture and Food, Western Australia to inform the 2016 Wild Dog Action Plan for Western Australia)
Pollock D (2021) Rediscovering a role for dingoes on Wooleen Station. Australian Zoologist 41, 480-486.
| Crossref | Google Scholar |
Pople A, Grigg G, Cairns S, Beard L, Alexander P (2000) Trends in the numbers of red kangaroos and emus on either side of the South Australian dingo fence: evidence for predator regulation? Wildlife Research 27, 269-276.
| Crossref | Google Scholar |
Prowse TA, Johnson CN, Cassey P, Bradshaw CJ, Brook BW (2015) Ecological and economic benefits to cattle rangelands of restoring an apex predator. Journal of Applied Ecology 52, 455-466.
| Crossref | Google Scholar |
Smith D, King R, Allen BL (2020a) Impacts of exclusion fencing on target and non‐target fauna: a global review. Biological Reviews 95, 1590-1606.
| Crossref | Google Scholar |
Smith D, Waddell K, Allen BL (2020b) Expansion of vertebrate pest exclusion fencing and its potential benefits for threatened fauna recovery in Australia. Animals 10, 1550.
| Crossref | Google Scholar |
Stephens D, Kennedy MS, Kreplins TL (2023) Stable dingo population structure and purity over 11 years of lethal management. Wildlife Research 50(12), 980-992.
| Crossref | Google Scholar |
Thomson P (1984) Dingoes and sheep in pastoral areas. Journal of the Department of Agriculture, Western Australia, Series 4 25, 27-31.
| Google Scholar |
Thomson P (1986) The effectiveness of aerial baiting for the control of dingoes in north-western Australia. Wildlife Research 13, 165-176.
| Crossref | Google Scholar |
van Eeden LM, Crowther MS, Dickman CR, Macdonald DW, Ripple WJ, Ritchie EG, Newsome TM (2018a) Managing conflict between large carnivores and livestock. Conservation Biology 32, 26-34.
| Crossref | Google Scholar |
Van Eeden LM, Eklund A, Miller JRB, López-Bao JV, Chapron G, Cejtin MR, Crowther MS, Dickman CR, Frank J, Krofel M (2018b) Carnivore conservation needs evidence-based livestock protection. PLoS Biology 16, e2005577.
| Crossref | Google Scholar |