The intertwining roles of caveolin, oxytocin receptor, and the associated signalling pathways in prostate cancer progression
M. L. Gould
A Department of Anatomy, University of Otago, P.O. Box 913, Dunedin 9054, New Zealand.
Reproduction, Fertility and Development 35(9) 493-503 https://doi.org/10.1071/RD22283
Published online: 23 May 2023
© 2023 The Author(s) (or their employer(s)). Published by CSIRO Publishing. This is an open access article distributed under the Creative Commons Attribution-NonCommercial-NoDerivatives 4.0 International License (CC BY-NC-ND)
Abstract
Caveolae are invaginations in the plasma membrane of most cell types and are present in the cells of normal prostate tissue. Caveolins are a family of highly conserved integral membrane proteins that oligomerise to form caveolae and interact with signalling molecules by providing a scaffold that sequesters signal transduction receptors in close proximity to each other. Signal transduction G proteins and G-protein-coupled receptors (GPCR), including oxytocin receptor (OTR), are localised within caveolae. Only one OTR has been identified, and yet, this single receptor both inhibits and stimulates cell proliferation. As caveolae sequester lipid-modified signalling molecules, these differing effects may be due to a change in location. The cavin1 necessary for caveolae formation is lost in prostate cancer progression. With the loss of caveolae, the OTR moves out onto the cell membrane influencing the proliferation and survival of prostate cancer cells. Caveolin-1 (cav-1) is reportedly overexpressed in prostate cancer cells and is associated with disease progression. This review focuses on the position of OTRs within caveolae, and their movement out onto the cell membrane. It explores whether movement of the OTR is related to changes in the activation of the associated cell signalling pathways that may increase cell proliferation and analyse whether caveolin and particularly cavin1 might be a target for future therapeutic stratagies.
Keywords: cancer progression, caveolae, cavin1, cell signalling pathways, GPCR, lipid rafts, proliferation, sequestration.
Introduction
Accumulating evidence suggests that the expression of caveolin protein, the structural component of caveolae, acts as a suppressor in some tumours. Caveolin-1 (cav-1) protein localises to cell membrane invaginations called caveolae and has been shown to bind and regulate many of the signalling proteins that are involved in oncogenesis.
Oxytocin (OT) and oxytocin receptors (OTRs) are present in the human prostate (Whittington et al. 2004). Although the OTR has a single isoform, the OT peptide can both stimulate or inhibit proliferation (Bussolati and Cassoni 2001). Whether OTR is on the cell membrane or sequestered within cell membrane microdomains, called caveolae, may dictate these effects (Guzzi et al. 2002). The localisation of oxytocin receptors to caveolae may play a role in the regulation of receptor signalling, as caveolae are involved in the regulation of membrane trafficking and signal transduction. Overall, the localisation of oxytocin receptors to caveolae may play a role in the regulation of oxytocin signalling and function in various tissues throughout the body, including the prostate.
The focus of this review is on the role of lipid rafts and the subpopulation of lipid rafts, caveolae, which sequester components involved in G-protein-coupled receptor (GPCR) signal transduction that define the functional and significant, cell-specific regions of signalling by GPCRs.
Caveolae are a subset of lipid rafts
Lipid rafts form part of the plasma membrane, they are fashioned by coalescing stabilised assemblies of sphingolipid, cholesterol and proteins that form platforms on the flat areas of the plasma membrane from where they facilitate membrane signalling and trafficking. Whilst all mammalian cells express lipid rafts (Hooper 1999), only a few express morphological caveolae (Ostrom and Insel 2004).
Caveolae have a similar lipid configuration to lipid rafts but also express caveolin proteins on the inner leaflet of the plasma membrane bilayer (Anderson 1998). They are 50–100 nm specialised flask-like invaginations, or ‘little caves’, indented into the plasma membrane. Caveolae are relatively stable structures and by anchoring cell surface receptors, they can regulate signal transduction (Li et al. 1996; Anderson 1998). Three isoforms of caveolins exist: cav-1, caveolin-2 (cav-2) and caveolin-3 (cav-3). Morphologically functional caveolae are created only if the cells express the predominant isoform cav-1 (Song et al. 1996) or the striated muscle-specific isoform cav-3 (Fra et al. 1995; Li et al. 1996; Tang et al. 1996; Engelman et al. 1997). Many researchers have shown that the expression of cav-1, but not cav-2, was able to form caveolae-like vesicles (Fra et al. 1995; Scherer et al. 1996; Engelman et al. 1997; Li et al. 1998).
The formation of caveolae is a complex process that involves the assembly of a protein complex, which includes cav-1 and cavin1. Cavin1 plays a critical role in the regulation of caveolae formation and stability. Cavin1 interacts with cav-1 to form a stable complex necessary for caveolae formation. Cavin1 is also involved in the regulation of caveolae size, number and distribution on the plasma membrane. Overall, the essential role of cavin1 in caveolae formation highlights the importance of this protein in cellular processes and underscores the complexity of the molecular mechanisms that govern the formation and maintenance of caveolae in cells (Fig. 1).
Schematic depiction of the protein-lipid organisation of caveolae. Sphingolipid- and cholesterol-rich domains are in blue, plasma membrane in pink and lipid rafts in green. Oxytocin receptor GPCR proteins are shown in gold, as the classical heptahelical structures with associated G proteins. Caveolin oligomers are shown in black. Cavin1 stabilises the caveolin proteins to form caveolae structures. Caveolae are composed of oligomeric caveolin molecules interleaved into the cytoplasmic membrane.
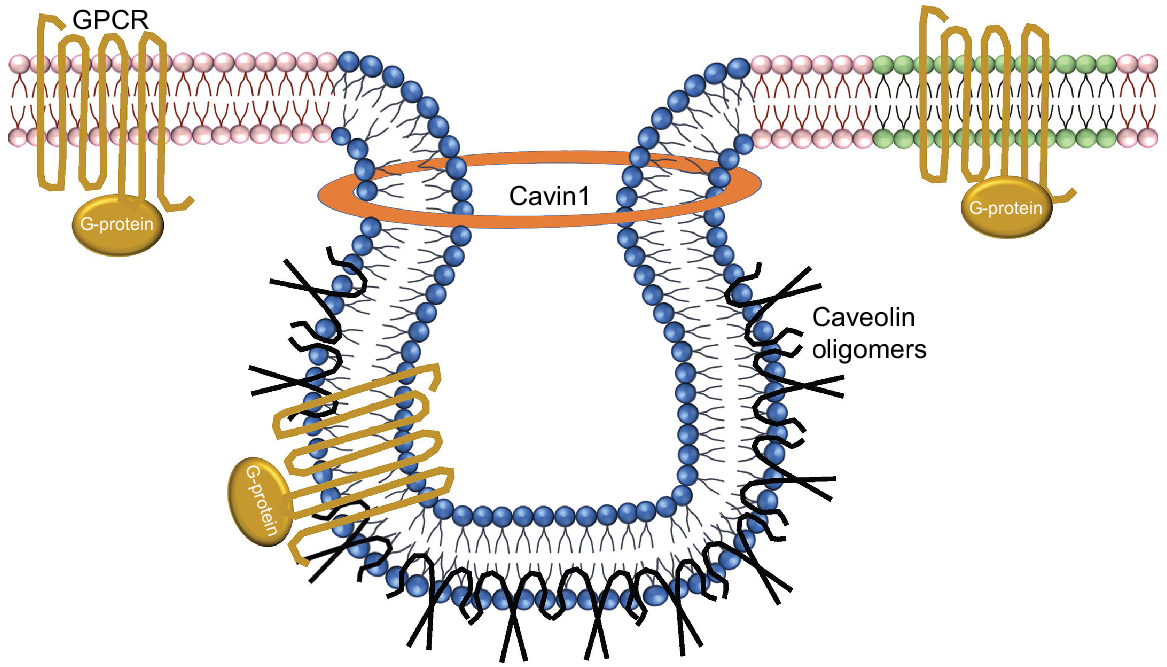
The sequestration of GPCR signalling molecules within lipid rafts or caveolae may be a collective mechanism, as restricting their movement would essentially increase the concentration of G proteins within a limited area, thereby favouring interactions amongst the various components in the signal transduction pathway. The caveolin-scaffolding domain recognises a well-defined caveolin-binding motif located within the enzymatically active catalytic domain of a given signalling molecule. Caveolin proteins form multivalent homo- and hetero-oligomers (Sargiacomo et al. 1995). The interface of the predominant cav-1 to signalling molecules is facilitated by a membrane-proximal region of caveolin, termed the caveolin-scaffolding domain (residues 82–101) as seen using domain-mapping studies. It is via this domain that cav-1 interacts not only with the G-protein α subunits. Mutational activation of the G-proteins signalling molecules can prevent interaction with the caveolin-scaffolding domain (Li et al. 1996) and may include H-Ras (G12V) and Gαs (Q227L) mutations found in human cancers.
In mouse embryonic fibroblast cells (NIH 3T3) transformed by activated oncogenes, cav-1 mRNA and protein expression were absent or severely reduced meaning that the cells also lost caveolae (Koleske et al. 1995). In these cells, cav-2 protein was not down-regulated in response to the oncogenic stimulus (Scherer et al. 1997). Furthermore, cells expressing the lowest amounts of cav-1 that consequently lacked caveolae formed larger colonies in soft agar, suggesting that such alterations may disrupt the necessary contact inhibition seen in normal cells (Koleske et al. 1995). In confluent normal prostate epithelial cells (PrEC), both cav-1 and -2 were localised to the edge of the cell consistent with cell-cell contact and correspondent to contact inhibition (Gould et al. 2010). Other researchers have also shown that both the distribution and the expression of cav-1 are markedly altered in normal confluent NIH 3T3 cells as cav-1 expression may mediate normal contact inhibition. Consequently, this would negatively regulate the activation state of the Ras-p42/44 MAP kinase cascade, positively regulating contact inhibition and growth arrest in normal cells (Galbiati et al. 1998; Galbiati et al. 2001).
Caveolin expression is up regulated in prostate cancer
Prostate cancer is the most commonly diagnosed male cancer, and yet much remains to be explained concerning the molecular and genetic events involved in prostate cancer progression. Most prostate cancers are slow growing and are contained within the prostate gland, i.e. ‘localised’ cancers. Unfortunately, in some men, this cancer can be aggressive and metastasise from the prostate to other parts of the body, particularly the bones and lymph nodes, eventually becoming fatal.
In the prostate, elevated cav-1 is correlated with cancer progression and this is also true of other urinary tract tumours, such as kidney and bladder cancers. Cav-1 is highly expressed in these tumours and rather than acting as a suppressor, is related to cancer progression and metastasis (Garnett 2016). The human genes encoding cav-1 and -2 are localised to an area termed the ‘suspected tumour suppressor locus’ (D7S522; chromosome 7q31.1). This highly conserved region encompasses a known fragile site and is frequently deleted. Deletion is associated with a loss of heterozygosity in a number of human cancers, including prostate cancer (Zenklusen et al. 1994; Engelman et al. 1998a, 1998b; Williams and Lisanti 2005). More studies need to be done to directly correlate genetic alterations at this site within cav-1 expression in prostate cancer (Hurlstone et al. 1999; Bachmann et al. 2008). Alterations in gene methylation have been shown to occur in prostate cancer, however, cav-1 gene methylation has not yet been shown to cause upregulation of cav-1 in prostate cancer (Cui et al. 2001). Cav-1 is also secreted by prostate cancer cells and this can facilitate cell survival and angiogenic activities, further defining cav-1 as playing a role in the prostate cancer microenvironment. Finally, cav-1 serum levels are increased in men with prostate cancer compared to patients without prostate cancer. Additionally, pre-operative cav-1 serum concentrations when compared to the Gleason grade have prognostic value in men undergoing radical prostatectomy (Tahir et al. 2003).
Loss of cavin1 expression in the prostate
The expression of cavin1 (also known as polymerase I and transcript release factor) is also required for the formation of caveolae (Hill et al. 2008) acting like the drawstring on a bag. Cavin1 is down regulated in prostate cancer and therefore may have some diagnostic potential. Decreased cavin1 expression in both epithelial and stromal tissue was seen in well differentiated cancer and no immunoreactivity was detected in tissue from patients with poorly differentiated cancer (Gould et al. 2010). In both tissue and cell lines, there is a loss of cavin1 with prostate tumour progression. We have also previously shown that with prostate cancer progression, caveolae are lost from the cell membrane (Gould et al. 2010). Re-expression of the protein cavin1 in androgen-independent prostate cancer (PC3) cells leads to the reappearance of caveolae and a reduction in the aggressive phenotype (Aung et al. 2011).
Epigenetics scrutinises changes in gene expression that happen without altering the DNA sequences, such as changes to the chromatin structure through histone modification and hypermethylation of the gene promoter region. Normal RWPE-1 prostate cell lines express higher levels of cavin1 compared to androgen dependent (LNCaP) and PC3 cell lines. When these cell lines are treated with DNA methylation and histone deacetylase (HDAC) inhibitors, cavin1 expression remained unaffected in the normal and androgen independent prostate cancer cells. However, the HDAC inhibitor, and HDAC inhibitor combined with the DNA methylation inhibitor, but not DNA methylation inhibitor alone, up-regulated Cavin1 expression in LNCaP cells (Low and Nicholson 2016). Bioinformatics of the potential HDAC genes showed that HDAC-2, -6 and -10 were probable candidate genes for the regulation of cavin1 expression, suggesting upstream regulatory mechanisms may be involved in modifying gene expression at differing stages of prostate cancer.
Hill et al. (2008) first described the lack of cavin1 in prostate cancer PC3 cells. Our group described the expression of cavin1 in normal prostate cell lines and tissues and that this expression was lost during the progression of prostate cancer (Gould et al. 2010). Additionally, over-expression of cavin1 led to a reduction in the proliferative capacity of PC3 cells and the silencing of cavin1 in the DU145 prostate cancer cell line increased the migratory capacity of these cells (Hill et al. 2012). The varying effects were mediated via the reduced expression of matrix metalloproteases, cytokines, proteases, and growth regulatory proteins (Aung et al. 2011; Inder et al. 2012).
In previous studies, we showed positive cav-1 immunostaining not only in the epithelium and stromal cells of human benign prostatic hyperplasia (BPH) tissue (Gould and Nicholson 2019) but also in normal epithelial and stromal cell lines (Gould et al. 2010). Other groups showed ~20% of BPH cases were positive for epithelial cav-1 (Mohammed and Helal 2017; Wang et al. 2018) and yet another research group have shown that cav-1 was not seen in prostate tissue epithelial cells at all, but was present in stromal cells (Moon et al. 2014). It may be that the N-terminal of cav-1 was cleaved in the prostate epithelium. Further studies could be done using techniques such as laser microdisection providing the focussed extraction of DNA, RNA or protein from specific types of cells.
In the prostate gland, cav-1 and cavin1 are expressed differently in the different cell types, including epithelial cells, stromal cells and smooth muscle cells. Epithelial cells are the primary cell type that makes up the glandular tissue of the prostate, and both cav-1 and cavin1 are expressed in these cells. Studies have shown that cav-1 and cavin1 expression is highest in the luminal epithelial cells that line the glandular ducts and produce secretions. Both cav-1 and cavin1 have been detected in stromal cells as well. In contrast, stromal levels of cav-1 were significantly decreased and all metastatic tumours were completely negative for stromal cav-1 staining in a subset of patients with primary prostate cancer (Di Vizio et al. 2009). Smooth muscle cells are also known to express cavin1, although the expression levels of this protein in the smooth muscle cells of the prostate are not well characterised.
Overall, the expression patterns in the different cells of the prostate suggest that these proteins may play important roles in regulating various cellular processes in the gland.
GPCR signalling compartmentation
GPCRs are seven-transmembrane helix proteins activated by ligand binding in the extracellular space that then transmits a signal to the cell through conformational changes that activate the heterotrimeric G-proteins, activating downstream signalling pathways. Individual GPCRs bind preferentially to multiple heterotrimeric G proteins, although some GPCRs can promiscuously couple to more than one G protein (Hermans 2003).
It is a necessity that the signalling proteins physically interact with high-affinity binding to transmit the required information. Taking into consideration the scarcity of signalling molecules within the cell, homogeneous distribution across the cell membrane is unlikely. To achieve the rapid signalling responses typical of GPCR activation, the concentration of signalling molecules within membrane microdomains may be a mechanism to explain the rapid response. The discovery of the caveolin proteins has provided a recognition system to biochemically identify caveolae and to demonstrate that signal transduction proteins are enriched within either lipid rafts or caveolae, or both (Anderson 1998; Razani et al. 2002; Pike 2003).
Importantly, not all GPCRs, or even G proteins are sequestered within lipid rafts. In fact, G proteins can also be located within both lipid rafts and non-raft fractions (Rybin et al. 2000; Ostrom et al. 2001).
Oxytocin receptor is a member of the GPCR family
The human prostate produces oxytocin (OT) and the oxytocin receptor (OTR) is also present in the gland. Higher levels of OT are found in tissue from men with BPH compared with men with prostate cancer (Nicholson 1996; Farina-Lipari et al. 2003).
OT treatment either has no effect or inhibits the proliferation of normal human prostate cells (Whittington et al. 2004). However, when malignant PC3 cells are treated with OT, increased cell proliferation is observed (Whittington et al. 2007). The OTR is a member of the GPCR family, however, only one OTR has been identified, which raises the question, how could one single receptor inhibit and stimulate cell proliferation and how does this relate to cancer activation and progression?
Earlier studies on the location of the OTR by Guzzi et al. (2002) showed that in the Madin–Darby canine kidney cell line, whether the OTR Gq-coupled receptor was compartmentalised within the cell membrane or was sequestered within caveolae could determine the proliferative effects of OT, that is localisation directly determined receptor signalling. Put simply, if the OTR was localised within the caveolae then there was a proliferative response, or if OTR was localised outside the caveolae, then inhibition of proliferation was seen. Furthermore, when OTR was located in the non-raft domains then its activation inhibited cell growth. Binding might desensitise the OTR when fused to caveolin and sequestration of the receptor within the lipid rafts indicates that its coupling to effector molecules may change the cellular response.
In normal cells and BPH tissue OTR co-localises with cavin1 suggesting localisation of the receptors in caveolae. Caveolae are present in PrEC, but the number of caveolae is significantly decreased in PC3 cells and cancer tissue (Hill et al. 2008; Gould et al. 2010). Along with the progression of cancer, the consequential loss of caveolae could allow for the movement of the cell surface receptors, one of which is OTR. This translocation could result in the activation of alternate signalling pathways that could favour cell proliferation, which would, in turn, favour the development and progression of prostate cancer (Rimoldi et al. 2003). Thus the loss of caveolae may contribute to, or facilitate, tumour growth by favouring cell proliferation and promoting the progression of prostate cancer.
Androgen withdrawal treatment (AWT) impedes prostate cancer progression initially, but cancer often escapes after a couple of years of treatment resulting in death (Harris et al. 2009). AWT initially causes tumour regression, however, later expanding tumorigenic populations occur alongside the subsequent breakdown of treatment (Arnold and Isaacs 2002).
Evidence exists that certain G proteins occur in different states. Gq preferentially localises to caveolae, whilst Gs and Gi prefer lipid rafts (Oh and Schnitzer 2001). Alongside the knowledge that caveolin is the unique identifier between caveolae and lipid rafts, Oh and Schnitzer in 2001 discovered that Gq, but not Gs and Gi immunoprecipitated together with cav-1, but when a cell was deficient in caveolin proteins then Gq would preferentially localise to lipid rafts. In other words, whereas any of the above G proteins can be localised in lipid rafts, Gq, of which OTR is one, preferentially localises into morphological caveolae, such as those available in normal prostate cells, where it binds to the caveolin scaffolding domain.
Localisation of the same protein molecules in caveolae can occur differently in different cell types. Low levels of OT in human prostate cells lead to localisation of OTR in lipid rafts (its native location), but with higher levels of OT, the OTR was found in non-raft fractions. Importantly, this is where the majority of both β1AR and β2AR were also detected (Ostrom and Insel 2004). These results suggest that lipid raft domains in some cells may contain a limited, saturable pool of signalling molecules, such as the enzyme adenylyl cyclase. Hence, different cells may localise the same protein differently. The implication being that the mechanisms overseeing lipid raft localisation could be independent of protein sequences, but may be dependent upon cell type instead. Perhaps these lipid modifications occur to a greater extent in one cell type compared to another and perhaps some cells contain only limited quantities of lipid rafts.
Caveolin and cancer progression
Cav-1 has been designated as a tumour suppressor as reduced expression of cav-1 is closely related to tumorigenesis. The reasoning behind this is that cav-1 impedes cell malignancy by inhibiting the cyclin D1 gene activity promoter, by inhibiting the mitogen-activated protein kinase (MAPK/ERK) pathway and by phosphorylating Src tyrosine kinase (Simpkins et al. 2012). Reduced expression of cav-1 can promote activation of gastric cancer-related fibroblasts, leading to gastric cancer (Shen et al. 2015) and reduced levels are also found in colorectal cancer (Bocci et al. 2012), ovarian cancer (Friedrich et al. 2013) and breast cancer (Xu et al. 2014).
However, in other tumours, the expression of cav-1 is significantly increased compared to normal tissues. Cav-1 is not expressed in normal liver tissue or normal liver cell lines but is increased in hepatocellular carcinoma and associated cell lines (Ting Tse et al. 2012), metastatic pancreatic cancer cells (Huang et al. 2012), hand, head and neck squamous cell carcinoma and metastasised kidney cancer cells (Steffens et al. 2011; Huang et al. 2012). Notably, in early-stage tumours that metastasise to the lymph nodes, cav-1 was highly upregulated and related to a poor prognosis (Steiner et al. 2012). Conversely, it was shown that decreased expression of cav-1 in prostate stroma contributes to tumour progression and that elevated cav-1 is highly correlated with human prostate cancer. We have shown that cav-1 and -2 were present in normal epithelial and stromal prostate cells grown in culture and in normal and BPH prostate tissue. This expression appeared to increase in prostate cancer tissue and again this was mirrored in vitro (Gould et al. 2010).
In prostate cancer, cav-1 may provide an important survival mechanism in environments with minimal or no androgens. In vitro cell culture studies with human prostate tumour cell lines have shown that cav-1 upregulation is correlated with increased cell survival, androgen independence, and increased metastatic potential (Nasu et al. 1998; Li et al. 2001) suggesting that cav-1 expression is associated with human prostate cancer progression (Yang et al. 1998). Furthermore, cav-1 can be down regulated during tumorigenesis in some cell types as oncologically transformed mouse embryonic fibroblast cells (NIH 3T3) showed down-regulated cav-1 protein and mRNA expression, up to 100-fold, related to the activation of the p42/44 MAPK cascade. Re-expression of caveolin and formation of caveolae was possible through the reverse engineering of NIH 3T3 cancer cells (Engelman et al. 1999). Additionally, anti-sense cav-1 inhibited the survival effects of testosterone and converted the castrate-resistant metastatic mouse prostate cancer cells to an androgen-sensitive phenotype (Nasu et al. 1998).
In PC3 cells that express cav-2, siRNA cav-2 knock down down-regulated CAV2 resulting in reduced cell numbers, migration and invasion in PC3 cells suggesting that CAV2 promoted malignant behaviour in an androgen-independent prostate cancer cell line, but further investigations need to be done in this area (Low and Nicholson 2017).
Alterations in cell signalling pathway activation
The role of caveolae in cell signalling pathways rely upon their ability to assemble together protein elements of signal transduction pathways. In this respect, we studied the MAPK/ERK pathway as these proteins communicate a signal from a receptor on the cell surface to the DNA in the cell nucleus. In spite of incorporating different treatments and a variety of experiments, the malignant cancer cells were not able to stimulate the phosphorylation of extracellular signal-regulated kinases (p-ERK) (Gould and Nicholson 2019). The MAPK/ERK pathway is usually activated by stimulation of OTR, which phosphorylates ERK, thereby stimulating mitosis. In the progression of all cancers, the stimulation of uncontrolled growth is a crucial step and this defect in the MAPK/ERK pathway instigates uncontrolled growth (Gioeli et al. 1999; Rick et al. 2012). In our study, in normal prostate cells, all treatments with OT or dihydrotestosterone (DHT) increased the expression of ERK (Fig. 2a, P < 0.0001), whereas, in PC3 cells the levels were severely reduced (Fig. 2b, P = 0.12). Confirmation of this was seen in poorly differentiated prostate cancer that expressed reduced levels of p-ERK. Whereas, in well-differentiated cancer, expression of p-ERK was seen, and this has been supported by other researchers (Kreisberg et al. 2004). Stimulation of the p-ERK pathway has been found in the human benign RWPE epithelial cell line and the WPMY stromal cell line derived from the same prostate (Xu et al. 2017). p-ERK has been implicated in the control of cell proliferation and differentiation. Consequently, we expected to see a change in the expression of p-ERK in PC3 cells because in normal cells, OTR was sequestered within caveolae (Guzzi et al. 2002) activating the signalling pathway via the phosphorylation of ERK, that inhibits cell proliferation.
Cell signalling pathway activation in normal and malignant prostate cells. (a, b) Extracellular signal-regulated kinase 1/2 (Erk1,2); (c, d) signal transducer and activator of transcription 1 (Stat1); (e, f) poly (ADP-ribose) polymerase (PARP); (g, h) Caspase 3; (i, j) p53; (k, l) p38; (m, n) stress-activated protein kinase (SAPK); (o, p) Ak strain transforming/phosphorylation of serine 473 (Ser473). The PathScan Intracellular Signalling Array (Cell Signalling) that detected the phosphorylation of signalling molecules was captured using the Odyssey digital imaging system (Li-CorBiosciences) and intensity was quantified using array analysis software. DRAQ5 5 mM (Abcam) determined the same number of cells were seeded in each well. Grey histograms denote normal prostate cells (PrEC); black are malignant cells (PC3) at 4 days, all grown in media containing androgens. OT is oxytocin, DHT is dihydrotestosterone. n = 4. *P = 0.05, **P = 0.01, ****P < 0.001.
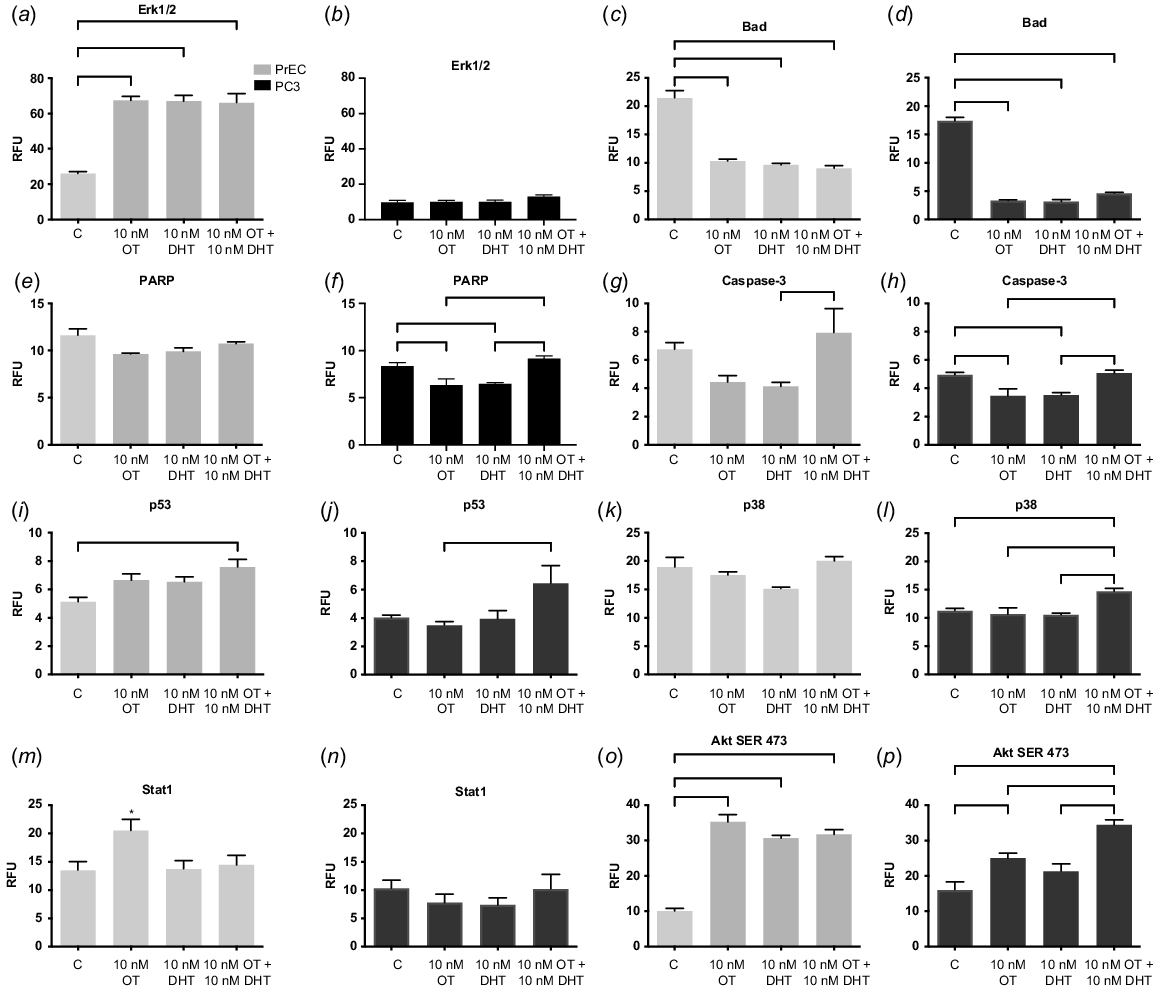
Malignant cells in the presence of OT can reduce the activation of the apoptotic pathway, specifically caspase-3, BCL2-associated death promoter (BAD) and poly (ADP-ribose) polymerase (PARP). Research indicates that increased expression of BAD can stimulate the proliferation of prostate cancer cells providing an advantage to the prostate tumour, whilst BAD dephosphorylation provides an increase in sensitivity of the cancer cells towards apoptosis (Smith et al. 2009). In our studies, BAD was markedly decreased in both cell types (Fig. 2c, d, P < 0.0001) indicating a possible governing pathway of OT and steroid hormones over this regulatory mechanism.
PARP initiates DNA repair and can regulate androgen receptor (AR) expression in advanced prostate cancer (Han et al. 2013). Interestingly, in malignant cells (Fig. 2f, P = 0.0005) but not in normal cells, (Fig. 2e, P = 0.0456) there was an increase in PARP expression with physiological OT and DHT, compared to OT and DHT alone. This was also seen in the increased expression of p38 in malignant cells (Fig. 2k, P = 0.0016) but not in normal cells (Fig. 2l, P = 0.09), and with Caspase in normal (Fig. 2g, P = 0.0230) and malignant (Fig. 2h, P = 0.0009) cells, which could initiate increased apoptosis (Tikhomirov and Carpenter 2004). This bears further investigation but could be related to the interaction of the AR and OTR, as we have indicated previously (Gould and Nicholson 2019). Further to this, P53 is a tumour suppressor gene that halts tumour formation by regulating cell division by keeping cells from proliferating uncontrollably. In normal cells, treatment with OT and DHT increased p53 (Fig. 2i, P = 0.0039), which was also seen in malignant cells (Fig. 2j, P = 0.0259). Interestingly, other researchers have found that p53 alone was sufficient to increase the expression of cav-1, but when both the proto-oncogenic tumour suppressor protein retinoblastoma protein (Rb1) and p53 were lost, as in the castration resistant phenotype in prostate cancer, even though p53 was an instigator of cancer progression, in the prostate this needed interaction with the other protein to create its full effect (Lin et al. 2019).
Deregulation of signal transducers and activators of transcription factors (STAT) has been implicated in prostate cancer cell proliferation and survival (Blando et al. 2011). Aberrant activation of STAT acts as a pro-survival signal for tumour cells by tightly regulating cell cycle progression, transformation and preventing apoptosis. STAT1 in particular suppresses cancer growth, progression and apoptosis by regulating caspase expression, or by interacting with p53. STAT1 knockout mice develop significantly more sarcomas than wild type mice (Kaplan et al. 1998). STAT1 hyperactivation has been implicated in multiple cancer types and STAT1 is induced by the use of docetaxel treatment in DU145 prostate cancer cells. Furthermore, STAT1 was overexpressed in a generated docetaxel-resistant cell line (DU145-DR). In our hands, with the treatment of OT in the normal prostate cells, then Stat1 expression was seen to increase, (Fig. 2m, P = 0.0363) whereas in the malignant PC3 cells (Fig. 2n, P = 0.5) no effect was seen. This supports other research that indicates STAT1 expression may function as either a suppressor or promoter of cancer development (Kaplan et al. 1998).
In PC3 cells, OT increased the activation of Akt (Ser473), the growth and survival factor in both normal and malignant cells (Fig. 2o, p, P < 0.0001). In androgen-independent prostate cancer, Akt hyperphosphorylates the AR hence increasing AR activity in a milieu of reduced androgens, hyperactivating AR without the presence of the ligand instigating increased proliferation (Yeh et al. 1998). In breast cancer, cav-1 triggers the PI3-K-Akt signalling pathway in prostate cancer cells promoting metastasis by interacting with angiogenic factors like VEGF, TGF-β1 and FGF2. Cav-1 can also increase cell expression in MCF-7 human breast adenocarcinoma cells where cav-1 overexpression was associated with AKT overactivity (Fiucci et al. 2002). In oesophageal squamous cell carcinoma, the lack of cav-1 expression activated AKT, consequently upregulating TGF-β1 and Synuclein Gamma (SNCG) (Wang et al. 2014). The presence of OT or DHT activated signalling pathways may stimulate cell proliferation and survival in PC3 cells, which could increase cell numbers thereby supporting the proliferative state of the tumour.
Caveolin proteins have been implicated in the development and progression of various types of cancer. In both prostate and breast cancer, caveolin expression is dysregulated with higher levels of caveolin associated with a poorer prognosis. In both cancer types, caveolin has been implicated in regulating cell growth, survival, and migration, and therefore may contribute to the capacity of the cancer cells to invade surrounding tissue and metastasise to distant sites. One of the unique features of caveolin in prostate cancer is its association with the AR, a key regulator of prostate development and function. Caveolin interacts with AR to modulate its activity, influencing the growth and survival of prostate cancer cells. This association, of course, is not present in breast cancer, but instead caveolin has been linked with regulating oestrogen receptor activity. While both cancers are hormonally regulated, the specific hormones involved and their effects on caveolin expression and activity differ. In prostate cancer, androgens are the key hormones, while in breast cancer, oestrogen and progesterone play the central role.
Discussion
The increased cav-1 expression identified in prostate cancer could be associated with interactions between phosphorylation and dephosphorylation of scaffolding proteins and the associated multiple signalling pathways suggest that cav-1 may perform an important role in the progression of prostate cancer. Increased expression of cav-1 is strongly related to prostate cancer occurrence, progression, and metastasis of the tumours. Cav-1 promotes the release of numerous growth factors by controlling transcription, up-regulating mRNA and protein expression levels, stimulating tumour angiogenesis and tumour formation.
By what means does cav-1 change its function from tumour suppressor to tumour growth promoter? In the development of prostate cancer, there is an increase in the levels of caveolin expression and a further increase in metastatic cancer (Yang et al. 1998). Many articles equate caveolin expression to the presence of morphological caveolae formation (Chang et al. 1994). Our studies have shown that this may not be true as caveolin is also expressed in non-caveolae parts of the cell membrane. In prostate cancer, caveolin protein cannot be associated with caveolae since we have shown that morphological caveolae are lost in cancer progression (Gould et al. 2010).
The outcome of these results allowed us to suggest that the OT peptide can confer different effects due to the position of OTR residing within the cell membrane. In the absence of caveolae, caveolin and cell surface receptors associate transiently with more mobile lipid rafts on the surface of the cell membrane (Fig. 3).
Schematic depiction of OTR signalling in lipid rafts and caveolae of normal and malignant prostate cancer cells. In (a) normal cells, OTR is located in caveolae and treatment with OT resulted in an inhibition of proliferation. In (b) malignant cells, there is a loss of caveolae and OTR moves to the lipid rafts where in response to OT there is a stimulation of proliferation, or in response to OT and T then OTR moves from the lipid rafts onto the cell membrane, again stimulating proliferation.
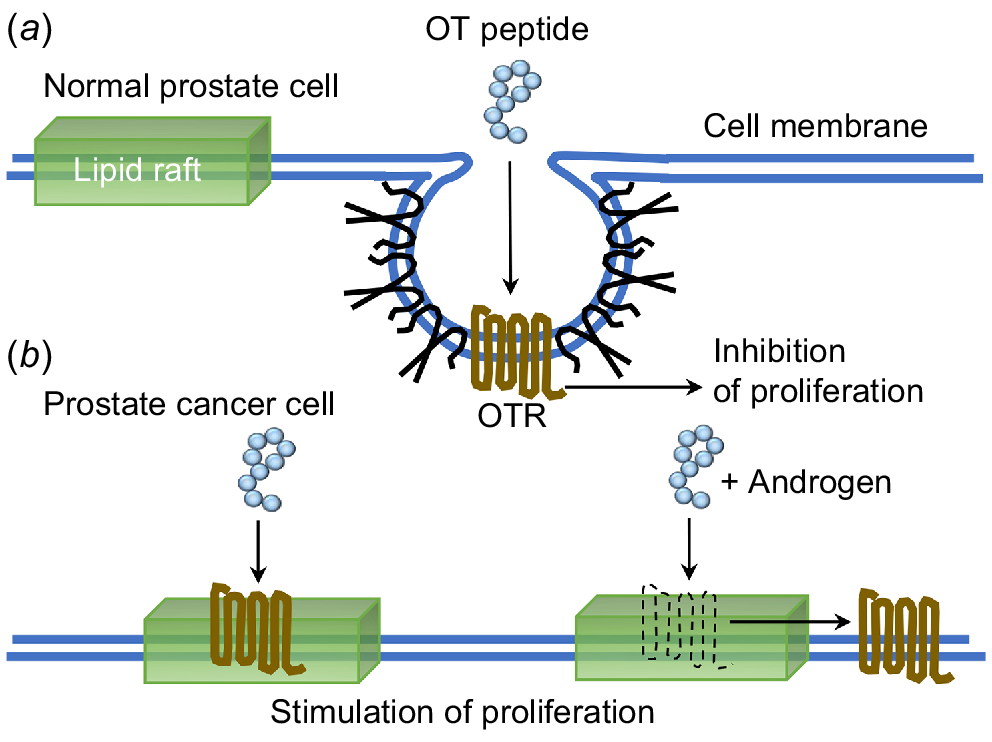
Future directions
Cell membrane receptors are proteins located on the surface of cells that play a key role in cell signalling, allowing cells to communicate with their environment and respond to changes in their surroundings. Dysregulation of cell membrane receptors has been implicated in cancer development and progression. The localisation of oxytocin receptors to caveolae may play a role in the regulation of receptor signalling, as caveolae are involved in the regulation of membrane trafficking and signal transduction. Specifically, the localisation of oxytocin receptors to caveolae may play a role in the regulation of oxytocin signalling and function in the prostate. As the expression of cavin1 is critical for morphological caveolae formation and the solidification of mobile caveolin proteins into immobile caveolae structures (Hill et al. 2008) and indeed, this may be a suitable biomarker for prostate cancer progression.
The focus of this review is on the role of lipid rafts and the subpopulation of lipid rafts, caveolae, which sequester components involved in GPCR signal transduction that define the functional and significant, cell-specific regions of signalling by GPCRs. Further studies are required to shed more light on cavin1 and caveolin in the context of this disease and their possibile use as a diagnostic tool. Specifically targeting these proteins would present a significant challenge as they are involved in a variety of processes with important roles in normal cellular function. Additionally, caveolae-associated proteins exhibit dynamic complex interactions with other proteins making it problematic to specifically target them without interfering with other downstream cellular processes.
Conclusion
The premise of lipid rafts and caveolae has garnered interest because these microdomains compartmentalise signalling proteins creating an intimate association between signalling molecules to facilitate the efficient fast flow of information through a specific signal transduction pathway. Understanding signal transduction in the environment of lipid rafts or caveolae is critical for interpreting the complexity of cell signalling in the cancer microenvironment. Cell-specific differences in the sequestration of signalling components within the microdomains might offer a potential prospect to target microdomains differentially influencing various receptors in different cell types. It is well known that caveolin proteins form structured cell membrane invaginations called caveolae that bind and regulate cell membrane receptors in prostate tissue. When cavin1 is lost, the caveolae structures are lost, and the sequestered receptors move out onto the cell membrane triggering signalling pathways promoting cancer progression.
The G protein-coupled receptor, OTR is an example of one such receptor that is sequestered within the caveolae of normal cells in the prostate and its association with caveolin is required for proper signalling but with cancer progression, cavin1 expression is lost and with the consequential loss of caveolae structures the OTR moves onto the cell membrane where it activates signalling pathways, from here, OTR is linked to the regulation of cell growth and survival in prostate cancer.
Developing new treatments for prostate cancer should focus on understanding the underlying mechanisms that drive the development, the switch to invasive malignancy and the progression of the disease. In this way, biomarkers such as cavin1 as a molecular indicator for prostate cancer could diagnose the disease earlier to facilitate more effective treatments.
Understanding these proteins highlights the complexity of reproductive biology and the importance of studying it from multiple angles in order to add a new perspective to our general understanding of cancer biology and to fully understand its intricacies.
References
Anderson, RGW (1998). The caveolae membrane system. Annual Review of Biochemistry 67, 199–225.| The caveolae membrane system.Crossref | GoogleScholarGoogle Scholar |
Arnold, JT, and Isaacs, JT (2002). Mechanisms involved in the progression of androgen-independent prostate cancers: it is not only the cancer cell’s fault. Endocrine-Related Cancer 9, 61–73.
| Mechanisms involved in the progression of androgen-independent prostate cancers: it is not only the cancer cell’s fault.Crossref | GoogleScholarGoogle Scholar |
Aung, CS, Hill, MM, Bastiani, M, Parton, RG, and Parat, M-O (2011). PTRF–cavin-1 expression decreases the migration of PC3 prostate cancer cells: role of matrix metalloprotease 9. European Journal of Cell Biology 90, 136–142.
| PTRF–cavin-1 expression decreases the migration of PC3 prostate cancer cells: role of matrix metalloprotease 9.Crossref | GoogleScholarGoogle Scholar |
Bachmann, N, Haeusler, J, Luedeke, M, Kuefer, R, Perner, S, Assum, G, Paiss, T, Hoegel, J, Vogel, W, and Maier, C (2008). Expression changes of CAV1 and EZH2, located on 7q31~q36, are rarely related to genomic alterations in primary prostate carcinoma. Cancer Genetics and Cytogenetics 182, 103–110.
| Expression changes of CAV1 and EZH2, located on 7q31~q36, are rarely related to genomic alterations in primary prostate carcinoma.Crossref | GoogleScholarGoogle Scholar |
Blando, JM, Carbajal, S, Abel, E, Beltran, L, Conti, C, Fischer, S, and DiGiovanni, J (2011). Cooperation between Stat3 and Akt signaling leads to prostate tumor development in transgenic mice. Neoplasia 13, 254–265.
| Cooperation between Stat3 and Akt signaling leads to prostate tumor development in transgenic mice.Crossref | GoogleScholarGoogle Scholar |
Bocci, G, Fioravanti, A, Orlandi, P, Di Desidero, T, Natale, G, Fanelli, G, Viacava, P, Naccarato, AG, Francia, G, and Danesi, R (2012). Metronomic ceramide analogs inhibit angiogenesis in pancreatic cancer through up-regulation of caveolin-1 and thrombospondin-1 and down-regulation of cyclin D1. Neoplasia 14, 833–845.
| Metronomic ceramide analogs inhibit angiogenesis in pancreatic cancer through up-regulation of caveolin-1 and thrombospondin-1 and down-regulation of cyclin D1.Crossref | GoogleScholarGoogle Scholar |
Bussolati, G, and Cassoni, P (2001). Editorial: the oxytocin/oxytocin receptor system – expect the unexpected. Endocrinology 142, 1377–1379.
| Editorial: the oxytocin/oxytocin receptor system – expect the unexpected.Crossref | GoogleScholarGoogle Scholar |
Chang, WJ, Ying, YS, Rothberg, KG, Hooper, NM, Turner, AJ, Gambliel, HA, De Gunzburg, J, Mumby, SM, Gilman, AG, and Anderson, RG (1994). Purification and characterization of smooth muscle cell caveolae. The Journal of Cell Biology 126, 127–138.
| Purification and characterization of smooth muscle cell caveolae.Crossref | GoogleScholarGoogle Scholar |
Cui, J, Rohr, LR, Swanson, G, Speights, VO, Maxwell, T, and Brothman, AR (2001). Hypermethylation of the caveolin-1 gene promoter in prostate cancer. Prostate 46, 249–256.
Di Vizio, D, Morello, M, Sotgia, F, Pestell, RG, Freeman, MR, and Lisanti, MP (2009). An absence of stromal caveolin-1 is associated with advanced prostate cancer, metastatic disease and epithelial Akt activation. Cell Cycle 8, 2420–2424.
| An absence of stromal caveolin-1 is associated with advanced prostate cancer, metastatic disease and epithelial Akt activation.Crossref | GoogleScholarGoogle Scholar |
Engelman, JA, Wykoff, CC, Yasuhara, S, Song, KS, Okamoto, T, and Lisanti, MP (1997). Recombinant expression of caveolin-1 in oncogenically transformed cells abrogates anchorage-independent growth. Journal of Biological Chemistry 272, 16374–16381.
| Recombinant expression of caveolin-1 in oncogenically transformed cells abrogates anchorage-independent growth.Crossref | GoogleScholarGoogle Scholar |
Engelman, JA, Zhang, XL, Galbiati, F, and Lisanti, MP (1998a). Chromosomal localization, genomic organization, and developmental expression of the murine caveolin gene family (Cav-1, -2, and -3): Cav-1 and Cav-2 genes map to a known tumor suppressor locus (6-a2/7q31). FEBS Letters 429, 330–336.
| Chromosomal localization, genomic organization, and developmental expression of the murine caveolin gene family (Cav-1, -2, and -3): Cav-1 and Cav-2 genes map to a known tumor suppressor locus (6-a2/7q31).Crossref | GoogleScholarGoogle Scholar |
Engelman, JA, Zhang, XL, and Lisanti, MP (1998b). Genes encoding human caveolin-1 and -2 are co-localized to the D7S522 locus (7q31.1), a known fragile site (FRA7G) that is frequently deleted in human cancers. FEBS Letters 436, 403–410.
| Genes encoding human caveolin-1 and -2 are co-localized to the D7S522 locus (7q31.1), a known fragile site (FRA7G) that is frequently deleted in human cancers.Crossref | GoogleScholarGoogle Scholar |
Engelman, JA, Zhang, XL, Razani, B, Pestell, RG, and Lisanti, MP (1999). p42/44 MAP kinase-dependent and -independent signaling pathways regulate caveolin-1 gene expression: activation of Ras-MAP kinase and protein kinase a signaling cascades transcriptionally down-regulates caveolin-1 promoter activity. Journal of Biological Chemistry 274, 32333–32341.
| p42/44 MAP kinase-dependent and -independent signaling pathways regulate caveolin-1 gene expression: activation of Ras-MAP kinase and protein kinase a signaling cascades transcriptionally down-regulates caveolin-1 promoter activity.Crossref | GoogleScholarGoogle Scholar |
Farina-Lipari, E, Lipari, D, Bellafiore, M, Anzalone, R, Cappello, F, and Valentino, B (2003). Presence of atrial natriuretic factor in normal and hyperplastic human prostate and its relationship with oxytocin localisation. European Journal of Histochemistry 47, 133–138.
| Presence of atrial natriuretic factor in normal and hyperplastic human prostate and its relationship with oxytocin localisation.Crossref | GoogleScholarGoogle Scholar |
Fiucci, G, Ravid, D, Reich, R, and Liscovitch, M (2002). Caveolin-1 inhibits anchorage-independent growth, anoikis and invasiveness in MCF-7 human breast cancer cells. Oncogene 21, 2365–2375.
| Caveolin-1 inhibits anchorage-independent growth, anoikis and invasiveness in MCF-7 human breast cancer cells.Crossref | GoogleScholarGoogle Scholar |
Fra, AM, Williamson, E, Simons, K, and Parton, RG (1995). De novo formation of caveolae in lymphocytes by expression of VIP21-caveolin. Proceedings of the National Academy of Sciences of the United States of America 92, 8655–8659.
| De novo formation of caveolae in lymphocytes by expression of VIP21-caveolin.Crossref | GoogleScholarGoogle Scholar |
Friedrich, T, Richter, B, Gaiser, T, Weiss, C, Janssen, K-P, Einwachter, H, Schmid, RM, Ebert, MPA, and Burgermeister, E (2013). Deficiency of caveolin-1 in Apcmin/+ mice promotes colorectal tumorigenesis. Carcinogenesis 34, 2109–2118.
| Deficiency of caveolin-1 in Apcmin/+ mice promotes colorectal tumorigenesis.Crossref | GoogleScholarGoogle Scholar |
Galbiati, F, Volonte, D, Engelman, JA, Watanabe, G, Burk, R, Pestell, RG, and Lisanti, MP (1998). Targeted downregulation of caveolin-1 is sufficient to drive cell transformation and hyperactivate the p42/44 MAP kinase cascade. The EMBO Journal 17, 6633–6648.
| Targeted downregulation of caveolin-1 is sufficient to drive cell transformation and hyperactivate the p42/44 MAP kinase cascade.Crossref | GoogleScholarGoogle Scholar |
Galbiati, F, Engelman, JA, Volonte, D, Zhang, XL, Minetti, C, Li, M, Hou, H, Kneitz, B, Edelmann, W, and Lisanti, MP (2001). Caveolin-3 null mice show a loss of caveolae, changes in the microdomain distribution of the dystrophin-glycoprotein complex, and t-tubule abnormalities. Journal of Biological Chemistry 276, 21425–21433.
| Caveolin-3 null mice show a loss of caveolae, changes in the microdomain distribution of the dystrophin-glycoprotein complex, and t-tubule abnormalities.Crossref | GoogleScholarGoogle Scholar |
Garnett, DJ (2016). Caveolae as a target to quench autoinduction of the metastatic phenotype in lung cancer. Journal of Cancer Research and Clinical Oncology 142, 611–618.
| Caveolae as a target to quench autoinduction of the metastatic phenotype in lung cancer.Crossref | GoogleScholarGoogle Scholar |
Gioeli, D, Mandell, JW, Petroni, GR, Frierson, HF, and Weber, MJ (1999). Activation of mitogen-activated protein kinase associated with prostate cancer progression. Cancer Research 59, 279–284.
Gould, ML, and Nicholson, HD (2019). Changes in receptor location affect the ability of oxytocin to stimulate proliferative growth in prostate epithelial cells. Reproduction, Fertility and Development 31, 1166–1179.
| Changes in receptor location affect the ability of oxytocin to stimulate proliferative growth in prostate epithelial cells.Crossref | GoogleScholarGoogle Scholar |
Gould, ML, Williams, G, and Nicholson, HD (2010). Changes in caveolae, caveolin, and polymerase 1 and transcript release factor (PTRF) expression in prostate cancer progression. The Prostate 70, 1609–1621.
| Changes in caveolae, caveolin, and polymerase 1 and transcript release factor (PTRF) expression in prostate cancer progression.Crossref | GoogleScholarGoogle Scholar |
Guzzi, F, Zanchetta, D, Cassoni, P, Guzzi, V, Francolini, M, Parenti, M, and Chini, B (2002). Localization of the human oxytocin receptor in caveolin-1 enriched domains turns the receptor-mediated inhibition of cell growth into a proliferative response. Oncogene 21, 1658–1667.
| Localization of the human oxytocin receptor in caveolin-1 enriched domains turns the receptor-mediated inhibition of cell growth into a proliferative response.Crossref | GoogleScholarGoogle Scholar |
Han, S, Brenner, JC, Sabolch, A, Jackson, W, Speers, C, Wilder-Romans, K, Knudsen, KE, Lawrence, TS, Chinnaiyan, AM, and Feng, FY (2013). Targeted radiosensitization of ETS fusion-positive prostate cancer through PARP1 inhibition. Neoplasia 15, 1207–IN36.
| Targeted radiosensitization of ETS fusion-positive prostate cancer through PARP1 inhibition.Crossref | GoogleScholarGoogle Scholar |
Harris, WP, Mostaghel, EA, Nelson, PS, and Montgomery, B (2009). Androgen deprivation therapy: progress in understanding mechanisms of resistance and optimizing androgen depletion. Nature Clinical Practice Urology 6, 76–85.
| Androgen deprivation therapy: progress in understanding mechanisms of resistance and optimizing androgen depletion.Crossref | GoogleScholarGoogle Scholar |
Hermans, E (2003). Biochemical and pharmacological control of the multiplicity of coupling at G-protein-coupled receptors. Pharmacology & Therapeutics 99, 25–44.
| Biochemical and pharmacological control of the multiplicity of coupling at G-protein-coupled receptors.Crossref | GoogleScholarGoogle Scholar |
Hill, MM, Bastiani, M, Luetterforst, R, Kirkham, M, Kirkham, A, Nixon, SJ, Walser, P, Abankwa, D, Oorschot, VMJ, Martin, S, Hancock, JF, and Parton, RG (2008). PTRF-cavin, a conserved cytoplasmic protein required for caveola formation and function. Cell 132, 113–124.
| PTRF-cavin, a conserved cytoplasmic protein required for caveola formation and function.Crossref | GoogleScholarGoogle Scholar |
Hill, MM, Daud, NH, Aung, CS, Loo, D, Martin, S, Murphy, S, Black, DM, Barry, R, Simpson, F, Liu, L, Pilch, PF, Hancock, JF, Parat, M-O, and Parton, RG (2012). Co-regulation of cell polarization and migration by caveolar proteins PTRF/cavin-1 and caveolin-1. PLoS ONE 7, e43041.
| Co-regulation of cell polarization and migration by caveolar proteins PTRF/cavin-1 and caveolin-1.Crossref | GoogleScholarGoogle Scholar |
Hooper, NM (1999). Detergent-insoluble glycosphingolipid/cholesterol-rich membrane domains, lipid rafts and caveolae (review). Molecular Membrane Biology 16, 145–156.
| Detergent-insoluble glycosphingolipid/cholesterol-rich membrane domains, lipid rafts and caveolae (review).Crossref | GoogleScholarGoogle Scholar |
Huang, C, Qiu, Z, Wang, L, Peng, Z, Jia, Z, Logsdon, CD, Le, X, Wei, D, Huang, S, and Xie, K (2012). A novel foxM1-caveolin signaling pathway promotes pancreatic cancer invasion and metastasis. Cancer Research 72, 655–665.
| A novel foxM1-caveolin signaling pathway promotes pancreatic cancer invasion and metastasis.Crossref | GoogleScholarGoogle Scholar |
Hurlstone, AFL, Reid, G, Reeves, JR, Fraser, J, Strathdee, G, Rahilly, M, Parkinson, EK, and Black, DM (1999). Analysis of the CAVEOLIN-1 gene at human chromosome 7q31.1 in primary tumours and tumour-derived cell lines. Oncogene 18, 1881–1890.
| Analysis of the CAVEOLIN-1 gene at human chromosome 7q31.1 in primary tumours and tumour-derived cell lines.Crossref | GoogleScholarGoogle Scholar |
Inder, KL, Zheng, YZ, Davis, MJ, Moon, H, Loo, D, Nguyen, H, Clements, JA, Parton, RG, Foster, LJ, and Hill, MM (2012). Expression of PTRF in PC-3 cells modulates cholesterol dynamics and the actin cytoskeleton impacting secretion pathways. Molecular & Cellular Proteomics 11, M111.012245.
| Expression of PTRF in PC-3 cells modulates cholesterol dynamics and the actin cytoskeleton impacting secretion pathways.Crossref | GoogleScholarGoogle Scholar |
Kaplan, DH, Shankaran, V, Dighe, AS, Stockert, E, Aguet, M, Old, LJ, and Schreiber, RD (1998). Demonstration of an interferon γ-dependent tumor surveillance system in immunocompetent mice. Proceedings of the National Academy of Sciences of the United States of America 95, 7556–7561.
| Demonstration of an interferon γ-dependent tumor surveillance system in immunocompetent mice.Crossref | GoogleScholarGoogle Scholar |
Koleske, AJ, Baltimore, D, and Lisanti, MP (1995). Reduction of caveolin and caveolae in oncogenically transformed cells. Proceedings of the National Academy of Sciences of the United States of America 92, 1381–1385.
| Reduction of caveolin and caveolae in oncogenically transformed cells.Crossref | GoogleScholarGoogle Scholar |
Kreisberg, JI, Malik, SN, Prihoda, TJ, Bedolla, RG, Troyer, DA, Kreisberg, S, and Ghosh, PM (2004). Phosphorylation of akt (ser473) is an excellent predictor of poor clinical outcome in prostate cancer. Cancer Research 64, 5232–5236.
| Phosphorylation of akt (ser473) is an excellent predictor of poor clinical outcome in prostate cancer.Crossref | GoogleScholarGoogle Scholar |
Li, S, Couet, J, and Lisanti, MP (1996). Src tyrosine kinases, Gα subunits, and H-Ras share a common membrane-anchored scaffolding protein, caveolin: caveolin binding negatively regulates the auto-activation of Src tyrosine kinases. Journal of Biological Chemistry 271, 29182–29190.
| Src tyrosine kinases, Gα subunits, and H-Ras share a common membrane-anchored scaffolding protein, caveolin: caveolin binding negatively regulates the auto-activation of Src tyrosine kinases.Crossref | GoogleScholarGoogle Scholar |
Li, S, Galbiati, F, Volonte, D, Sargiacomo, M, Engelman, JA, Das, K, Scherer, PE, and Lisanti, MP (1998). Mutational analysis of caveolin-induced vesicle formation. Expression of caveolin-1 recruits caveolin-2 to caveolae membranes. FEBS Letters 434, 127–134.
| Mutational analysis of caveolin-induced vesicle formation. Expression of caveolin-1 recruits caveolin-2 to caveolae membranes.Crossref | GoogleScholarGoogle Scholar |
Li, L, Yang, G, Ebara, S, Satoh, T, Nasu, Y, Timme, TL, Ren, C, Wang, J, Tahir, SA, and Thompson, TC (2001). Caveolin-1 mediates testosterone-stimulated survival/clonal growth and promotes metastatic activities in prostate cancer cells. Cancer Research 61, 4386–4392.
Lin, C-J, Yun, E-J, Lo, U-G, Tai, Y-L, Deng, S, Hernandez, E, Dang, A, Chen, Y-A, Saha, D, Mu, P, Lin, H, Li, T-K, Shen, T-L, Lai, C-H, and Hsieh, J-T (2019). The paracrine induction of prostate cancer progression by caveolin-1. Cell Death & Disease 10, 834.
| The paracrine induction of prostate cancer progression by caveolin-1.Crossref | GoogleScholarGoogle Scholar |
Low, J-Y, and Nicholson, HD (2016). The up-stream regulation of polymerase-1 and transcript release factor(PTRF/Cavin-1) in prostate cancer: an epigenetic analysis. AIMS Molecular Science 3, 466–478.
| The up-stream regulation of polymerase-1 and transcript release factor(PTRF/Cavin-1) in prostate cancer: an epigenetic analysis.Crossref | GoogleScholarGoogle Scholar |
Low, J-Y, and Nicholson, HD (2017). Investigating the role of caveolin-2 in prostate cancer cell line. AIMS Molecular Science 4, 82–88.
| Investigating the role of caveolin-2 in prostate cancer cell line.Crossref | GoogleScholarGoogle Scholar |
Mohammed, DA, and Helal, DS (2017). Prognostic significance of epithelial/stromal caveolin-1 expression in prostatic hyperplasia, high grade prostatic intraepithelial hyperplasia and prostatic carcinoma and its correlation with microvessel density. Journal of the Egyptian National Cancer Institute 29, 25–31.
| Prognostic significance of epithelial/stromal caveolin-1 expression in prostatic hyperplasia, high grade prostatic intraepithelial hyperplasia and prostatic carcinoma and its correlation with microvessel density.Crossref | GoogleScholarGoogle Scholar |
Moon, H, Lee, CS, Inder, KL, Sharma, S, Choi, E, Black, DM, Lê Cao, K-A, Winterford, C, Coward, JI, Ling, MT, Australian Prostate Cancer BioResource Craik, DJ, Parton, RG, Russell, PJ, and Hill, MM (2014). PTRF/cavin-1 neutralizes non-caveolar caveolin-1 microdomains in prostate cancer. Oncogene 33, 3561–3570.
| PTRF/cavin-1 neutralizes non-caveolar caveolin-1 microdomains in prostate cancer.Crossref | GoogleScholarGoogle Scholar |
Nasu, Y, Timme, TL, Yang, G, Bangma, CH, Li, L, Ren, C, Park, SH, DeLeon, M, Wang, J, and Thompson, TC (1998). Suppression of caveolin expression induces androgen sensitivity in metastatic androgen-insensitive mouse prostate cancer cells. Nature Medicine 4, 1062–1064.
| Suppression of caveolin expression induces androgen sensitivity in metastatic androgen-insensitive mouse prostate cancer cells.Crossref | GoogleScholarGoogle Scholar |
Nicholson, HD (1996). Oxytocin: a paracrine regulator of prostatic function. Reviews of Reproduction 1, 69–72.
| Oxytocin: a paracrine regulator of prostatic function.Crossref | GoogleScholarGoogle Scholar |
Oh, P, and Schnitzer, JE (2001). Segregation of heterotrimeric G proteins in cell surface microdomains. G(q) binds caveolin to concentrate in caveolae, whereas G(i) and G(s) target lipid rafts by default. Molecular Biology of the Cell 12, 685–698.
| Segregation of heterotrimeric G proteins in cell surface microdomains. G(q) binds caveolin to concentrate in caveolae, whereas G(i) and G(s) target lipid rafts by default.Crossref | GoogleScholarGoogle Scholar |
Ostrom, RS, and Insel, PA (2004). The evolving role of lipid rafts and caveolae in G protein-coupled receptor signaling: implications for molecular pharmacology. British Journal of Pharmacology 143, 235–245.
| The evolving role of lipid rafts and caveolae in G protein-coupled receptor signaling: implications for molecular pharmacology.Crossref | GoogleScholarGoogle Scholar |
Ostrom, RS, Gregorian, C, Drenan, RM, Xiang, Y, Regan, JW, and Insel, PA (2001). Receptor number and caveolar co-localization determine receptor coupling efficiency to adenylyl cyclase. Journal of Biological Chemistry 276, 42063–42069.
| Receptor number and caveolar co-localization determine receptor coupling efficiency to adenylyl cyclase.Crossref | GoogleScholarGoogle Scholar |
Pike, LJ (2003). Lipid rafts: bringing order to chaos. Journal of Lipid Research 44, 655–667.
| Lipid rafts: bringing order to chaos.Crossref | GoogleScholarGoogle Scholar |
Razani, B, Wang, XB, Engelman, JA, Battista, M, Lagaud, G, Zhang, XL, Kneitz, B, Hou, H, Christ, GJ, Edelmann, W, and Lisanti, MP (2002). Caveolin-2-deficient mice show evidence of severe pulmonary dysfunction without disruption of caveolae. Molecular and Cellular Biology 22, 2329–2344.
| Caveolin-2-deficient mice show evidence of severe pulmonary dysfunction without disruption of caveolae.Crossref | GoogleScholarGoogle Scholar |
Rick, FG, Schally, AV, Szalontay, L, Block, NL, Szepeshazi, K, Nadji, M, Zarandi, M, Hohla, F, Buchholz, S, and Seitz, S (2012). Antagonists of growth hormone-releasing hormone inhibit growth of androgen-independent prostate cancer through inactivation of ERK and Akt kinases. Proceedings of the National Academy of Sciences of the United States of America 109, 1655–1660.
| Antagonists of growth hormone-releasing hormone inhibit growth of androgen-independent prostate cancer through inactivation of ERK and Akt kinases.Crossref | GoogleScholarGoogle Scholar |
Rimoldi, V, Reversi, A, Taverna, E, Rosa, P, Francolini, M, Cassoni, P, Parenti, M, and Chini, B (2003). Oxytocin receptor elicits different EGFR/MAPK activation patterns depending on its localization in caveolin-1 enriched domains. Oncogene 22, 6054–6060.
| Oxytocin receptor elicits different EGFR/MAPK activation patterns depending on its localization in caveolin-1 enriched domains.Crossref | GoogleScholarGoogle Scholar |
Rybin, VO, Xu, X, Lisanti, MP, and Steinberg, SF (2000). Differential targeting of β-adrenergic receptor subtypes and adenylyl cyclase to cardiomyocyte caveolae. A mechanism to functionally regulate the camp signaling pathway. Journal of Biological Chemistry 275, 41447–41457.
| Differential targeting of β-adrenergic receptor subtypes and adenylyl cyclase to cardiomyocyte caveolae. A mechanism to functionally regulate the camp signaling pathway.Crossref | GoogleScholarGoogle Scholar |
Sargiacomo, M, Scherer, PE, Tang, Z, Kubler, E, Song, KS, Sanders, MC, and Lisanti, MP (1995). Oligomeric structure of caveolin: implications for caveolae membrane organization. Proceedings of the National Academy of Sciences of the United States of America 92, 9407–9411.
| Oligomeric structure of caveolin: implications for caveolae membrane organization.Crossref | GoogleScholarGoogle Scholar |
Scherer, PE, Okamoto, T, Chun, M, Nishimoto, I, Lodish, HF, and Lisanti, MP (1996). Identification, sequence, and expression of caveolin-2 defines a caveolin gene family. Proceedings of the National Academy of Sciences of the United States of America 93, 131–135.
| Identification, sequence, and expression of caveolin-2 defines a caveolin gene family.Crossref | GoogleScholarGoogle Scholar |
Scherer, PE, Lewis, RY, Volonte, D, Engelman, JA, Galbiati, F, Couet, J, Kohtz, DS, van Donselaar, E, Peters, P, and Lisanti, MP (1997). Cell-type and tissue-specific expression of caveolin-2: caveolins 1 and 2 co-localize and form a stable hetero-oligomeric complex in vivo. Journal of Biological Chemistry 272, 29337–29346.
| Cell-type and tissue-specific expression of caveolin-2: caveolins 1 and 2 co-localize and form a stable hetero-oligomeric complex in vivo.Crossref | GoogleScholarGoogle Scholar |
Shen, X-J, Zhang, H, Tang, G-S, Wang, X-D, Zheng, R, Wang, Y, Zhu, Y, Xue, X-C, and Bi, J-W (2015). Caveolin-1 is a modulator of fibroblast activation and a potential biomarker for gastric cancer. International Journal of Biological Sciences 11, 370–379.
| Caveolin-1 is a modulator of fibroblast activation and a potential biomarker for gastric cancer.Crossref | GoogleScholarGoogle Scholar |
Simpkins, SA, Hanby, AM, Holliday, DL, and Speirs, V (2012). Clinical and functional significance of loss of caveolin-1 expression in breast cancer-associated fibroblasts. The Journal of Pathology 227, 490–498.
| Clinical and functional significance of loss of caveolin-1 expression in breast cancer-associated fibroblasts.Crossref | GoogleScholarGoogle Scholar |
Smith, AJ, Karpova, Y, D’Agostino, R, Willingham, M, and Kulik, G (2009). Expression of the Bcl-2 protein BAD promotes prostate cancer growth. PLoS ONE 4, e6224.
| Expression of the Bcl-2 protein BAD promotes prostate cancer growth.Crossref | GoogleScholarGoogle Scholar |
Song, KS, Scherer, PE, Tang, ZL, Okamoto, T, Li, S, Chafel, M, Chu, C, Kohtz, DS, and Lisanti, MP (1996). Expression of caveolin-3 in skeletal, cardiac, and smooth muscle cells: caveolin-3 is a component of the sarcolemma and co-fractionates with dystrophin and dystrophin-associated glycoproteins. Journal of Biological Chemistry 271, 15160–15165.
| Expression of caveolin-3 in skeletal, cardiac, and smooth muscle cells: caveolin-3 is a component of the sarcolemma and co-fractionates with dystrophin and dystrophin-associated glycoproteins.Crossref | GoogleScholarGoogle Scholar |
Steffens, S, Schrader, AJ, Blasig, H, Vetter, G, Eggers, H, Trankenschuh, W, Kuczyk, MA, and Serth, J (2011). Caveolin 1 protein expression in renal cell carcinoma predicts survival. BMC Urology 11, 25.
| Caveolin 1 protein expression in renal cell carcinoma predicts survival.Crossref | GoogleScholarGoogle Scholar |
Steiner, I, Jung, K, Miller, K, Stephan, C, and Erbersdobler, A (2012). Expression of endothelial factors in prostate cancer: a possible role of caveolin-1 for tumour progression. Oncology Reports 27, 389–395.
| Expression of endothelial factors in prostate cancer: a possible role of caveolin-1 for tumour progression.Crossref | GoogleScholarGoogle Scholar |
Tahir, SA, Ren, C, Timme, TL, Gdor, Y, Hoogeveen, R, Morrisett, JD, Frolov, A, Ayala, G, Wheeler, TM, and Thompson, TC (2003). Development of an immunoassay for serum caveolin-1: a novel biomarker for prostate cancer. Clinical Cancer Research 9, 3653–3659.
Tang, ZL, Scherer, PE, Okamoto, T, Song, K, Chu, C, Kohtz, DS, Nishimoto, I, Lodish, HF, and Lisanti, MP (1996). Molecular cloning of caveolin-3, a novel member of the caveolin gene family expressed predominantly in muscle. Journal of Biological Chemistry 271, 2255–2261.
| Molecular cloning of caveolin-3, a novel member of the caveolin gene family expressed predominantly in muscle.Crossref | GoogleScholarGoogle Scholar |
Tikhomirov, O, and Carpenter, G (2004). Ligand-induced, p38-dependent apoptosis in cells expressing high levels of epidermal growth factor receptor and ErbB-2. Journal of Biological Chemistry 279, 12988–12996.
| Ligand-induced, p38-dependent apoptosis in cells expressing high levels of epidermal growth factor receptor and ErbB-2.Crossref | GoogleScholarGoogle Scholar |
Ting Tse, EY, Fat Ko, FC, Kwan Tung, EK, Chan, LK, Wah Lee, TK, Wai Ngan, ES, Man, K, Tsai Wong, AS, Ng, IO-L, and Ping Yam, JW (2012). Caveolin-1 overexpression is associated with hepatocellular carcinoma tumourigenesis and metastasis. The Journal of Pathology 226, 645–653.
| Caveolin-1 overexpression is associated with hepatocellular carcinoma tumourigenesis and metastasis.Crossref | GoogleScholarGoogle Scholar |
Wang, S, Zhang, C, Liu, Y, Xu, C, and Chen, Z (2014). Functional polymorphisms of caveolin-1 variants as potential biomarkers of esophageal squamous cell carcinoma. Biomarkers 19, 652–659.
| Functional polymorphisms of caveolin-1 variants as potential biomarkers of esophageal squamous cell carcinoma.Crossref | GoogleScholarGoogle Scholar |
Wang, X, Liu, Z, and Yang, Z (2018). Expression and clinical significance of caveolin-1 in prostate cancer after transurethral surgery. BMC Urology 18, 102.
| Expression and clinical significance of caveolin-1 in prostate cancer after transurethral surgery.Crossref | GoogleScholarGoogle Scholar |
Whittington, K, Assinder, S, Gould, M, and Nicholson, H (2004). Oxytocin, oxytocin-associated neurophysin and the oxytocin receptor in the human prostate. Cell and Tissue Research 318, 375–382.
| Oxytocin, oxytocin-associated neurophysin and the oxytocin receptor in the human prostate.Crossref | GoogleScholarGoogle Scholar |
Whittington, K, Connors, B, King, K, Assinder, S, Hogarth, K, and Nicholson, H (2007). The effect of oxytocin on cell proliferation in the human prostate is modulated by gonadal steroids: implications for benign prostatic hyperplasia and carcinoma of the prostate. The Prostate 67, 1132–1142.
| The effect of oxytocin on cell proliferation in the human prostate is modulated by gonadal steroids: implications for benign prostatic hyperplasia and carcinoma of the prostate.Crossref | GoogleScholarGoogle Scholar |
Williams, TM, and Lisanti, MP (2005). Caveolin-1 in oncogenic transformation, cancer, and metastasis. American Journal of Physiology-Cell Physiology 288, C494–C506.
| Caveolin-1 in oncogenic transformation, cancer, and metastasis.Crossref | GoogleScholarGoogle Scholar |
Xu, J, Agyemang, S, Qin, Y, Aysola, K, Giles, M, Oprea, G, O’Regan, RM, Partridge, EE, Harris-Hooker, S, Rice, VM, Reddy, ES, and Rao, VN (2014). A novel pathway that links caveolin-1 down-regulation to BRCA1 dysfunction in serous epithelial ovarian cancer cells. Challenges in Cancer Detection and Therapy 1, 004.
Xu, H, Fu, S, Chen, Q, Gu, M, Zhou, J, Liu, C, Chen, Y, and Wang, Z (2017). The function of oxytocin: a potential biomarker for prostate cancer diagnosis and promoter of prostate cancer. Oncotarget 8, 31215–31226.
| The function of oxytocin: a potential biomarker for prostate cancer diagnosis and promoter of prostate cancer.Crossref | GoogleScholarGoogle Scholar |
Yang, G, Truong, LD, Timme, TL, Ren, C, Wheeler, TM, Park, SH, Nasu, Y, Bangma, CH, Kattan, MW, Scardino, PT, and Thompson, TC (1998). Elevated expression of caveolin is associated with prostate and breast cancer. Clinical Cancer Research 4, 1873–1880.
Yeh, S, Miyamoto, H, Nishimura, K, Kang, H, Ludlow, J, Hsiao, P, Wang, C, Su, C, and Chang, C (1998). Retinoblastoma, a tumor suppressor, is a coactivator for the androgen receptor in human prostate cancer DU145 cells. Biochemical and Biophysical Research Communications 248, 361–367.
| Retinoblastoma, a tumor suppressor, is a coactivator for the androgen receptor in human prostate cancer DU145 cells.Crossref | GoogleScholarGoogle Scholar |
Zenklusen, JC, Thompson, JC, Troncoso, P, Kagan, J, and Conti, CJ (1994). Loss of heterozygosity in human primary prostate carcinomas: a possible tumor suppressor gene at 7q31.1. Cancer Research 54, 6370–6373.