Genome-wide DNA-binding profile of SRY-box transcription factor 3 (SOX3) in mouse testes
Dale McAninch

A School of Biological Sciences and Robinson Research Institute, University of Adelaide, North Terrace, Adelaide, SA 5005, Australia.
B Adelaide Medical School, University of Adelaide, North Terrace, Adelaide, SA 5005, Australia.
C Precision Medicine Theme, South Australia Health and Medical Research Institute, North Terrace, Adelaide, SA 5000, Australia.
D Corresponding author. Email: paul.thomas@adelaide.edu.au
Reproduction, Fertility and Development 32(16) 1260-1270 https://doi.org/10.1071/RD20108
Submitted: 17 April 2020 Accepted: 1 October 2020 Published: 10 November 2020
Abstract
Spermatogenesis is the male version of gametogenesis, where germ cells are transformed into haploid spermatozoa through a tightly controlled series of mitosis, meiosis and differentiation. This process is reliant on precisely timed changes in gene expression controlled by several different hormonal and transcriptional mechanisms. One important transcription factor is SRY-box transcription factor 3 (SOX3), which is transiently expressed within the uncommitted spermatogonial stem cell population. Sox3-null mouse testes exhibit a block in spermatogenesis, leading to infertility or subfertility. However, the molecular role of SOX3 during spermatogonial differentiation remains poorly understood because the genomic regions targeted by this transcription factor have not been identified. In this study we used chromatin immunoprecipitation sequencing to identify and characterise the endogenous genome-wide binding profile of SOX3 in mouse testes at Postnatal Day 7. We show that neurogenin3 (Neurog3 or Ngn3) is directly targeted by SOX3 in spermatogonial stem cells via a novel testes-specific binding site. We also implicate SOX3, for the first time, in direct regulation of histone gene expression and demonstrate that this function is shared by both neural progenitors and testes, and with another important transcription factor required for spermatogenesis, namely promyelocytic leukaemia zinc-finger (PLZF). Together, these data provide new insights into the function of SOX3 in different stem cell contexts.
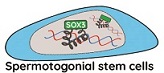
Keywords: chromatin immunoprecipitation (ChIP), gene regulation, histones, spermatogonia, testis, transcription factor.
References
Adikusuma, F., Pederick, D., McAninch, D., Hughes, J., and Thomas, P. (2017). Functional equivalence of the SOX2 and SOX3 transcription factors in the developing mouse brain and testes. Genetics 206, 1495–1503.| Functional equivalence of the SOX2 and SOX3 transcription factors in the developing mouse brain and testes.Crossref | GoogleScholarGoogle Scholar | 28515211PubMed |
Ambrosetti, D. C., Basilico, C., and Dailey, L. (1997). Synergistic activation of the fibroblast growth factor 4 enhancer by Sox2 and Oct-3 depends on protein-protein interactions facilitated by a specific spatial arrangement of factor binding sites. Mol. Cell. Biol. 17, 6321–6329.
| Synergistic activation of the fibroblast growth factor 4 enhancer by Sox2 and Oct-3 depends on protein-protein interactions facilitated by a specific spatial arrangement of factor binding sites.Crossref | GoogleScholarGoogle Scholar | 9343393PubMed |
Amoutzias, G. D., Robertson, D. L., Van de Peer, Y., and Oliver, S. G. (2008). Choose your partners: dimerization in eukaryotic transcription factors. Trends Biochem. Sci. 33, 220–229.
| Choose your partners: dimerization in eukaryotic transcription factors.Crossref | GoogleScholarGoogle Scholar | 18406148PubMed |
Bailey, T. L., Johnson, J., Grant, C. E., and Noble, W. S. (2015). The MEME suite. Nucleic Acids Res. 43, W39–W49.
| The MEME suite.Crossref | GoogleScholarGoogle Scholar | 25953851PubMed |
Bergsland, M., Ramskold, D., Zaouter, C., Klum, S., Sandberg, R., and Muhr, J. (2011). Sequentially acting Sox transcription factors in neural lineage development. Genes Dev. 25, 2453–2464.
| Sequentially acting Sox transcription factors in neural lineage development.Crossref | GoogleScholarGoogle Scholar | 22085726PubMed |
Boskovic, A., and Torres-Padilla, M. E. (2013). How mammals pack their sperm: a variant matter. Genes Dev. 27, 1635–1639.
| How mammals pack their sperm: a variant matter.Crossref | GoogleScholarGoogle Scholar | 23913918PubMed |
Buaas, F. W., Kirsh, A. L., Sharma, M., McLean, D. J., Morris, J. L., Griswold, M. D., de Rooij, D. G., and Braun, R. E. (2004). Plzf is required in adult male germ cells for stem cell self-renewal. Nat. Genet. 36, 647–652.
| Plzf is required in adult male germ cells for stem cell self-renewal.Crossref | GoogleScholarGoogle Scholar | 15156142PubMed |
Bundschuh, R., Hayot, F., and Jayaprakash, C. (2003). The role of dimerization in noise reduction of simple genetic networks. J. Theor. Biol. 220, 261–269.
| The role of dimerization in noise reduction of simple genetic networks.Crossref | GoogleScholarGoogle Scholar | 12468297PubMed |
Buske, F. A., Boden, M., Bauer, D. C., and Bailey, T. L. (2010). Assigning roles to DNA regulatory motifs using comparative genomics. Bioinformatics 26, 860–866.
| Assigning roles to DNA regulatory motifs using comparative genomics.Crossref | GoogleScholarGoogle Scholar | 20147307PubMed |
Bylund, M., Andersson, E., Novitch, B. G., and Muhr, J. (2003). Vertebrate neurogenesis is counteracted by Sox1–3 activity. Nat. Neurosci. 6, 1162–1168.
| Vertebrate neurogenesis is counteracted by Sox1–3 activity.Crossref | GoogleScholarGoogle Scholar | 14517545PubMed |
Costoya, J. A., Hobbs, R. M., Barna, M., Cattoretti, G., Manova, K., Sukhwani, M., Orwig, K. E., Wolgemuth, D. J., and Pandolfi, P. P. (2004). Essential role of Plzf in maintenance of spermatogonial stem cells. Nat. Genet. 36, 653–659.
| Essential role of Plzf in maintenance of spermatogonial stem cells.Crossref | GoogleScholarGoogle Scholar | 15156143PubMed |
Crooks, G. E., Hon, G., Chandonia, J. M., and Brenner, S. E. (2004). WebLogo: a sequence logo generator. Genome Res. 14, 1188–1190.
| WebLogo: a sequence logo generator.Crossref | GoogleScholarGoogle Scholar | 15173120PubMed |
Dann, C. T., Alvarado, A. L., Molyneux, L. A., Denard, B. S., Garbers, D. L., and Porteus, M. H. (2008). Spermatogonial stem cell self-renewal requires OCT4, a factor downregulated during retinoic acid-induced differentiation. Stem Cells 26, 2928–2937.
| Spermatogonial stem cell self-renewal requires OCT4, a factor downregulated during retinoic acid-induced differentiation.Crossref | GoogleScholarGoogle Scholar | 18719224PubMed |
Feng, J., Liu, T., and Zhang, Y. (2011). Using MACS to identify peaks from ChIP-Seq data. Curr. Protoc. Bioinformatics 34, 2.14.1–2.14.14.
| Using MACS to identify peaks from ChIP-Seq data.Crossref | GoogleScholarGoogle Scholar |
Filipponi, D., Hobbs, R. M., Ottolenghi, S., Rossi, P., Jannini, E. A., Pandolfi, P. P., and Dolci, S. (2007). Repression of kit expression by Plzf in germ cells. Mol. Cell. Biol. 27, 6770–6781.
| Repression of kit expression by Plzf in germ cells.Crossref | GoogleScholarGoogle Scholar | 17664282PubMed |
Grant, C. E., Bailey, T. L., and Noble, W. S. (2011). FIMO: scanning for occurrences of a given motif. Bioinformatics 27, 1017–1018.
| FIMO: scanning for occurrences of a given motif.Crossref | GoogleScholarGoogle Scholar | 21330290PubMed |
Han, J., Zhang, Z., and Wang, K. (2018). 3C and 3C-based techniques: the powerful tools for spatial genome organization deciphering. Mol. Cytogenet. 11, 21.
| 3C and 3C-based techniques: the powerful tools for spatial genome organization deciphering.Crossref | GoogleScholarGoogle Scholar | 29541161PubMed |
Heinz, S., Benner, C., Spann, N., Bertolino, E., Lin, Y. C., Laslo, P., Cheng, J. X., Murre, C., Singh, H., and Glass, C. K. (2010). Simple combinations of lineage-determining transcription factors prime cis-regulatory elements required for macrophage and B cell identities. Mol. Cell 38, 576–589.
| Simple combinations of lineage-determining transcription factors prime cis-regulatory elements required for macrophage and B cell identities.Crossref | GoogleScholarGoogle Scholar | 20513432PubMed |
Huh, N. E., Hwang, I. W., Lim, K., You, K. H., and Chae, C. B. (1991). Presence of a bi-directional S phase-specific transcription regulatory element in the promoter shared by testis-specific TH2A and TH2B histone genes. Nucleic Acids Res. 19, 93–98.
| Presence of a bi-directional S phase-specific transcription regulatory element in the promoter shared by testis-specific TH2A and TH2B histone genes.Crossref | GoogleScholarGoogle Scholar | 2011515PubMed |
Kamachi, Y., and Kondoh, H. (2013). Sox proteins: regulators of cell fate specification and differentiation. Development 140, 4129–4144.
| Sox proteins: regulators of cell fate specification and differentiation.Crossref | GoogleScholarGoogle Scholar | 24086078PubMed |
Kamachi, Y., Uchikawa, M., and Kondoh, H. (2000). Pairing SOX off: with partners in the regulation of embryonic development. Trends Genet. 16, 182–187.
| Pairing SOX off: with partners in the regulation of embryonic development.Crossref | GoogleScholarGoogle Scholar | 10729834PubMed |
Kamachi, Y., Uchikawa, M., Tanouchi, A., Sekido, R., and Kondoh, H. (2001). Pax6 and SOX2 form a co-DNA-binding partner complex that regulates initiation of lens development. Genes Dev. 15, 1272–1286.
| Pax6 and SOX2 form a co-DNA-binding partner complex that regulates initiation of lens development.Crossref | GoogleScholarGoogle Scholar | 11358870PubMed |
Koubi, M., Poplineau, M., Vernerey, J., N’Guyen, L., Tiberi, G., Garciaz, S., El-Kaoutari, A., Maqbool, M. A., Andrau, J. C., Guillouf, C., Saurin, A. J., and Duprez, E. (2018). Regulation of the positive transcriptional effect of PLZF through a non-canonical EZH2 activity. Nucleic Acids Res. 46, 3339–3350.
| Regulation of the positive transcriptional effect of PLZF through a non-canonical EZH2 activity.Crossref | GoogleScholarGoogle Scholar | 29425303PubMed |
Kulakovskiy, I. V., Vorontsov, I. E., Yevshin, I. S., Sharipov, R. N., Fedorova, A. D., Rumynskiy, E. I., Medvedeva, Y. A., Magana-Mora, A., Bajic, V. B., Papatsenko, D. A., Kolpakov, F. A., and Makeev, V. J. (2018). HOCOMOCO: towards a complete collection of transcription factor binding models for human and mouse via large-scale ChIP-Seq analysis. Nucleic Acids Res. 46, D252–D259.
| HOCOMOCO: towards a complete collection of transcription factor binding models for human and mouse via large-scale ChIP-Seq analysis.Crossref | GoogleScholarGoogle Scholar | 29140464PubMed |
Langmead, B. (2010). Aligning short sequencing reads with Bowtie. Curr. Protoc. Bioinformatics 32, 11.7.1–11.7.14.
| Aligning short sequencing reads with Bowtie.Crossref | GoogleScholarGoogle Scholar |
Laronda, M. M., and Jameson, J. L. (2011). Sox3 functions in a cell-autonomous manner to regulate spermatogonial differentiation in mice. Endocrinology 152, 1606–1615.
| Sox3 functions in a cell-autonomous manner to regulate spermatogonial differentiation in mice.Crossref | GoogleScholarGoogle Scholar | 21248142PubMed |
Li, R., Vannitamby, A., Zhang, J. G., Fehmel, E. L., Southwell, B. R., and Hutson, J. M. (2015). Oct4-GFP expression during transformation of gonocytes into spermatogonial stem cells in the perinatal mouse testis. J. Pediatr. Surg. 50, 2084–2089.
| Oct4-GFP expression during transformation of gonocytes into spermatogonial stem cells in the perinatal mouse testis.Crossref | GoogleScholarGoogle Scholar | 26386877PubMed |
Liu, C. F., and Lefebvre, V. (2015). The transcription factors SOX9 and SOX5/SOX6 cooperate genome-wide through super-enhancers to drive chondrogenesis. Nucleic Acids Res. 43, 8183–8203.
| The transcription factors SOX9 and SOX5/SOX6 cooperate genome-wide through super-enhancers to drive chondrogenesis.Crossref | GoogleScholarGoogle Scholar | 26150426PubMed |
Lovelace, D. L., Gao, Z., Mutoji, K., Song, Y. C., Ruan, J., and Hermann, B. P. (2016). The regulatory repertoire of PLZF and SALL4 in undifferentiated spermatogonia. Development 143, 1893–1906.
| The regulatory repertoire of PLZF and SALL4 in undifferentiated spermatogonia.Crossref | GoogleScholarGoogle Scholar | 27068105PubMed |
Ma, W., Noble, W. S., and Bailey, T. L. (2014). Motif-based analysis of large nucleotide data sets using MEME-ChIP. Nat. Protoc. 9, 1428–1450.
| Motif-based analysis of large nucleotide data sets using MEME-ChIP.Crossref | GoogleScholarGoogle Scholar | 24853928PubMed |
McAninch, D., and Thomas, P. (2014). Identification of highly conserved putative developmental enhancers bound by SOX3 in neural progenitors using ChIP-Seq. PLoS One 9, e113361.
| Identification of highly conserved putative developmental enhancers bound by SOX3 in neural progenitors using ChIP-Seq.Crossref | GoogleScholarGoogle Scholar | 25409526PubMed |
McAninch, D., Makela, H. M., La, H. M., Hughes, J. N., Lovell-Badge, R., Hobbs, R. M., and Thomas, P. Q. (2020). SOX3 promotes generation of committed spermatogonia in postnatal mouse testes. Sci. Rep. 10, 6751.
| SOX3 promotes generation of committed spermatogonia in postnatal mouse testes.Crossref | GoogleScholarGoogle Scholar | 32317665PubMed |
McLean, C. Y., Bristor, D., Hiller, M., Clarke, S. L., Schaar, B. T., Lowe, C. B., Wenger, A. M., and Bejerano, G. (2010). GREAT improves functional interpretation of cis-regulatory regions. Nat. Biotechnol. 28, 495–501.
| GREAT improves functional interpretation of cis-regulatory regions.Crossref | GoogleScholarGoogle Scholar | 20436461PubMed |
Montellier, E., Boussouar, F., Rousseaux, S., Zhang, K., Buchou, T., Fenaille, F., Shiota, H., Debernardi, A., Hery, P., Curtet, S., Jamshidikia, M., Barral, S., Holota, H., Bergon, A., Lopez, F., Guardiola, P., Pernet, K., Imbert, J., Petosa, C., Tan, M., Zhao, Y., Gerard, M., and Khochbin, S. (2013). Chromatin-to-nucleoprotamine transition is controlled by the histone H2B variant TH2B. Genes Dev. 27, 1680–1692.
| Chromatin-to-nucleoprotamine transition is controlled by the histone H2B variant TH2B.Crossref | GoogleScholarGoogle Scholar | 23884607PubMed |
Nakagawa, T., Nabeshima, Y., and Yoshida, S. (2007). Functional identification of the actual and potential stem cell compartments in mouse spermatogenesis. Dev. Cell 12, 195–206.
| Functional identification of the actual and potential stem cell compartments in mouse spermatogenesis.Crossref | GoogleScholarGoogle Scholar | 17276338PubMed |
Ng, C. K., Li, N. X., Chee, S., Prabhakar, S., Kolatkar, P. R., and Jauch, R. (2012). Deciphering the Sox–Oct partner code by quantitative cooperativity measurements. Nucleic Acids Res. 40, 4933–4941.
| Deciphering the Sox–Oct partner code by quantitative cooperativity measurements.Crossref | GoogleScholarGoogle Scholar | 22344693PubMed |
Oatley, J. M., Oatley, M. J., Avarbock, M. R., Tobias, J. W., and Brinster, R. L. (2009). Colony stimulating factor 1 is an extrinsic stimulator of mouse spermatogonial stem cell self-renewal. Development 136, 1191–1199.
| Colony stimulating factor 1 is an extrinsic stimulator of mouse spermatogonial stem cell self-renewal.Crossref | GoogleScholarGoogle Scholar | 19270176PubMed |
Ohba, S., He, X., Hojo, H., and McMahon, A. P. (2015). Distinct transcriptional programs underlie Sox9 regulation of the mammalian chondrocyte. Cell Rep. 12, 229–243.
| Distinct transcriptional programs underlie Sox9 regulation of the mammalian chondrocyte.Crossref | GoogleScholarGoogle Scholar | 26146088PubMed |
Palena, C. M., Gonzalez, D. H., and Chan, R. L. (1999). A monomer–dimer equilibrium modulates the interaction of the sunflower homeodomain leucine-zipper protein Hahb-4 with DNA. Biochem. J. 341, 81–87.
| 10377247PubMed |
Raverot, G., Weiss, J., Park, S. Y., Hurley, L., and Jameson, J. L. (2005). Sox3 expression in undifferentiated spermatogonia is required for the progression of spermatogenesis. Dev. Biol. 283, 215–225.
| Sox3 expression in undifferentiated spermatogonia is required for the progression of spermatogenesis.Crossref | GoogleScholarGoogle Scholar | 15893302PubMed |
Reiprich, S., and Wegner, M. (2015). From CNS stem cells to neurons and glia: Sox for everyone. Cell Tissue Res. 359, 111–124.
| From CNS stem cells to neurons and glia: Sox for everyone.Crossref | GoogleScholarGoogle Scholar | 24894327PubMed |
Rizzoti, K., Brunelli, S., Carmignac, D., Thomas, P. Q., Robinson, I. C., and Lovell-Badge, R. (2004). SOX3 is required during the formation of the hypothalamo–pituitary axis. Nat. Genet. 36, 247–255.
| SOX3 is required during the formation of the hypothalamo–pituitary axis.Crossref | GoogleScholarGoogle Scholar | 14981518PubMed |
Rogers, N., Cheah, P. S., Szarek, E., Banerjee, K., Schwartz, J., and Thomas, P. (2013). Expression of the murine transcription factor SOX3 during embryonic and adult neurogenesis. Gene Expr. Patterns 13, 240–248.
| Expression of the murine transcription factor SOX3 during embryonic and adult neurogenesis.Crossref | GoogleScholarGoogle Scholar | 23665444PubMed |
Sakaki-Yumoto, M., Kobayashi, C., Sato, A., Fujimura, S., Matsumoto, Y., Takasato, M., Kodama, T., Aburatani, H., Asashima, M., Yoshida, N., and Nishinakamura, R. (2006). The murine homolog of SALL4, a causative gene in Okihiro syndrome, is essential for embryonic stem cell proliferation, and cooperates with Sall1 in anorectal, heart, brain and kidney development. Development 133, 3005–3013.
| The murine homolog of SALL4, a causative gene in Okihiro syndrome, is essential for embryonic stem cell proliferation, and cooperates with Sall1 in anorectal, heart, brain and kidney development.Crossref | GoogleScholarGoogle Scholar | 16790473PubMed |
Shinagawa, T., Huynh, L. M., Takagi, T., Tsukamoto, D., Tomaru, C., Kwak, H. G., Dohmae, N., Noguchi, J., and Ishii, S. (2015). Disruption of Th2a and Th2b genes causes defects in spermatogenesis. Development 142, 1287–1292.
| Disruption of Th2a and Th2b genes causes defects in spermatogenesis.Crossref | GoogleScholarGoogle Scholar | 25742800PubMed |
Siepel, A., Bejerano, G., Pedersen, J. S., Hinrichs, A. S., Hou, M., Rosenbloom, K., Clawson, H., Spieth, J., Hillier, L. W., Richards, S., Weinstock, G. M., Wilson, R. K., Gibbs, R. A., Kent, W. J., Miller, W., and Haussler, D. (2005). Evolutionarily conserved elements in vertebrate, insect, worm, and yeast genomes. Genome Res. 15, 1034–1050.
| Evolutionarily conserved elements in vertebrate, insect, worm, and yeast genomes.Crossref | GoogleScholarGoogle Scholar | 16024819PubMed |
Siggers, T., and Gordan, R. (2014). Protein–DNA binding: complexities and multi-protein codes. Nucleic Acids Res. 42, 2099–2111.
| Protein–DNA binding: complexities and multi-protein codes.Crossref | GoogleScholarGoogle Scholar | 24243859PubMed |
Singh, R., Bassett, E., Chakravarti, A., and Parthun, M. R. (2018). Replication-dependent histone isoforms: a new source of complexity in chromatin structure and function. Nucleic Acids Res. 46, 8665–8678.
| Replication-dependent histone isoforms: a new source of complexity in chromatin structure and function.Crossref | GoogleScholarGoogle Scholar | 30165676PubMed |
Song, H. W., and Wilkinson, M. F. (2014). Transcriptional control of spermatogonial maintenance and differentiation. Semin. Cell Dev. Biol. 30, 14–26.
| Transcriptional control of spermatogonial maintenance and differentiation.Crossref | GoogleScholarGoogle Scholar | 24560784PubMed |
Tanaka, S., Kamachi, Y., Tanouchi, A., Hamada, H., Jing, N., and Kondoh, H. (2004). Interplay of SOX and POU factors in regulation of the Nestin gene in neural primordial cells. Mol. Cell. Biol. 24, 8834–8846.
| Interplay of SOX and POU factors in regulation of the Nestin gene in neural primordial cells.Crossref | GoogleScholarGoogle Scholar | 15456859PubMed |
Ueda, J., Harada, A., Urahama, T., Machida, S., Maehara, K., Hada, M., Makino, Y., Nogami, J., Horikoshi, N., Osakabe, A., Taguchi, H., Tanaka, H., Tachiwana, H., Yao, T., Yamada, M., Iwamoto, T., Isotani, A., Ikawa, M., Tachibana, T., Okada, Y., Kimura, H., Ohkawa, Y., Kurumizaka, H., and Yamagata, K. (2017). Testis-specific histone variant H3t gene is essential for entry into spermatogenesis. Cell Rep. 18, 593–600.
| Testis-specific histone variant H3t gene is essential for entry into spermatogenesis.Crossref | GoogleScholarGoogle Scholar | 28099840PubMed |
Weiss, J., Meeks, J. J., Hurley, L., Raverot, G., Frassetto, A., and Jameson, J. L. (2003). Sox3 is required for gonadal function, but not sex determination, in males and females. Mol. Cell. Biol. 23, 8084–8091.
| Sox3 is required for gonadal function, but not sex determination, in males and females.Crossref | GoogleScholarGoogle Scholar | 14585968PubMed |
Wilson, M., and Koopman, P. (2002). Matching SOX: partner proteins and co-factors of the SOX family of transcriptional regulators. Curr. Opin. Genet. Dev. 12, 441–446.
| Matching SOX: partner proteins and co-factors of the SOX family of transcriptional regulators.Crossref | GoogleScholarGoogle Scholar | 12100890PubMed |
Wood, H. B., and Episkopou, V. (1999). Comparative expression of the mouse Sox1, Sox2 and Sox3 genes from pre-gastrulation to early somite stages. Mech. Dev. 86, 197–201.
| Comparative expression of the mouse Sox1, Sox2 and Sox3 genes from pre-gastrulation to early somite stages.Crossref | GoogleScholarGoogle Scholar | 10446282PubMed |
Yuan, H., Corbi, N., Basilico, C., and Dailey, L. (1995). Developmental-specific activity of the FGF-4 enhancer requires the synergistic action of Sox2 and Oct-3. Genes Dev. 9, 2635–2645.
| Developmental-specific activity of the FGF-4 enhancer requires the synergistic action of Sox2 and Oct-3.Crossref | GoogleScholarGoogle Scholar | 7590241PubMed |