Differential effect of melatonin on ram spermatozoa depending on the allelic variant of the RsaI polymorphism of the MTR1A gene, incubation medium and season
Victoria Peña-Delgado





A
Abstract
The RsaI polymorphism of the melatonin receptor MTNR1A gene affects seasonal reproduction in sheep, but its effect on ram spermatozoa and their response to melatonin is unknown.
This study aims to evaluate whether RsaI polymorphism of the MTNR1A gene influences the response of ram spermatozoa to in vitro added melatonin.
Spermatozoa from rams carrying different RsaI allelic variants were incubated with melatonin in a TALP medium or a capacitation-triggering medium during the reproductive and non-reproductive seasons. After incubation, sperm motility, membrane integrity, mitochondria activity, oxidative damage, apoptotic markers and capacitation status were assessed.
In the reproductive season, the T/T genotype was related to some adverse effects of melatonin when spermatozoa were incubated in TALP medium, whereas the C/C genotype was linked with adverse effects when the hormone was added in a capacitation-triggering medium. The decapacitating effect of melatonin on spermatozoa was also different depending on genotype.
The melatonin effect on spermatozoa from rams carrying different RsaI genotypes differed depending on the season and the medium.
The knowledge of the RsaI allelic variant of the MTNR1A gene of rams could be helpful when carrying out in vitro reproductive techniques in the ovine species.
Keywords: Capacitation, incubation, melatonin, MTNR1A polymorphism, ram sperm, receptor, RsaI, sheep.
Introduction
Breeds of sheep located in temperate regions are highly seasonal in terms of reproductive capability, which is regulated by photoperiod and melatonin secretion (Rosa and Bryant 2003; Chemineau et al. 2008). Melatonin, which is also well known as an antioxidant molecule, is a hormone that can exert its actions by two different mechanisms: directly, by crossing the plasma membrane, or by binding to its specific receptors (Zhao et al. 2019). Two high-affinity melatonin receptors, called MT1 and MT2, both belonging to the G protein-coupled receptors family, have been identified in mammals (Dubocovich and Markowska 2005). The MT1 receptor seems to be the one that plays a key role in controlling photoperiod-induced seasonality, at least in hamsters (Weaver et al. 1996) and appears to be related to sheep seasonality (Notter 2008). The MT1 gene, named MTNR1A, has two exons divided by a long intron (Messer et al. 1997). In exon II, there are several polymorphic sites, among which the RsaI and MnlI polymorphisms are of particular interest because they affect the seasonal reproductive functions of different sheep breeds (Pelletier et al. 2000; Carcangiu et al. 2009; Mateescu et al. 2009).
In Rasa Aragonesa breed, which is a local Spanish breed with a short seasonal anoestrous (Forcada et al. 1992), the T allele of Rsal polymorphism has been associated with a more significant percentage of oestrous cyclic ewes (Martínez-Royo et al. 2012) during the non-reproductive season, with a shorter anoestrous period and a higher number of complete cycles per year (Calvo et al. 2018). Despite all these experiments performed in ewes, few studies examine the impact of the RsaI polymorphism on rams since they show a less marked seasonality (Rosa and Bryant 2003; Avdi et al. 2004). Nonetheless, some changes that affect the reproductive performance of rams have been described. Rasa Aragonesa ram lambs born in autumn that carry the TT genotype have a greater ability to reproduce in their first spring, and adult TT rams exhibit a more intense reproductive behaviour, evaluated by a sexual behaviour test carried out in spring (Abecia et al. 2020). However, this genotype was not associated with the social hierarchy in the ram flock (Abecia et al. 2022).
The melatonin receptors MT1 and MT2 are present in the sperm plasma membrane of many species, including ovine (Casao et al. 2012; González-Arto et al. 2016b). Moreover, the activities of these receptors have been reported in human spermatozoa by 2-[125I]-iodomelatonin binding (van Vuuren et al. 1992) and by using antagonists both in hamster, ram and human spermatozoa (Fujinoki 2008; Espino et al. 2011; Gonzalez-Arto et al. 2016a). This, together with the fact that melatonin is present in ram seminal plasma (Casao et al. 2010a), suggests that some of the aforementioned differences observed in animals carrying different genotypes for the MT1 receptor could be due to changes occurring at the cellular level, more specifically, in their reproductive cells. However, the influence of the RsaI polymorphism in the ram sperm response to melatonin remains unknown to date. Thus, the aim of this work was to evaluate whether RsaI polymorphism of the MTNR1A gene influences the response of ram spermatozoa to in vitro added melatonin. To achieve this aim, spermatozoa from rams carrying different RsaI allelic variants were incubated with melatonin in a TALP medium (Experiment 1) or in a TALP medium supplemented with a cocktail of substances that induce capacitation in the ovine species (Experiment 2) during the reproductive and non-reproductive seasons.
Materials and methods
Unless otherwise stated, all reagents were purchased from Sigma-Aldrich (St. Louis, MO, USA).
Animals, semen collection and processing
Twelve adult Rasa Aragonesa rams were genotyped for the RsaI polymorphism of the MTNR1A gene as follows: genotype C/C (n = 4), C/T (n = 4) and T/T (n = 4). The genotyping was performed as previously described by Abecia et al. (2020). All rams were housed at the Experimental Farm of the University of Zaragoza (Zaragoza, Spain). All experimental procedures were approved by the Ethics Commission for Animal Experimentation of the University of Zaragoza (Project authorization number: PI47/21), under the Spanish Policy for Experimental Animal Protection (RD53/2013), which meets the Directive 2010/63/EU of the European Parliament on the protection of animals used for scientific purposes.
For this study, during the reproductive and non-reproductive seasons, second ejaculates from each male were collected every 2 days individually using an artificial vagina (Ollero et al. 1996), and samples were maintained at 37°C until analysis. Sperm samples collected from rams carrying C/C, C/T and T/T genotypes were named CC, CT and TT samples for simplicity.
A dextran swim-up procedure (García-López et al. 1996) was used to obtain a seminal plasma-free sperm population from each ejaculate. This procedure was performed in a modified human tubular fluid medium (mHTF) containing 200 mM sucrose, 50 mM NaCl, 18.6 mM sodium lactate, 21 mM HEPES, 10 mM KCl, 2.8 mM glucose, 0.4 mM MgSO4, 0.3 mM sodium pyruvate, 0.3 mM K2HPO4. Briefly, 0.5 mL of semen was placed at the bottom of a 15 mm diameter round tube, and 0.5 mL of dextran solution (30 mg of dextran/mL of mHTF medium) was carefully deposited on top of it. On top of the dextran layer, 1.5 mL of mHTF containing 5 mg/mL BSA (BSA-mHTF) was added very slowly without mixing the media. The tube was incubated at 37°C in a vertical position for 15 min, and 750 μL of the upper phase was removed, and another 750 μL of MS-BSA was added. The process was repeated three more consecutive times, collecting four supernatants. The first one was discarded due to seminal plasma contamination, and the mixture of the remaining three was used for sperm capacitation. After the swim-up procedure, sperm concentration was calculated using a Neubauer chamber (Marienfeld, Lauda-Königshofen, Germany).
Sperm incubation
Swim-up-selected spermatozoa, in aliquots of 1.6 × 108 cells/mL, were incubated for 3 h at 39°C in a humidified incubator with 5% CO2 in the air. To analyse the effect of melatonin on sperm cells from males carrying different RsaI genotypes, the hormone was solubilised in dimethyl sulphoxide (DMSO) and phosphate-buffered saline (PBS) and added to the sperm samples at the beginning of the incubation to yield a final concentration of 1 μM on the basis of our previous research (Casao et al. 2010b; Gimeno-Martos et al. 2019). The final concentration of DMSO in melatonin samples was 0.1%, and the same DMSO concentration was included in control samples. Incubations in Experiment 1 were performed in a complete TALP medium (Parrish et al. 1988) composed of 100 mM NaCl, 3.1 mM KCl, 25 mM NaHCO3, 0.3 mM NaH2PO4, 21.6 mM Na lactate, 3 mM CaCl2, 0.4 mM MgCl2, 10 mM HEPES, 1 mM Na pyruvate, 5 mM glucose and 5 mg/mL bovine serum albumin (BSA), pH 7.2. However, this medium is not able to fully promote capacitation in ram sperm (Colas et al. 2008). Thus, to study the effect of melatonin on capacitating conditions, in Experiment 2, spermatozoa were incubated in a TALP medium supplemented with a cocktail of c-AMP elevating agents (1 mM dibutyryl (db)-cAMP, 1 mM caffeine, 1 mM theophylline, 0.2 mM okadaic acid and 2.5 mM methyl-β-cyclodextrin). This medium, already proven for capacitating ram spermatozoa (Grasa et al. 2006; Colas et al. 2008), was named CK (Cocktail medium). Also, 5 μL of D-penicillamine 1 mM was added to each sample in Experiment 2 to prevent sperm agglutination (Leahy et al. 2016).
After 3 h of incubation, sperm motility, membrane integrity, mitochondria activity, intracellular levels of reactive oxygen species (ROS) and superoxide (O2˙−) and apoptotic markers (phosphatidylserine inversion and caspase activation), as well as sperm capacitation status were assessed.
Sperm motility analysis
Total and progressive motility were evaluated using the OpenCASA motility module, a free and open-source software developed by our group (Alquézar-Baeta et al. 2019). For this assessment, a dilution of each sample (3 × 107 cells/mL) was made in a medium with the following composition: 0.25 M sucrose, 100 mM EGTA, 0.5 mM sodium phosphate, 50 mM glucose, 100 mM HEPES and 20 mM KOH. A drop of 8 μL of each diluted sample was placed between a pre-warmed slide and a coverslip and maintained at 37°C in a heated slide holder during analysis. The spermatozoa were recorded with a video camera (Basler acA1920; Basler Vision Components, Ahrensburg, Germany) mounted on a microscope (Nikon Eclipse 50i, Nikon Instruments Int, Tokyo, Japan) equipped with a 10× negative-phase contrast lens. Recorded videos were evaluated with the following settings: 60 frames per second, 120 frames, 800 × 600 pixels image resolution, 10 μm2 minimum cell size, 100 μm2 maximum cell size, progressive motility: STR (straightness coefficient) >80% and VAP (mean velocity) >90 μm/s, 10 μm/s minimum VCL (curvilinear velocity), 100 μm/s VCL lower threshold, 200 μm/s VCL upper threshold, 30 frames minimum track length and 20 μm maximum displacement between frames. Since the OpenCASA motility module provides the values of progressive motility calculated on the number of total cells and not on the total motile cells, the percentage of progressive spermatozoa over total motile spermatozoa was calculated.
Flow cytometry analysis
All flow cytometry measurements were performed using a Beckman Coulter FC 500 flow cytometer (Beckman Coulter Inc., Fullerton, CA, USA) equipped with CXP software, two lasers of excitation (argon-ion laser, 488 nm; and red solid-state laser, 633 nm) and five filters of absorbance (FL1-525, FL2-575, w; ±5 nm each bandpass filter). A flow rate stabilised at 200–300 cells/s was used, and a minimum of 20,000 events were recorded in all experiments. The sperm population was gated for further analysis based on its specific forward (FS) and side scatter (SS) properties and other non-sperm events were excluded.
A modification of the procedure described by Harrison and Vickers (1990) was followed to determine cell membrane integrity (viability). Samples (300 μL; 5.3 × 106 cells/mL) were stained with 3 μL of 10 μM carboxyfluorescein diacetate (CFDA) and 3 μL of 7.3 μM propidium iodide (PI) and fixed with 3 μL formaldehyde (0.5% (v/v) in water). After 15 min of incubation at 37°C in darkness, samples were analysed by flow cytometry. The monitored parameters were FS log, SS log, FL1 log (CFDA) and FL4 log (PI). The percentage of viable spermatozoa (CFDA+/PI−) was evaluated.
MitoTracker® Deep Red FM is a probe that passively diffuses across the plasma membrane and accumulates in active mitochondria (Hallap et al. 2005). This probe can be combined with Yo-Pro-1 (Thermo Fisher Scientific, Waltham, MA, United States) to exclude the non-viable population of spermatozoa from the analysis. Sperm samples (300 μL; 5.3 × 106 cells/mL) were stained with 3 μL of 10 μM MitoTracker® Deep Red FM and 3 μL of 1.5 mM Yo-Pro-1, and fixed with 3 μL formaldehyde (0.5% (v/v) in water). After incubation (10 min, 37°C in darkness), samples were analysed by flow cytometry, and the monitored parameters were FS log, SS log, FL1 log (Yo-Pro-1) and FL4 log (MitoTracker® Deep Red FM). The percentage of viable spermatozoa with active mitochondria (Mitotracker® Deep Red FM +/Yo-Pro-1-) was assessed.
ROS levels were assessed by using 2′,7′-Dichlorodihydrofluorescein diacetate (H2DCFDA), which is freely permeable across cell membranes (Bass et al. 1983) and converted into non-permeable and non-fluorescent 2′,7′-dichlorodihydrofluorescein (H2DCF) by intracellular esterases. This molecule is then oxidised by H2O2 to dichlorofluorescein (DCF), which emits fluorescence at 530 nm in response to 488 nm excitation (Carter et al. 1994). This probe was combined with PI to exclude the non-viable population from the analysis. For the analysis, sperm samples (300 μL; 5.3 × 106 cells/mL) were stained with 5 μL of 10 μM H2DCFDA and 3 μL of 1.5 mM PI and fixed with 3 μL formaldehyde (0.5% (v/v) in water). After incubation (15 min, 37°C in darkness), samples were analysed by flow cytometry, and the monitored parameters were FS log, SS log, FL1 log (H2DCFDA) and FL4 log (PI). The percentage of viable spermatozoa with low levels of ROS (H2DCFDA-/PI-) was evaluated.
To detect intracellular superoxide (O2˙−) levels in viable spermatozoa, dihydroethidium (DHE, hydroethidine) was used. This probe is permeable to cells, and it is oxidised by superoxide (O2˙−) to the red fluorescent compound ethidium (E) (Guthrie and Welch 2006). DHE was combined with Yo-Pro-1 to exclude the non-viable population from the analysis. For the assessment, sperm samples (300 μL; 5.3 × 106 cells/mL) were loaded with 1 μL of 4 μM DHE and 1 μL of 40 nM Yo-Pro-1 fluorochromes and incubated at 37°C for 40 min in darkness. After incubation, samples were analysed by flow cytometry, and the monitored parameters were FS log, SS log, FL1 log (Yo-Pro-1) and FL4 log (E). The percentage of viable spermatozoa with low superoxide levels (E-/Yo-Pro1-) was evaluated.
Annexin V can be used as a sensitive probe to detect phosphatidylserine (PS) exposure on the cell membrane since this protein has a high affinity for PS (Vermes et al. 1995). For the analysis, aliquots of 50 μL of sperm samples were diluted (2.7 × 107 cells/mL) with 250 μL of 1X binding buffer (provided in the commercial kit; Binding Buffer Apoptosis Detection Kit, Life Technologies, Carlsbad, CA, USA) and stained with 3 μL of 1.5 mM PI and 2 μL FITC-Annexin V conjugate (also provided in the kit). After 15 min of incubation (37°C in darkness), samples were assessed by flow cytometry. The monitored parameters were FS log, SS log, FL1 log (Annexin-V) and FL4 log (PI). The percentage of viable spermatozoa with no PS translocation (Annexin-/PI-) was evaluated.
Active caspase-3 and -7 were detected using the Vybrant™ FAM Caspase-3 and -7 Assay Kit for flow cytometry (Thermo Fisher Scientific, Waltham, MA, USA). For the assay, samples of 300 μL (5.3 × 106 cells/mL) were stained with 3 μL of the 30 × FAM-Peptide-FMK and were incubated at 37°C and 5% CO2 in darkness for 60 min. After washing twice with 300 μL of the washing buffer supplied with the kit by centrifuging (600g, 8 min, room temperature), the obtained pellet was resuspended in 300 μL of washing buffer, and cells were evaluated by flow cytometry. Just before the analysis, cells were mixed with 0.5 μL of 2 mM Ethidium Homodimer-1 (EthD-1, Thermo Fisher Scientific, Waltham, MA, USA) to identify the non-viable population. The monitored parameters were FS log, SS log, FL1 log (FITC-VADFMK; carboxyfluorescein (FAM) derivative of benzyloxycarbonyl-valinealanine-aspartic acid-fluoromethyl ketone (VAD-FMK)) and FL4 log (EthD-1). The percentage of viable spermatozoa without active caspases (FITC-VADFMK-/EthD-1-) was evaluated.
Assessment of capacitation status by chlortetracycline (CTC) staining
The capacitation status was determined using chlortetracycline (CTC) staining (Ward and Storey 1984). A CTC solution (750 μM) was prepared daily in a buffer containing 20 mM Tris, 130 mM NaCl and 5 μM cysteine (pH 7.8) and passed through a 0.22 μm filter. For the analysis, 20 μL of sperm sample (1.6 × 108 cells/mL) were mixed with 20 μL of CTC solution and fixed with 5 μL of 1.25% (w/v) paraformaldehyde in 0.5 M Tris-HCl (pH 7.8). Samples were incubated at 4°C in the dark for at least 30 min. After incubation, a 4 μL aliquot of the stained sample was placed on a glass slide and mixed with 2 μL of 0.22 M triethylenediamine (DABCO) in glycerol:PBS (9:1, v/v) at room temperature and semi-darkness. Samples were then covered with 24 × 48 mm coverslips, sealed with nail polish, and stored in the dark at −20°C. To evaluate CTC patterns, samples were examined using a Nikon Eclipse E-400 microscope (Kanagawa, Japan) under epifluorescence illumination using a V-2 A filter, and 200 spermatozoa were scored per slide. Sperm classification followed Gillan et al. (1997): non-capacitated spermatozoa (with even yellow fluorescence over the head, with or without a bright equatorial band); capacitated cells (with fluorescence on the acrosome) and acrosome-reacted cells (without fluorescence on the head and with or without a bright equatorial band).
Statistical analysis
Data are shown as median, interquartile range (IQR), 1.5 × IQR and the outliers by box and whiskers diagrams (Tukey style). Normality was evaluated by the Kolmogorov–Smirnov test. Differences between control samples (TALP or Cocktail (CK)) and melatonin-treated samples were analysed with Wilcoxon matched-pairs signed-rank test, which compares two paired groups (in our experiments, each swim-up selected sperm sample from rams carrying different RsaI allelic variants incubated with melatonin was compared with its TALP or CK medium without melatonin control). Statistical analyses were performed with SPSS Statistics v.26 (IBM Analytic, Armonk, NY, USA), and figures were created with GraphPad Prism v.8 (La Jolla, CA, USA).
Results
Experiment 1: influence of melatonin in sperm samples from rams carrying different RsaI allelic variants incubated in TALP medium
The addition of melatonin to TALP-incubated samples did not have an effect on total sperm motility for any of the RsaI genotypes tested, either in the reproductive or in the non-reproductive season (Supplemental Table 1). However, during the reproductive season, melatonin provoked a significant decrease (P = 0.047) in the percentage of progressive spermatozoa in TT samples (Fig. 1b). No effect was observed during the non-reproductive season (Fig. 1a) or for the rest of the genotypes.
Box-and-whisker plot representing the median (line within the box), the interquartile range (IQR: length of the box), the 1.5IQR (whiskers above and below the box) and the outliers (black dot) of the percentages of progressive motile spermatozoa in TALP-incubated sperm samples (3 h at 39°C, 100% humidity, 5% CO2) from rams carrying different RsaI genotypes without (Control, TALP) or with melatonin 1 μM (TALP + Mel 1 μM) during the non-reproductive (a) and the reproductive season (b). n = 11 for non-reproductive season and n = 10 for reproductive season. *indicates significant differences (P < 0.05) between the control and the melatonin-treated samples.
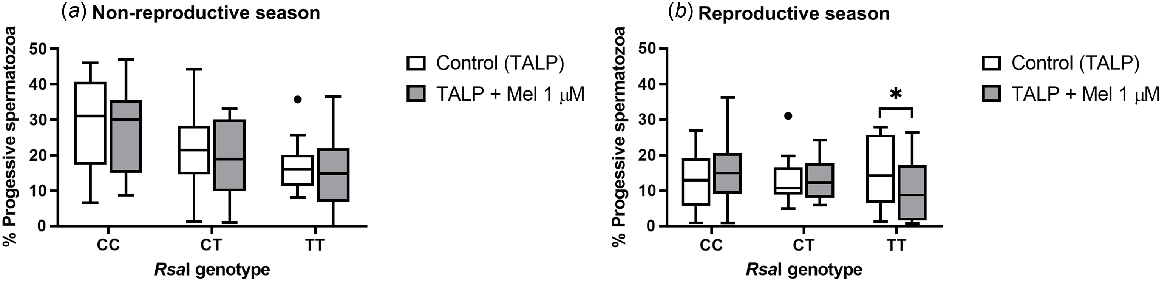
During both non-reproductive and reproductive seasons, there were no statistical differences in terms of viability (membrane integrity), mitochondrial activity or oxidative damage (both the production of reactive oxygen species (ROS) and superoxide (O2˙−) between the control and melatonin-treated samples in any of the genotypes tested (Supplemental Table 2).
Regarding the inversion of phosphatidylserine (PS), assessed by Annexin-V binding, melatonin 1 μM caused a significant decrease (P = 0.0195) in the percentage of viable spermatozoa with no PS translocation in TT samples during the reproductive season (Fig. 2b). However, this effect was not detected for the other genotypes in any season (Fig. 2a).
Box-and-whisker plot representing the median (line within the box), the interquartile range (IQR: length of the box), the 1.5IQR (whiskers above and below the box) and the outliers (black dot) of the percentages of viable spermatozoa with no phosphatidylserine (PS) inversion in TALP-incubated sperm samples (3 h at 39°C, 100% humidity, 5% CO2) from rams carrying different RsaI genotypes without (Control, TALP) or with melatonin 1 μM (TALP + Mel 1 μM) during the non-reproductive (a) and the reproductive season (b). n = 11 for non-reproductive season and n = 10 for reproductive season. *P < 0.05 indicates significant differences between the control and the treated samples.
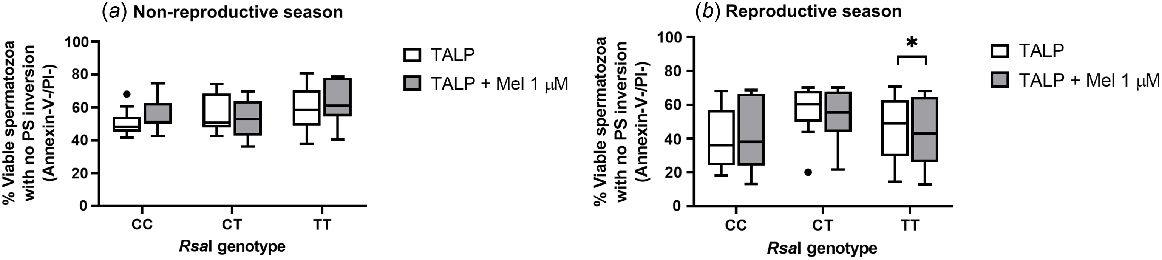
When evaluating active caspases, no significant effects were caused by melatonin 1 μM in comparison to control samples, since the percentage of viable sperm cells with no active caspases was not altered in any genotype, both during the non-reproductive and reproductive seasons (Supplemental Table 3).
Melatonin only affected the sperm capacitation status during the reproductive season in CT and TT samples (Fig. 3b). In these samples, melatonin induced a slight decapacitating effect, increasing the percentage of non-capacitated sperm cells (for CT, P = 0.0313; for TT, P = 0.0215) and decreasing the percentage of capacitated spermatozoa (for CT, P = 0.0313; for TT, P = 0.0195) when compared with the control samples. However, this effect was not seen in CC samples and was not observed for any samples during the non-reproductive season.
Box-and-whisker plot representing the median (line within the box), the interquartile range (IQR: length of the box), the 1.5IQR (whiskers above and below the box) and the outliers (black dot) of the percentages of non-capacitated, capacitated and reacted spermatozoa in sperm samples incubated in TALP medium (3 h at 39°C, 100% humidity, 5% CO2) from rams carrying different RsaI genotypes without (Control, TALP) or with melatonin 1 μM (TALP + Mel 1 μM) during the non-reproductive (a) and the reproductive season (b). n = 10 in both seasons. *P < 0.05 indicates significant differences between the control and the treated samples.
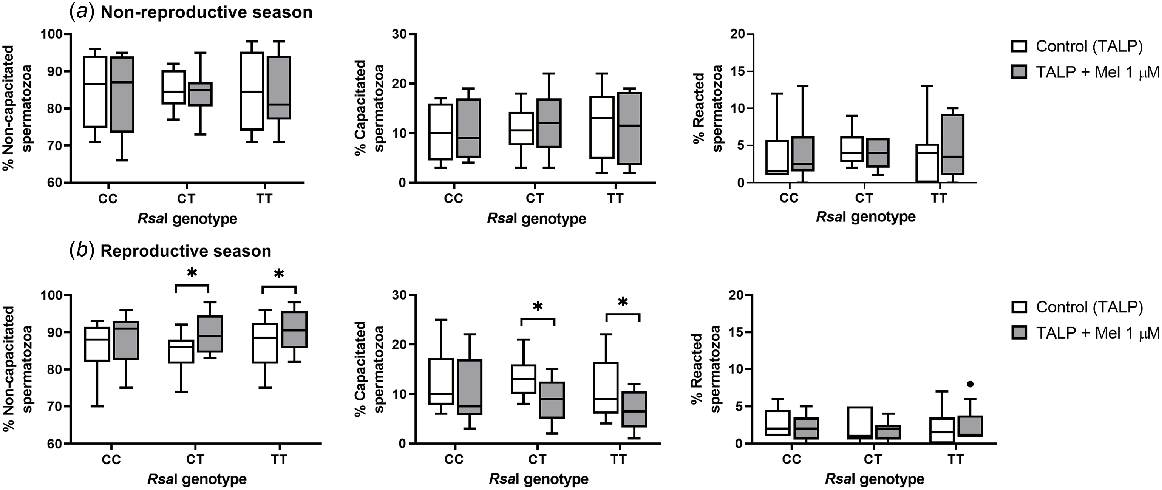
Experiment 2: influence of melatonin in sperm samples from rams carrying different RsaI allelic variants incubated in a ram sperm capacitation-trigger medium (CK medium)
As seen in TALP-incubated samples, the addition of melatonin to CK-incubated samples did not have an effect on total sperm motility for any of the RsaI genotypes tested either in the reproductive or the non-reproductive season (Supplemental Table 4). However, the presence of melatonin significantly decreased (P = 0.0156) the percentage of progressive spermatozoa in CC samples compared with control samples during the reproductive season (Fig. 4b). In contrast, no effect was observed during the non-reproductive season (Fig. 4a) or for the rest of the genotypes.
Box-and-whisker plot representing the median (line within the box), the interquartile range (IQR: length of the box) and the 1.5IQR (whiskers above and below the box) of the percentages of progressive motile spermatozoa in sperm samples incubated (3 h at 39°C, 100% humidity, 5% CO2) in TALP medium with cAMP-elevating agents (CK medium) from rams carrying different RsaI genotypes without (Control, CK) or with melatonin 1 μM (CK + Mel 1 μM) during the non-reproductive (a) and the reproductive season (b). n = 11 for non-reproductive season and n = 10 for reproductive season. *indicates significant differences (P < 0.05) between the control and the melatonin-treated samples.
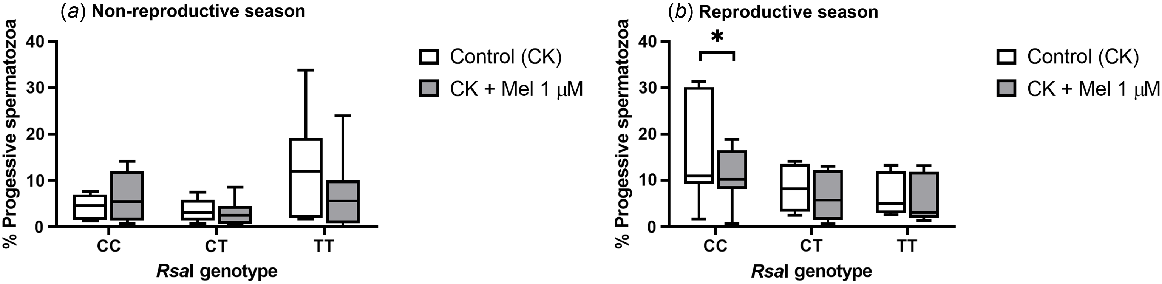
When incubations were performed in the CK medium, no statistically significant changes were observed in terms of intracellular superoxide (O2˙−) levels and viability (membrane integrity) between melatonin-treated and control samples during either the reproductive or non-reproductive seasons (Supplemental Table 5).
However, when evaluating intracellular ROS levels, there was a significant increase (P = 0.015) in the percentage of viable sperm cells with low levels of ROS in melatonin-incubated CT samples when compared with CT control samples only in the reproductive season (Fig. 5b). But no other changes were detected for the other genotypes, regardless of the season assessed.
Box-and-whisker plot representing the median (line within the box), the interquartile range (IQR: length of the box), the 1.5IQR (whiskers above and below the box) and the outliers (black dot) of the percentages of viable sperm cells with low levels of intracellular ROS levels in sperm samples incubated (3 h at 39°C, 100% humidity, 5% CO2) in TALP medium with cAMP-elevating agents (CK medium) from rams carrying different RsaI genotypes without (Control, CK) or with melatonin 1 μM (CK + Mel 1 μM) during the non-reproductive (a) and the reproductive season (b). n = 11 for the non-reproductive; n = 10 for reproductive season. *P < 0.05 indicates significant differences between the control and the treated samples.
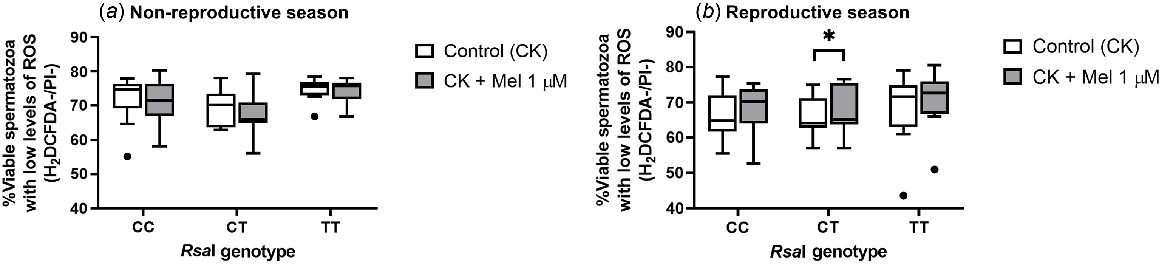
The effect of melatonin on the mitochondrial activity of CK-incubated samples was also evidenced only in the reproductive season. For CC samples, incubation with melatonin significantly reduced (P = 0.0371) the percentage of viable sperm cells with active mitochondria. In contrast, for the TT samples, melatonin significantly increased this percentage (P = 0.0273) (Fig. 6b).
Box-and-whisker plot representing the median (line within the box), the interquartile range (IQR: length of the box) and the 1.5IQR (whiskers above and below the box) of the percentages of viable sperm cells with active mitochondria in sperm samples incubated (3 h at 39°C, 100% humidity, 5% CO2) in TALP medium with cAMP-elevating agents (CK medium) from rams carrying different RsaI genotypes without (Control, CK) or with melatonin 1 μM (CK + Mel 1 μM) during the non-reproductive (a) and the reproductive season (b). n = 11 for the non-reproductive; n = 10 for reproductive season. *P < 0.05 indicates significant differences between the control and the treated samples.
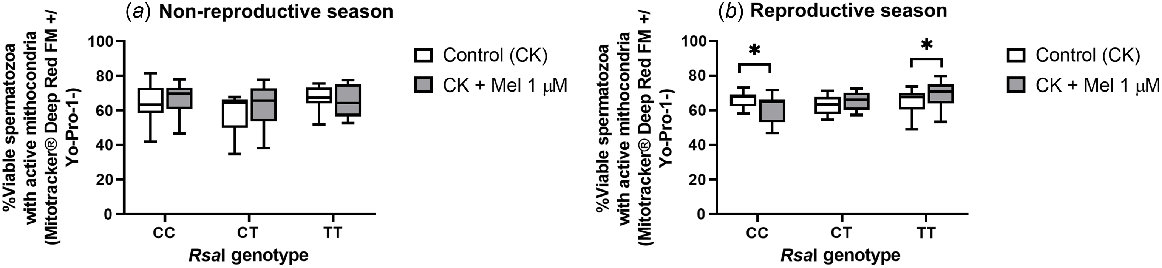
In this case, the addition of melatonin had no effect in terms of PS inversion, either in the non-reproductive or in the reproductive seasons (Supplemental Table 6). But in terms of caspase activation, this addition significantly increased (P = 0.0156) the percentage of viable sperm without active caspases in CT samples (Fig. 7b). This effect was again not observed in the non-reproductive season.
Box-and-whisker plot representing the median (line within the box), the interquartile range (IQR: length of the box), the 1.5IQR (whiskers above and below the box) and the outliers (black dot) of the percentages of viable with no active caspases in sperm samples incubated in TALP medium with cAMP-elevating agents (3 h at 39°C, 100% humidity, 5% CO2) from rams carrying different RsaI genotypes without (Control, CK) or with melatonin 1 μM (CK + Mel 1 μM) during the non-reproductive (a) and the reproductive season (b). n = 11 for the non-reproductive; n = 10 for reproductive season. *P < 0.05 indicates significant differences between the control and the treated samples.
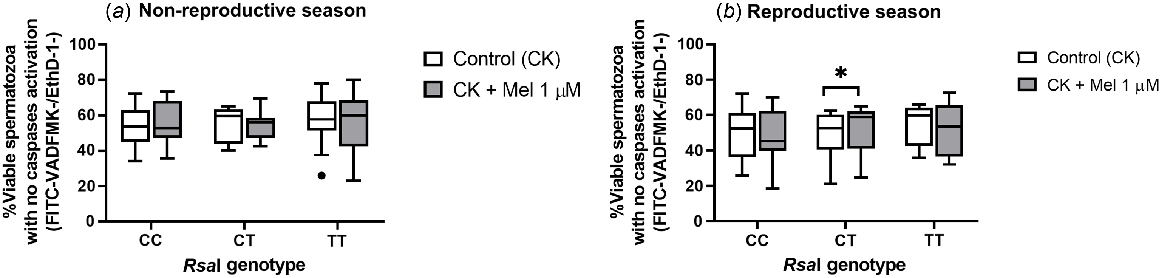
When melatonin was added during incubation in a CK medium, different effects were observed depending on the samples in both seasons. During the non-reproductive season, melatonin in CT and CC samples increased the percentage of non-capacitated spermatozoa (for CC, P = 0.047; for CT, P = 0.008) and decreased the rate of capacitated ones (for CC, P = 0.041; for CT, P = 0.011) when compared with the control samples. CC samples also showed a significant decrease (P = 0.02) in the percentage of reacted spermatozoa compared to control samples. However, during the reproductive season, melatonin 1 μM had a decapacitating effect in all sperm samples, significantly increasing the percentage of non-capacitated spermatozoa (for CC and CT, P = 0.005; for TT, P = 0.008) and decreasing the percentage of capacitated spermatozoa (P = 0.002, Fig. 8b).
Box-and-whisker plot representing the median (line within the box), the interquartile range (IQR: length of the box), the 1.5IQR (whiskers above and below the box) and the outliers (black dot) of the percentages of non-capacitated, capacitated and reacted spermatozoa in sperm samples incubated (3 h at 39°C, 100% humidity, 5% CO2) in TALP medium with cAMP-elevating agents (CK medium) from rams carrying different RsaI genotypes without (Control, CK) or with melatonin 1 μM (CK + Mel 1 μM) during the non-reproductive (a) and the reproductive season (b). n = 11 for the non-reproductive; n = 10 for reproductive season. *P < 0.05 and **P < 0.01 indicate significant differences between the control and the treated samples.
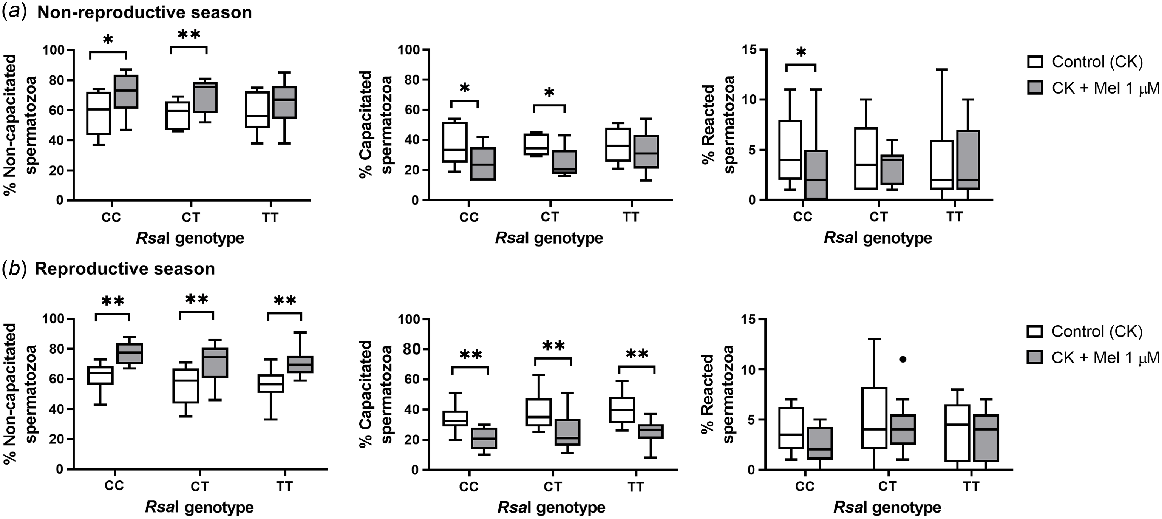
Discussion
The melatonin receptor MT1, encoded by the MTNR1A gene, presents one RFLP polymorphic site for the RsaI enzyme in exon II (Messer et al. 1997) that affects the seasonal reproductive functions of different sheep breeds (Carcangiu et al. 2009; Luridiana et al. 2016; Starič et al. 2020). In the Rasa Aragonesa breed, the T allele of this polymorphism has been associated with a shorter anoestrous period in ewes (Calvo et al. 2018) and a more intense reproductive behaviour in rams (Abecia et al. 2020), although with lower fertility (Abecia et al. 2023). However, the possible effects of this polymorphism on sperm cells have not yet been described, and so the influence of the RsaI genotypes in sperm quality and sperm response to melatonin remains unknown to date.
The results of Experiment 1 revealed that, during the non-reproductive season, the addition of melatonin 1 μM to TALP-incubated samples had no effect on sperm motility, viability, oxidative damage and apoptosis for any of the analysed genotypes. However, during the reproductive season, melatonin decreased the percentage of progressive and viable spermatozoa with no phosphatidylserine inversion when added to sperm samples from rams carrying the TT genotype. It had no effect on CC or CT samples. The MT1 receptor could be involved in modulating ram sperm motility, since our previous research group revealed that MT1 is present in the neck and in the middle part of the flagellum (Casao et al. 2012; González-Arto et al. 2016b), which is where mitochondria are located. Moreover, Fujinoki (2008) described that melatonin can potentiate sperm hyperactivation through the MT1 receptor in hamster sperm, with hyperactivation being a process generally characterised by non-progressive motility (Ooi et al. 2014). In the present work, the decrease in progressive motility only shown by spermatozoa from TT rams could imply that samples from these males show a different affinity or response to the presence of melatonin than samples from CC or CT rams. Moreover, melatonin also decreased the percentage of viable spermatozoa with no PS inversion in TT samples compared with the control ones, which suggests that this genotype may have a different sensitivity to the presence of melatonin in the TALP medium during the reproductive season.
However, the addition of the hormone did not alter sperm viability, which was evaluated as membrane integrity. The lack of effect of melatonin at 1 μM concentration on plasma membrane integrity is in concordance with previous results (Casao et al. 2010b; Gimeno-Martos et al. 2019; Fernández-Alegre et al. 2020), although other authors have reported beneficial effects of this hormone on sperm viability, but using higher melatonin concentrations and in experiments carried out with frozen (Succu et al. 2011; Ashrafi et al. 2013; Najafi et al. 2018; Zhu et al. 2019) or refrigerated (Kumar et al. 2022) spermatozoa. The presence of melatonin in TALP medium did not affect intracellular ROS and superoxide levels, mitochondrial activity or caspase activation for any of the genotypes tested in both seasons. This finding is intriguing, given that melatonin is well known for its antioxidant and antiapoptotic role (Zhao et al. 2019). With regard to its antioxidant role, melatonin can scavenge reactive oxygen species directly after crossing the plasma membrane, but it can also regulate oxidative stress by modulating the activities of antioxidant enzymes, among other mechanisms (Reiter et al. 2000). Concerning its antiapoptotic function, it has been described that the MT1 receptor may be involved in the suppression of apoptosis in some cell types (Barberino et al. 2017) and human spermatozoa (Espino et al. 2011).
This lack of effect of melatonin in TALP medium may be due to the fact that this medium itself cannot trigger the capacitation process in ram sperm (Colas et al. 2008). Considering that capacitation and apoptosis share signalling pathways (Luna et al. 2017) and that ROS are involved in both processes, we could hypothesise that melatonin may not play such a protective role in TALP medium since spermatozoa suffer fewer alterations when incubated in these lower capacitating conditions, regardless of their receptor genotype. Even so, a slight decapacitating effect was observed for CT and TT samples incubated in this medium during the reproductive season, as previously described by our group (Casao et al. 2010b). Nonetheless, a lower melatonin concentration, in the pM or nM range, which seems to promote capacitation in ovine spermatozoa when incubated without c-AMP elevating agents (Casao et al. 2010b), would help to clarify the involvement of RsaI allelic variants on the antiapoptotic and antioxidant effect exerted by melatonin in ram sperm.
When spermatozoa were incubated in Cocktail medium (CK, with c-AMP elevating agents) in Experiment 2, the addition of 1 μM melatonin did not affect sperm motility, viability, oxidative damage or apoptosis for any of the analysed genotypes during the non-reproductive season, as observed in Experiment 1. However, during the reproductive season, we found that melatonin provoked a significant decrease in the percentage of progressive motile and viable spermatozoa with active mitochondria in CC samples compared to its controls. On the contrary, the hormone significantly increased the rate of viable cells with low ROS levels and the percentage of viable cells with no active caspases in CT samples. Thus, in this experiment, the effect of melatonin 1 μM on CT samples is in concordance with the previously described antioxidant (Gimeno-Martos et al. 2019; Miguel-Jiménez et al. 2021) and antiapoptotic effect (Carvajal-Serna et al. 2021) in Cocktail medium. Moreover, melatonin significantly increased the percentage of viable spermatozoa with active mitochondria in TT samples compared with controls. Regarding sperm capacitation, the decapacitating role of melatonin 1 μM was evident for CT and CC samples in the non-reproductive season and all samples during the reproductive one in accordance with our previous research (Gimeno-Martos et al. 2019; Carvajal-Serna et al. 2021; Miguel-Jiménez et al. 2021).
Although RsaI polymorphism of the melatonin receptor 1A gene is known to be a silent mutation (Carcangiu et al. 2009), Calvo et al. (2018) described that it is in linkage disequilibrium with another polymorphism that does induce an amino acid change in the final protein. Moreover, the regulatory sequences of the gene linked to the RsaI polymorphism or other mutations in exon II, like MnlI, which is associated with another single nucleotide polymorphism that promotes a change from valine to isoleucine at position 220 of the transmembrane domain 5 (TM5) of the chain, might explain the observed differences in this work (Carcangiu et al. 2009; Trecherel et al. 2010; Pulinas et al. 2022). In relation to sperm capacitation, the effect of melatonin seems to be exerted via the MT2 receptor (Gonzalez-Arto et al. 2016a), so carrying different genotypes for the MT1 receptor may not affect the response to melatonin during capacitation. However, it has been reported that MT1 and MT2 receptors can form homo- and heterodimers (Ayoub et al. 2002), so variations in the response to melatonin of the sperm samples from rams with different RsaI allelic variants could be due to the mutated MT1 receptor affecting the functionality of the MT2 receptor when forming heterodimers.
Interestingly, the effect that melatonin had on spermatozoa from rams carrying different genotypes differs depending on the season. We could hypothesise that the distribution or density of MT1 receptors on the spermatozoa may vary depending on the allelic variant between seasons. Or, as melatonin concentration in seminal plasma changes throughout the year, with higher levels during the breeding season (Casao et al. 2010a), the higher amount of melatonin present in seminal plasma could affect the affinity or the activity of the MT1 receptor depending on the genotype during the reproductive season when a concentration of melatonin in the μM range was added to the medium. Nonetheless, the effect of lower melatonin concentrations on the different RsaI allelic variants remains unknown.
In conclusion, during the reproductive season, the presence of allele T in homozygosity in rams seems to be related to some adverse effects of melatonin when spermatozoa are incubated in a non-capacitating medium. In contrast, the presence of allele C in homozygosity would be linked with negative effects when the hormone is added to spermatozoa incubated in a capacitation-triggering medium. The decapacitating effect of melatonin on spermatozoa from rams carrying different genotypes is also different. Thus, taking into account the genotype of the rams based on their RsaI allelic variants of the MTNR1A gene could be helpful when carrying out in vitro reproductive techniques in the ovine species. Nonetheless, further studies on the effect of other MTNR1A gene polymorphisms (like MnlI) and other melatonin concentrations are necessary to clarify the involvement of MT1 receptor on ram spermatozoa functionality.
Conflicts of interest
The authors declare no conflicts of interest. Jose Alfonso Abecia is an Editor of the journal but was blinded from the peer review process for this paper.
Declaration of funding
This work was supported by Ministerio de Ciencia e Innovación, Spain (PID2020-113239RB-100) and Gobierno de Aragón (A07_23R). V P-D has a predoctoral contract from the Diputación General de Aragón (DGA).
Acknowledgements
The authors would like to thank the Universidad de Zaragoza’s Servicio General de Apoyo a la Investigación-SAI for their assistance, particularly J Burillo.
References
Abecia JA, Mura MC, Carvajal-Serna M, Pulinas L, Macías A, Casao A, Pérez-Pe R, Carcangiu V (2020) Polymorphisms of the melatonin receptor 1A (MTNR1A) gene influence the age at first mating in autumn-born ram-lambs and sexual activity of adult rams in spring. Theriogenology 157, 42-47.
| Crossref | Google Scholar | PubMed |
Abecia JA, Heredia A, Pérez-Pe R, Casao A, Carcangiu V, Mura MC, Miranda-De la Lama G (2022) Polymorphisms of the melatonin receptor 1A (MTNR1A) gene affect the sexual performance of Rasa Aragonesa rams without changing their social dominance. Journal of Animal Behaviour and Biometeorology 10, 2231.
| Crossref | Google Scholar |
Abecia JA, Macías A, Laviña A, Martín E, Casao A, Canto F, Carvajal-Serna M, Peña-Delgado V, Pérez-Pe R (2023) Polymorphisms of the melatonin receptor 1A (MTNR1A) gene of Rasa Aragonesa rams affect their fertility after artificial insemination. Animal - Science Proceedings 14, 77-78.
| Crossref | Google Scholar |
Alquézar-Baeta C, Gimeno-Martos S, Miguel-Jiménez S, Santolaria P, Yániz J, Palacín I, Casao A, Cebrián-Pérez JÁ, Muiño-Blanco T, Pérez-Pé R (2019) OpenCASA: A new open-source and scalable tool for sperm quality analysis. PLOS Computational Biology 15, e1006691.
| Crossref | Google Scholar |
Ashrafi I, Kohram H, Ardabili FF (2013) Antioxidative effects of melatonin on kinetics, microscopic and oxidative parameters of cryopreserved bull spermatozoa. Animal Reproduction Science 139, 25-30.
| Crossref | Google Scholar |
Avdi M, Banos G, Stefos K, Chemineau P (2004) Seasonal variation in testicular volume and sexual behavior of Chios and Serres rams. Theriogenology 62, 275-282.
| Crossref | Google Scholar | PubMed |
Ayoub MA, Couturier C, Lucas-Meunier E, Angers S, Fossier P, Bouvier M, Jockers R (2002) Monitoring of ligand-independent dimerization and ligand-induced conformational changes of melatonin receptors in living cells by bioluminescence resonance energy transfer. Journal of Biological Chemistry 277, 21522-21528.
| Crossref | Google Scholar | PubMed |
Barberino RS, Menezes VG, Ribeiro AEAS, Palheta RC, Jr, Jiang X, Smitz JEJ, Matos MHT (2017) Melatonin protects against cisplatin-induced ovarian damage in mice via the MT1 receptor and antioxidant activity. Biology of Reproduction 96, 1244-1255.
| Crossref | Google Scholar | PubMed |
Bass DA, Parce JW, Dechatelet LR, Szejda P, Seeds MC, Thomas M (1983) Flow cytometric studies of oxidative product formation by neutrophils: a graded response to membrane stimulation. Journal of Immunology 130, 1910-1917.
| Crossref | Google Scholar | PubMed |
Calvo JH, Serrano M, Martinez-Royo A, Lahoz B, Sarto P, Ibañez-Deler A, Folch J, Alabart JL (2018) SNP rs403212791 in exon 2 of the MTNR1A gene is associated with reproductive seasonality in the Rasa aragonesa sheep breed. Theriogenology 113, 63-72.
| Crossref | Google Scholar | PubMed |
Carcangiu V, Mura MC, Vacca GM, Pazzola M, Dettori ML, Luridiana S, Bini PP (2009) Polymorphism of the melatonin receptor MT1 gene and its relationship with seasonal reproductive activity in the Sarda sheep breed. Animal Reproduction Science 116, 65-72.
| Crossref | Google Scholar | PubMed |
Carter WO, Narayanan PK, Robinson JP (1994) Intracellular hydrogen peroxide and superoxide anion detection in endothelial cells. Journal of Leukocyte Biology 55, 253-258.
| Crossref | Google Scholar | PubMed |
Carvajal-Serna M, Cardozo-Cerquera JA, Grajales-Lombana HA, Casao A, Pérez-Pe R (2021) Sperm behavior and response to melatonin under capacitating conditions in three sheep breeds subject to the equatorial photoperiod. Animals 11, 1828.
| Crossref | Google Scholar |
Casao A, Cebrián I, Asumpção ME, Pérez-Pé R, Abecia JA, Forcada F, Cebrián-Pérez JA, Muiño-Blanco T (2010a) Seasonal variations of melatonin in ram seminal plasma are correlated to those of testosterone and antioxidant enzymes. Reproductive Biology and Endocrinology 8, 59.
| Crossref | Google Scholar | PubMed |
Casao A, Mendoza N, Pérez-Pé R, Grasa P, Abecia JA, Forcada F, Cebrián-Pérez JA, Muino-Blanco T (2010b) Melatonin prevents capacitation and apoptotic-like changes of ram spermatozoa and increases fertility rate. Journal of Pineal Research 48, 39-46.
| Crossref | Google Scholar | PubMed |
Casao A, Gallego M, Abecia JA, Forcada F, Pérez-Pé R, Muio-Blanco T, Cebrin-Ṕrez JL (2012) Identification and immunolocalisation of melatonin MT(1) and MT(2) receptors in Rasa Aragonesa ram spermatozoa. Reproduction, Fertility and Development 24, 953-961.
| Crossref | Google Scholar | PubMed |
Chemineau P, Guillaume D, Migaud M, Thiéry JC, Pellicer-Rubio MT, Malpaux B (2008) Seasonality of reproduction in mammals: intimate regulatory mechanisms and practical implications. Reproduction in Domestic Animals 43(Suppl 2), 40-47.
| Crossref | Google Scholar | PubMed |
Colas C, James P, Howes L, Jones R, Cebrian-Perez JA, Muiño-Blanco T (2008) Cyclic-AMP initiates protein tyrosine phosphorylation independent of cholesterol efflux during ram sperm capacitation. Reproduction, Fertility and Development 20, 649-658.
| Crossref | Google Scholar | PubMed |
Dubocovich ML, Markowska M (2005) Functional MT1 and MT2 melatonin receptors in mammals. Endocrine 27, 101-110.
| Crossref | Google Scholar | PubMed |
Espino J, Ortiz Á, Bejarano I, Lozano GM, Monllor F, García JF, Rodríguez AB, Pariente JA (2011) Melatonin protects human spermatozoa from apoptosis via melatonin receptor- and extracellular signal-regulated kinase-mediated pathways. Fertility and Sterility 95, 2290-2296.
| Crossref | Google Scholar | PubMed |
Fernández-Alegre E, Álvarez-Fernández I, Domínguez JC, Casao A, Martínez-Pastor F (2020) Melatonin non-linearly modulates bull spermatozoa motility and physiology in capacitating and non-capacitating conditions. International Journal of Molecular Sciences 21, 2701.
| Crossref | Google Scholar |
Forcada F, Abecia JA, Sierra I (1992) Seasonal changes in oestrus activity and ovulation rate in Rasa Aragonesa ewes maintained at two different body condition levels. Small Ruminant Research 8, 313-324.
| Crossref | Google Scholar |
Fujinoki M (2008) Melatonin-enhanced hyperactivation of hamster sperm. Reproduction 136, 533-541.
| Crossref | Google Scholar | PubMed |
García-López N, Ollero M, Muiño-Blanco T, Cebrián-Pérez JA (1996) A dextran swim-up procedure for separation of highly motile and viable ram spermatozoa from seminal plasma. Theriogenology 46, 141-151.
| Crossref | Google Scholar |
Gillan L, Evans AG, Maxwell WMC (1997) Capacitation status and fertility of fresh and frozen-thawed ram spermatozoa. Reproduction, Fertility and Development 9, 481-488.
| Crossref | Google Scholar | PubMed |
Gimeno-Martos S, Casao A, Yeste M, Cebrián-Pérez JA, Muiño-Blanco T, Pérez-Pé R (2019) Melatonin reduces cAMP-stimulated capacitation of ram spermatozoa. Reproduction, Fertility and Development 31, 420-431.
| Crossref | Google Scholar | PubMed |
Gonzalez-Arto M, Luna C, Pérez-Pé R, Muiño-Blanco T, Cebrián-Pérez JA, Casao A (2016a) New evidence of melatonin receptor contribution to ram sperm functionality. Reproduction, Fertility and Development 28, 924-935.
| Crossref | Google Scholar | PubMed |
González-Arto M, Vicente-Carrillo A, Martínez-Pastor F, Fernández-Alegre E, Roca J, Miró J, Rigau T, Rodríguez-Gil JE, Pérez-Pé R, Muiño-Blanco T, Cebrián-Pérez JA, Casao A (2016b) Melatonin receptors MT1 and MT2 are expressed in spermatozoa from several seasonal and nonseasonal breeder species. Theriogenology 86, 1958-1968.
| Crossref | Google Scholar | PubMed |
Grasa P, Cebrián-Pérez JÁ, Muiño-Blanco T (2006) Signal transduction mechanisms involved in in vitro ram sperm capacitation. Reproduction 132, 721-732.
| Crossref | Google Scholar | PubMed |
Guthrie HD, Welch GR (2006) Determination of intracellular reactive oxygen species and high mitochondrial membrane potential in Percoll-treated viable boar sperm using fluorescence-activated flow cytometry. Journal of Animal Science 84, 2089-2100.
| Crossref | Google Scholar | PubMed |
Hallap T, Nagy S, Jaakma Ü, Johannisson A, Rodriguez-Martinez H (2005) Mitochondrial activity of frozen-thawed spermatozoa assessed by MitoTracker Deep Red 633. Theriogenology 63, 2311-2322.
| Crossref | Google Scholar | PubMed |
Harrison RA, Vickers SE (1990) Use of fluorescent probes to assess membrane integrity in mammalian spermatozoa. Journal of Reproduction and Fertility 88, 343-352.
| Crossref | Google Scholar | PubMed |
Kumar T, Kumar P, Saini N, Bhalothia SK, Prakash C, Mahla AS, Kumar A (2022) Shielding effect of melatonin improves seminal quality and oxidative stress indices during chilled storage of ram semen. Tropical Animal Health and Production 54, 197.
| Crossref | Google Scholar |
Leahy T, Rickard JP, Aitken RJ, de Graaf SP (2016) Penicillamine prevents ram sperm agglutination in media that support capacitation. Reproduction 151, 167-177.
| Crossref | Google Scholar | PubMed |
Luna C, Mendoza N, Casao A, Pérez-Pé R, Cebrián-Pérez JA, Muiño-Blanco T (2017) c-Jun N-terminal kinase and p38 mitogen-activated protein kinase pathways link capacitation with apoptosis and seminal plasma proteins protect sperm by interfering with both routes. Biology of Reproduction 96, 800-815.
| Crossref | Google Scholar | PubMed |
Luridiana S, Mura MC, Daga C, Cosso G, Bodano S, Farci F, Zidda F, Carcangiu V (2016) Influences of melatonin treatment, melatonin receptor 1A (MTNR1A) and kisspeptin (KiSS-1) gene polymorphisms on first conception in Sarda ewe lambs. Reproduction, Fertility and Development 28, 750-756.
| Crossref | Google Scholar | PubMed |
Martínez-Royo A, Lahoz B, Alabart JL, Folch J, Calvo JH (2012) Characterisation of the Melatonin Receptor 1A (MTNR1A) gene in the Rasa Aragonesa sheep breed: Association with reproductive seasonality. Animal Reproduction Science 133, 169-175.
| Crossref | Google Scholar | PubMed |
Mateescu RG, Lunsford AK, Thonney ML (2009) Association between melatonin receptor 1A gene polymorphism and reproductive performance in Dorset ewes. Journal of Animal Science 87, 2485-2488.
| Crossref | Google Scholar | PubMed |
Messer LA, Wang L, Tuggle CK, Yerle M, Chardon P, Pomp D, Womack JE, Barendse W, Crawford AM, Notter DR, Rothschild MF (1997) Mapping of the melatonin receptor 1a (MTNR1A) gene in pigs, sheep, and cattle. Mammalian Genome 8, 368-370.
| Crossref | Google Scholar | PubMed |
Miguel-Jiménez S, Pina-Beltrán B, Gimeno-Martos S, Carvajal-Serna M, Casao A, Pérez-Pe R (2021) NADPH Oxidase 5 and melatonin: involvement in Ram Sperm Capacitation. Frontiers in Cell and Developmental Biology 9, 655794.
| Crossref | Google Scholar |
Najafi A, Adutwum E, Yari A, Salehi E, Mikaeili S, Dashtestani F, Abolhassani F, Rashki L, Shiasi S, Asadi E (2018) Melatonin affects membrane integrity, intracellular reactive oxygen species, caspase3 activity and AKT phosphorylation in frozen thawed human sperm. Cell and Tissue Research 372, 149-159.
| Crossref | Google Scholar |
Notter DR (2008) Genetic aspects of reproduction in sheep. Reproduction in Domestic Animals 43, 122-128.
| Crossref | Google Scholar | PubMed |
Ollero M, Muiño-Blanco T, López-Pérez MJ, Cebrián-Pérez JA (1996) Viability of ram spermatozoa in relation to the abstinence period and successive ejaculations. International Journal of Andrology 19, 287-292.
| Crossref | Google Scholar | PubMed |
Ooi EH, Smith DJ, Gadêlha H, Gaffney EA, Kirkman-Brown J (2014) The mechanics of hyperactivation in adhered human sperm. Royal Society Open Science 1, 140230.
| Crossref | Google Scholar |
Parrish JJ, Susko-Parrish J, Winer MA, First NL (1988) Capacitation of bovine sperm by heparin. Biology of Reproduction 38, 1171-1180.
| Crossref | Google Scholar | PubMed |
Pelletier J, Bodin L, Hanocq E, Malpaux B, Teyssier J, Thimonier J, Chemineau P (2000) Association between expression of reproductive seasonality and alleles of the gene for Mel(1a) receptor in the ewe. Biology of Reproduction 62, 1096-1101.
| Crossref | Google Scholar | PubMed |
Pulinas L, Starič J, Cosso G, Curone G, Mura MC, Carcangiu V, Luridiana S (2022) MTNR1A gene polymorphisms and reproductive recovery after seasonal anoestrus in different Mediterranean sheep breeds. Animal Reproduction Science 236, 106905.
| Crossref | Google Scholar |
Reiter RJ, Tan DX, Osuna C, Gitto E (2000) Actions of melatonin in the reduction of oxidative stress. A review. Journal of Biomedical Science 7, 444-458.
| Crossref | Google Scholar |
Rosa HJD, Bryant MJ (2003) Seasonality of reproduction in sheep. Small Ruminant Research 48, 155-171.
| Crossref | Google Scholar |
Starič J, Farci F, Luridiana S, Mura MC, Pulinas L, Cosso G, Carcangiu V (2020) Reproductive performance in three Slovenian sheep breeds with different alleles for the MTNR1A gene. Animal Reproduction Science 216, 106352.
| Crossref | Google Scholar |
Succu S, Berlinguer F, Pasciu V, Satta V, Leoni GG, Naitana S (2011) Melatonin protects ram spermatozoa from cryopreservation injuries in a dose-dependent manner. Journal of Pineal Research 50, 310-318.
| Crossref | Google Scholar | PubMed |
Trecherel E, Batailler M, Chesneau D, Delagrange P, Malpaux B, Chemineau P, Migaud M (2010) Functional characterisation of polymorphic variants for ovine MT1 melatonin receptors: Possible implication for seasonal reproduction in sheep. Animal Reproduction Science 122, 328-334.
| Crossref | Google Scholar | PubMed |
van Vuuren RJ, Pitout MJ, van Aswegen CH, Theron JJ (1992) Putative melatonin receptor in human spermatozoa. Clin Biochem 25, 125-127.
| Crossref | Google Scholar | PubMed |
Vermes I, Haanen C, Steffens-Nakken H, Reutellingsperger C (1995) A novel assay for apoptosis flow cytometric detection of phosphatidylserine expression on early apoptotic cells using fluorescein labelled Annexin V. Journal of Immunological Methods 184, 39-51.
| Crossref | Google Scholar | PubMed |
Ward CR, Storey BT (1984) Determination of the time course of capacitation in mouse spermatozoa using a chlortetracycline fluorescence assay. Developmental Biology 104, 287-296.
| Crossref | Google Scholar | PubMed |
Weaver DR, Liu C, Reppert SM (1996) Nature’s knockout: the Mel1b receptor is not necessary for reproductive and circadian responses to melatonin in Siberian hamsters. Molecular Endocrinology 10, 1478-1487.
| Crossref | Google Scholar | PubMed |
Zhao D, Yu Y, Shen Y, Liu Q, Zhao Z, Sharma R, Reiter RJ (2019) Melatonin synthesis and function: evolutionary history in animals and plants. Frontiers in Endocrinology 10, 249.
| Crossref | Google Scholar | PubMed |
Zhu Z, Li R, Lv Y, Zeng W (2019) Melatonin protects rabbit spermatozoa from cryo-damage via decreasing oxidative stress. Cryobiology 88, 1-8.
| Crossref | Google Scholar |