Generation of reproductively mature offspring from the endangered green and golden bell frog Litoria aurea using cryopreserved spermatozoa
Rose Upton

A The Conservation Biology Research Group, School of Environmental and Life Sciences, The University of Newcastle, Callaghan, NSW 2308, Australia.
B FAUNA Research Alliance, PO Box 5092, Kahibah, NSW 2290, Australia.
C Centre for Conservation Ecology and Genomics, Institute for Applied Ecology, University of Canberra, Bruce, ACT 2617, Australia.
D Taronga Institute of Science and Learning, Taronga Conservation Society Australia, Taronga Western Plains Zoo, Dubbo, NSW 2830, Australia.
E San Diego Zoo Global-Beckman Center for Conservation Research, 15600 San Pasqual Valley Road, Escondido, CA 92027, USA.
F School of Mathematical and Physical Sciences, University of Newcastle, Callaghan, NSW 2308, Australia.
G Corresponding author. Email: rose.upton@uon.edu.au
Reproduction, Fertility and Development 33(9) 562-572 https://doi.org/10.1071/RD20296
Submitted: 16 November 2020 Accepted: 25 February 2021 Published: 6 April 2021
Journal Compilation © CSIRO 2021 Open Access CC BY
Abstract
Amphibians are becoming increasingly reliant on captive breeding programs for continued survival. Assisted reproductive technologies including gamete cryopreservation and IVF can help reduce costs of breeding programs, provide insurance against extinction and assist genetic rescue in wild populations. However, the use of these technologies to produce reproductively mature offspring has only been demonstrated in a few non-model species. We aimed to optimise sperm cryopreservation in the threatened frog Litoria aurea and generate mature offspring from frozen–thawed spermatozoa by IVF. We tested three concentrations (1.4, 2.1 and 2.8 M) of the cryoprotectants dimethylsulfoxide (DMSO) and glycerol with 0.3 M sucrose. Using DMSO was more likely to result in recovery of sperm motility, vitality and acrosome integrity than glycerol, regardless of concentration, with forward progressive motility being most sensitive to damage. The lowest concentrations of 1.4 and 2.1 M provided the best protection regardless of cryoprotectant type. Spermatozoa cryopreserved in 2.1 M DMSO outperformed spermatozoa cryopreserved in equivalent concentrations of glycerol in terms of their ability to fertilise ova, resulting in higher rates of embryos hatching and several individuals reaching sexual maturity. We have demonstrated that sperm cryopreservation and subsequent offspring generation via IVF is a feasible conservation tool for L. aurea and other threatened amphibians.
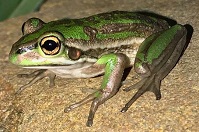
Keywords: anuran, artificial fertilisation, captive survival-assurance colonies, cryoconservation, genome resource bank, green and golden bell frog, Pelodryadidae, spermatozoa.
Introduction
The current reality of global amphibian conservation is that the magnitude of ongoing global declines overshadows the underwhelming financial and logistical resources available to support the number of species requiring intervention (Zippel et al. 2011; Bishop et al. 2012; Della Togna et al. 2020). In extreme cases, some amphibian species extinct in the wild persist only in conservation breeding programs (CBPs), whereas the management of others with extant wild populations relies heavily on ex situ breeding for wild population augmentation (Zippel et al. 2011; Clulow et al. 2014). Current investment in the establishment of CBPs will undoubtedly continue to increase but, unless alternative methods can be incorporated into current management practices, already constrained resources will continue to challenge any hope of recovery (Zippel et al. 2011; Bishop et al. 2012; Della Togna et al. 2020; Howell et al. 2020).
Assisted reproductive technologies (ARTs), including gamete induction through exogenous hormones, sperm cryopreservation and IVF, have the potential to increase the number of species that could be saved (Clulow and Clulow 2016). These technologies increase the potential to breed recalcitrant species, manage genetics more effectively through the storage of founder genomes (genetic rescue) and can circumvent issues relating to mate selection, all while reducing costs of individual programs (Clulow and Clulow 2016; Della Togna et al. 2020; Howell et al. 2020).
Steady progress has occurred in the development of amphibian sperm cryopreservation protocols (Clulow and Clulow 2016) since the earliest reports (Luyet and Hodapp 1938), leading to the development of protocols for approximately 40 species (Browne et al. 2019; Clulow et al. 2019). In addition, it has been demonstrated that live progeny can be obtained using cryopreserved spermatozoa via IVF in the common amphibian laboratory models Xenopus laevis and Silurana tropicalis (Sargent and Mohun 2005; Mansour et al. 2009; Pearl et al. 2017) and a few other non-laboratory species (Shishova et al. 2011; Uteshev et al. 2013; Upton et al. 2018). Although only one published report has demonstrated a full life cycle of progeny produced from amphibian cryopreserved spermatozoa with subsequent generations produced by natural breeding (for X. laevis; Pearl et al. 2017), these successes highlight the importance of ARTs and their application in amphibian conservation and management.
Amphibians have been declining severely since the mid-20th century, with a key driver being the amphibian fungal disease chytridiomycosis (Berger et al. 1998; O’Hanlon et al. 2018; Scheele et al. 2019). The worst wildlife panzootic ever recorded (Bower et al. 2017), chytridiomycosis is thought to be responsible for the decline or extinction of at least 501 species globally (Scheele et al. 2019). Eradication of the pathogen is considered unlikely and there are very few options for mitigating the effect in wild populations (Clulow et al. 2018). Australian amphibians have not escaped the mass declines, with a recent assessment indicating that approximately 46 species (approximately 20% of all Australian frogs) are either extinct or threatened with extinction (Gillespie et al. 2020). Major threats outside of chytridiomycosis include climate change, habitat alteration, invasive species and the increased frequency and intensity of bushfires (Stuart et al. 2004; Gillespie et al. 2020), all of which are difficult to eradicate or contain in the time frame required to avoid further loss of amphibian biodiversity. Thus, there is a major imperative to further develop amphibian ARTs and introduce biobanking as a management tool as a key priority in the fight against extinction, loss of population genetic diversity and further catastrophic loss of biodiversity (Clulow and Clulow 2016; Clulow et al. 2019; Della Togna et al. 2020; Howell et al. 2020).
The green and golden bell frog Litoria aurea is an endangered Australian species that has experienced extirpations across more than 90% of its range over the past few decades (Mahony et al. 2013). Chytridiomycosis is thought to be the key driver behind its demise (Stockwell et al. 2008; Abu Bakar et al. 2016; Campbell et al. 2019), with few management strategies existing for wild populations (but see Clulow et al. 2018), making it a prime candidate species for management through biobanking and ARTs, as well as a model species for other endangered pelodryadids in the bell frog complex.
Herein, we report recovery of live, motile spermatozoa following successful cryopreservation in L. aurea as a model threatened tree frog (Family Pelodryadidae), and the subsequent generation of sexually mature adults by performing IVF with frozen–thawed spermatozoa. Because there are differing reports in the literature on post-thaw sperm recovery across amphibian species (Beesley et al. 1998; Browne et al. 1998, 2002b; Mugnano et al. 1998; Michael and Jones 2004; Mansour et al. 2009, 2010), and few comparing the effects of different cryoprotectants and concentrations on sperm fertilisation and embryo development, we compared different concentrations (1.4, 2.1 and 2.8 M) of two commonly used cryoprotectants, namely dimethylsulfoxide (DMSO) and glycerol. Our aims were to determine which cryoprotectant and concentrations resulted in the highest post-thaw recovery of sperm motility, vitality, acrosome integrity and hatching rates, and, importantly, whether tadpoles derived from cryopreserved spermatozoa were capable of metamorphosing and developing through to sexual maturity.
Materials and methods
Animals
All animals in this study were sourced from the University of Newcastle’s L. aurea captive breeding colony. Animals were housed in vegetated, seminatural outdoor mesocosms (75 cm × 75 cm × 110 cm) containing approximately 25% area terrestrial substrate (gravel) with vegetation, 75% water and stacked brick microrefuges throughout. Animals were supplemented with crickets one to two times per week and exposed to natural day–night light regimes, being able to bask at will. Animals were transferred to the laboratory from the outdoor holding mesocosms on the day experiments were performed.
All captive husbandry and experiments were conducted in accordance with institutional and national Australian standards for the care and welfare of animals, and in accordance with the University of Newcastle’s animal ethics approval (Permit A-2013-328). Animals were originally collected under NSW Scientific Licence SL101269.
Testicular excision and sperm collection
Testes were collected opportunistically for sperm cryopreservation experiments when male L. aurea were killed for a separate study. Five males were killed throughout September and October 2014 by immersion in 0.4% w/v ethyl 3-aminobenzoate methanesulfonate (MS-222; Sigma Aldrich; E10521) buffered with 0.4% w/v sodium bicarbonate, followed by excision of the heart. Both testes were removed from each individual and placed in 1 mL Simplified Amphibian Ringer (SAR; 113 mM NaCl, 1 mM CaCl2, 2 mM KCl, 3.6 mM NaHCO3; recipe from Browne et al. 1998) for maceration and use in cryopreservation or IVF experiments.
Experiment 1: sperm cryopreservation
A sperm suspension for each male (Fig. 1a) was prepared by macerating each pair of testes in the 1 mL holding SAR solution by gently teasing the tissues apart with fine forceps to release the spermatozoa. Two cryoprotectants at three concentrations were tested, namely DMSO and glycerol at concentrations of 1.4, 2.1 and 2.8 M (10%, 15% and 20% v/v), each in combination with 0.3 M sucrose (10% w/v sucrose). A control treatment of 0.3 M sucrose with no additional additives was also tested. Prefreeze vitality and motility for the initial sperm suspension in SAR were assessed before aliquots from each macerate were assigned to the seven treatments for cryopreservation experiments.
![]() |
Cryopreservation trials involved making up one 120-µL aliquot for each treatment from each testicular macerate by extending a 20-µL aliquot of the testicular macerate 1:6 with the appropriate cryoprotectants (initial cryoprotectant concentration as described above). The final concentrations of the cryoprotectants following dilution with sperm suspensions were 1.2, 1.8 and 2.4 M. Prepared aliquots were then loaded into 0.25-mL capacity Cassou straws (IMV Technologies) and sealed with polyvinyl alcohol (PVA) powder (Sigma). Straws were loaded into a programmable freezer (Freeze Control CL-836; CryoLogic) and frozen using a set cooling ramp outlined in Upton et al. (2018). Briefly, this involved straws being held for 10 min at 2.0°C, followed by cooling at a rate of –1.0°C min–1 to –8.0°C, followed by cooling at a rate of –3.0°C min–1 to –16.0°C and then at a rate of –3.4°C min–1 to –80.0°C, at which stage straws were quenched in liquid nitrogen (Upton et al. 2018). Straws were thawed in air early in 2018, approximately 3 years and 4 months later, by placing them on the bench at room temperature (~21°C) for 2 min, before motility, vitality and acrosome integrity were assessed.
Sperm assessment
Spermatozoa were assessed for vitality, motility and acrosome integrity. Sperm vitality (scored as the proportion of cells with intact cell plasma membranes) was assessed with a dye exclusion assay. Eosin-Y (Sigma Aldrich; E4009; 0.4% w/v diluted in 0.9% w/v saline) was mixed 1:1 with sperm samples to form a volume of 10 µL, incubated for at least 30 s at room temperature and then scored under a brightfield microscope at a magnification of ×400. Spermatozoa that stained pink were scored as dead because the cell membrane became permeable to the dye, whereas sperm with a clear cytoplasm, indicating the cells were functioning and able to exclude the dye, were scored as live. Sperm motility was assessed after activation by dilution of the sperm samples 1:6 in distilled water and allowing the samples to sit for at least 2 min for activation to occur. Spermatozoa were scored as being in one of three motility states: non-motile, motile without forward progression or motile with forward progression. For each sample, 100–200 spermatozoa were scored across at least four randomly selected fields of view. One slide was made per straw, with one overall count for each of the motility and vitality assessments.
For assessment of acrosome integrity, 10–20 μL spermatozoa was smeared evenly across a Superfrost slide (ThermoScientific) immediately after thawing; samples were allowed to air dry and were then fixed with 100% methanol. Slides were rehydrated twice in SAR for 5 min before incubation with fluorescein isothiocyanate (FITC)-conjugated Arachis hypogaea (peanut) agglutinin (PNA-FITC; Sigma Aldrich; E10521). PNA-FITC was applied onto the slide at a final concentration of 60 µg mL–1 in SAR and the slides were incubated overnight at 5°C in a darkened humidity chamber. Spermatozoa were counterstained with propidium iodide at a final concentration of 0.5 µM for a maximum of 2 min. Slides were rinsed with SAR for 5 min and examined under a Motic BA310E microscope equipped with an epifluorescence device. Spermatozoa with a fluorescently stained green tip and red head were counted as having an intact acrosome and spermatozoa with only a red stained head were counted as not (Fig. 1b). At least 100 spermatozoa were counted across four fields of view. Two slide replicates per straw with two counts of each were made.
Experiment 2: IVF trials
IVF experiments were conducted in 2018 to test the fertilising capacity of spermatozoa cryopreserved in 2014. IVF was performed with spermatozoa exposed to 2.1 M DMSO and glycerol (one of the determined optimal concentrations for obtaining live, motile spermatozoa) in two ways: (1) fresh, unfrozen spermatozoa from new males but exposed to cryoprotectants in the same manner as cryopreserved spermatozoa to test for cryoprotectant toxicity without freezing; and (2) frozen–thawed spermatozoa to test the effects of the full cryopreservation process. Fresh, unfrozen sperm samples were derived from two males killed in 2018 on the same day that IVF was performed (one for each of 2 days an IVF was performed). Two additional control treatments were added to demonstrate that the quality of the ova was not a factor in any low fertility rates observed from unfrozen and frozen sperm treatments. These included: (1) fresh spermatozoa without any cryoprotectants added, used to demonstrate ova quality in the absence of any other factors; and (2) ‘fertilisations’ with SAR containing no spermatozoa to control for possible parthenogenic activation of ova.
Ova were obtained from four females by monitoring amplecting pairs from the captive breeding colony until first signs of oviposition began, at which point the females were separated from the males and moved to a cool room (~18°C) to delay oviposition until IVF experiments were ready to be performed. The females were held individually in containers containing SAR and were allowed to warm to room temperature before the beginning of IVF. When experiments were ready to start, ova were expelled from the cloaca into Petri dishes via gentle massage of the abdomen, after which spermatozoa were immediately added to the dishes. IVF experiments were conducted over 2 days on 30 January and 1 February 2018. On Day 1, a subset of ova (n = 11 ± 1 per dish; n = 3 dishes per treatment, per animal) from Females 1–3 was fertilised with fresh spermatozoa from one freshly killed male and with cryopreserved spermatozoa from one male cryopreserved in 2014. On Day 2, a subset of ova (n = 11 ± 1 per dish; n = 6 dishes per treatment, per animal) from the fourth female was fertilised with spermatozoa from a second freshly killed male and with cryopreserved spermatozoa from a second male cryopreserved in 2014.
Sperm preparation and IVF
Fresh and thawed spermatozoa exposed to cryoprotectants were washed by first spinning down the spermatozoa in a centrifuge at 2000g for 5 min at 21°C to remove the cryoprotectants before the spermatozoa were resuspended in SAR. The sperm concentration was made to be roughly equal between samples (~1 × 106 cells mL–1; mean (±s.d.) 1.04 × 106 ± 3.29 × 104; determined to be sufficient for maximum fertilisation; Browne et al. 1998). This was achieved by first measuring sperm concentration using a Neubauer haemocytometer and diluting spermatozoa with SAR so that the final concentration following activation would be equal in each treatment. A 130-µL aliquot of activated sperm solution was then pipetted directly onto the ova in each treatment dish.
Successful fertilisations were confirmed by rotation of the animal poles (as indicated by the dark hemisphere of the ova facing up), which typically occurred within 30–60 min after the addition of spermatozoa. At this point, the dishes were flooded with 10% v/v SAR. The proportion of developing embryos subsequently reaching various developmental stages (Gosner 1960) was recorded, including Gosner Stage 3 (first cleavage), Stage 4 (second cleavage), Stages 7–9 (blastula), Stages 10–12 (gastrula), Stages 13–17 (neurula), Stages 17–19 (organogenesis) and Stage 20 (hatched tadpole). Hatched tadpoles were transferred to plastic terraria (30 cm × 18 cm × 24 cm; grouped and pooled by treatment) and maintained at room temperature on a 13-h : 11-h dark–light cycle, reflecting natural day length at the time of fertilisation. After 1 month of development, tadpoles were transferred into outdoor mesocosms. Tadpoles were fed ad libitum a mixture of spirulina (100% Organic Super Greens; Synergy Natural Products) and ground trout pellet (Ridley Aquafeed; Ridley AgriProducts), in a ratio of 50:50, once a week. Due to logistical restraints, tadpoles were pooled by treatment and no further monitoring or analysis was conducted other than to assess whether frogs that metamorphosed were able to reach sexual maturity.
Assessment of sexual maturation
Animals that survived to adulthood were checked for signs of sexual maturity, as indicated by the appearance of secondary sexual characteristics. These included darkened nuptial pads and vocalisation for males and increased abdominal size and the presence of mature ovarian follicles in females. The presence of mature follicles in the females was detected via ultrasound using a LOGIQ e ultrasound (GE Healthcare) equipped with an L8-18i-rs linear hockey stick probe (range 6.7–18 MHz) set to musculoskeletal setting at a scan depth of 2 cm. The probe was wetted with aged tap water as a conductor and was held gently against the abdomen for imaging.
Statistical analysis
Sperm cryopreservation data
Generalised linear mixed models (GLMM) were used to fit binary logistic regressions to four sperm parameters: vitality, acrosome integrity (interpreted as the proportion of successful cases; i.e. live spermatozoa or intact acrosome with the total number of spermatozoa used as weights in the model) and two motility parameters (forward-progressive and non-progressive motility; interpreted as proportions, with the weights equalling non-motile spermatozoa plus the number of cases of each respective category). The first model implemented a two-factor design using multiple categories and levels: Factor 1, cryoprotectant type (DMSO or glycerol); and Factor 2, cryoprotectant concentration (1.4, 2.1 and 2.8 M). Interactions between cryoprotectant type and concentration were tested and not found to be significant. Thus, all models were simplified by removing the interaction. This allowed the comparison of cryoprotectant type, averaged across cryoprotectant concentration, and vice versa. In the second model (containing treatments and controls), a separate logistic regression model was generated with all eight treatments treated as a single categorical variable. This allowed comparison of treatments to the fresh control (unfrozen, no cryoprotectants) and the cryopreserved sperm control containing 0.3 M sucrose only, without cryoprotectants. Overdispersion was addressed for all models in this experiment using an observation-level random effect.
IVF data
A logistic regression was fitted to each of the eight Gosner developmental stages assessed in the IVF trial using a GLMM with a fixed effect variable for the five treatments and nested random effects (individual female ID, treatment within each female and each replicate within each treatment). In a separate model, the eight developmental stages were added as an additional fixed effect along with a random effect in which development stage was nested within replicates. The second model was the basis for testing the interaction between development stage and treatment. Due to computational problems when the cryopreserved 2.1 M glycerol treatment was included (because all counts were zero in the last four stages of development), data for that treatment were excluded when testing the significance of the interaction between development stage and treatment.
The glmer program in the R version 3.6.2 (R Foundation for Statistical Computing) package lme4 (version 1.1-23) was used for all GLMM modelling in this study (Bates et al. 2015). Model estimated marginal means (EMMs) and 95% confidence limits were determined for each condition and back-transformed to proportions. Odds ratios (ORs) comparing treatments were also generated using the package emmeans (version 1.4.8; Lenth et al. 2018).
Results
Experiment 1: recovery of spermatozoa after cryopreservation
Effects of cryoprotectant type and concentration on sperm motility, vitality and acrosome integrity
There were significant effects of cryoprotectant type and concentration on both post-thaw forward progressive motility (likelihood ratio test (LRT) χ12 = 27.0 (P < 0.001) and χ22 = 13.0 (P < 0.01) respectively) and acrosome integrity (LRT χ12 = 3.7 (P = 0.05) and χ22 = 6.9 (P < 0.05) respectively). In addition, there were significant effects of cryoprotectant type on non-progressive motility (LRT χ22 = 18.3, P < 0.001) and vitality (LRT χ22 = 10.5, P < 0.001). There was no significant effect of cryoprotectant concentration on either non-progressive motility or vitality. In all cases, DMSO resulted in greater post-thaw motility and vitality than glycerol, with mixed results for the optimal concentrations of each.
ORs were used to further compare results between treatments (Supplementary Table S1). When results were averaged across cryoprotectant concentration, DMSO was almost sevenfold more likely than glycerol to have a positive effect on forward progressive motility (OR 6.7; 95% confidence interval (CI) 3.7–12.0; Table S1) and threefold more likely than glycerol to have a positive effect on non-progressive motility (OR 3.2, 95% CI 2.0–5.0; Table S1). DMSO was also twice as likely to have a positive effect on vitality than glycerol (OR 2.0, 95% CI 1.37–3.0) and 1.2-fold more likely to have a positive effect on acrosome integrity (OR 1.2, 95% CI 1.0–1.5). When results were averaged across cryoprotectant type, there were no significant differences between cryoprotectant concentrations on non-progressive motility or vitality, but the 1.4 and 2.1 M concentrations were four- and threefold more likely respectively to have a positive effect on forward progressive motility than the concentration of 2.8 M (Table S1). The concentration of 1.4 M was 1.4-fold more likely to have a positive effect on acrosome integrity than the concentration of 2.8 M (OR 1.4, 95% CI 1.1–1.8). For the full set of ORs and confidence limits, see Table S1.
Post-thaw recovery in cryopreservation treatments versus fresh, unfrozen spermatozoa
There was a significant effect of cryoprotectant treatment on forward progressive motility, non-progressive motility, vitality and acrosome integrity (LRT χ72 = 65.5 (P < 0.001), χ72 = 55.0 (P < 0.001), χ72 = 91.8 (P < 0.001) and χ62 = 13.7 (P = 0.03) respectively). ORs were generated to further compare individual treatments to the two control groups (fresh sperm control with no cryoprotectants added and 0.3M sucrose only; Tables S2, S3). Fresh spermatozoa had significantly higher forward and non-progressive motility and vitality than any of the cryopreserved treatments (Fig. 2). Compared with the best cryoprotectant (2.1 M DMSO), fresh spermatozoa were almost ninefold more likely to have forward progressive motility (OR 8.7, 95% CI 3.4–22.3) and 60-fold more likely to have intact membranes (OR 60.4, 95% CI 24.5–148.7).
![]() |
Of the cryoprotectant treatments, 2.1 M DMSO performed significantly better than all other cryoprotectants at recovering spermatozoa with forward progressive motility, apart from 1.4 M DMSO, with little difference between 1.4 and 2.1 M DMSO (OR 1.4, 95% CI 0.6–3.3; Table S2; Fig. 2). There was a significant reduction in forward progressive motility for spermatozoa cryopreserved in 2.8 M DMSO compared with other DMSO treatments and the fresh control (Fig. 2; for ORs, see Table S2). There was a significant reduction in all motility parameters and vitality for spermatozoa cryopreserved with any concentration of glycerol compared with 1.4 and 2.1 M DMSO treatments. Forward progressive motility was preserved better in the 0.3 M sucrose only control than in the 2.1 and 2.8 M glycerol treatments (Fig. 2; for ORs, see Table S2).
The best for post-thaw preservation of acrosome integrity was seen with 1.4 M DMSO (Fig. 3). Although there was no significant difference in the preservation of acrosome integrity between 1.4 and 2.1 M DMSO, compared with 2.8 M DMSO, 1.4 M DMSO was 1.4-fold more likely to result in intact acrosomes (OR 1.4, 95% CI 1.0–1.19). The preservation of acrosome integrity was also significantly better with 1.4 M DMSO than both 2.1 and 2.8 M glycerol, as well as 0.3 M sucrose (Table S3). The difference between DMSO and glycerol treatments was less pronounced for acrosome integrity (Fig. 3).
![]() |
Experiment 2: IVF using frozen–thawed spermatozoa
Effects of cryoprotectant type on embryo survival
There was a significant effect of treatment on embryo survival at each of the eight Gosner developmental stages monitored, with LRT χ42 ranging from 24.7 to 35.7 (P < 0.001). In all cases, fertilisation with fresh, unfrozen spermatozoa yielded a higher proportion of surviving embryos, with fertilisation with spermatozoa cryopreserved in 2.1 M DMSO being the only cryopreserved treatment to result in embryo survival beyond blastulation. ORs were used to further compare results between treatments (Table S4).
The models showed that fresh, unfrozen spermatozoa produced a higher proportion of embryos surviving to first cleavage (EMM 86%) than DMSO-treated cryopreserved spermatozoa (EMM 66%), although the difference did not reach statistical significance (Fig. 4a; Table S4). There was no significant difference in the proportion of surviving embryos produced from fresh unfrozen spermatozoa compared with DMSO-treated, unfrozen spermatozoa at any stage of development, except Stages 17–19 (organogenesis; Tables S4–S10). Compared with the proportion of surviving embryos produced from fresh, unfrozen spermatozoa, all other treatments had a significantly reduced embryo survival (Tables S4–S11).
![]() |
Embryos produced with unfrozen spermatozoa treated with 2.1 M DMSO were 15-fold more likely to survive to first cleavage than those produced with unfrozen spermatozoa treated with 2.1 M glycerol (OR 15.3, 95% CI 3.7–63.3) and 14-fold more likely to survive to first cleavage than their counterparts produced using cryopreserved spermatozoa (2.1 M DMSO; OR 13.7, 95% CI 3.3–55.6). The proportion of surviving embryos was significantly higher for those produced with unfrozen spermatozoa treated with 2.1 M DMSO than for those produced with unfrozen spermatozoa treated with 2.1 M glycerol and those produced with cryopreserved spermatozoa treated with 2.1 M DMSO at each stage of development (Fig. 4; for ORs, see Tables S4–S11).
The proportion of surviving embryos was similar between those produced with cryopreserved spermatozoa treated with 2.1 M DMSO and those produced by cryopreserved spermatozoa treated with 2.1 M glycerol until blastula development, at which stage the proportion of surviving embryos was 8.5-fold higher for those produced by cryopreserved spermatozoa treated with 2.1 M DMSO than those produced by cryopreserved spermatozoa treated with 2.1 M glycerol (Fig. 4b; OR 8.5, 95% CI 1.1–65.3). The differences in the proportions of embryos surviving remained significant for the remaining stages of development, with no embryos produced from cryopreserved spermatozoa treated with 2.1 M glycerol surviving beyond gastrulation (Tables S8–11).
More embryos survived to hatching from fresh, unfrozen spermatozoa than any other treatment (EMM 43%). Fertilisations with unfrozen, 2.1 M DMSO-treated spermatozoa resulted in more embryos hatching than fertilisations with cryopreserved 2.1 M DMSO-treated spermatozoa (EMM 30%). The proportion of embryos produced from cryopreserved spermatozoa treated with 2.1 M DMSO surviving to hatching was 2% (Fig. 4c).
Progression of embryos through embryogenesis and metamorphosis to reproductive maturity
There was no difference in the rate of progression from fertilisation to hatching between any treatment (LRT χ242 = 33.4, P = 0.10), with development from first cleavage to hatching taking approximately 3 days (Fig. 4a–c).
Metamorphosis began to occur in two of the treatment groups (unfrozen DMSO and unfrozen glycerol) at 70 days after fertilisation (April 2018), with metamorphosis beginning in the remaining treatments (fresh and cryopreserved with DMSO) only 2 days later (Fig. 5). As of February 2019, all remaining tadpoles had metamorphosed, some after overwintering.
![]() |
In 2019, surviving males from all treatment groups developed nuptial pads and began to vocalise, thus displaying signs of sexual maturity (Fig. 6a). In 2020, females from all treatment groups developed swollen abdomens as a sign of developing gravidity (females typically take a year longer to reach sexual maturity). Sonograms confirmed the presence of follicles in females from all treatments (Fig. 6b).
![]() |
Discussion
This study adds to the growing number of reports of successful sperm cryopreservation in amphibians (Browne et al. 2019; Clulow et al. 2019). Importantly, we demonstrated successful generation of sexually mature L. aurea adults from cryopreserved spermatozoa. Most reports on the recovery of spermatozoa following cryopreservation stop at the assessment of the motility and vitality of the spermatozoa after thawing. There are far fewer reports of the viability of cryopreserved spermatozoa assessed by fertilisation trials (Mansour et al. 2009; Shishova et al. 2011; Langhorne et al. 2013; Upton et al. 2018), which is an important goal in the cryobiology of gametes as the ultimate measure of success (Clulow and Clulow 2016). By achieving successful sperm cryopreservation in L. aurea and subsequent fertilisations via IVF that resulted in sexually mature frogs, we provide another proof-of-concept example of the validity and utility of ARTs as an important conservation strategy for imperilled amphibians.
The successful production of live progeny from cryopreserved spermatozoa in a threatened amphibian illustrates the legitimacy of biobanking as a management tool and helps address the recommendation of the Amphibian Conservation Action Plan (Della Togna et al. 2020). Despite no female gametes or embryos having been successfully cryopreserved in amphibians to date (but see Lawson et al. (2013) for successful cryopreservation of amphibian embryonic cells), the technologies are developing rapidly in fish and corals and will likely complement amphibian biobanking of male gametes in years to come (Khosla et al. 2017; Daly et al. 2018).
The range of penetrating cryoprotectants tested in the present study (DMSO and glycerol; 1.4, 2.1 and 2.8 M) was similar to that used in other amphibians in the literature. As with a range of other studies (Beesley et al. 1998; Browne et al. 1998, 2002b; Mugnano et al. 1998; Mansour et al. 2009), DMSO resulted in higher sperm recovery after cryopreservation than glycerol, which appears to be more toxic than DMSO and shows a strong concentration effect on the most sensitive sperm recovery parameter of forward progressive motility (Fig. 2a). Nevertheless, there are some amphibian studies that do report better recovery with glycerol (Michael and Jones 2004; Mansour et al. 2010) and some that report good post-thaw recovery and fertilisation using N,N-dimethylformamide as a cryoprotectant (Shishova et al. 2011; Hinkson et al. 2019).
With the exception of forward progressive motility and acrosome integrity, this study showed little effect of cryoprotectant concentrations on sperm quality (Figs 2, 3). No effect of cryoprotectant concentration was observed on proportion of live spermatozoa (Fig. 2), a similar response to that found by Mugnano et al. (1998) on Lithobates sylvaticus using 1.5 and 3.0 M DMSO and glycerol. Interestingly, some studies found no difference in the proportion of intact cells versus motile cells recovered after cryopreservation (Mugnano et al. 1998; Mansour et al. 2009). In the present study, we found a reduction in motility compared with vitality, indicating that non-lethal intracellular damage may be occurring as a result of sperm cryopreservation in L. aurea.
We found that lower concentrations of DMSO (1.4 or 2.1 M) resulted in higher recovery of spermatozoa after cryopreservation. This suggests that 2.8 M DMSO, along with 2.1 and 2.8 M glycerol, was cytotoxic, as reflected by the negative effects on sperm forward progressive motility following cryopreservation (Fig. 2). Mansour et al. (2009) found that lower concentrations of DMSO in the range of 0.7 M were optimal for sperm cryopreservation in X. laevis compared with a range of concentrations of other cryoprotectants, including methanol and glycerol, and reported a cytotoxic effect at 4°C using 0.7 M glycerol that affected vitality and motility.
Our results indicate that for L. aurea, and perhaps other pelodryadid tree frogs, optimisation of cryoprotectant media should be focused on using DMSO as the primary cryoprotectant. Several studies report that DMSO at concentrations ranging from 1.4 to 2.1 M, with or without sucrose, is useful for cryopreservation in X. laevis (Mansour et al. 2009), Rhinella marina (Browne et al. 1998, 2002a, 2002c, 2002d; Proaño and Pérez 2017), L. sylvaticus (Mugnano et al. 1998), and several pelodryadid and myobatrachid species (Browne et al. 2002b; Upton et al. 2018).
We are unaware of any studies that have tested the effects of cryoprotectants on the preservation of acrosome integrity in amphibian spermatozoa, but the results of the present study indicate that although overall the acrosome may be more resistant to damage than other structures that facilitate motility, lower concentrations (1.4 or 2.1 M) of DMSO are optimal for preserving the acrosome (Fig. 3). Acrosomal integrity assays, which have been used to assess the fertilisation capabilities of fresh amphibian spermatozoa (Takamune 1987; Ueda et al. 2002), could be used as a tool to assess cryoprotectants when ova are not available for fertilisation trials. However, the present study showed little difference in acrosome integrity across treatments (Fig. 3) and that acrosome integrity was not a good predictor of fertilisation.
Our IVF trials indicated that successful hatching of embryos fertilised with cryopreserved spermatozoa was dependent on cryoprotectant type. Fresh spermatozoa mixed with glycerol or spermatozoa cryopreserved in glycerol both fertilised fewer ova than fresh DMSO-treated spermatozoa (Fig. 4). Similar cleavage rates observed between fresh spermatozoa with glycerol and glycerol-treated cryopreserved spermatozoa indicate that the cellular damage resulting in reduced cleavage was likely related to the glycerol itself and not exacerbated by the process of cryopreservation. In contrast, results from the DMSO treatment groups indicated that lower cleavage rates were predominately caused by damage during cryopreservation, because fresh spermatozoa mixed with DMSO resulted in fertilisation rates comparable to those for the fresh, untreated control groups.
A small number of other studies provide comparative data on fertilisation and development rates in amphibians using cryopreserved spermatozoa. Mansour et al. (2009) reported X. laevis hatch rates of approximately 68% in unfrozen sperm controls and variable (but generally low) hatch rates in frozen–thawed sperm treatments, the highest of which was approximately 48%, but typically ranged from 5% to 28%. A 2010 study in Rana temporaria found fertility was low in unfrozen sperm controls (~34% fertilised) and that the fertilisation rate from cryopreserved spermatozoa was approximately 23% (Mansour et al. 2010). Our best result in L. aurea using cryopreserved spermatozoa was a fertilisation rate of 12.7% using spermatozoa cryopreserved in 2.1 M DMSO. Although this is lower than values reported in other studies, the effect is unlikely to be due solely to the cryopreservation protocol. Our data indicated the rate of attrition over the period of embryogenesis was similar across all treatments after lower fertilisation rates associated with cryopreservation treatments were taken into account. This suggests that the processes of IVF and subsequent raising of embryos likely played a role in the attrition rate. This outcome is supported by data from other studies. Different fertilisation rates from similar IVF protocols applied to two different species (X. laevis and R. temporaria) indicated species- or genus-level effects associated with IVF processes (Mansour et al. 2009, 2010).
Future studies would benefit from further considering the mechanics and developmental implications of cryoinjury. For example, DNA damage may translate into developmental and fitness effects in adult offspring (Morrow et al. 2017) and L. aurea may be a good model to investigate this. Nevertheless, the present study has produced mature adults of a threatened amphibian, capable of exhibiting natural breeding behaviours, by using both fresh and cryopreserved spermatozoa to fertilise fresh ova via IVF. Future studies on the reproductive viability and evaluation of the fitness of F1 progeny and beyond produced from cryopreserved spermatozoa are still needed. However, this study is a valuable step towards incorporating ARTs as a management tool in threatened amphibian captive breeding programs. Current recommendations for genetic rescue of species that are in danger or at risk of extinction call for the maximisation of genetic diversity from a carefully selected source population (Ralls et al. 2020). Biobanking can provide long-term storage and logistically viable strategies (Howell et al. 2020) while implementing careful management procedures that assist in curtailing the inevitable loss of genetic diversity that is currently playing an increasing role in the extinction of amphibians.
Conflicts of interest
Simon Clulow, Natalie Calatayud and John Clulow are guest Associate Editors. Despite this relationship, they did not at any stage have editor-level access to this manuscript while in peer review, as is the standard practice when handling manuscripts submitted by an editor of this Journal. The authors have no further conflicts of interest to declare.
Acknowledgements
Rose Upton was supported financially by an Australian Government Research Training Program scholarship. The authors thank Kaya Klop-Toker and Agnes Kovacs for animal husbandry; Darcie Brett, John Gould and Cassandra Bugir for photographs; Barbara Fraser for assistance with the acrosome staining protocol; and Justine O’Brien and Rebecca Hobbs for the provision of laboratory space and equipment. We would also like to acknowledge and respect the Pambalong people of the Awabakal Nation, the traditional custodians of the land on which we work, and pay respect to the Elders past, present and future.
References
Abu Bakar, A., Bower, D. S., Stockwell, M. P., Clulow, S., Clulow, J., and Mahony, M. J. (2016). Susceptibility to disease varies with ontogeny and immunocompetence in a threatened amphibian. Oecologia 181, 997–1009.| Susceptibility to disease varies with ontogeny and immunocompetence in a threatened amphibian.Crossref | GoogleScholarGoogle Scholar | 27021312PubMed |
Bates, D., Mächler, M., Bolker, B., and Walker, S. (2015). Fitting Linear Mixed-Effects Models Using lme4. J. Stat. Softw. 67, 1–48.
| Fitting Linear Mixed-Effects Models Using lme4.Crossref | GoogleScholarGoogle Scholar |
Beesley, S. G., Costanzo, J. P., and Lee, R. E. (1998). Cryopreservation of spermatozoa from freeze-tolerant and -intolerant anurans. Cryobiology 37, 155–162.
| Cryopreservation of spermatozoa from freeze-tolerant and -intolerant anurans.Crossref | GoogleScholarGoogle Scholar | 9769166PubMed |
Berger, L., Speare, R., Daszak, P., Green, D. E., Cunningham, A. A., Goggin, C. L., Slocombe, R., Ragan, M. A., Hyatt, A. D., McDonald, K. R., Hines, H. B., Lips, K. R., Marantelli, G., and Parkes, H. (1998). Chytridiomycosis causes amphibian mortality associated with population declines in the rain forests of Australia and Central America. Proc. Natl Acad. Sci. USA 95, 9031–9036.
| Chytridiomycosis causes amphibian mortality associated with population declines in the rain forests of Australia and Central America.Crossref | GoogleScholarGoogle Scholar | 9671799PubMed |
Bishop, P. J., Angulo, A., Lewis, J. P., Moore, R. D., Rabb, G. B., and Moreno, J. G. (2012). The Amphibian Extinction Crisis: What will it take to put the action into the Amphibian Conservation Action Plan? S.A.P.I.EN.S 5, 97–111.
Bower, D. S., Lips, K. R., Schwarzkopf, L., Georges, A., and Clulow, S. (2017). Amphibians on the brink. Science 357, 454.
| Amphibians on the brink.Crossref | GoogleScholarGoogle Scholar | 28774916PubMed |
Browne, R. K., Clulow, J., Mahony, M. J., and Clark, A. K. (1998). Successful recovery of motility and fertility of cryopreserved cane toad (Bufo marinus) sperm. Cryobiology 37, 339–345.
| Successful recovery of motility and fertility of cryopreserved cane toad (Bufo marinus) sperm.Crossref | GoogleScholarGoogle Scholar | 9917350PubMed |
Browne, R. K., Clulow, J., and Mahony, M. J. (2002a). The effect of saccharides on the post-thaw recovery of cane toad (Bufo marinus) spermatozoa. Cryo Letters 23, 121–128.
| 12050780PubMed |
Browne, R. K., Clulow, J., and Mahony, M. J. (2002b). The short-term storage and cryopreservation of spermatozoa from hylid and myobatrachid frogs. Cryo Letters 23, 129–136.
| 12050781PubMed |
Browne, R. K., Davis, J., Clulow, J., and Pomering, M. (2002c). Storage of cane toad Bufo marinus sperm for 6 days at 0°C with subsequent cryopreservation. Reprod. Fertil. Dev. 14, 267–273.
| Storage of cane toad Bufo marinus sperm for 6 days at 0°C with subsequent cryopreservation.Crossref | GoogleScholarGoogle Scholar | 12467350PubMed |
Browne, R. K., Mahony, M. J., and Clulow, J. (2002d). A comparison of sucrose, saline, and saline with egg-yolk diluents on the cryopreservation of cane toad (Bufo marinus) sperm. Cryobiology 44, 251–257.
| A comparison of sucrose, saline, and saline with egg-yolk diluents on the cryopreservation of cane toad (Bufo marinus) sperm.Crossref | GoogleScholarGoogle Scholar | 12237090PubMed |
Browne, R. K., Silla, A. J., Upton, R., Della-Togna, G., Marcec-Greaves, R., Shishova, N. V., Uteshev, V. K., Proano, B., Perez, O. D., Mansour, N., Kaurova, S. A., Gakhova, E. N., Cosson, J., Dyzuba, B., Kramarova, L. I., McGinnity, D., Gonzalez, M., Clulow, J., and Clulow, S. (2019). Sperm collection and storage for the sustainable management of amphibian biodiversity. Theriogenology 133, 187–200.
| Sperm collection and storage for the sustainable management of amphibian biodiversity.Crossref | GoogleScholarGoogle Scholar | 31155034PubMed |
Campbell, L., Bower, D. S., Clulow, S., Stockwell, M., Clulow, J., and Mahony, M. (2019). Interaction between temperature and sublethal infection with the amphibian chytrid fungus impacts a susceptible frog species. Sci. Rep. 9, 83.
| Interaction between temperature and sublethal infection with the amphibian chytrid fungus impacts a susceptible frog species.Crossref | GoogleScholarGoogle Scholar | 30643160PubMed |
Clulow, J., and Clulow, S. (2016). Cryopreservation and other assisted reproductive technologies for the conservation of threatened amphibians and reptiles: Bringing the ARTs up to speed. Reprod. Fertil. Dev. 28, 1116–1132.
| Cryopreservation and other assisted reproductive technologies for the conservation of threatened amphibians and reptiles: Bringing the ARTs up to speed.Crossref | GoogleScholarGoogle Scholar |
Clulow, J., Trudeau, V. L., and Kouba, A. J. (2014). Amphibian declines in the twenty-first century: Why we need assisted reproductive technologies. Adv. Exp. Med. Biol. 753, 275–316.
| Amphibian declines in the twenty-first century: Why we need assisted reproductive technologies.Crossref | GoogleScholarGoogle Scholar | 25091914PubMed |
Clulow, S., Gould, J., James, H., Stockwell, M., Clulow, J., and Mahony, M. (2018). Elevated salinity blocks pathogen transmission and improves host survival from the global amphibian chytrid pandemic: Implications for translocations. J. Appl. Ecol. 55, 830–840.
| Elevated salinity blocks pathogen transmission and improves host survival from the global amphibian chytrid pandemic: Implications for translocations.Crossref | GoogleScholarGoogle Scholar |
Clulow, J., Upton, R., Trudeau, V. L., and Clulow, S. (2019). Amphibian assisted reproductive technologies: moving from technology to application. In ‘Reproductive Sciences in Animal Conservation’. (Eds P. Comizzoli, J. L. Brown, and W. V. Holt) pp. 413–463. (Springer International Publishing: Cham.)
Daly, J., Zuchowicz, N., Nuñez Lendo, C. I., Khosla, K., Lager, C., Henley, M., Bischof, J., Kleinhans, F. W., Lin, C., Peters, E., and Hagedorn, M. (2018). Successful cryopreservation of coral larvae using vitrification and laser warming. Cryobiology 85, 161–162.
| Successful cryopreservation of coral larvae using vitrification and laser warming.Crossref | GoogleScholarGoogle Scholar |
Della Togna, G., Howell, L. G., Clulow, J., Langhorne, C. J., Marcec-Greaves, R., and Calatayud, N. E. (2020). Evaluating amphibian biobanking and reproduction for captive breeding programs according to the Amphibian Conservation Action Plan objectives. Theriogenology 150, 412–431.
| Evaluating amphibian biobanking and reproduction for captive breeding programs according to the Amphibian Conservation Action Plan objectives.Crossref | GoogleScholarGoogle Scholar | 32127175PubMed |
Gillespie, G. R., Roberts, J. D., Hunter, D., Hoskin, C. J., Alford, R. A., Heard, G. W., Hines, H., Lemckert, F., Newell, D., and Scheele, B. C. (2020). Status and priority conservation actions for Australian frog species. Biol. Conserv. 247, 108543.
| Status and priority conservation actions for Australian frog species.Crossref | GoogleScholarGoogle Scholar |
Gosner, K. L. (1960). A Simplified Table for Staging Anuran Embryos and Larvae with Notes on Identification. Herpetologica 16, 183–190.
Hinkson, K. M., Baecher, J. A., and Poo, S. (2019). Cryopreservation and hormonal induction of spermic urine in a novel species: The smooth-sided toad (Rhaebo guttatus). Cryobiology 89, 109–111.
| Cryopreservation and hormonal induction of spermic urine in a novel species: The smooth-sided toad (Rhaebo guttatus).Crossref | GoogleScholarGoogle Scholar | 31078579PubMed |
Howell, L. G., Frankham, R., Rodger, J. C., Witt, R. R., Clulow, S., Upton, R. M. O., and Clulow, J. (2020). Integrating biobanking minimises inbreeding and produces significant cost benefits for a threatened frog captive breeding programme. Conserv. Lett. , .
| Integrating biobanking minimises inbreeding and produces significant cost benefits for a threatened frog captive breeding programme.Crossref | GoogleScholarGoogle Scholar |
Khosla, K., Wang, Y., Hagedorn, M., Qin, Z., and Bischof, J. (2017). Gold Nanorod Induced Warming of Embryos from the Cryogenic State Enhances Viability. ACS Nano 11, 7869–7878.
| Gold Nanorod Induced Warming of Embryos from the Cryogenic State Enhances Viability.Crossref | GoogleScholarGoogle Scholar | 28702993PubMed |
Langhorne, C. J., Calatayud, N. E., Kouba, A. J., Feugang, J. M., Vance, C. K., and Willard, S. T. (2013). 026 Cryoconservation: successful sperm cryopreservation and develop-mental outcomes using endangered North American amphibians. Cryobiology 67, 405.
| 026 Cryoconservation: successful sperm cryopreservation and develop-mental outcomes using endangered North American amphibians.Crossref | GoogleScholarGoogle Scholar |
Lawson, B., Clulow, S., Mahony, M. J., and Clulow, J. (2013). Towards gene banking amphibian maternal germ lines: Short-term incubation, cryoprotectant tolerance and cryopreservation of embryonic cells of the frog, Limnodynastes peronii. PLoS One 8, e60760.
| Towards gene banking amphibian maternal germ lines: Short-term incubation, cryoprotectant tolerance and cryopreservation of embryonic cells of the frog, Limnodynastes peronii.Crossref | GoogleScholarGoogle Scholar | 23577155PubMed |
Lenth, R., Singmann, H., Love, J., Buerkner, P., and Herve, M. (2018) Emmeans: estimated marginal means, aka least-squares means. In ‘R package version 1.2.2’. Available at https://CRAN.R-project.org/package=emmeans [verified 10 March 2021].
Luyet, B. J., and Hodapp, E. L. (1938). Revival of Frog’s Spermatozoa Vitrified in Liquid Air. Proc. Soc. Exp. Biol. Med. 39, 433–434.
| Revival of Frog’s Spermatozoa Vitrified in Liquid Air.Crossref | GoogleScholarGoogle Scholar |
Mahony, M. J., Hamer, A. J., Pickett, E. J., McKenzie, D. J., Stockwell, M. P., Garnham, J. I., Keely, C. C., Deboo, M. L., O’Meara, J., and Pollard, C. J. (2013). Identifying conservation and research priorities in the face of uncertainty: a review of the threatened bell frog complex in eastern Australia. Herpetol. Conserv. Biol. 8, 519–538.
Mansour, N., Lahnsteiner, F., and Patzner, R. A. (2009). Optimization of the cryopreservation of African clawed frog (Xenopus laevis) sperm. Theriogenology 72, 1221–1228.
| Optimization of the cryopreservation of African clawed frog (Xenopus laevis) sperm.Crossref | GoogleScholarGoogle Scholar | 19766299PubMed |
Mansour, N., Lahnsteiner, F., and Patzner, R. A. (2010). Motility and cryopreservation of spermatozoa of European common frog, Rana temporaria. Theriogenology 74, 724–732.
| Motility and cryopreservation of spermatozoa of European common frog, Rana temporaria.Crossref | GoogleScholarGoogle Scholar | 20537698PubMed |
Michael, S. F., and Jones, C. (2004). Cryopreservation of spermatozoa of the terrestrial Puerto Rican frog, Eleutherodactylus coqui. Cryobiology 48, 90–94.
| Cryopreservation of spermatozoa of the terrestrial Puerto Rican frog, Eleutherodactylus coqui.Crossref | GoogleScholarGoogle Scholar | 14969686PubMed |
Morrow, S., Gosálvez, J., López-Fernández, C., Arroyo, F., Holt, W. V., and Guille, M. J. (2017). Effects of freezing and activation on membrane quality and DNA damage in Xenopus tropicalis and Xenopus laevis spermatozoa. Reprod. Fertil. Dev. 29, 1556–1566.
| Effects of freezing and activation on membrane quality and DNA damage in Xenopus tropicalis and Xenopus laevis spermatozoa.Crossref | GoogleScholarGoogle Scholar | 27692061PubMed |
Mugnano, J. A., Costanzo, J. P., Beesley, S. G., and Lee, R. E. (1998). Evaluation of glycerol and dimethyl sulfoxide for the cryopreservation of spermatozoa from the wood frog (Rana sylvatica). Cryo Letters 19, 249–254.
O’Hanlon, S. J., Rieux, A., Farrer, R. A., Rosa, G. M., Waldman, B., Bataille, A., Kosch, T. A., Murray, K. A., Brankovics, B., Fumagalli, M., Martin, M. D., Wales, N., Alvarado-Rybak, M., Bates, K. A., Berger, L., Böll, S., Brookes, L., Clare, F., Courtois, E. A., Cunningham, A. A., Doherty-Bone, T. M., Ghosh, P., Gower, D. J., Hintz, W. E., Höglund, J., Jenkinson, T. S., Lin, C.-F., Laurila, A., Loyau, A., Martel, A., Meurling, S., Miaud, C., Minting, P., Pasmans, F., Schmeller, D. S., Schmidt, B. R., Shelton, J. M. G., Skerratt, L. F., Smith, F., Soto-Azat, C., Spagnoletti, M., Tessa, G., Toledo, L. F., Valenzuela-Sánchez, A., Verster, R., Vörös, J., Webb, R. J., Wierzbicki, C., Wombwell, E., Zamudio, K. R., Aanensen, D. M., James, T. Y., Gilbert, M. T. P., Weldon, C., Bosch, J., Balloux, F., Garner, T. W. J., and Fisher, M. C. (2018). Recent Asian origin of chytrid fungi causing global amphibian declines. Science 360, 621–627.
| Recent Asian origin of chytrid fungi causing global amphibian declines.Crossref | GoogleScholarGoogle Scholar | 29748278PubMed |
Pearl, E., Morrow, S., Noble, A., Lerebours, A., Horb, M., and Guille, M. (2017). An optimized method for cryogenic storage of Xenopus sperm to maximise the effectiveness of research using genetically altered frogs. Theriogenology 92, 149–155.
| An optimized method for cryogenic storage of Xenopus sperm to maximise the effectiveness of research using genetically altered frogs.Crossref | GoogleScholarGoogle Scholar | 28237331PubMed |
Proaño, B., and Pérez, O. D. (2017). In vitro fertilizations with cryopreserved sperm of Rhinella marina (Anura: Bufonidae) in Ecuador. Amphib. Reptile Conserv. 11, 1–6.
Ralls, K., Sunnucks, P., Lacy, R. C., and Frankham, R. (2020). Genetic rescue: A critique of the evidence supports maximizing genetic diversity rather than minimizing the introduction of putatively harmful genetic variation. Biol. Conserv. 251, 108784.
| Genetic rescue: A critique of the evidence supports maximizing genetic diversity rather than minimizing the introduction of putatively harmful genetic variation.Crossref | GoogleScholarGoogle Scholar |
Sargent, M. G., and Mohun, T. J. (2005). Cryopreservation of sperm of Xenopus laevis and Xenopus tropicalis. Genesis 41, 41–46.
| Cryopreservation of sperm of Xenopus laevis and Xenopus tropicalis.Crossref | GoogleScholarGoogle Scholar | 15645449PubMed |
Scheele, B. C., Pasmans, F., Skerratt, L. F., Berger, L., Martel, A., Beukema, W., Acevedo, A. A., Burrowes, P. A., Carvalho, T., Catenazzi, A., De la Riva, I., Fisher, M. C., Flechas, S. V., Foster, C. N., Frías-Álvarez, P., Garner, T. W. J., Gratwicke, B., Guayasamin, J. M., Hirschfeld, M., Kolby, J. E., Kosch, T. A., La Marca, E., Lindenmayer, D. B., Lips, K. R., Longo, A. V., Maneyro, R., McDonald, C. A., Mendelson, J., Palacios-Rodriguez, P., Parra-Olea, G., Richards-Zawacki, C. L., Rödel, M.-O., Rovito, S. M., Soto-Azat, C., Toledo, L. F., Voyles, J., Weldon, C., Whitfield, S. M., Wilkinson, M., Zamudio, K. R., and Canessa, S. (2019). Amphibian fungal panzootic causes catastrophic and ongoing loss of biodiversity. Science 363, 1459–1463.
| Amphibian fungal panzootic causes catastrophic and ongoing loss of biodiversity.Crossref | GoogleScholarGoogle Scholar | 30923224PubMed |
Shishova, N. R., Uteshev, V. K., Kaurova, S. A., Browne, R. K., and Gakhova, E. N. (2011). Cryopreservation of hormonally induced sperm for the conservation of threatened amphibians with Rana temporaria as a model research species. Theriogenology 75, 220–232.
| Cryopreservation of hormonally induced sperm for the conservation of threatened amphibians with Rana temporaria as a model research species.Crossref | GoogleScholarGoogle Scholar | 21040966PubMed |
Stockwell, M., Clulow, S., Clulow, J., and Mahony, M. (2008). The impact of the Amphibian Chytrid Fungus Batrachochytrium dendrobatidis on a Green and Golden Bell Frog Litoria aurea reintroduction program at the Hunter Wetlands Centre Australia in the Hunter Region of NSW. Aust. J. Agric. Res. 34, 379–386.
Stuart, S. N., Chanson, J. S., Cox, N. A., Young, B. E., Rodrigues, A. S. L., Fishman, D. L., and Waller, R. W. (2004). Status and trends of amphibian declines and extinctions worldwide. Science 306, 1783–1786.
| Status and trends of amphibian declines and extinctions worldwide.Crossref | GoogleScholarGoogle Scholar | 15486254PubMed |
Takamune, K. (1987). Detection of acrosome-reacted toad sperm based on specific lectin binding to the inner acrosomal membrane. Gamete Res. 18, 215–223.
| Detection of acrosome-reacted toad sperm based on specific lectin binding to the inner acrosomal membrane.Crossref | GoogleScholarGoogle Scholar | 3507373PubMed |
Ueda, Y., Yoshizaki, N., and Iwao, Y. (2002). Acrosome reaction in sperm of the frog, Xenopus laevis: Its detection and induction by oviductal pars recta secretion. Dev. Biol. 243, 55–64.
| Acrosome reaction in sperm of the frog, Xenopus laevis: Its detection and induction by oviductal pars recta secretion.Crossref | GoogleScholarGoogle Scholar | 11846477PubMed |
Upton, R., Clulow, S., Mahony, M. J., and Clulow, J. (2018). Generation of a sexually mature individual of the Eastern dwarf tree frog, Litoria fallax, from cryopreserved testicular macerates: proof of capacity of cryopreserved sperm derived offspring to complete development. Conserv. Physiol. 6, coy043.
| Generation of a sexually mature individual of the Eastern dwarf tree frog, Litoria fallax, from cryopreserved testicular macerates: proof of capacity of cryopreserved sperm derived offspring to complete development.Crossref | GoogleScholarGoogle Scholar | 30151196PubMed |
Uteshev, V. K., Shishova, N., Kaurova, S., Manokhin, A., and Gakhova, E. (2013). Collection and cryopreservation of hormonally induced sperm of pool frog (Pelophylax lessonae). Russ. J. Herpetol. 20, 105–109.
Zippel, K., Johnson, K., Gagliardo, R., Gibson, R., McFadden, M., Browne, R., Martinez, C., and Townsend, E. (2011). The Amphibian Ark: a global community for ex situ conservation of amphibians. Herpetol. Conserv. Biol. 6, 340–352.