Expression of platelet derived growth factor (PDGF) ligands and receptors in the ovine ovary and effects of PDGFBB on granulosa cell function
Peter Smith A , Karen L. Reader

A
B
C
D
Abstract
While platelet derived growth factors (PDGF) are expressed in the ovary of some species, relatively little is known regarding the potential role that PDGF play in regulating ovarian follicular development.
To determine the effects of PDGF on granulosa cell function from ovine antral follicles and characterise the expression of PDGF ligands and receptors in developing follicles.
The effects of the PDGF ligand PDGFBB on thymidine incorporation and progesterone production of granulosa cells were determined with established bioassays. Expression patterns of PDGF ligands, PDGFA, B, C, D, and receptors, PDGFRA and B, were determined using in situ hybridisation. The more sensitive technique of RT-PCR was used to confirm expression of PDGFRA and B in granulosa cells of antral follicles.
Thymidine incorporation was increased, and progesterone production decreased, from granulosa cells in response to PDGFBB. Oocytes, granulosa cells, thecal layers and corpora lutea expressed at least one PDGF family member. Granulosa cells had faint expression of PDGF receptors, with thecal and luteal tissue also expressing PDGF receptors. Expression of both ligands and receptors was observed in stroma around the follicle.
In sheep, ovarian follicles express both PDGF ligands and receptors, and PDGFBB regulated both thymidine incorporation and progesterone production.
In sheep, PDGF was identified as an additional locally produced growth factor that regulates follicular function, stimulating granulosa cell proliferation and inhibiting progesterone production. Future work to better understand the role of the different ligands and receptors at different stages of ovarian follicular development seems warranted.
Keywords: corpus luteum, follicle, granulosa cell bioassay, mRNA expression, ovary, platelet derived growth factor receptors, platelet derived growth factors, sheep.
Introduction
The platelet derived growth factors (PDGFs) have been shown to drive cellular responses, including proliferation, migration, survival and deposition of extracellular matrix (Zou et al. 2022). They are disulfide linked dimers of two glycosylated polypeptide chains. Four PDGF subunits are known (PDGFA, PDGFB, PDGFC and PDGFD), but only PDGFA and B can form heterodimers, thus five PDGF dimers have been identified. Two related receptors are known: alpha (PDGFRA) and beta (PDGFRB), and these can also function as homo- or heterodimers (Hoch and Soriano 2003; Fredriksson et al. 2004). The ligands PDGFAA, AB, BB and CC can bind to and activate the alpha receptor, while PDGFBB and DD can bind and activate the beta receptor. PDGFAB, BB and CC can also activate the heterodimeric receptor PDGFR A/B (Fredriksson et al. 2004).
Expression of PDGFs and their receptors have been observed in the ovaries of multiple species, including rats, humans and goats (Sleer and Taylor 2007a; Pinkas et al. 2008; Brito et al. 2012, 2015). Treatment of 4-day old rat ovaries in culture with PDGFAB resulted in a significant decrease in the percentage of primordial follicles present and a concomitant increase in the percentage of primary (early growing) follicles, suggesting that at least PDGFAB may play a role in initiating follicular growth (Nilsson et al. 2006). Similarly, treatment of prepubertal equine chorionic gonadotrophin (eCG)-treated rats with a PDGFR inhibitor increased ovarian apoptosis, leading to follicular atresia, suggesting a role for PDGF in supporting follicular development (Pascuali et al. 2015). Addition of PDGF to cultured preantral follicles increased FSH stimulated growth in goat follicles (Brito et al. 2012).
The role of growth factors within the ovary are known to vary between species. For example, the roles of the oocyte derived growth factors, bone morphogenetic protein 15 (BMP15) and growth differentiation factor 9 (GDF9), are quite different between species, with BMP15 critical for normal ovarian growth in sheep but not rodents (Juengel and McNatty 2005). Therefore, we aimed to understand: (a) which PDGF and PDGFR were expressed during ovarian follicular development in sheep and (b) how PDGFs regulate granulosa function. To do this, we determined the effects of PDGFBB on thymidine incorporation and progesterone production in granulosa cells of medium size (1–2 mm) antral follicles. Additionally, we used in situ hybridisation and RT-PCR to determine the pattern of expression of PDGFA, PDGFB, PDGFC and PDGFD as well as PDGFRA and PDGFRB during follicular development.
Materials and methods
Except where indicated, laboratory chemicals were obtained from Roche Diagnostics N.Z. Ltd (Auckland, New Zealand), Invitrogen (Auckland, New Zealand) or BDH Chemicals New Zealand (Palmerston North, New Zealand).
Granulosa cell culture
Granulosa cells were isolated from ovaries collected from the local abattoir and cultured as previously described (Juengel et al. 2004). Briefly, ovarian follicles 1–2 mm in diameter were dissected from the ovary and granulosa cells collected using a wire loop. Oocytes, oocyte-cumulus cell complexes and follicular debris were removed, and remaining granulosa cells were collected following centrifugation at 300g. The effects of human PDGFBB (10 ng/mL; R&D Systems Inc, MN USA) on cell proliferation, as measured by 3H-thymidine incorporation, and differentiation, as measured by progesterone production, were determined. Platelet derived growth factor BB was chosen as it is known to interact with all three receptor forms (Zou et al. 2022), thus it provides an overview of the effects of PDGF on granulosa cell function. For both bioassays, PDGFBB was added at the beginning and maintained throughout the duration of the culture period. The incorporation of 3H-thymidine was determined following a 24-h culture (20,000 viable cells per well) in M199 media, using a Wallac Trilux MicroBeta 1450 liquid scintillation counter (Biolab, Auckland, NZ). Secretion of progesterone was measured following culture of granulosa cells (100,000 viable cells per well) in an enriched McCoys medium with 30 ng/mL androstenedione, 3 ng/mL ovine FSH and 1 ng/mL insulin-like growth factor 1 (IGF-1). Cells were cultured for 6 days with media being replaced every 48 h. Progesterone concentrations in media from the last 48 h in culture were determined using radioimmunoassay (RIA) (Lun et al. 1998). No samples were below the sensitivity of the assay, and the intra- and inter-assay coefficients of variation were below 20%. DNA concentration following the 6-day culture was determined using Hoechst 33258 dye (Sigma, Auckland, NZ) as described (Juengel et al. 2004). No samples were below the sensitivity of the assay and intra- and inter-assay coefficients of variation were < 10%. A minimum of four independent pools of granulosa cells, collected on a minimum of three days were used in the bioassays to evaluate proliferation and differentiation. Differences between control and PDGFBB treated granulosa cells were determined using paired t-tests.
Ovary collection
Romeny × Texel ewes and lambs were used. All animal experiments were approved by the Invermay Animal Ethics Committee. Ovaries were collected from 4-week-old lambs and post-pubertal ewes 10 months, 2 years and 8 years-of-age (n = 5 animals per age group). For post-pubertal ewes, ovaries were collected during the mid-luteal phase (day 12 after oestrus expression, as determined by a vasectomised ram wearing a marking harness).
All ewes were humanely killed with a 20 mL intravenous injection of a solution containing 500 mg/mL of sodium pentobarbitone (Pentobarb 500, South Island Chemicals, Christchurch, New Zealand). Ovaries were removed and fixed in 4% paraformaldehyde at 4°C overnight. Following an overnight wash in 70% ethanol, ovaries were processed for histology following a standard procedure.
In situ hybridisation
Total cellular RNA, isolated from ovarian tissue using TRIzol following manufacturer’s instructions, was used to generate cDNA encoding a portion of the coding genes of interest. Briefly, 5 μg RNA was reverse transcribed using Superscript III and oligo-dT primers, following manufacturer’s instructions. Resulting cDNA was amplified using either HotMaster Taq (Eppendorf AG, Hamburg, Germany; PDGFA, PDGFB, PDGFD, PDGFRB) or Qiagen Taq (Biolab Scientific Ltd, Wellington, New Zealand; PDGFC and PDGFRA). Conditions for HotMaster Taq were 1 cycle of denaturation at 94°C for 2 min; 40 cycles at 94°C for 20 s (denaturation), annealing temperature given in Table 1 for 15 s, 72°C for 50 s for extension; and one cycle at 72°C for final extension. Conditions for Qiagen were one cycle at 94°C for 3 min for denaturation; 40 cycles at 94°C for 1 min (denaturation), annealing temperature given in Table 1 for 1 min, 72°C for 2 min for extension; and one cycle at 72°C for 10 min for final extension. The resulting PCR products were ligated into pGEMTeasy vectors (Promega; Dade Diagnostics PTY Ltd, Auckland, New Zealand) according to manufacturer’s instructions. Identity and orientation of the insert were confirmed by sequencing (Waikato DNA Sequencing Facility; The University of Waikato; Hamilton, New Zealand). The in situ hybridisation protocol is based on the method of Tisdall et al. (1994) and has been published previously (Juengel et al. 2000). A total of five animals at each age were examined for each gene, with a minimum of 3–4 slides observed. The follicle classification system of Lundy et al. (1999) was used to characterise the expression pattern during follicular development. Non-specific signal was monitored by inclusion of slides hybridised to the sense probe. For all genes, signal of the sense probe was similar or lower in intensity to that observed over the portion of the slide without tissue that had been exposed to either the sense or antisense probe, and therefore was considered to be non-specific (data not shown).
Gene | Primer sequences | Annealing temperature (°C) | Product length (bp) | |
---|---|---|---|---|
Generation of in situ hybridisation probes | ||||
PDGFA | For: GTC AGA TCC ACA GCA TCC G Rev: GCT ACA ATA CTT GCT TTG ATG TCA C | 58 | 670 | |
PDGFB | For: CGC CAA CTT CCT GGT GTG Rev: CTC CGA GGC TCT CCT TCA G | 58 | 403 | |
PDGFC | For: CTT ATT CGG TAT CTT CAA CCA G Rev: AAC AGG CAC AGT TCC CAC CAC | 50 | 268 | |
PDGFD | For: TCC CAG GAG AAA ACA MGG ATA CA Rev: CTG TAA CGC TTG RCA TCA TCA TT | 52 | 559 | |
PDGFRA | For: AAA CCC AGG TAT GAA ATC CG Rev: CTT CTC TGG ATG GTG GCT C | 50 | 447 | |
PDGFRB | For: TCA ACC TGC TGG GGG C Rev: AGG CTG TTG AAG ATG CTC TC | 58 | 642 | |
For RT-PCR | ||||
PDGFRA | For: GAC TCA AGT TCC GTC CTC CA Rev: CCT TGG TTT CTG TTT CCA AAT G | Variable | 431 | |
PDGFRB | For: GCT CTA CAT CTT TGT GCC AGA T Rev: TTG ATG GAT GAC ACC TGG AG | Variable | 300 | |
B2M | For: GGC CTG CTG TCG CTG TCT Rev: TTC TGG CGG GTG TCT TGA GT | Variable | 78 |
Determining expression of PDGFRA and PDGFRB in isolated and cultured granulosa cells
Granulosa cells were collected as described for granulosa cell culture and plated as for determining the effects of PDGFBB on progesterone production. Granulosa cells were either frozen at −80°C directly after isolation (n = 6 pools) or after 2 days in culture. Total cellular RNA was isolated using TRIzol (Invitrogen, Auckland, New Zealand) following manufacturer’s instructions and cDNA was synthesised using Superscript III. The product was amplified (Taq polymerase; Invitrogen) using the primers listed in Table 1 and the following conditions: denaturing for 2 min at 95°C; 4 × 4 cycles at 95°C for 20 s (denaturation), 65°C for 30 s (annealing), 72°C for 1 min (extension), with annealing temperature decreasing 3°C every 4 cycles; 20 cycles at 95°C for 20 s (denaturation), 53°C for 30 s (annealing), 72°C for 1 min (extension); and a final extension of 5 min at 72°C. The resulting PCR products were visualised following electrophoresis through 2% (PDGFRA and PDGFRB) or 3% (beta-2-microglobulin; B2M; amplification positive control) agarose gels and sequenced to confirm identity.
Results
Effects of PDGFBB on granulosa cell function (Fig. 1)
Addition of PDGFBB stimulated (P < 0.05) thymidine incorporation into freshly collected granulosa cells 4.33 ± 1.10 fold compared to untreated control cells. Additionally, culture of granulosa cells with PDGFBB for 6 days resulted in a decrease (P < 0.01) in progesterone secretion to 0.33 ± 0.03 of control cells during the last 48 h of culture, with no change in DNA content (1.06 ± 0.09 relative to control cells).
Expression of PDGFs and their receptors in the ovary as determined by in situ hybridisation (Figs. 2–4)
Expression of PDGFA and B was observed in oocytes at all stages of follicular development, from Type 1/1a through to large antral follicles. In granulosa cells of healthy follicles, expression of PDGFA and B was first observed in Type 2 follicles and continued to be observed throughout development of the follicle. Granulosa cell expression of PDGFC in healthy follicles was first evident in large preantral follicles (Type 3/4) and was observed in small, but not larger, antral follicles. In healthy follicles, expression in the thecal layers was not apparent until the large preantral stage (Type 4) and was initially restricted to PDGFB. At antral stages, PDGFB was expressed predominantly in the theca interna, whereas PDGFA was expressed in both theca interna and externa. Healthy follicles did not express PDGF receptors until after antrum formation, with PDGFRB limited to theca interna but PDGFRA observed in both theca interna and externa. In atretic follicles, expression of PDGFA and B appeared to decrease in granulosa cells but increase in the thecal layers. Expression of PDGFC appeared to increase in atretic follicles, with expression now observed in granulosa cells and in the thecal layers of large atretic antral follicles. Expression of PDGFD was not observed in ovarian follicles. The mid-luteal corpus luteum expressed PDGFA, B, C and D as well as PDGFRA and B, with receptor expression often associated with the vasculature. In the ovarian stroma, expression of PDGFA, B and C, as well as PDGFRA and B mRNA was observed around follicles and appeared to be associated with the vasculature.
Corresponding brightfield (a, c, e, g) and darkfield (b, d, f, h) images of ovarian tissue after hybridisation to PDGFA (a, b), PDGFB (c, d), PDGFC (e, f) or PDGFD (g, h) cRNA. Expression of PDGFA (panel b) can be observed in the oocyte (o), granulosa (g) and theca (t) of various stages of follicle development. Expression can also be observed in the ovarian stroma around the follicles. In panel (d), expression of PDGFB can be observed in the granulosa and theca of a type 5 antral follicle, as well as the ovarian stroma. In panel (f), expression of PDGFC can be observed in the granulosa of a healthy antral follicle and the granulosa and theca of an atretic antral follicle, as well as in ovarian stroma. Expression of PDGFD was only detected in the corpus luteum (star, panel h). Arrowhead, primary follicles; arrow, type 3 and 4 follicles; grey stippled block arrow, atretic antral follicle; solid block arrow, healthy antral follicle. Scale bars equal approximately 100 μm.
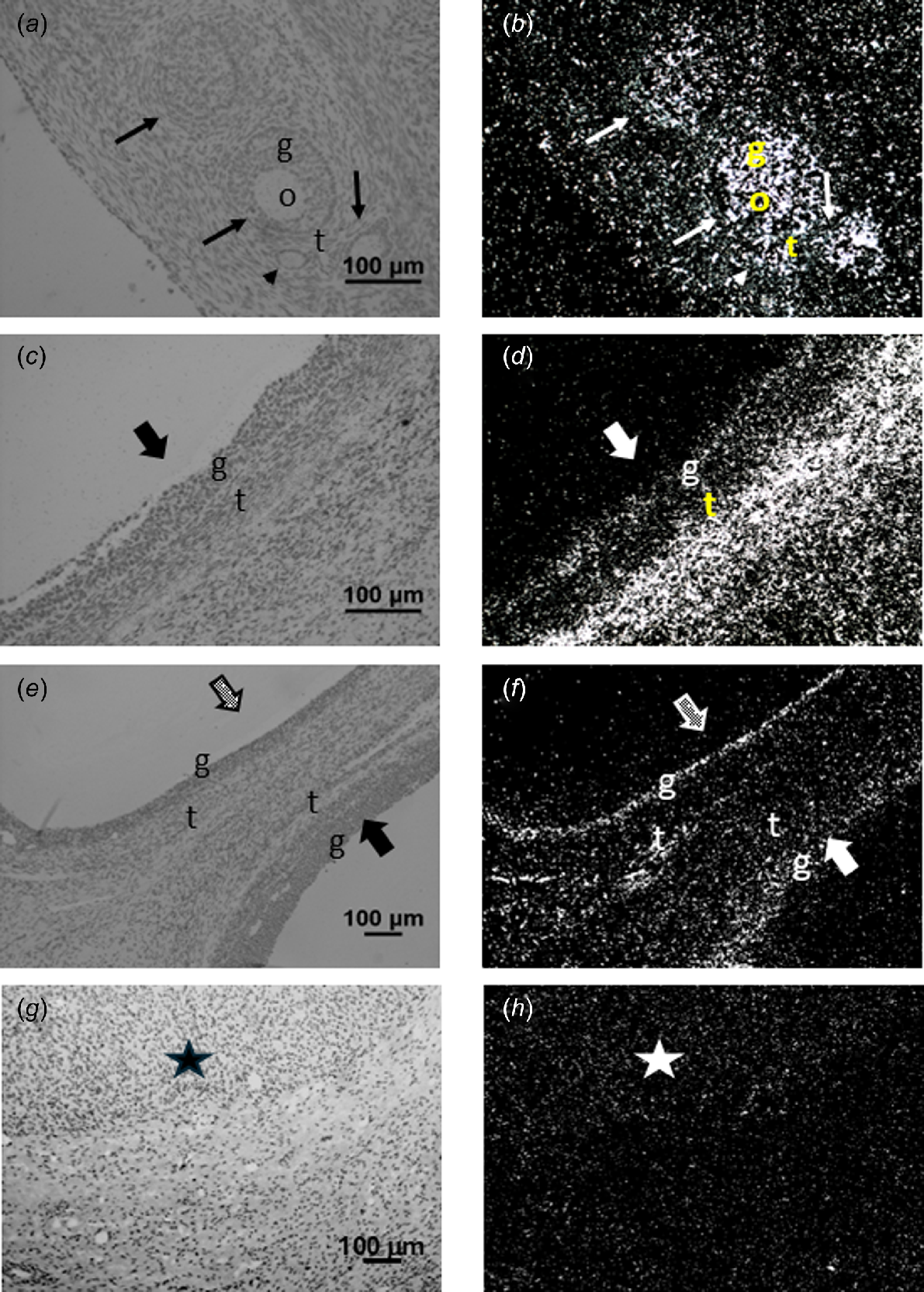
Corresponding brightfield (a, c, e, g) and darkfield images (b, d, f, h) of ovarian tissue after hybridisation to PDGFRA (a–d) or PDGFRB (e–h) cRNA. In panel (b) note that PDGFRA expression can be observed in the theca (t) but not in the oocyte (o) or granulosa (g) of the growing type 3 and 4 follicles (arrows). Note as well the expression observed in the ovarian stroma (panels b and d) and corpus luteum (star; panel d). In panel (f), note expression of PDGFRB was not observed in the oocyte or granulosa of this type 4 follicle, but was observed in the theca. Similarly, in panel (h), expression of PDGFRB was observed in the theca, but not granulosa of this healthy antral follicle (solid block arrow). Again, expression of PDGFRB is noted in the ovarian stroma in both panel (f) and (h). Scale bars equal approximately 100 μm.
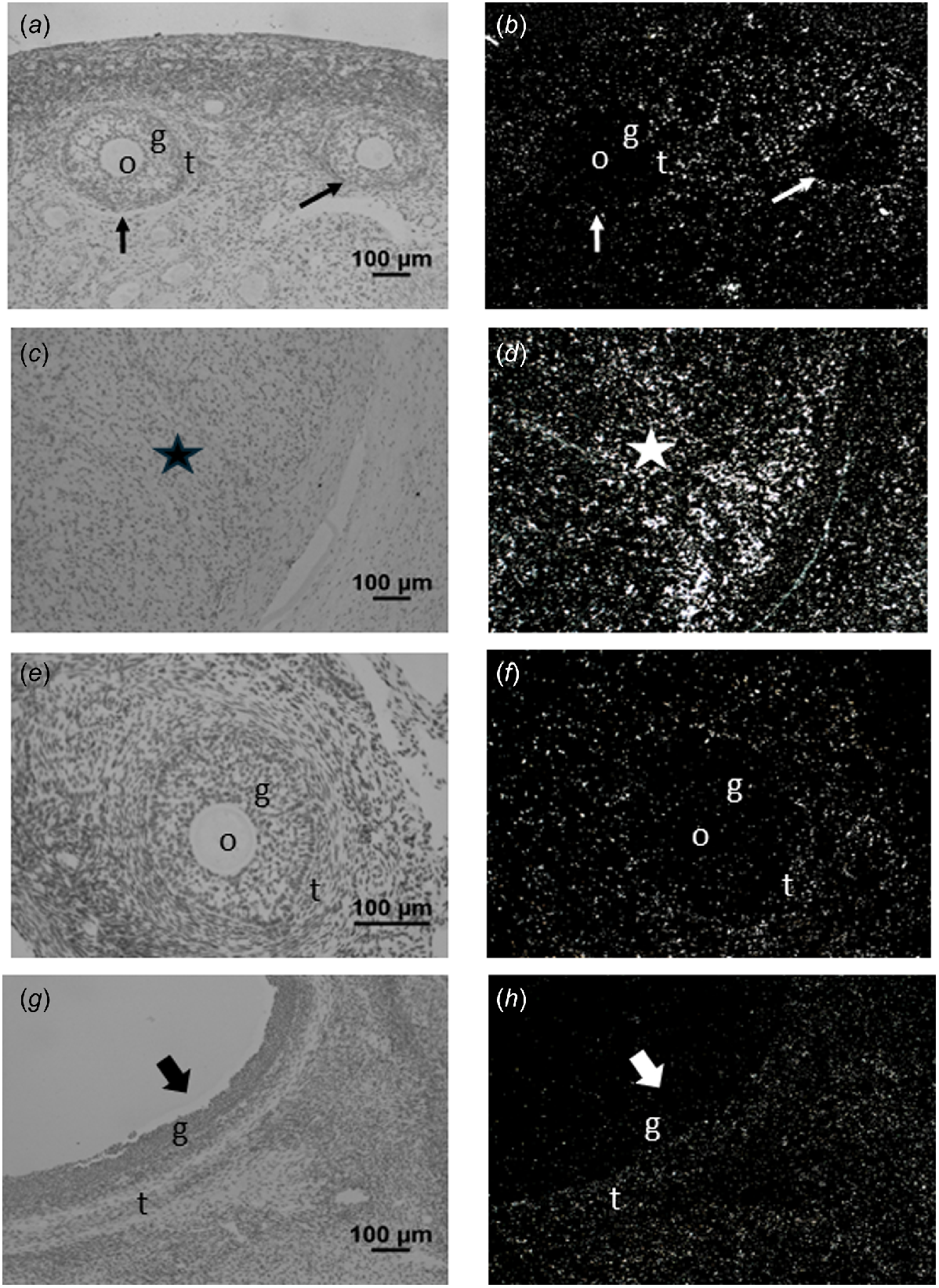
Schematic representation of expression of PDGFA–D and PDGFRA and B in growing and atretic follicles, as assessed using in situ hybridisation. Follicle type is indicated below the follicle. Blue arrow indicates expression in oocyte, black arrows highlight expression in granulosa cells, purple arrows indicate expression in the stroma/developing theca of preantral follicles, orange arrows indicate expression in the theca interna, and green arrows indicating expression in theca externa. A down arrow after the gene name indicates that the signal appeared weaker than in healthy follicles. An up arrow indicates that the signal appeared stronger than in healthy follicles, whereas a ± indicates that the signal was observed in some but not all follicles. Some panels depicting follicle types have been previously shown (Juengel and McNatty 2005; Scaramuzzi et al. 2011).
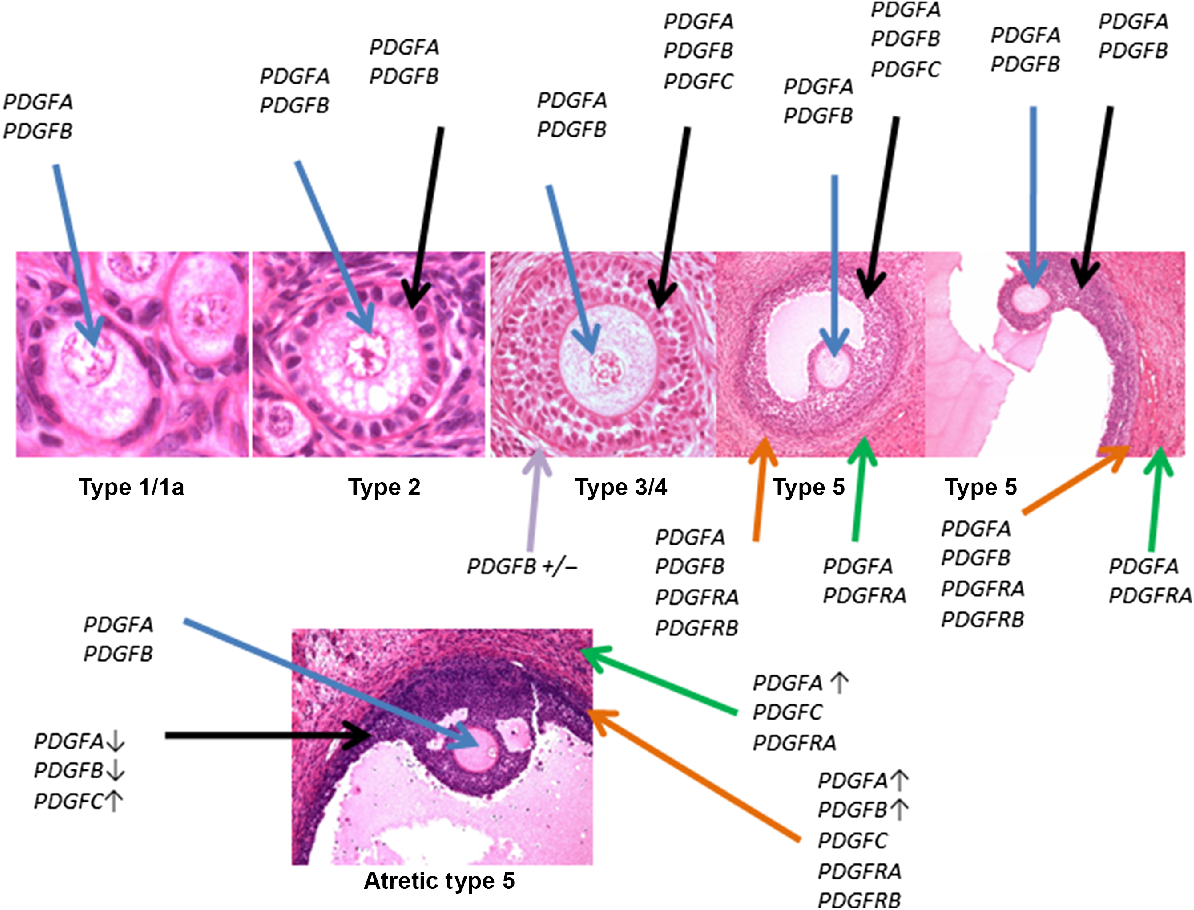
Expression of PDGFRA and B in granulosa cells as determined by RT-PCR (Fig. 5)
The inability to localise either PDGFRA or B in granulosa cells was surprising, given the strong effects of PDGFBB on granulosa cell function. Therefore, the more sensitive method of RT-PCR was used to determine if low expression of PDGFRA or B was present in granulosa cells of antral follicles 1–2 mm in diameter. Of six pools of granulosa cells, four showed obvious signal for both PDGFRA and B, with one pool showing weak expression and the remaining pool below the sensitivity of the assay (not detectable). Culture of the granulosa cells for 48 h resulted in strong expression in the two pools examined. This included the pool of cells with non-detectable expression prior to culture.
Determination of expression of mRNA encoding PDGFRA and PDGFRB in granulosa cells that were either freshly isolated (lanes 3–8) or cultured (Cult) for 48 h (lanes 9 and 10). Results from positive control samples from ovaries (Ov) collected from a day 75 fetus (lane 2), a 4-week-old lamb (lane 1 and 12) or an adult ewe (lane 11) and negative controls (−Ctl) (genomic DNA; lane 13 and water blank; lane 14) are also shown. Size of resulting PCR product is indicated on the left with molecular weight markers (100 bp graduations), indicated by MW. Identity of PDGFR product is indicated on the right. Expression of B2M is indicated on the bottom as a positive control for the method.
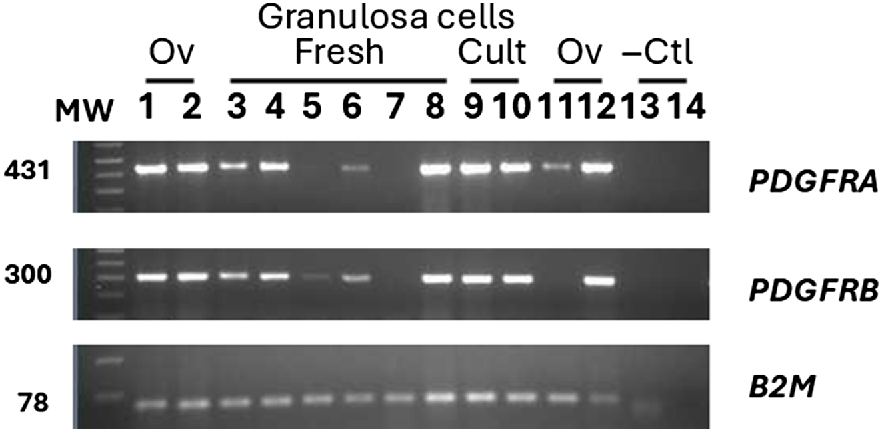
Discussion
Ovine oocytes of all follicle sizes expressed PDGFA and B mRNA, adding these growth factors to the growing number of oocyte secreted growth factors in this species. Using immunocytochemistry, Brito et al. (2015) observed localisation of PDGFA, B, C and D in caprine oocytes, similarly to what had been observed in rats (Sleer and Taylor 2007a) and humans, albeit expression of PDGFC and D were not determined in humans (Pinkas et al. 2008). Whether the lack of expression of PDGFC and D in ovine oocytes is related to differences between species or reduced sensitivity of the in situ hybridisation method is unknown. However, it should be noted that in both rats and goats (Sleer and Taylor 2007a; Brito et al. 2015), strong expression of PDGFC and D mRNA/protein were observed in oocytes, supporting a subtle difference in expression pattern for this family of ligands in sheep compared to other species, albeit with PDGF ligands produced by the oocytes of all species examined.
As the follicle begins to grow, granulosa cells also begin to express PDGFA and B, with C first being observed in the larger preantral follicles. With the exception of the lack of detectable PDGFD in the ovine ovarian follicles, this pattern is similar to that observed in goats (Brito et al. 2015), with expression in rat granulosa cells limited to PDGFA (Sleer and Taylor 2007a). Pinkas et al. (2008) observed localisation of PDGFA and B in granulosa cells of some, but not all, follicles. Gasser et al. (2024) localised PDGFA and B to granulosa cells of antral, including atretic and dominant, follicles in cattle. Again, while expression of PDGF ligands in the granulosa cells of growing follicles is consistent across species, there appears to be subtle differences in the patterns of expression. Of interest in sheep, expression of PDGFA and B appeared decreased when the follicle was noted as atretic, whereas PDGFC expression appeared to increase. This is supportive of PDGFC having a differing role in ovine ovarian follicular development than PDGFA and B, with further research warranted to better understand the protein localisation and role of each of the PDGF ligands on follicular development.
In the thecal layers, PDGFB was the first family member detected in the theca interna at the later preantral stage, with expression of PDGFA observed shortly thereafter in small antral follicles. In the theca externa of healthy follicles, only PDGFA was observed. This pattern of expression for theca interna is similar to that observed in goats, rats, humans and cows (Sleer and Taylor 2007a; Pinkas et al. 2008; Brito et al. 2015; Gasser et al. 2024). This is in contrast to the expression pattern of PDGFC and D which has been observed in theca of goat or rat follicles (Sleer and Taylor 2007a; Brito et al. 2015), but was not detected in ovine thecal layers in the current study. Whether these differences are related to species differences or differences in methodologies (immunohistochemistry vs in situ hybridisation) is unclear. It is also of interest to note that PDGFC was observed in the theca of atretic ovine follicles in the current study, whereas the studies on rats and goats did not differentiate on follicular health. This could be an underlying methodological cause for the difference in expression of PDGFC observed between species. Strong expression of PDGFA, B and C in the thecal layers of atretic follicles is suggestive of a role for these growth factors in regulation of follicular atresia. Given the apparent differences in expression of the various PDGF ligands between healthy and atretic follicles observed in both granulosa and theca cells, further work to better understand how the different PDGF isoforms affect both granulosa and theca cell health seems warranted.
In the current study with ovine follicles, expression of the PDGF receptors was limited to the theca interna and externa when assessed by in situ hybridisation; however, the very sensitive technique of RT-PCR revealed that expression of PDGFRA and B was observable in some pools of granulosa cells. Expression of PDGFR in theca and granulosa of antral follicles has been observed in goats, rats and humans (Sleer and Taylor 2007a; Pinkas et al. 2008; Brito et al. 2015), albeit in humans, expression in the theca was limited to PDGFRA.
In the current study with ovine ovaries, expression of ligands and their receptors were also observed in corpora lutea, as well as the stroma surrounding follicles, often associated with the vasculature. This expression pattern is similar to what has been observed in human, goats and rats (Sleer and Taylor 2007a, 2007b; Pinkas et al. 2008; Brito et al. 2015) and supports a role for PDGF in regulation of luteal function and regulation of the vasculature of ovarian follicles and corpora lutea.
Reinforcing the localisation of PDGFRA and B and their role in ovine granulosa cells was the strong effects on thymidine incorporation and progesterone production observed in response to PDGFBB in culture. Aligned with the observed stimulation of thymidine incorporation in the current study, in early studies with porcine granulosa cell cultures containing a low concentration of platelet-poor plasma-derived serum, PDGF facilitated granulosa cell proliferation by other growth factors such as epidermal growth factor (May et al. 1990). However, it should also be noted that in the current study, the culture conditions utilised for measuring the effects on progesterone production likely induces some luteinisation of the ovine granulosa cells (Juengel et al. 2004). Therefore, given expression of the receptors in luteal tissue, some of the effects observed regarding downregulation of progesterone production could be related to regulation of luteal function. In rats, treatment with human chorionic gonadotropin upregulated PDGFRB. Furthermore, inhibition of PDGF receptor activity at the time of ovulation induction reduced corpora lutea number, potentially through disruption of the vasculature (Sleer and Taylor 2007b). In the current study, partial luteinisation of the granulosa cells could also explain why DNA content was not different between PDGF treated and control cultures in the longer-term culture, in contrast to the stimulation of thymidine uptake in the shorter-term culture. The lack of stimulation of DNA content in the longer culture conditions is similar to what May et al. (1990) observed, in that PDGF alone did not increase cell numbers when assessed in a longer-term culture.
There have been studies examining the effects of PDGF on ovarian follicular development using in vivo models or culture of whole ovaries/follicles. These are primarily focused on the early stages of follicular development. In cultured goat preantral follicles, treatment with PDGF increased antrum formation and, when given with FSH, stimulated the growth of preantral follicles (Brito et al. 2012). In the current study, stimulation of thymidine incorporation is also consistent with a role of PDGF in follicular growth. Experiments in rats support the role of PDGF in initiating follicular growth, as treatment of four day old rat ovaries in culture with the PDGF ligand PDGFAB resulted in a significant decrease in the percentage of primordial follicles present and a concomitant increase in the percentage of primary (early growing) follicles (Nilsson et al. 2006). In prepubertal eCG-treated rats, treatment with a PDGFR inhibitor increased ovarian apoptosis, leading to follicular atresia (Pascuali et al. 2015). In the current study, we noted apparent alterations in expression of mRNA encoding PDGF ligands, also supportive of a role in follicular atresia.
In conclusion, expression of multiple PDGF family members and their receptors in all stages of ovarian follicular development is consistent with a role of this growth factor family in regulating ovarian follicular development in sheep. Further research to confirm protein localisation of the PDGF ligands and receptors in growing follicles in sheep would further our understanding of potential differences in the role of these growth factors among species. Treatment of granulosa cells from antral follicles with PDGFBB stimulated thymidine uptake and inhibited progesterone production. These responses are consistent with a growth factor that stimulates follicular growth while inhibiting differentiation. These effects, stimulation of thymidine incorporation and suppression of progesterone production are similar to the combined effects of the oocyte derived growth factors GDF9 and BMP15, functions which are closely related to regulation of ovulation rate (Juengel et al. 2013). Future work to more fully understand the effects of PDGF on steroid production, including comparisons of different PDGF isoforms, investigations into the effects of PDGF on oestradiol production, as well as characterising the mechanisms of action in altering steroid secretion, would provide additional insights into how PDGF are affecting ovarian function. Expression of PDGF and their receptors was observed in luteal tissue and in stroma around the follicles, often associated with the vasculature. Thus, these growth factors may also be influencing development of the vasculature of the growing follicle and corpus luteum, as well as directly affecting luteal function.
Data availability
Data used in the manuscript is available from the corresponding author upon reasonable request.
Conflicts of interest
Jennifer L. Juengel is co-editor in chief of Reproduction, Fertility and Development. To mitigate this potential conflict of interest, she had no editor-level access to this manuscript during peer review. All other authors declare they have no known conflicts of interest.
Declaration of funding
This work was supported by AgResearch’s Strategic Science Investment Fund from NZ Ministry of Business, Innovation and Employment. Approval for publication was granted by AgResearch.
Acknowledgements
The authors would like to acknowledge the significant influence that Ken McNatty has had on our research careers, as a mentor, colleague, collaborator and friend. The authors would also like to acknowledge the technical assistance of Laurel Quirke, Lisa Haydon and Stan Lun from the Wallaceville and Invermay Reproduction laboratories. We would also like to thank the farm staff at Invermay for help with animal management and collection of tissue.
References
Brito IR, Lima IM, Saraiva MVA, Silva CMG, Magalhaes-Padilha DM, Araujo VR, Barreto Luz V, Barbalho Silva AW, Campello CC, Silva JR, Figueiredo JR (2012) Expression levels of mRNA-encoding PDGF receptors in goat ovaries and the influence of PDGF on the in vitro development of caprine pre-antral follicles. Reproduction in Domestic Animals 47(5), 695-703.
| Crossref | Google Scholar | PubMed |
Brito IR, Sales AD, Rodrigues GQ, Lobo CH, Castro SV, Silva AWB, Moura AAA, Silva JRV, Rodrigues APR, Figueiredo JR (2015) Differential gene expression and immunolocalization of platelet-derived growth factors and their receptors in caprine ovaries. Domestic Animal Endocrinology 51, 46-55.
| Crossref | Google Scholar | PubMed |
Fredriksson L, Li H, Eriksson U (2004) The PDGF family: four gene products form five dimeric isoforms. Cytokine & Growth Factor Reviews 15(4), 197-204.
| Crossref | Google Scholar | PubMed |
Gasser FB, Stassi AF, Lujan Velazquez MMd, Etchevers L, Amweg AN, Salvetti NR, Ortega HH, Baravalle ME (2024) Altered expression of angiogenic factors in dominant preovulatory follicles of dairy cattle treated with ACTH. Animal Reproduction Science 262, 107415.
| Crossref | Google Scholar |
Hoch RV, Soriano P (2003) Roles of PDGF in animal development. Development 130(20), 4769-4784.
| Crossref | Google Scholar | PubMed |
Juengel JL, McNatty KP (2005) The role of proteins of the transforming growth factor-β superfamily in the intraovarian regulation of follicular development. Human Reproduction Update 11(2), 144-161.
| Crossref | Google Scholar |
Juengel JL, Quirke LD, Tisdall DJ, Smith P, Hudson NL, McNatty KP (2000) Gene expression in abnormal ovarian structures of ewes homozygous for the inverdale prolificacy gene. Biology of Reproduction 62(6), 1467-1478.
| Crossref | Google Scholar | PubMed |
Juengel JL, Bibby AH, Reader KL, Lun S, Quirke LD, Haydon LJ, McNatty KP (2004) The role of transforming growth factor-beta (TGF-beta) during ovarian follicular development in sheep. Reproductive Biology and Endocrinology 2, 78.
| Crossref | Google Scholar | PubMed |
Juengel JL, Davis GH, McNatty KP (2013) Using sheep lines with mutations in single genes to better understand ovarian function. Reproduction 146(4), R111-R123.
| Crossref | Google Scholar | PubMed |
Lun S, Smith P, Lundy T, O’Connell A, Hudson N, McNatty KP (1998) Steroid contents of and steroidogenesis in vitro by the developing gonad and mesonephros around sexual differentiation in fetal sheep. Reproduction 114(1), 131-139.
| Crossref | Google Scholar | PubMed |
Lundy T, Smith P, O’Connell A, Hudson NL, McNatty KP (1999) Populations of granulosa cells in small follicles of the sheep ovary. Reproduction 115(2), 251-262.
| Crossref | Google Scholar | PubMed |
May JV, Frost JP, Bridge AJ (1990) Regulation of granulosa cell proliferation: facilitative roles of platelet-derived growth factor and low density lipoprotein. Endocrinology 126(6), 2896-2905.
| Crossref | Google Scholar | PubMed |
Nilsson EE, Detzel C, Skinner MK (2006) Platelet-derived growth factor modulates the primordial to primary follicle transition. Reproduction 131(6), 1007-1015.
| Crossref | Google Scholar | PubMed |
Pascuali N, Scotti L, Abramovich D, Irusta G, Di Pietro M, Bas D, Tesone M, Parborell F (2015) Inhibition of platelet-derived growth factor (PDGF) receptor affects follicular development and ovarian proliferation, apoptosis and angiogenesis in prepubertal eCG-treated rats. Molecular and Cellular Endocrinology 412, 148-158.
| Crossref | Google Scholar |
Pinkas H, Fisch B, Rozansky G, Felz C, Kessler-Icekson G, Krissi H, Nitke S, Ao A, Abir R (2008) Platelet-derived growth factors (PDGF-A and -B) and their receptors in human fetal and adult ovaries. Molecular Human Reproduction 14(4), 199-206.
| Crossref | Google Scholar | PubMed |
Scaramuzzi RJ, Baird DT, Campbell BK, Driancourt M-A, Dupont J, Fortune JE, Gilchrist RB, Martin GB, McNatty KP, McNeilly AS, Monget P, Monniaux D, Vinoles C, Webb R (2011) Regulation of folliculogenesis and the determination of ovulation rate in ruminants. Reproduction, Fertility and Development 23(3), 444-467.
| Crossref | Google Scholar | PubMed |
Sleer LS, Taylor CC (2007a) Cell-type localization of platelet-derived growth factors and receptors in the postnatal rat ovary and follicle. Biology of Reproduction 76(3), 379-390.
| Crossref | Google Scholar | PubMed |
Sleer LS, Taylor CC (2007b) Platelet-derived growth factors and receptors in the rat corpus luteum: localization and identification of an effect on luteogenesis. Biology of Reproduction 76(3), 391-400.
| Crossref | Google Scholar | PubMed |
Tisdall DJ, Hudson N, Smith P, McNatty KP (1994) Localization of ovine follistatin and α and βA inhibin mRNA in the sheep ovary during the oestrous cycle. Journal of Molecular Endocrinology 12(2), 181-193.
| Crossref | Google Scholar | PubMed |
Zou X, Tang X-Y, Qu Z-Y, Sun Z-W, Ji C-F, Li Y-J, Guo S-D (2022) Targeting the PDGF/PDGFR signaling pathway for cancer therapy: a review. International Journal of Biological Macromolecules 202, 539-557.
| Crossref | Google Scholar | PubMed |