Processes underpinning natural capital account compilation highlight the potential for low-input grazing to mitigate farm carbon emissions while also improving biodiversity outcomes
Rachel Lawrence


A Bush Heritage Australia, Melbourne, Victoria 3000, Australia.
B Fenner School of Environment and Society, Australian National University, Canberra, ACT 2600, Australia.
C Integrated Futures, Gundaroo, NSW 2620, Australia.
D Vanguard Business Services, Dubbo, NSW 2875, Australia.
The Rangeland Journal 45(1) 27-35 https://doi.org/10.1071/RJ22053
Submitted: 31 August 2022 Accepted: 28 April 2023 Published: 6 June 2023
© 2023 The Author(s) (or their employer(s)). Published by CSIRO Publishing on behalf of the Australian Rangeland Society. This is an open access article distributed under the Creative Commons Attribution-NonCommercial-NoDerivatives 4.0 International License (CC BY-NC-ND)
Abstract
Remnant woodland areas are commonly part of livestock grazing operations in temperate Australia. These remnant areas can store substantial amounts of carbon and have important biodiversity value, but are at risk of ongoing decline due to nutrient enrichment, overgrazing, clearing of woody debris and lack of tree recruitment. The process of compiling experimental farm-scale natural capital accounts (NCA) for 11 wool-growing businesses in temperate Australia demonstrated that some wool growers may be managing these areas in ways that sustained, and at times regenerated, carbon and other natural values. When managed sensitively, these largely native areas provide forage and shelter for livestock production while carbon and associated biodiversity is also protected, and in some cases regenerated. The farm-scale NCA process highlighted that for some farm businesses net sequestration of carbon can occur at a farm-scale, substantially owing to the management approach applied to these remnant areas. The process highlighted the potential for livestock grazing approaches underpinned by the ecosystem services provided by native grasses, forbs and woodlands (i.e. low-input rather than nutrient enrichment and pasture modification) to contribute to balancing carbon emissions from other areas of more intensive management within a farm business. With the current momentum worldwide aimed at achieving net zero emissions, there is an opportunity that has not existed previously to conserve, and sometimes regenerate, these remnant woodland areas on farmland. This could contribute to reversing a crisis of biodiversity loss in this threatened ecosystem while also helping farm businesses to reduce overall carbon emissions.
Keywords: biodiversity conservation, climate change adaptation, greenhouse gas fluxes, landscape ecology, nature-based solutions, sustainable livelihoods, system of environmental economic accounting, woodland ecology.
Introduction
Temperate grassy woodlands provide the production base and a range of provisioning and non-provisioning ecosystem services to farm businesses, as well as broader society in south-eastern Australia. Livestock grazing of these woodlands can be compatible with the retention of highly biodiverse landscapes (Barrett et al. 1994; Tremont 1994; McIntyre 2005; Dorrough et al. 2006; McIntyre et al. 2022), with eucalypts and associated native understorey also storing substantial carbon (Eldridge and Wilson 2002). Due to the suitability of these areas for agriculture, maximising agricultural production has typically been prioritised over the preservation of the natural ecosystem (Smith et al. 2013; Unc et al. 2021). Consequently, temperate woodland ecosystems are critically endangered in Australia (Australian Government, Department of Agriculture, Water and Environment 2022b) with ongoing clearing and intensification of these woodlands continuing unabated (EPA 2021).
Along with preserving remaining biodiversity values in temperate woodland areas, protecting carbon that is currently stored in forest and woodlands is critical for climate change mitigation (Keith et al. 2021). Although the conservation of relatively fragmented and lower conservation value woodland areas is usually not prioritised, carbon and biodiversity values within them, along with a range of both provisioning (i.e. forage and fodder production and stability, surface water quantity, apiary resources, timber production) and non-provisioning (such as genetic resources, green house gas (GHG) regulation, water quality regulation, resistance to weed invasion, resources and habitat for wildlife, salinity control) can be retained, and potentially regenerated, when grazing is managed in ways that protect these values (Lavorel et al. 2015).
A range of private land conservation schemes exist to compensate farmers, usually financially, to protect areas of higher value native biodiversity (Australian Government, Department of Agriculture, Water and Environment 2022a). Such schemes less commonly focus on woodlands of relatively lower biodiversity value even though these areas, including where only scattered trees remain, can still be important for biodiversity, as well as habitat connectivity in the landscape mosaic (Reid and Landsberg 2000; Gibbons and Boak 2002; Gibbons et al. 2008). Sadly, the intrinsic natural values of these lower conservation value areas, as well the ecosystem services they provide, generally go unrecognised in the context of agricultural production goals. This is particularly true in higher productivity temperate areas where lower-input approaches that integrate ecological and agricultural outcomes simultaneously are usually seen as a cost to livestock productivity and business bottom-lines (Waters et al. 2020).
Individual farms are unique and have been shaped to varying degrees by prior environmental, socio-political and economic influences. Farms are usually mosaic landscapes (Kotliar and Wiens 1990; McIntyre and Barrett 1992) and comprise highly modified (e.g. intensive sown pastures and crops), partially modified (e.g. remnant areas in varying states of transition from pre-European reference condition) and largely unmodified wild areas such as high conservation-value woodlands and forests that are usually excluded from production (McIntyre et al. 2002). It is typically the partially modified areas that remain vulnerable to agricultural intensification (such as nutrient enrichment, sowing of introduced pasture species and clearing of woody debris) and corresponding loss of carbon and biodiversity (Dorrough et al. 2007). It is in these areas that opportunities exist to protect, and in some cases increase, above- and below ground carbon stocks and biodiversity simultaneously, while also allowing for continuing low-input livestock production. If mechanisms exist that can enable recognition of both ecological and production values from different areas of the farm mosaic, there is potential for greater preservation of both carbon and biodiversity in these areas and therefore in the broader (threatened) bioregion.
This viewpoint paper presents insights gained from the compilation of experimental farm-scale natural capital accounts (hereafter NCA) as a framework for better understanding the differing values of areas of a farm with differing ecological characteristics and how these different areas could be managed to achieve a range of business goals, in addition to agricultural productivity.
The process
Experimental farm-scale NCAs were undertaken for 11 wool growing businesses in the temperate woodland regions of eastern Australia (Australian Wool Innovation 2021). Farms were all grazing enterprises, with fine wool production a significant part of their business. A focus of the NCA approach was the identification of different parts of the farm mosaic to account for the range of values (and ecosystem services) likely to be associated with areas with varying ecological characteristics. In addition to agricultural production, these different areas of a farm generate other benefits including, but not limited to, protection of water resources, stable carbon storage, carbon sequestration and biodiversity conservation. The NCA process followed the United Nations-endorsed System of Environmental Economic Accounting Experimental Ecosystem Accounts (United Nations et al. 2014). Remote imagery (Google Earth) was used to divide farms into multiple ‘ecosystem assets’ (contiguous areas with similar ecological characteristics and in a similar condition; hereafter EAs) to enable the creation of an Ecological Asset Register (EAR). The ecosystem condition of different EAs could then be recognised for their capacity to sequester and store carbon and conserve biodiversity alongside their use for agricultural production.
A simplified box gum grassy woodland State and Transition model (STM) from Whitten et al. (2010), developed from the grassland model of McIntyre and Lavorel (2007) to incorporate trees, was used to compile the EARs. The range of states in the model were relevant to EAs on the farm, with states differing according to their production and environmental values. Although vegetation changes are continuous, a range of states are identified in the model that are relevant to the EAs being recognised for their production and/or environmental values. The states ranged from reference (similar to pre-1750 wooded areas) to a highly modified condition with an exotic dominated groundlayer (Fig. 1, Supplementary material Table S1). Condition states of different areas of the farm were determined by rapid on-ground ecological surveys of representative areas. Because of the experimental nature of the accounts, resources for these surveys were limited and therefore qualitative but based on well recognised attributes of a healthy woodland ecosystem (McIntyre et al. 2002). Assessment of condition state considered current management and land-use history, tree canopy cover and condition, perennial groundcover, the proportion and diversity of native plants in the ground-layer vegetation, and retention (or not) of coarse woody debris. A judgement was also made by ecologists as to whether areas were likely to be increasing in carbon and biodiversity value (mature trees retained, dead trees left as either standing or fallen timber and regenerating trees) or losing carbon through tree death, removal of standing and fallen dead timber and a lack of tree regeneration. For areas of the farm not visited, condition states were ‘imputed’ through interpretation of aerial/satellite imagery (both Google Earth and Farm Map4D). Confidence in the assignation was recorded as either ‘visited’ or ‘imputed’ in the EAR. The compiled EARs summarised how much of each farm was in each STM state and helped understand what might be contributing to the different emission balances for the farms.
Illustration of the condition categories (states) found in box-gum grassy woodlands in the study. States range from high tree cover and high native diversity to reduced or no tree cover and low plant diversity with increasing nutrient enrichment and grazing pressure. This state and transition model is based on the grassland model of McIntyre and Lavorel (2007) and adapted to include trees by Whitten et al. (2010).
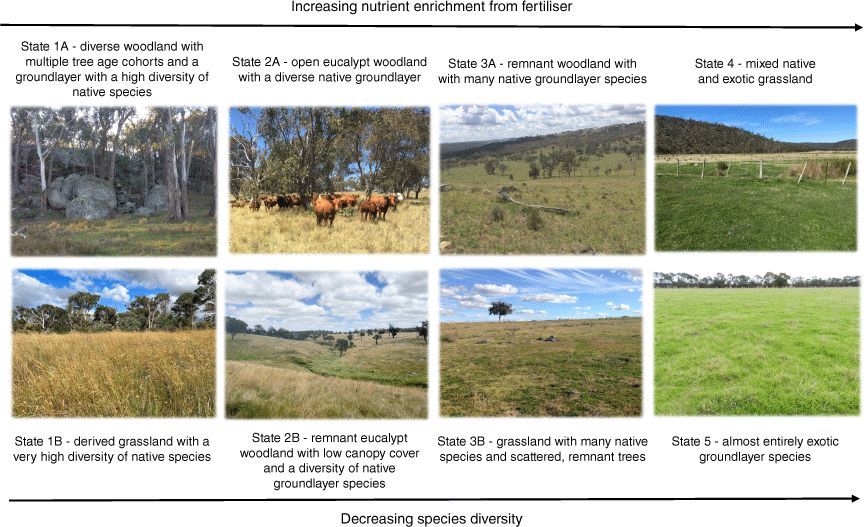
Carbon sequestration models were generated for each farm using FullCAM (Australian Government, Department of Industry, Science, Energy and Resources 2022). This provided an estimate of carbon stocks in the woody vegetation (above- and below-ground biomass) for each STM category, along with a realistic rate of change between states. The modelled sequestration rates were used in combination with the ecologist’s assessment of whether an EA was regenerating, stable or degrading, to estimate carbon gain (or loss) in each EA. Modelled sequestration rates for a given STM category varied from farm to farm because the FullCAM modelling takes into account localised factors such as rainfall, elevation and the productive potential for tree growth in that locale (details of the method including FullCAM parameters are described in Ogilvy et al. (2022).
The outcomes
The 11 farms in the study varied substantially in the degree of modification from the original woodland ecosystem. At one end of the spectrum were farms with semi-intact grassy woodlands, some areas of variable tree density according to landscape position and limited areas that were heavily modified. At the other end of the spectrum were farms that had historically been highly modified from the original ecosystem with livestock production predominantly on introduced pastures, and some areas (limited) with non-forage crops and irrigation. In between these two extremes were farms that (a) had historically been extensively modified in the areas used for production but were being managed in ways that restored tree cover through planting to mimic a woodland ecosystem and (b) still had good amounts of tree cover in production areas but were not being managed in ways that would conserve (or regenerate) overall remnant tree cover in the future.
The overall NCA process, which included consideration of energy inputs and GHG outputs (both fossil fuel-derived and enteric livestock emissions) applied to the 11 farms, made it apparent that for five of the farms, substantial (>0.1 t CO2e ha−1 year−1) net carbon sequestration was occurring and exceeded emissions from farm operations (such as machinery, transport, agrochemicals and general fossil fuel-derived energy consumption), livestock and from the loss of biogenic carbon in declining woodlands (Table 1). Where carbon sequestration was considered only in areas used for agricultural production (i.e. excluding areas not grazed or cropped and areas used for forestry operations) net emissions were still negative for these five farms, meaning that the net emissions balance for these five farms was not a result of sequestration from forested areas outside the agricultural production area. Net carbon sequestration was particularly high on two of the farms (Farms #1 and #3) where substantial woodland regeneration was considered by ecologists to have been occurring as a result of low-input grazing approaches. Notably, these two farms were in a condition that approximated an intact or semi-intact grassy box woodland, with farm production generally derived from the native woodland environment that was managed in ways that supported the protection of natural assets on the farm (McIntyre et al. 2002). Farm #4 is considered as an outlier as the positive emission balance is likely to be due to a shorter timeframe for emissions (2 years compared to 5 years) and recent substantial acquisition of land and corresponding purchase of livestock meaning that this snapshot of time is not representative of what is likely to be occurring over timeframes of 5 years.
Property | Mean annual rainfall (1985–2015; mm) | Mean altitude (m) | Number of years data used for emission averaging | Gross C sequestration (t CO2e ha−1 year−1 for the whole property) | Area of property used for agricultural productionA (%) | Area of property used for forestry productionB (%) | Area of property excluded from forestry and agricultural productionC (%) | Gross sequestration (t CO2e ha−1 year−1 of production area) | Farm emissions (t CO2e (incl. pre-farm) ha−1 year−1) | Net sequestration (t CO2e ha−1 year−1 of production area) |
---|---|---|---|---|---|---|---|---|---|---|
1 | 773 | 865 | 10 | −3.44 | 97.9 | 0 | 1.9 | −3.43 | 0.73 | −2.70 |
2 | 772 | 1052 | 6 | −1.79 | 94.4 | 0 | 4.6 | −1.85 | 1.55 | −0.30 |
3 | 711 | 538 | 10 | −1.91 | 99.5 | 0 | 0.5 | −1.89 | 0.75 | −1.14 |
4D | 631 | 293 | 2 | −1.87 | 94.7 | 0 | 4.5 | −1.88 | 5.86 | 3.99 |
5 | 677 | 343 | 5 | −1.66 | 79.9 | 0 | 20.1 | −1.81 | 0.37 | −1.45 |
6 | 690 | 476 | 10 | −1.56 | 82.9 | 0 | 15.8 | −1.53 | 1.16 | −0.37 |
7 | 532 | 295 | 5 | −0.69 | 41.8 | 0 | 58.0 | −0.10 | 1.65 | 1.55 |
8 | 642 | 116 | 5 | −0.21 | 90.5 | 0 | 9.2 | 0.02 | 1.68 | 1.70 |
9 | 714 | 62 | 5 | −1.17 | 88.2 | 6.8 | 3.5 | 0.26 | 1.42 | 1.68 |
10 | 735 | 72 | 5 | −1.72 | 21.1 | 4.5 | 73.8 | 0.36 | 0.99 | 1.34 |
11 | 868 | 580 | 5 | 1.01 | 88.1 | 0 | 10.7 | 1.12 | 2.63 | 3.75 |
Farms 1, 3 and 5, in particular, use a low-input approach to managing remnant native areas that constitute 98%, 99% and 80% respectively, of the overall farm area. Minor areas of the farm used predominantly for infrastructure, such as domestic areas, are excluded from calculations.
AAll areas other than those excluded from grazing or cropping (including watercourses if removed from regular grazing rotation), areas used for forestry production and domestic/infrastructure areas.
BAreas used for forestry that is not integrated with agricultural production.
CAreas where conservation (including watercourse protection) is the primary purpose and that are usually excluded from grazing.
DProperty 4 is italicised because it is an outlier because of emissions data being available only for 2 years, which included the substantial purchase of livestock during that time.
Making carbon loss/gain over time transparent between different areas
The case studies referred to here are based on a single (i.e. baseline survey) natural capital assessment for each farm, with these baseline accounts being supported by FullCam modelling to determine carbon storage and flows across time and in hindsight. Were the assessments of natural capital performed at two separate time intervals (i.e. Time 0 and Time 1 several years later), the compilation of the EAR would enable a detailed record and understanding of carbon gain or loss across different components of the farm landscape over time. This could, in turn, inform management decisions based on the conservation of carbon (and biodiversity) in addition to agricultural production. Table 2 shows two examples of carbon stocks and flows of a hypothetical farm under different management scenarios and a way in which this might be recorded in the natural capital accounts by using the STM condition categories. Scenario A aims to maximise productivity through nutrient enrichment and pasture intensification, including in remnant areas. This is typical regional agronomic management for the regions considered (Scott et al. 2013; Lawrence et al. 2019). Scenario B shows what could feasibly happen with an approach that applies low-input grazing management to remnant woodland areas. Such an approach is likely to include minimal use of fertiliser, planned grazing to ensure appropriate recovery of desired species and retention of coarse woody debris that provides habitat for wildlife and maximally retains resources such as plant debris and water in the landscape. The approach of Scenario B limits pasture intensification to areas that have already lost canopy trees and are predominantly introduced species in the ground layer. In Scenario A, the farm loses carbon (and corresponding biodiversity) because of woodland decline over time, whereas in Scenario B, the amount of carbon in the vegetation increases. Biodiversity values, due to an increase in native plant abundance, diversity and habitat, also increase in Scenario B. Both scenarios protect their high conservation value areas (State 1A) to maintain carbon and biodiversity values in these areas, with the difference being in the approach to management of partially modified woodland areas.
Item | STM category | |||||||
---|---|---|---|---|---|---|---|---|
1A | 2A | 3A | 2B | 3B | 4 | 5 | ||
Carbon (Mg C ha–1) stored in non-herbaceous vegetation, namely, living and dead, standing and fallen timber | 58 | 42 | 19 | 9 | 9 | 2 | 0 | |
Scenario A | ||||||||
Area (ha) at T0 | 120 | 270 | 480 | 360 | 70 | 110 | 30 | Total area = 1440 ha |
Carbon storage in woody vegetation for this ecosystem State at T0 | 6960 | 11 340 | 9120 | 3240 | 630 | 220 | 0 | 31 510 t C@T0 |
Area (ha) at T1 | 120 | 250 | 500 | 320 | 40 | 130 | 80 | Total area = 1440 ha |
Carbon storage in woody vegetation for this ecosystem State at T1 | 6960 | 10 500 | 9500 | 2880 | 360 | 260 | 0 | 30 460 t C@T1 |
= 1050 t C lost (3854 t CO2e) | ||||||||
Scenario B | ||||||||
Area (ha) at T0 | 120 | 270 | 480 | 360 | 70 | 110 | 30 | 1440 ha |
Carbon storage in woody vegetation for this ecosystem State at T0 | 6960 | 11 340 | 9120 | 3240 | 630 | 220 | 0 | 31 510 t C@T0 |
Area (ha) at T1 | 120 | 380 | 430 | 310 | 60 | 90 | 50 | 1440 ha |
Carbon storage in woody vegetation for this ecosystem State at T1 | 6960 | 15 960 | 8170 | 2790 | 540 | 180 | 0 | 34 600 t C@T1 |
= 3090 t C gained (11 340 t CO2e) |
In Scenario A, the farm is managed with a focus on intensifying livestock production that threatens the remaining carbon stored in vegetation, leading to its slow decline. In Scenario B, remnant areas are managed with a low-input approach, with a focus on protecting and regenerating the values of those areas. In both scenarios, 120 ha of high-value native woodland (State 1A) is protected under a conservation/biodiversity scheme. In Scenario B, some pasture intensification does occur (20 ha), but this is limited to an area already quite modified from the original vegetation condition (i.e. in State 4 or State 5). The values for carbon storage in different STM classes are approximate only and based on the rapid literature review method outlined in the text. Figures are for carbon storage in vegetation only and do not include consideration of soil carbon. T0 and T1 refer to a baseline assessment at Time 0, and a follow up assessment at Time 1 several years later.
Under the GHG Protocol Agricultural Guidance for carbon accounting (WRI and WBCSD 2020), both gains and losses of carbon should be recorded in the GHG inventory of a farm business (i.e. as land-use change or the loss/gain of biogenic carbon). The value of using an NCA framework with opening and closing balances as shown in these hypothetical examples is that it creates transparency as to where in the landscape, and under what conditions, carbon losses and gains are likely to be occurring. This improves the capacity of the land manager to make decisions based on the longer-term (i.e. decades) protection of natural capital, as well as shorter-term agricultural productivity. It also enables managers to maximise agricultural productivity only on areas of the landscape where those practices are unlikely to compromise other important ecological values.
Implications
A range of methods and calculators already exists for assessing both the carbon and biodiversity impacts of farm operations. These are part of an emerging assembly of tools seeking to recognise sustainability and conservation outcomes on farms. Ideally, sustainable farm management will not compromise overall business goals, and in some cases may enhance them through creation of new income streams through a range of mechanisms such as stewardship payments or supply-chain recognition. Sustainability aspirations are likely to be best met when different components of the farm landscape are used in ways that are optimal to the full range of ecosystem services that different areas can provide, rather than provisioning services only and in the context of the full range of business goals, which increasingly are likely to include reducing carbon footprints and protecting biodiversity by using nature-based solutions (such as low-input grazing).
Ultimately, management of farm ecosystems to optimise sustainability (including climate mitigation) while continuing farm production will only be as good as the information that supports any decision-making (Keith et al. 2021). The approach to the compilation of NCA used in these experimental accounts speaks to this need by providing a record of the range of production and/or ecological outcomes that might be possible from different areas of the farm landscape.
Increasingly, there is pressure for agriculture to reduce its carbon footprint and potentially be a part of climate change solutions. This means that a range of carbon footprint recognition schemes, either as offsets or through supply chain recognition are now either available to farmers or are under development. For some examples and discussions see Çapitals Coalition (2020), van der Lugt et al. (2020) and Kashmanian and Moore (2014). Such schemes can help compensate farmers for any loss of short-term agricultural productivity that might occur, or be perceived to be occurring, where converting to lower-input, more nature-based, grazing systems. Financial recognition via such schemes can also help compensate for costs such as additional fencing and water infrastructure that might be required to manage stock in ways that support woodland protection and regeneration.
A current example of one of these schemes is the Australian Government’s human-induced regeneration (which falls under the Emissions Reduction Fund; ERF) where carbon credits can be generated through altered management of woodland ecosystems to that which facilitates natural regeneration of the woody biomass (Clean Energy Regulator 2021). In general, uptake of this scheme has been good in lower- productivity rangeland areas, but minimal in temperate areas where the private opportunity costs are seen as greater (Waters et al. 2020). If there was wider recognition and awareness of the dual carbon and biodiversity value of temperate remnant woodland areas, this could help counter the shorter-term rewards anticipated from more intensive agronomic practices. We suggest the NCA process enables a robust, transparent and detailed recording of carbon (and biodiversity) losses and gains that are a consequence of those management actions.
Rather than trading carbon for offset purposes, farm businesses may choose to retain carbon gains as offsets for their own future emissions in the farm enterprise or to ‘inset’ emissions along their supply chain (World Economic Forum 2022). This alternative may be more appropriate where areas are of insufficient size to access ERF type schemes or where the costs associated with participation in an ERF program outweigh the potential income from carbon credit sales. The NCA process may also allow recognition of low-input approaches to grazing that are already in place and, therefore, cannot be recognised under the ERF.
Encouraging the uptake of frameworks that recognise carbon storage as well as protecting areas of remaining native biodiversity on farms has important advantages. Particularly significant is that carbon already stored in existing vegetation and soils is very stable, so better to maintain what is there in the first place than have to restore it later. The alternative scenario of carbon stored in vegetation and soils being lost through agricultural intensification, with replacement by tree planting now or in coming years, means it will take many decades or centuries for the lost carbon to be recovered (Keith et al. 2021). There is also a high chance that plantings intended to replace lost woodland vegetation are at risk of failure as they will be less resilient than a diverse native ecosystem that is continually regenerating and has evolved to adapt to local conditions (Keith et al. 2021). It is important to also recognise that the protection of the floristically diverse, deep-rooted perennial pastures associated with native grassy woodlands will have a greater capacity to adapt to future climate change scenarios than fertilised and sown pastures that are inherently unstable and commonly annual dominated (Simpson and Langford 1996; Bird et al. 2004). Thus, in many cases, these relatively diverse native areas can provide resilient fodder and shelter for livestock production, alongside a range of other ecosystem services, particularly in the face of climate change (Lavorel et al. 2015). Although likely to be of lower productivity in favourable seasons, these areas require fewer, potentially increasingly costly, external inputs to support production, while also providing a range of additional ecosystem services and may potentially be an important contributor to business profitability. Greater attention to preserving these areas may therefore be important for the agricultural industry to adapt to climate change in multiple ways.
The experimental NCAs discussed in this paper were underpinned by previous sustainability frameworks that identified landscape configurations seeking to balance long-term ecological sustainability and agronomic production (McIntyre et al. 2002; Smith et al. 2013). This accounting approach builds on that previous work by enabling some quantification of those landscape attributes and what each component contributes to the overall goals of a farm business.
With carbon and biodiversity in the landscape often intimately connected (Barber et al. 2020), a process that enables the two to be considered together is likely to be invaluable for supply-chain recognition where that is appropriate. The current urgency for climate action, biodiversity protection and awareness of the value of nature-based solutions provides incentives that have not previously existed for farmers to manage in ways that protect remnant areas. Ultimately this has the potential to better conserve remnant woodlands and woody vegetation on farms in temperate Australia where these areas persist.
This viewpoint paper, based on experimental farm-scale NCA, communicates a strategy that could encourage greater implementation of low-input grazing practices in appropriate, partially modified areas, as an alternative to common agronomic practices that can have a heavy carbon footprint and threaten remaining biodiversity. Further development of farm-scale NCA methodology is required to ensure that methods are both robust and cost-effective. It is also important to gain an improved understanding of trade-offs between carbon and biodiversity protection (and potentially regeneration) and long-term agricultural productivity and profitability, especially in an increasingly variable climate. With the alternative carbon and biodiversity value of remnant areas and their important role in balancing farm emissions being made apparent through the NCA process, rather than being seen as less productive areas to be altered, there is an increased likelihood of remnant vegetation management being managed in a way that balances the need for profitable businesses, while also protecting biodiversity and reducing overall carbon footprints. Ultimately this could enable a segment of the agricultural industry to be better recognised for good environmental stewardship.
Data availability
The data that support this study cannot be publicly shared due to ethical or privacy reasons but may be shared upon reasonable request to the corresponding author if appropriate.
Acknowledgements
We gratefully appreciate comments from Heather Keith and AWI staff who informally reviewed early versions of the manuscript and whose comments greatly improved the clarity of the paper. We sincerely thank the two anonymous reviewers for their helpful feedback. We also acknowledge the contributions made by the demonstration farms where case studies were undertaken. AWI is grateful for its funding, which is primarily provided by Australian woolgrowers through a wool levy and by the Australian Government which provides a matching contribution for eligible R&D activities © 2022 Australian Wool Innovation Limited.
References
Australian Government, Department of Agriculture, Water and Environment (2022a) Private Landholders. Available at https://www.awe.gov.au/agriculture-land/land/nrs/getting-involved/private-landholders [accessed 24 May 2022]
Australian Government, Department of Agriculture, Water and Environment (2022b) Species Profile and Threats Database. Available at http://www.environment.gov.au/cgi-bin/sprat/public/sprat.pl [accessed 24 May 2022]
Australian Government, Department of Industry, Science, Energy and Resources (2022) Full Carbon Accounting Model (FullCAM). Available at https://www.industry.gov.au/data-and-publications/full-carbon-accounting-model-fullcam [accessed 24 May 2022]
Australian Wool Innovation (2021) Natural Capital Accounting. Measuring Environmental Health with Natural Capital Accounting. Available at https://www.wool.com/land/regenerative-agriculture/natural-capital-accounting/ [accessed 9 November 2021]
Barrett GW, Ford HA, Recher H (1994) Conservation of woodland birds in a fragmented rural landscape. Pacific Conservation Biology 1(3), 245-256.
| Crossref | Google Scholar |
Bird PR, Jackson TT, Kearney GA, Saul GR, Waller RA, Whipp G (2004) The effect of improved pastures and grazing management on soil water storage on a basaltic plains site in south-west Victoria. Australian Journal of Experimental Agriculture 44, 559-569.
| Crossref | Google Scholar |
Çapitals Coalition (2020) Draft TEEB for Agriculture and Food: Operational Guidelines for Business. Available at http://teebweb.org/wp-content/uploads/2020/11/TEEBAgriFood-Operational-Guidelines.pdf [accessed 25 May 2022]
Clean Energy Regulator (2021) Human-induced regeneration of a permanent, even-aged, native forest. Available at http://www.cleanenergyregulator.gov.au/ERF/Choosing-a-project-type/Opportunities-for-the-land-sector/Vegetation-methods/Human-Induced%20regeneration%20of%20a%20permanent%20even-aged%20native%20forest [accessed 8 November 2021]
Dorrough J, Moxham C, Turner V, Sutter G (2006) Soil phosphorus and tree cover modify the effects of livestock grazing on plant species richness in Australian grassy woodland. Biological Conservation 130(3), 394-405.
| Crossref | Google Scholar |
Dorrough J, Moll J, Crosthwaite J (2007) Can intensification of temperate Australian livestock production systems save land for native biodiversity? Agriculture, Ecosystems & Environment 121, 222-232.
| Crossref | Google Scholar |
Eldridge DJ, Wilson BR (2002) Carbon storage in soil and vegetation in paired roadside sites in the box woodlands of eastern Australia. Australian Forestry 65(4), 268-272.
| Crossref | Google Scholar |
Gibbons P, Boak M (2002) The value of paddock trees for regional conservation in an agricultural landscape. Ecological Management & Restoration 3(3), 205-210.
| Crossref | Google Scholar |
Gibbons P, Lindenmayer DB, Fischer J, Manning AD, Weinberg A, Seddon J, Ryan P, Barrett G (2008) The future of scattered trees in agricultural landscapes. Conservation Biology 22(5), 1309-1319.
| Crossref | Google Scholar |
Kashmanian RM, Moore JR (2014) Building greater sustainability in supply chains. Environmental Quality & Management 23(4), 13-37.
| Google Scholar |
Keith H, Vardon M, Obst C, Young V, Houghton RA, Mackey B (2021) Evaluating nature-based solutions for climate mitigation and conservation requires comprehensive carbon accounting. Science of the Total Environment 769, 144341.
| Crossref | Google Scholar |
Kotliar NB, Wiens JA (1990) Multiple scales of patchiness and patch structure: a hierarchical framework for the study of heterogeneity. Oikos 59, 253-260.
| Crossref | Google Scholar |
Lavorel S, Colloff MJ, McIntyre S, Doherty MD, Murphy HT, Metcalfe DJ, Dunlop M, Williams RJ, Wise RM, Williams KJ (2015) Ecological mechanisms underpinning climate adaptation services. Global Change Biology 21(1), 12-31.
| Crossref | Google Scholar |
Lawrence R, Whalley R, Reid N, Rader R (2019) Short-duration rotational grazing leads to improvements in landscape functionality and increased perennial herbaceous plant cover. Agriculture, Ecosystems & Environment 281, 134-144.
| Crossref | Google Scholar |
McIntyre S (2005) Biodiversity attributes of different sward structures in grazed grassland. Ecological Management and Restoration 6, 71-73.
| Crossref | Google Scholar |
McIntyre S, Barrett GW (1992) Habitat variegation, an alternative to fragmentation. Conservation Biology 6(1), 146-147.
| Crossref | Google Scholar |
McIntyre S, Lavorel S (2007) A conceptual model of land use effects on the structure and function of herbaceous vegetation. Agriculture, Ecosystems & Environment 119(1–2), 11-21.
| Crossref | Google Scholar |
McIntyre S, Lewis J, Nicholls AO (2022) Potential for conservation and restoration following long-term pastoral use in a temperate forest–woodland–grassland mosaic on the Southern Tablelands, New South Wales. Cunninghamia 22, 027-044.
| Google Scholar |
Ogilvy S, O’Brien D, Lawrence R, Gardner M (2022) A natural capital accounting framework to communicate the environmental credentials of individual wool-producing businesses. Sustainability Accounting, Management and Policy Journal 13(4), 765-802.
| Crossref | Google Scholar |
Scott JM, Gaden CA, Edwards C, Paull DR, Marchant R, Hoad J, Sutherland H, Coventry T, Dutton P (2013) Selection of experimental treatments, methods used and evolution of management guidelines for comparing and measuring three grazed farmlet systems. Animal Production Science 53(8), 628-642.
| Crossref | Google Scholar |
Smith FP, Prober SM, House AP, McIntyre S (2013) Maximizing retention of native biodiversity in Australian agricultural landscapes: the 10:20:40:30 guidelines. Agriculture, Ecosystems & Environment 166, 35-45.
| Crossref | Google Scholar |
Tremont R (1994) Life-history attributes of plants in grazed and ungrazed grasslands on the Northern Tablelands of New South Wales. Australian Journal of Botany 42(5), 511-530.
| Crossref | Google Scholar |
Unc A, Altdorff D, Abakumov E, Adl S, Baldursson S, Bechtold M, Cattani DJ, Firbank LG, Grand S, Guðjónsdóttir M, Kallenbach C, Kedir AJ, Li P, McKenzie DB, Misra D, Nagano H, Neher DA, Niemi J, Oelbermann M, et al. (2021) Expansion of agriculture in Northern Cold-Climate Regions: a cross-sectoral perspective on opportunities and challenges. Frontiers in Sustainable Food Systems 5, 663448.
| Crossref | Google Scholar |
United Nations, European Commission, Food and Agriculture Organisation of the United Nations, Organisation for Economic Cooperation and Development, & World Bank Group (2014) ‘System of Environmental-Economic Accounting 2012—Experimental Ecosystem Accounting’. (p. 177) (United Nations) Available at https://unstats.un.org/unsd/envaccounting/seeaRev/eea_final_en.pdf [accessed 25 May 2022]
Waters C, Cowie A, Wang B, Simpson M, Gray J, Simmons A, Stephens S (2020) ‘Abatement opportunities from the agriculture sector in NSW: Modelling to support the development of the Primary Industries Productivity and Abatement Program.’ (NSW Department of Primary Industry) Available at https://www.dpi.nsw.gov.au/__data/assets/pdf_file/0005/1315562/FINAL_May2021Abatement-opprtunities-in-NSW.pdf [accessed 17 November 2021]
World Economic Forum (2022) Explainer: carbon insetting vs offsetting. Available at https://www.weforum.org/agenda/2022/03/carbon-insetting-vs-offsetting-an-explainer/
WRI, WBCSD (2020) ‘Greenhouse Gas Protocol Agricultural Guidance.’ p. 103. (World Resources Institute and World Business Council for Sustainable Development) Available at https://ghgprotocol.org/sites/default/files/standards/GHG%20Protocol%20Agricultural%20Guidance%20%28April%2026%29_0.pdf [accessed 8 November 2022]