Efficiency of the zinc chelator 1,10-phenanthroline for assisted oocyte activation following ICSI in pigs
Olinda Briski A B , Juan P. Cabeza


A
B
C
D
Abstract
In vitro embryo production in pigs is an important tool for advancing biomedical research. Intracytoplasmic sperm injection (ICSI) circumvents the polyspermy problems associated with conventional IVF in porcine. However, the suboptimal efficiency for ICSI in pigs requires new strategies to increase blastocyst formation rates.
To investigate novel methods for assisted activation using the zinc chelator 1,10-phenanthroline (PHEN), and to improve embryo developmental competence and quality of ICSI porcine blastocyst.
ICSI embryos were treated with PHEN after or before sperm injection, recording pronuclear formation, blastocyst rate and the expression of SMARCA4, OCT4, SOX2 and CDX2.
Neither electrical nor PHEN significantly improves pronuclear formation rates before or after ICSI. Following in vitro culture to the blastocyst stage, no significant differences were observed in developmental rates among the groups. Moreover, the use of PHEN did not alter the total cell number or the expression of OCT4, SOX2 and CDX2 in pig ICSI blastocysts.
Assisted oocyte activation with PHEN does not affect the preimplantation development of ICSI-derived pig embryos.
These results hold significance in refining and advancing the application of assisted oocyte activation techniques. They offer insights into addressing fertility issues and propelling advancements in human and animal reproductive medicine.
Keywords: assisted oocyte activation, chelating agent, in vitro breeding, pluripotency, preimplantation embryo development, swine.
Introduction
The polyspermy challenge associated with conventional IVF protocols has prompted the utilisation of intracytoplasmic sperm injection (ICSI) as an alternative approach in porcine reproductive techniques (Nguyen et al. 2021; Sun et al. 2022). Moreover, ICSI has been instrumental in generating transgenic pigs (Kurome et al. 2006; Yong et al. 2006; Umeyama et al. 2012; Watanabe et al. 2012; Matsunari et al. 2014) and, more recently, the advancement of the CRISPR-Cas9 system has further allowed the co-injection of gene-editing tools together with the sperm, as reported in humans (Ma et al. 2017) and in pigs (Briski et al. 2024). Despite the potential advantages of ICSI, it still presents biological limitations in pigs, and its efficiency remains low. As reported for cattle, failures in sperm nucleus decondensation and male pronuclear formation have been described after ICSI in pigs, and more than half of the oocytes after ICSI exhibited this phenomenon (Lee et al. 2003; Nakai et al. 2016a). Additionally, delayed cleavage and compromised development in ICSI porcine embryos in comparison to IVF embryos have been reported (Nakai et al. 2014). These findings could be associated with failures in oocyte activation after sperm injection, including reported alterations in sperm-specific phospholipase C (PLCζ) release and calcium oscillations (Nakai et al. 2016a).
To enhance ICSI efficiency, various strategies focused on sperm pre-treatments and/or oocyte-assisted activation (AOA) have been investigated (García-Roselló et al. 2009). Oocyte-assisted activation to support ICSI embryo development has been employed in various species (Salamone et al. 2017; Briski and Salamone 2022), including humans in which achievement of normal offspring births has been reported (Taylor et al. 2010; Fesahat et al. 2020) and mice, using sperm lacking PLCζ (Hirose et al. 2023). AOA through chemical or physical agents was attempted for improvement in blastocyst rates in pigs (Probst and Rath 2003; Kikuchi et al. 2006; Nakai et al. 2006, 2024; Tian et al. 2006) and, in some cases, achieving births (Nakai et al. 2003). Despite these strategies, to date, there is no standardised and efficient AOA protocol for ICSI in pigs. Interestingly, recent studies have shown high oocyte activation rates using zinc chelators in pigs (Uh et al. 2019, 2022; Cabeza et al. 2022). Although one of the most commonly used zinc chelators is N,N,N0,N0-tetrakis(2-pyridylmethyl) ethylenediamine (TPEN), other zinc chelators such as 1,10-phenanthroline (PHEN) has been recently tested for porcine oocyte activation (Uh et al. 2019; Cabeza et al. 2022), generating sufficient stimulus for oocyte activation without impacting parthenogenetic blastocyst quality (Cabeza et al. 2022).
Following fertilisation, dynamic chromatin remodelling takes place in both maternal and paternal DNA, a phenomenon directly correlated with oocyte activation (Ducibella and Fissore 2008; Suzuki et al. 2010; Kim et al. 2011; Kashir et al. 2022). An analysis of pronuclear levels of SWI/SNF related, matrix associated, actin dependent regulator of chromatin, subfamily a, member 4 (SMARCA4 or BRG1), a key chromatin remodeler, crucial for the maternal-to-zygotic transition (Sun et al. 2007; Eckersley-Maslin et al. 2018), could provide valuable insights into the quality of the activated zygotes (Gambini et al. 2020; Arroyo-Salvo et al. 2024). Furthermore, pluripotency-associated proteins, including POU class 5 homeobox 1 (POU5F1 or OCT4), SRY-box transcription factor 2 (SOX2) and caudal type homeobox 2 (CDX2), serve as established markers for blastocyst quality across various species. In porcine IVF blastocysts, OCT4 exhibits presence in both the inner cell mass (ICM) and the trophectoderm (TE), while SOX2 is specifically expressed in the ICM (Liu et al. 2015; Lee et al. 2021); CDX2 is uniquely expressed in TE cells (Kuijk et al. 2008; Vackova et al. 2011; Lim et al. 2013). Notably, most of these markers have not yet been characterised altogether in ICSI pig blastocysts to our knowledge.
This study aimed to assess the efficiency of the zinc chelator PHEN in enhancing ICSI outcomes in pigs. We compared pronuclear formation and SMARCA4 recruitment levels among different AOA treatments, and we evaluated preimplantation development rates and embryo quality by determining blastocyst total cell number and the localisation patterns of the key cell differentiation markers OCT4, SOX2 and CDX2.
Materials and methods
Unless otherwise stated, all chemicals were obtained from Merck KGaA, Darmstadt, Germany.
Experiment 1. Pronuclear formation after parthenogenetic activation and evaluation of SMARCA4 pronuclear levels after ICSI
After in vitro maturation, porcine oocytes were artificially activated with electrical activation (ICSI-e) or with PHEN (PHEN) or subjected to ICSI with boar sperm cells followed by PHEN AOA (ICSI-PHEN). Presumptive zygotes were fixed, stained and subjected to immunofluorescence to determine pronuclear formation and levels of SMARCA4. The proportion of embryos with one pronucleus (1-PN) or two pronuclei (2-PN) was recorded. Three biological replicates were performed; 20–80 zygotes/group.
Experiment 2. Pronuclear formation and recruitment of SMARCA4 using PHEN before or after ICSI
In vitro matured porcine oocytes were activated with PHEN immediately before (PHEN-ICSI) or after ICSI (ICSI-PHEN). Presumptive zygotes were fixed, stained and subjected to immunofluorescence as explained for Experiment 1. Three biological replicates were performed; 60–70 zygotes/group.
Experiment 3. In vitro development and blastocyst quality of ICSI and ICSI-PHEN embryos
The in vitro development up to the blastocyst stage was compared between ICSI and ICSI-PHEN. Blastocysts from each experimental group were fixed for immunofluorescence to determine the total number of cells, and the number of cells expressing OCT4, SOX2 and CDX2. Three biological replicates were performed for this experiment; 10–15 blastocyst/group.
Ethics and animal welfare statement
The experiments conducted in this manuscript did not necessitate approval from the Ethics and Animal Welfare Committee of the Faculty of Agriculture, University of Buenos Aires, as they did not involve any living animals (CICUAL-FAUBA, Res. CD 1476/19, Regulations for the care and use of animals for teaching, research, and services).
Recovery and in vitro maturation of porcine oocytes
Ovaries were collected from gilts at a local slaughterhouse located in the province of Buenos Aires and transported to the laboratory at around 25°C within 2 h of collection. Cumulus–oocyte complexes (COCs) from 3 to 6 mm in diameter follicles were aspirated using an 18-gauge needle attached to a 10-mL disposable syringe. Compact COCs were selected and matured in 500 μL drops of tissue culture medium (TCM-199- 31100–035; Gibco, Grand Island, NY, USA) under mineral oil (0121-1; Fisher Scientific, Pittsburgh, PA, USA), supplemented with 0.3 mM sodium pyruvate (P2256), 100 μM cysteamine (M9768), 5 μg/mL myo-inositol, 1 μg/mL insulin transferrin selenium (ITS; product no. 51300–044; Gibco), 1% antibiotic-antimycotic (15240–096 Gibco), 10% (v/v) porcine follicular fluid, 20 ng/mL epidermal growth factor (PHG0315, Gibco, Carlsbad, CA, USA) and 10 μg/mL of follicle-stimulating hormone (FSH) (NIH-FSH-P1Folltropin, Bioniche, Caufield Junction Caufield North, Vic., Australia). Maturation was performed at 38.5°C in a humidified atmosphere of 5% CO2 for 44 h.
Oocyte artificial activation
Cumulus cells of in vitro matured COCs were removed by vortexing for 1 min in hyaluronidase (H-4272) solution: 1 mg/mL in TALP-H. A group of oocytes was incubated for 30 min in 1 mM PHEN in TALP-H at 38.5°C (Cabeza et al. 2022) (PART-PHEN). PHEN’s effectiveness in reducing intracellular zinc levels in matured pig oocytes has already been described (Uh et al. 2019). For electrical activation, a group of oocytes were placed in the groove of a 0.5 mm wide chamber containing a solution of 0.3 M mannitol (M9647), 0.05 mM CaCl2 (C7902), 0.1 mM MgSO4 (63,138) and 1 mg/mL polyvinyl alcohol (P8136) and subjected to a single pulse of 1.2 kV/cm for 80 ms, using a BTX Electro-Cell Manipulator 2001 (BTX, San Diego, CA, USA) (Buemo et al. 2016) (PART-e). These oocyte activation protocols were also used for AOA. After ICSI, presumptive zygotes were activated with PHEN (ICSI-PHEN) or electrically (PART-e) and, in Experiment 2, a group of oocytes was activated with PHEN before ICSI (PHEN-ICSI).
Intracytoplasmic sperm injection
A pool of ejaculates from boars (F1 Camborough/Line 337, Pietrain x Duroc, Agroceres PIC) were used, diluted 1:1 with extender (Androstar Plus®, Minitube, Germany) and refrigerated at 17°C. Sperm selection was performed by swim-up, previously described by Navarro-Serna et al. (2021). Then, 0.5 μL of media containing motile spermatozoa was taken from the supernatant and placed in a 3-μL drop of 7% polyvinylpyrrolidone (PVP) solution (Irvine Scientific, Santa Ana, CA, USA). Mature oocytes were placed in the same ICSI Petri dish in a drop of TALP-H covered with mineral oil (0121-1; Fisher Scientific). The dish was then placed on the warm stage of a Nikon TiU inverted microscope with Narishige micromanipulators and air microinjectors. A single sperm was selected in the PVP droplet, cutting the tail at the mid-portion and then moved to the TALP-H droplet to be injected into a matured oocyte using a 9-μm injection micropipette (MIC-9-30, Origio, CooperSurgical Fertility Companies, Målov, Denmark).
In vitro embryo culture
Presumptive zygotes from all experimental groups were transferred into the porcine zygote medium (PZM; Yoshioka et al. 2002) droplets medium and incubated at 38.5°C, 5% CO2, 5% O2, 90% N2. A group of zygotes of each experimental treatment was removed from in vitro culture at 18–20 h after ICSI or artificial activation for pronuclear formation assessment. Cleavage and blastocyst rates were recorded on days 2 and 7, respectively. On day 5, the media was renewed. Day 7 blastocysts were fixed for immunofluorescence analysis.
Zygotes and blastocyst fixation, immunofluorescence and cell counting
Presumptive zygotes from each experimental group were centrifugated for 10 min at 1000g to move lipids to the periphery and enhance the visualisation of pronuclei. Then, they were fixed for 20 min in 4% formaldehyde (47,608) in DPBS, rinsed in DPBS with 0.4% w/v bovine serum albumin (BSA, A6003) and stored at 4°C. Embryos were treated with permeabilisation solution (DPBS containing 0.2% v/v Triton X-100 (9036-19-5) for 15 min and washed in blocking buffer (DPBS containing 0.1% v/v Tween 20 (P9416) and 0.4% w/v BSA). Then, they were incubated with SMARCA4 antibody (Brg-1 (G-7), 1:100, SC-17796, mouse monoclonal, Santa Cruz Biotechnology, Dallas, TX, USA; AB_626762) overnight at 4°C in blocking buffer. A negative control group without primary antibody was included for all assays. After washing, all embryos were incubated with a secondary antibody (Alexa Fluor1Plus 594, 1:1000, donkey anti-mouse, #A32744, Thermo Fisher Scientific) in a blocking buffer for 1 h in the dark. Blastocysts were fixed for 20 min in 4% formaldehyde (50980487, Electron Microscopy Sciences, Hatfield, PA USA) in DPBS, rinsed in DPBS with 0.4% w/v bovine serum albumin (BSA, A6003) and stored at 4°C in 1 mL tubes. Blastocysts were treated with permeabilisation solution (DPBS containing 0.2% v/v Triton X-100 (21,123)) for 15 min and washed in blocking buffer (DPBS containing 0.1% v/v Tween 20 (P9416) and 0.4% w/v BSA). A negative control group without primary antibody was included for all assays. Then, they were incubated with SOX2 antibody (Sox2 (D9B8N), 1:200, rabbit monoclonal, Cell Signalling, #23064) overnight at 4°C in a blocking buffer. The day after, they were incubated for 1 h at room temperature with CDX2 and OCT4 antibodies (Oct-3/4 Antibody (N-19), 1:100, goat polyclonal, sc-8628). After washing, embryos were incubated with secondary antibodies (Alexa Fluor® 555, 1:1000, donkey anti-goat, #21432, Alexa Fluor® 488, 1:1000, donkey anti-mouse IgG #A21202, Alexa Fluor® 488, 1:1000, donkey anti-rabbit, #A-31573, Thermo Fisher Scientific, Waltham, MA, USA) in blocking buffer for 1 h at room temperature in the dark. Finally, presumptive zygotes and blastocysts were mounted in Vectashield® containing 1.5 μg/mL DAPI (Vector Laboratories, Burlingame, CA, USA), and slides were scanned using an inverted confocal microscope (Olympus IX83 Spinning Disk Confocal System). The analysis of total cell number and quantification of SOX2 positive (SOX2+), OCT4 positive (OCT4+) and CDX2 positive (CDX2+) nuclei was performed manually with Fiji image processing software (Schneider et al. 2012). For image analysis, we use the depth of nuclei information within an embryo to facilitate accurate cell counting as described by (Gambini and Williams 2016). After immunofluorescence, presumptive zygotes were classified according to the presence of pronuclei: two PN (2-PN), one PN with the presence of a semi-condensed or condensed sperm (1-PN) or a semi-condensed or condensed sperm with no evidence of PN (no activation). Embryos that exhibited a different pattern were included in the ‘other’ category. Oocytes without evidence of sperm were excluded from the analysis. The intensity of SMARCA4 protein in PN was only measured in ICSI zygotes with the presence of 2 PN. A region of interest was drawn around each pronucleus and the average pixel intensity was determined with using Fiji software (version 2.15.0, 2024) (Schneider et al. 2012).
Statistical analysis
All statistical analyses were performed using GraphPad Prism software. Embryo preimplantation development rates were compared using Fisher’s exact test with a confidence interval (CI) of 95%. Comparisons of the two groups were performed using the Mann–Whitney test. Comparisons of three groups were performed using the Kruskal–Wallis test, with Dunn’s multiple comparisons tests. Differences were considered statistically significant at P < 0.05.
Results
Experiment 1: Pronuclear formation and recruitment of SMARCA4 assisting with PHEN after ICSI
To assess the impact of AOA following ICSI, pronuclear formation rates of parthenogenetically activated oocytes were compared among conventional ICSI, ICSI followed by PHEN treatment (ICSI-PHEN) and ICSI assisted with electrical activation (ICSI-e) (Table 1). ICSI-PHEN increased the activation rate, associated with an increment in the proportion of zygotes presenting 1-PN with condensed sperm. In contrast, the proportion of 2-PN remained stable among groups (P > 0.05). However, a significant difference was observed in the non-activated oocytes of the conventional ICSI group compared to the ICSI-PHEN and PART-PHEN groups. Embryos with 1-PN from both the ICSI and ICSI-PHEN groups were accompanied by condensed sperm (Fig. 1). Subsequently, we assessed SMARCA4 intensity levels among 2-PN embryos from ICSI and ICSI-PHEN experimental groups. No significant differences were found in SMARCA4 intensity between the pronuclei, and in both groups, most of the 2-PN embryos exhibited symmetric SMARCA4 intensity (Table 2, Fig. 2).
Group | # Presumptive zygotes | # 2-PN (%) | # 1-PN (%) | # No activation (%) | # Other (%) | |
---|---|---|---|---|---|---|
ICSI | 81 | 24 (29.62) | 28 (34.56)a | 15 (18.51) | 5 (6.17) | |
ICSI-e | 39 | 10 (25.64) | 18 (46.15)a,b | 8 (20.51) | 3 (7.69) | |
PART-e | 21 | 3 (14.28) | 14 (66.67)b | 0 (0) | 4 (19) | |
ICSI-PHEN | 40 | 12 (30) | 20 (50)a,b | 7 (17.50) | 1 (2.50) | |
PART-PHEN | 66 | 11 (16.67) | 45 (68.18)b | 10 (15.15) | 0 (0) |
PN, pronucleus; ICSI-e, ICSI assisted with electrical activation; PART-e, electrical parthenogenetic activation; PHEN, 1,10-phenanthroline; ICSI-PHEN, ICSI assisted with PHEN; PART-PHEN, haploid parthenogenetic activation using PHEN.
Different superscript letters indicate statistical significance (Fisher’s exact test, P < 0.05).
Representative DAPI staining of ICSI zygotes from the indicated groups, observed under the inverted confocal microscope (Olympus IX83 Spinning Disk Confocal System). (a) Zygote with 2-PN. (b) Zygote with 1-PN and sperm head, and two polar bodies. (c) Non-activated oocyte, presence of sperm head and metaphase II (MII).
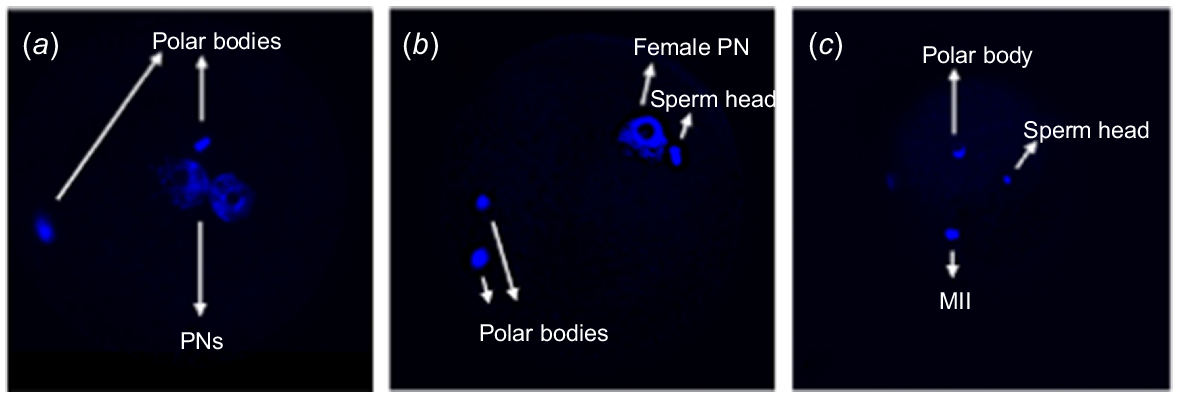
Groups | # Zygotes with 2-PN | # Zygotes with symmetric intensity (%) | # Zygotes with asymmetric intensity (%) | |
---|---|---|---|---|
ICSI | 11 | 10 (90) | 1 (9.1) | |
ICSI-PHEN | 16 | 12 (75) | 4 (25) |
PN, pronucleus; PHEN, 1,10-phenanthroline; ICSI-PHEN, ICSI assisted with PHEN.
Fisher’s exact test (P < 0.05).
Experiment 2: Pronuclear formation using AOA with PHEN before and after ICSI
As assisting oocyte activation after ICSI with PHEN did not result in a yield of 2-PN rates, we then evaluated the use of the zin chelator PHEN before performing ICSI, a strategy previously reported for mice (Kishigami et al. 2004). No significant differences were observed in the percentage of 2-PN, 1-PN and non-activated oocytes (Table 3).
Groups | # Presumptive zygotes | # 2-PN (%) | # 1-PN (%) | # No activation (%) | # Other (%) | |
---|---|---|---|---|---|---|
PHEN-ICSI | 69 | 15 (21.74) | 45 (65.22) | 5 (7.25) | 4 (5.79) | |
ICSI-PHEN | 67 | 25 (37.31) | 34 (50.74) | 4 (5.97) | 4 (5.97) |
PN, pronucleus; PHEN, 1,10-phenanthroline; ICSI-PHEN, ICSI assisted with PHEN.
Chi-squared test (P < 0.05).
Experiment 3: Effects of PHEN on embryo development, embryo quality and cell differentiation markers of ICSI and ICSI-PHEN blastocysts
Since no differences were observed in pronuclear formation rates with PHEN AOA, in Experiment 3, embryo developmental rates, number of cells in blastocysts and cell differentiation markers (CDX2, SOX2 and OCT4), including SOX2/CDX2 ratio, were assessed among ICSI, ICSI-PHEN and PART-PHEN experimental groups (Fig. 3). ICSI followed by AOA with PHEN did not alter blastocyst rates compared to conventional ICSI (Table 4). No significant differences were observed in total blastocyst cell number or the expression patterns of OCT4, CDX2, SOX2 and SOX2/CDX2 ratio between ICSI, ICSI-PHEN and PART-PHEN (Fig. 4).
Immunofluorescence staining with DAPI and pluripotency markers CDX2, OCT4 and SOX2 of porcine blastocysts from the indicated groups. ICSI, conventional injection; ICSI-PHEN, PHEN-assisted ICSI; PART-PHEN, haploid parthenogenetic activation with PHEN; PHEN, 1,10-phenanthroline.
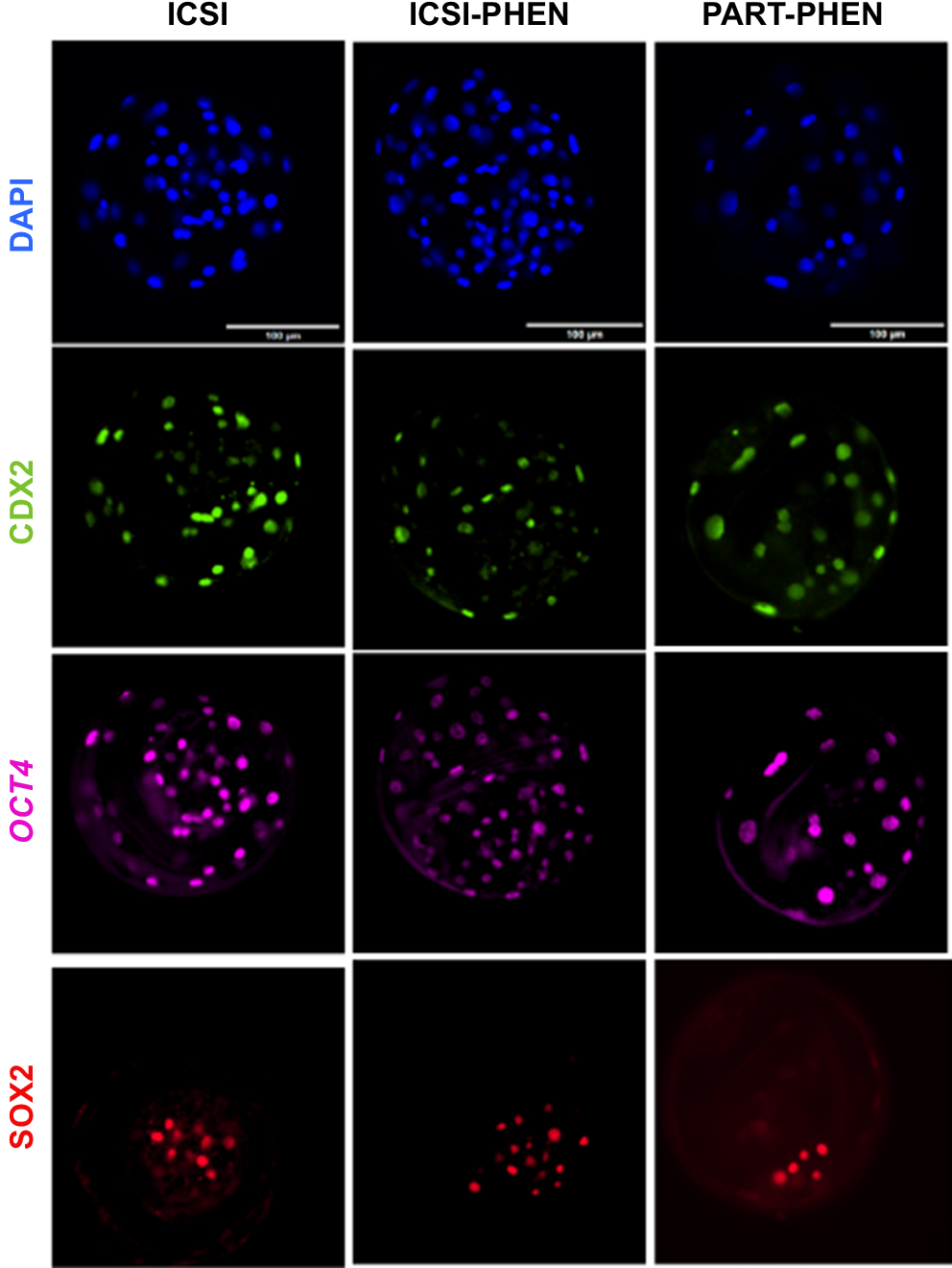
Groups | # Oocytes | # Cleavage (%) | # Blastocysts (%) | |
---|---|---|---|---|
ICSI | 101 | 88 (87.12) | 14 (13.86) | |
ICSI-PHEN | 136 | 93 (68.38) | 28 (20.58) | |
PART-PHEN | 104 | 73 (70.19) | 17 (16.34) |
PHEN, 1,10-phenanthroline; ICSI-PHEN, ICSI assisted with PHEN; PART-PHEN, PHEN haploid parthenogenetic activation.
Chi-squared exact test (P < 0.05).
Analysis of the total number of cells and the number of cells expressing pluripotency markers CDX2, OCT4 and SOX2 in porcine blastocysts from ICSI, ICSI-PHEN, and PART-PHEN. Blastocysts from three biological replicates were analysed; 10–15 embryos/group; P < 0.05, Mann–Whitney test and Kruskal–Wallis with Dunn’s multiple test. No statistical differences were found between the groups. PHEN, 1,10-phenanthroline; ICSI, conventional injection; ICSI-PHEN, ICSI assisted with PHEN; PART-PHEN, haploid parthenogenetic activation with PHEN.
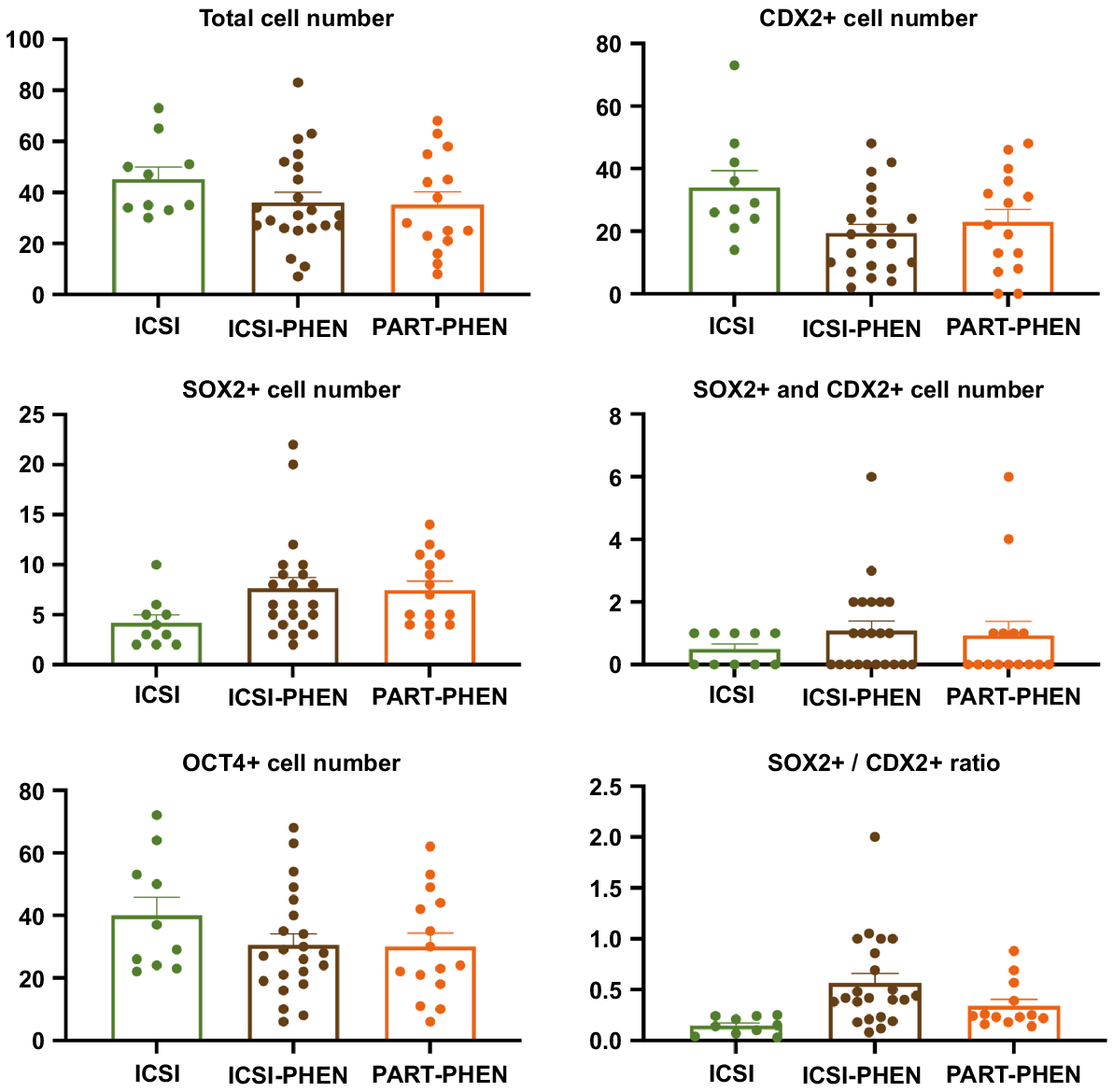
Discussion
In this study, we explored for the first time the use of the zinc chelator PHEN to enhance AOA for ICSI in pigs. While our research confirmed the efficacy of zinc chelation as an activator agent, its application immediately after or before ICSI did not lead to an increase in the formation of bi-pronuclear zygotes, neither did it enhance embryo development or quality following in vitro culture up to the blastocyst stage.
The rate of pronuclear development following ICSI in pigs rarely exceeds 50% (Nakai et al. 2016a), aligning with the outcomes observed in our control group undergoing ICSI without any additional treatments. While electrical activation and zinc chelation treatment yielded high rates of oocyte activation, evidenced by mono-pronuclear formation rates, these treatments did not increase the incidence of 2-PN formations of ICSI embryos. Although some previous reports have demonstrated that bi-pronuclear formation after ICSI can be improved by AOA (Lai et al. 2001; Lee et al. 2003; Tian et al. 2006; Nakai et al. 2016b; de Macedo et al. 2019), our results together with previous literature (Lee et al. 1998, 2003; Probst and Rath 2003) underscore the challenge in enhancing pig ICSI outcomes by the use of AOA and suggests that sperm decondensation and chromatin arrangement failures associated with male pronuclear formation in pigs after ICSI are not predominantly due to an inadequate ability of the sperm to induce activation. Notably, the improvement in pronuclear formation rates between assisted and non-assisted ICSI findings reported by de Macedo et al. (2019) is not translated into blastocyst development and they did not yield any porcine ICSI blastocysts without AOA. On the contrary, several research groups have reported the development of pig ICSI blastocysts without AOA as we observed in our study (Nakai et al. 2003; Yong et al. 2006; García-Roselló et al. 2009, 2010). Thus, AOA might play a crucial role in increasing ICSI performance, particularly under suboptimal culture conditions. In rodent models, pre-activation of oocytes has been demonstrated to increase the efficiency of ICSI, particularly in instances where spermatozoa fail to initiate activation (Kishigami et al. 2004; Torikai et al. 2023), and also resulted in beneficial increased resilience of the oocytes to the mechanical stresses imparted during the ICSI procedure. We did not observe differences in pronuclear formation rates in ICSI using preactivated oocytes with PHEN, indicating that, unlike in mouse and rat models, oocyte pre-activation might not show any apparent benefits for improving zygote formation in pigs. Although ICSI was performed within 1 h after zinc chelation in our study, pronuclear formation rates were reported to be reduced when injecting sperm after 3 h of electrical activation (Kim et al. 2002). Although SMARCA4 is dispensable for pronuclear formation in mice, its critical role as a key protein essential for the zygotic to embryo transition in mammals (Bultman et al. 2006) and female fertility (Abedini et al. 2023) is well-documented. In our investigation, the analysis of SMARCA4 intensity within the pronuclei of 2-PN zygotes derived from both standard ICSI and ICSI followed by PHEN treatment revealed predominantly symmetric SMARCA4 distribution between the pronuclei. Importantly, this observation indicates that zinc chelation treatment does not impact on the pronuclear recruitment of SMARCA4. Nevertheless, a small subset of embryos displayed notable asymmetry in SMARCA4 intensity across the pronuclei, a phenomenon observed in previous porcine ICSI studies (Gambini et al. 2020). This observed variability in SMARCA4 intensity may underlie the compromised developmental progression to the blastocyst stage observed in some porcine ICSI-derived zygotes.
Assisted oocyte activation by zinc chelation in our study did not yield an increase in preimplantation embryo development, further confirming that AOA with zinc chelation alone does not improve ICSI outcomes in pigs. Interestingly, it was reported that ICSI in pigs, compared to IVF embryos, has delayed cleavage and compromised development (Nakai et al. 2014). Thus, evaluation of embryo quality results is fundamental to understanding if the use of AOA leads to an improvement of quality. We observed no differences in the total number of cells or the number of cells expressing OCT4, CDX2 and SOX2 among the groups. Both ICSI and ICSI-PHEN groups showed the expected localisation pattern previously reported for in vivo-derived (Kirchhof et al. 2000), parthenogenic (Lee et al. 2021; Cabeza et al. 2022) and IVF blastocysts (Liu et al. 2015) in pigs. Our results regarding total cell number and ICM cells are similar to those previously reported for assisted ICSI embryos obtained by AOA with ionomycin and TPEN (de Macedo et al. 2019). Similarly to what we observed with SMARCA4 expression levels, AOA by PHEN treatment does not impact the normal expression of these markers. In contrast to diploid blastocysts, which exhibit higher developmental rates when using PHEN compared to our haploid embryos (Cabeza et al. 2022), it is noteworthy that the developmental blastocyst rate of parthenogenetic haploid porcine embryos is similar to those produced through ICSI in our study. Consequently, blastocysts resulting from AOA ICSI treatments might essentially be parthenogenetic embryos. This represents a significant drawback of integrating AOA into ICSI programs.
In conclusion, our findings suggest that the addition of the zinc chelator PHEN after ICSI did not enhance the rates of 2-PN formation. Furthermore, the absence of notable improvement in overall ICSI efficiency implies that the use of PHEN zinc chelation does not compromise embryo viability or quality. These results hold significance in refining and advancing the application of AOA techniques. Particularly, they offer insights into addressing fertility issues and propelling advancements in human and animal reproductive medicine.
Declaration of funding
This work was supported by Secretaría de Ciencia y Técnica (UBACyT) [0020170100669BA], Proyectos de Investigación Científica y Tecnológica (PICT) [2016-4347].
Author contributions
Conceptualisation: O.B., D.F.S. and A.G.; methodology: O.B., J.P.C.; formal analysis: O.B. and A.G.; investigation: O.B., J.P.C. and A. G.; writing – original draft: O.B. and A.G.; writing – review and editing: O.B. and A.G.; supervision: O.B., D.F.S., R.F.M. and A.G.; funding acquisition: O.B., D.F.S., R.F.M. and A. G. All authors have read and agreed to the published version of the manuscript.
Acknowledgements
The authors would like to thank New Organs Biotech for providing resources and facilitating our research, as well as laboratory technician Carla Ciampi and the Minguillón Slaughterhouse for their contributions.
References
Abedini A, Landry DA, Macaulay AD, Vaishnav H, Parbhakar A, Ibrahim D, Salehi R, Maranda V, Macdonald E, Vanderhyden BC (2023) SWI/SNF chromatin remodeling subunit Smarca4/BRG1 is essential for female fertility. Biology of Reproduction 108, 279-291.
| Crossref | Google Scholar |
Arroyo-Salvo C, Cogollo Villarreal MY, Clérico G, Flores Bragulat AP, Niño Vargas A, Castañeira C, Briski O, Alonso C, Plaza J, Zeledon JM, Losinno L, Miragaya M, Sansinena M, Martinez SP, Gambini A (2024) The ability of donkey sperm to induce oocyte activation and mule embryo development after ICSI. Theriogenology 218, 200-207.
| Crossref | Google Scholar | PubMed |
Briski O, Salamone DF (2022) Past, present and future of ICSI in livestock species. Animal Reproduction Science 246, 106925.
| Crossref | Google Scholar |
Briski O, La Motta GE, Ratner LD, Allegroni FA, Pillado S, Álvarez G, Gutierrez B, Tarragona L, Zaccagnini A, Acerbo M, Ciampi C, Fernández-Martin R, Salamone DF (2024) Comparison of ICSI, IVF, and in vivo derived embryos to produce CRISPR-Cas9 gene-edited pigs for xenotransplantation. Theriogenology 220, 43-55.
| Crossref | Google Scholar | PubMed |
Buemo CP, Gambini A, Moro LN, Hiriart MI, Fernández-Martín R, Collas P, Salamone DF (2016) Embryo aggregation in pig improves cloning efficiency and embryo quality. PLoS ONE 11, e0146390.
| Crossref | Google Scholar |
Bultman SJ, Gebuhr TC, Pan H, Svoboda P, Schultz RM, Magnuson T (2006) Maternal BRG1 regulates zygotic genome activation in the mouse. Genes & Development 20, 1744-1754.
| Crossref | Google Scholar | PubMed |
Cabeza JP, Cámera J, Briski O, Felipe MY, Salamone DF, Gambini A (2022) Preimplantation developmental competence of bovine and porcine oocytes activated by zinc chelation. Animals 12, 3560.
| Crossref | Google Scholar |
de Macedo MP, Glanzner WG, Rissi VB, Gutierrez K, Currin L, Baldassarre H, Bordignon V (2019) A fast and reliable protocol for activation of porcine oocytes. Theriogenology 123, 22-29.
| Crossref | Google Scholar | PubMed |
Ducibella T, Fissore R (2008) The roles of Ca2+, downstream protein kinases, and oscillatory signaling in regulating fertilization and the activation of development. Developmental Biology 315, 257-279.
| Crossref | Google Scholar | PubMed |
Eckersley-Maslin MA, Alda-Catalinas C, Reik W (2018) Dynamics of the epigenetic landscape during the maternal-to-zygotic transition. Nature Reviews Molecular Cell Biology 19, 436-450.
| Crossref | Google Scholar | PubMed |
Fesahat F, Henkel R, Agarwal A (2020) Globozoospermia syndrome: an update. Andrologia 52, e13459.
| Crossref | Google Scholar |
Gambini A, Williams CJ (2016) LUTs of blastocyst nuclei for quantification. Molecular Reproduction and Development 83, 575.
| Crossref | Google Scholar |
Gambini A, Duque Rodríguez M, Rodríguez MB, Briski O, Flores Bragulat AP, Demergassi N, Losinno L, Salamone DF (2020) Horse ooplasm supports in vitro preimplantation development of zebra ICSI and SCNT embryos without compromising YAP1 and SOX2 expression pattern. PLoS ONE 15, e0238948.
| Crossref | Google Scholar |
García-Roselló E, García-Mengual E, Coy P, Alfonso J, Silvestre MA (2009) Intracytoplasmic sperm injection in livestock species: an update. Reproduction in Domestic Animals 44, 143-151.
| Crossref | Google Scholar | PubMed |
García-Vázquez FA, Ruiz S, Matás C, Izquierdo-Rico MJ, Grullón LA, De Ondiz A, Vieira L, Avilés-López K, Gutiérrez-Adán A, Gadea J (2010) Production of transgenic piglets using ICSI-sperm-mediated gene transfer in combination with recombinase RecA. Reproduction 140, 259-272.
| Crossref | Google Scholar | PubMed |
Hirose N, Kikuchi Y, Kageyama A, Sugita H, Sakurai M, Kawata Y, Terakawa J, Wakayama T, Ito J, Kashiwazaki N (2023) Successful production of offspring derived from phospholipase C zeta-deficient sperm by additional artificial activation. Life 13, 980.
| Crossref | Google Scholar |
Kashir J, Ganesh D, Jones C, Coward K (2022) Oocyte activation deficiency and assisted oocyte activation: mechanisms, obstacles and prospects for clinical application. Human Reproduction Open 2022, hoac003.
| Crossref | Google Scholar |
Kikuchi K, Nakai M, Shimada A, Kashiwazaki N (2006) Production of viable porcine embryos by in vitro fertilization (IVF) and intracytoplasmic sperm injection (ICSI). Journal of Mammalian Ova Research 23, 96-106.
| Crossref | Google Scholar |
Kim B-K, Lee Y-J, Cui X-S, Kim N-H (2002) Chromatin and microtubule organisation in maturing and pre-activated porcine oocytes following intracytoplasmic sperm injection. Zygote 10, 123-129.
| Crossref | Google Scholar | PubMed |
Kim AM, Bernhardt ML, Kong BY, Ahn RW, Vogt S, Woodruff TK, O’Halloran TV (2011) Zinc sparks are triggered by fertilization and facilitate cell cycle resumption in mammalian eggs. ACS Chemical Biology 6, 716-723.
| Crossref | Google Scholar | PubMed |
Kirchhof N, Carnwath JW, Lemme E, Anastassiadis K, Schöler H, Niemann H (2000) Expression pattern of Oct-4 in preimplantation embryos of different species. Biology of Reproduction 63, 1698-1705.
| Crossref | Google Scholar | PubMed |
Kishigami S, Wakayama S, Van Thuan N, Wakayama T (2004) Similar time restriction for intracytoplasmic sperm injection and round spermatid injection into activated oocytes for efficient offspring production. Biology of Reproduction 70, 1863-1869.
| Crossref | Google Scholar | PubMed |
Kuijk EW, Du Puy L, Van Tol HTA, Oei CHY, Haagsman HP, Colenbrander B, Roelen BAJ (2008) Differences in early lineage segregation between mammals. Developmental Dynamics 237, 918-927.
| Crossref | Google Scholar | PubMed |
Kurome M, Ueda H, Tomii R, Naruse K, Nagashima H (2006) Production of transgenic-clone pigs by the combination of ICSI-mediated gene transfer with somatic cell nuclear transfer. Transgenic Research 15, 229-240.
| Crossref | Google Scholar | PubMed |
Lai L, Sun Q, Wu G, Murphy CN, Kühholzer B, Park K-W, Bonk AJ, Day BN, Prather RS (2001) Development of porcine embryos and offspring after intracytoplasmic sperm injection with liposome transfected or non-transfected sperm into in vitro matured oocytes. Zygote 9, 339-346.
| Crossref | Google Scholar | PubMed |
Lee JW, Kim N-H, Lee HT, Chung KS (1998) Microtubule and chromatin organization during the first cell-cycle following intracytoplasmic injection of round spermatid into porcine oocytes. Molecular Reproduction and Development 50, 221-228.
| Crossref | Google Scholar | PubMed |
Lee J-W, Tian XC, Yang X (2003) Failure of male pronucleus formation is the major cause of lack of fertilization and embryo development in pig oocytes subjected to intracytoplasmic sperm injection. Biology of Reproduction 68, 1341-1347.
| Crossref | Google Scholar | PubMed |
Lee M, Choi K-H, Oh J-N, Kim S-H, Lee D-K, Choe GC, Jeong J, Lee C-K (2021) SOX2 plays a crucial role in cell proliferation and lineage segregation during porcine pre-implantation embryo development. Cell Proliferation 54, e13097.
| Crossref | Google Scholar |
Lim KT, Gupta MK, Lee SH, Jung YH, Han DW, Lee HT (2013) Possible involvement of Wnt/β-catenin signaling pathway in hatching and trophectoderm differentiation of pig blastocysts. Theriogenology 79, 284-290.e2.
| Crossref | Google Scholar | PubMed |
Liu S, Bou G, Sun R, Guo S, Xue B, Wei R, Cooney AJ, Liu Z (2015) Sox2 is the faithful marker for pluripotency in pig: evidence from embryonic studies. Developmental Dynamics 244, 619-627.
| Crossref | Google Scholar | PubMed |
Ma H, Marti-Gutierrez N, Park S-W, Wu J, Lee Y, Suzuki K, Koski A, Ji D, Hayama T, Ahmed R, Darby H, Van Dyken C, Li Y, Kang E, Park A-R, Kim D, Kim S-T, Gong J, Gu Y, Xu X, Battaglia D, Krieg SA, Lee DM, Wu DH, Wolf DP, Heitner SB, Belmonte JCI, Amato P, Kim J-S, Kaul S, Mitalipov S (2017) Correction of a pathogenic gene mutation in human embryos. Nature 548, 413-419.
| Crossref | Google Scholar | PubMed |
Matsunari H, Kobayashi T, Watanabe M, Umeyama K, Nakano K, Kanai T, Matsuda T, Nagaya M, Hara M, Nakauchi H, Nagashima H (2014) Transgenic pigs with pancreas-specific expression of green fluorescent protein. Journal of Reproduction and Development 60, 230-237.
| Crossref | Google Scholar | PubMed |
Nakai M, Kashiwazaski N, Takizawa A, Hayashi Y, Nakatsukasa E, Fuchimoto D-I, Noguchi J, Kaneko H, Shino M, Kikuchi K (2003) Viable piglets generated from porcine oocytes matured in vitro and fertilized by intracytoplasmic sperm head injection. Biology of Reproduction 68, 1003-1008.
| Crossref | Google Scholar | PubMed |
Nakai M, Kashiwazaki N, Takizawa A, Maedomari N, Ozawa M, Noguchi J, Kaneko H, Shino M, Kikuchi K (2006) Morphologic changes in boar sperm nuclei with reduced disulfide bonds in electrostimulated porcine oocytes. Reproduction 131, 603-611.
| Crossref | Google Scholar | PubMed |
Nakai M, Ozawa M, Maedomari N, Noguchi J, Kaneko H, Ito J, Onishi A, Kashiwazaki N, Kikuchi K (2014) Delay in cleavage of porcine embryos after intracytoplasmic sperm injection (ICSI) shows poorer embryonic development. Journal of Reproduction and Development 60, 256-259.
| Crossref | Google Scholar | PubMed |
Nakai M, Ito J, Suzuki S-I, Fuchimoto D-I, Sembon S, Suzuki M, Noguchi J, Kaneko H, Onishi A, Kashiwazaki N, Kikuchi K (2016a) Lack of calcium oscillation causes failure of oocyte activation after intracytoplasmic sperm injection in pigs. Journal of Reproduction and Development 62, 615-621.
| Crossref | Google Scholar | PubMed |
Nakai M, Ito J, Kashiwazaki N, Men NT, Tanihara F, Noguchi J, Kaneko H, Onishi A, Kikuchi K (2016b) Treatment with protein kinase C activator is effective for improvement of male pronucleus formation and further embryonic development of sperm-injected oocytes in pigs. Theriogenology 85, 703-708.
| Crossref | Google Scholar | PubMed |
Nakai M, Suzuki S-I, Fuchimoto D-I, Sembon S, Kikuchi K (2024) Oocyte activation with phospholipase Cζ mRNA induces repetitive intracellular Ca2+ rises and improves the quality of pig embryos after intracytoplasmic sperm injection. Journal of Reproduction and Development 70, 229-237.
| Crossref | Google Scholar | PubMed |
Navarro-Serna S, Hachem A, Canha-Gouveia A, Hanbashi A, Garrappa G, Lopes JS, et al. (2021) Generation of nonmosaic, two-pore channel 2 Biallelic knockout pigs in one generation by CRISPR-Cas9 microinjection before oocyte insemination. The CRISPR Journal 4, 132-146.
| Crossref | Google Scholar | PubMed |
Nguyen HT, Dang-Nguyen TQ, Somfai T, Men NT, Beck-Woerner B, Viet Linh N, Xuan Nguyen B, Noguchi J, Kaneko H, Kikuchi K (2021) Excess polyspermy reduces the ability of porcine oocytes to promote male pronuclear formation after in vitro fertilization. Animal Science Journal 92, e13650.
| Crossref | Google Scholar |
Probst S, Rath D (2003) Production of piglets using intracytoplasmic sperm injection (ICSI) with flowcytometrically sorted boar semen and artificially activated oocytes. Theriogenology 59(3-4), 961-973.
| Crossref | Google Scholar | PubMed |
Salamone DF, Canel NG, Rodríguez MB (2017) Intracytoplasmic sperm injection in domestic and wild mammals. Reproduction 154, F111-F124.
| Crossref | Google Scholar | PubMed |
Schneider CA, Rasband WS, Eliceiri KW (2012) NIH Image to ImageJ: 25 years of image analysis. Nature Methods 9, 671-675.
| Crossref | Google Scholar | PubMed |
Sun F, Tang F, Yan AY, Fang HY, Sheng HZ (2007) Expression of SRG3, a chromatin-remodelling factor, in the mouse oocyte and early preimplantation embryos. Zygote 15, 129-138.
| Crossref | Google Scholar | PubMed |
Sun J-T, Liu J-H, Jiang X-Q, Luo X, Yuan J-D, Zhang Q, Qi X-Y, Lee S, Liu Z-H, Jin J-X (2022) Tannin reduces the incidence of polyspermic penetration in porcine oocytes. Antioxidants 11, 2027.
| Crossref | Google Scholar |
Suzuki T, Yoshida N, Suzuki E, Okuda E, Perry ACF (2010) Full-term mouse development by abolishing Zn2+-dependent metaphase II arrest without Ca2+ release. Development 137, 2659-2669.
| Crossref | Google Scholar | PubMed |
Taylor SL, Yoon SY, Morshedi MS, Lacey DR, Jellerette T, Fissore RA, Oehninger S (2010) Complete globozoospermia associated with PLCζ deficiency treated with calcium ionophore and ICSI results in pregnancy. Reproductive BioMedicine Online 20, 559-564.
| Crossref | Google Scholar | PubMed |
Tian J-H, Wu Z-H, Liu L, Cai Y, Zeng S-M, Zhu S-E, Liu G-S, Li Y, Wu C-X (2006) Effects of oocyte activation and sperm preparation on the development of porcine embryos derived from in vitro-matured oocytes and intracytoplasmic sperm injection. Theriogenology 66, 439-448.
| Crossref | Google Scholar | PubMed |
Torikai K, Shimizu K, Nagatomo H, Kasai M, Kato-Itoh M, Kamada Y, Shibasaki I, Jeon H, Kikuchi R, Wakayama S, Suchy F, Nakauchi H, Wakayama T, Mizutani E (2023) Removal of sperm tail using trypsin and pre-activation of oocyte facilitates intracytoplasmic sperm injection in mice and rats. Journal of Reproduction and Development 69, 48-52.
| Crossref | Google Scholar | PubMed |
Uh K, Ryu J, Zhang L, Errington J, Machaty Z, Lee K (2019) Development of novel oocyte activation approaches using Zn2+ chelators in pigs. Theriogenology 125, 259-267.
| Crossref | Google Scholar | PubMed |
Uh K, Hay A, Chen P, Reese E, Lee K (2022) Design of novel oocyte activation methods: the role of zinc. Biology of Reproduction 106, 264-273.
| Crossref | Google Scholar | PubMed |
Umeyama K, Saito H, Kurome M, Matsunari H, Watanabe M, Nakauchi H, Nagashima H (2012) Characterization of the ICSI-mediated gene transfer method in the production of transgenic pigs. Molecular Reproduction and Development 79, 218-228.
| Crossref | Google Scholar | PubMed |
Vackova I, Novakova Z, Krylov V, Okada K, Kott T, Fulka H, Motlik J (2011) Analysis of marker expression in porcine cell lines derived from blastocysts produced in vitro and in vivo. Journal of Reproduction and Development 57, 594-603.
| Crossref | Google Scholar | PubMed |
Watanabe M, Kurome M, Matsunari H, Nakano K, Umeyema K, Shiota A, Nakauchi H, Nagashima H (2012) The creation of transgenic pigs expressing human proteins using BAC-derived, full-length genes and intracytoplasmic sperm injection-mediated gene transfer. Transgenic Research 21, 605-618.
| Crossref | Google Scholar | PubMed |
Yong HY, Hao Y, Lai L, Li R, Murphy CN, Rieke A, Wax D, Samuel M, Prather RS (2006) Production of a transgenic piglet by a sperm injection technique in which no chemical or physical treatments were used for oocytes or sperm. Molecular Reproduction and Development 73, 595-599.
| Crossref | Google Scholar | PubMed |
Yoshioka K, Suzuki C, Tanaka A, Anas IM-K, Iwamura S (2002) Birth of piglets derived from porcine zygotes cultured in a chemically defined medium. Biology of Reproduction 66, 112-119.
| Crossref | Google Scholar | PubMed |