Role of resistin in the porcine uterus: effects on endometrial steroidogenesis
Marlena Gudelska



A
B
C
D
E
Abstract
The adipose tissue produces adipokines – hormones essential to many biological functions, including reproduction.
We hypothesised that resistin, one of the adipokines, is present in the blood plasma, uterine luminal flushings (ULF) and uterus of pigs during the oestrous cycle and early pregnancy, and that resistin influences uterine steroidogenesis.
This study aimed to determine the expression of resistin in the porcine endometrium and myometrium during the cycle and pregnancy by quantitative real-time polymerase chain reaction and western blot (WB). The adipokine concentrations in blood plasma and ULF were defined by enzyme-linked immunosorbent assay. The impact of resistin on progesterone (P4) and oestradiol (E2) secretion and steroidogenic enzyme proteins’ expression were determined by radioimmunoassay and WB, respectively. The effect of resistin on protein kinase B (Akt) protein phosphorylation was determined by WB.
The study’s results identified the resistin gene and protein expression in the porcine endometrium and myometrium. Moreover, the expression of adipokine in the uterus, its concentrations in the blood plasma and ULF, and its impact on the endometrial P4 and E2 production, are dependent on the hormonal milieu related to the phase of the cycle and/or period of pregnancy. Furthermore, resistin enhances the phosphorylation of the Akt signaling pathway.
The current findings suggest that resistin may control the steroidogenesis process in the porcine endometrium and could be an important regulator of pig reproduction.
In the long-term perspective, the results obtained in this study may help improve farm animal breeding.
Keywords: gestation, oestrous cycle, pig, reproduction, resistin, steroidogenesis, steroids, uterus.
Introduction
Many biological processes in the body depend on hormones produced by the adipose tissue. Adipokines, adipocyte-derived hormones, participate in numerous processes, such as maintaining proper energy homeostasis by controlling hunger and satiety, insulin sensitivity, carbohydrate and fat metabolism, and inflammation (Fasshauer and Blüher 2015). The peptide resistin, so named because of its capability to induce insulin resistance and establish a connection between obesity and diabetes, is a member of the adipokines family. Resistin is also known in the literature as adipocyte-specific secretory factor (ADSF) or found in inflammatory zone 3 (FIZZ3). The hormone is a cysteine-rich protein encoded by the RETN gene and belongs to the resistin-like molecule (RELM) family (Steppan et al. 2001). In the human blood, resistin occurs in the form of a dimer through the formation of disulfide bridges between monomers, the formation of which depends on a large number of cysteine residues (Banerjee and Lazar 2001). Although resistin was discovered several years ago, its receptor has still not been identified. Since resistin performs pleiotropic functions, it is considered that there may be more than one receptor type for this adipokine. It has been shown that resistin binds to the isoform of decorin (DCN), the orphan tyrosine kinase-like receptor 1 (ROR1), the adenylyl cyclase-associated protein 1 (CAP1), and Toll-like receptor 4 (TLR4) (Tarkowski et al. 2010; Daquinag et al. 2011; Sánchez-Solana et al. 2012; Lee et al. 2014). McTernan et al. (2002) suggest that resistin may be a link between obesity, insulin resistance, diabetes, and their complications. It is known that resistin can exert its effects by influencing signaling pathways such as protein kinase B (Akt), extracellular signal-regulated kinases (ERK) 1 and 2 (ERK1/2) and p38 mitogen-activated protein kinases (MAPK), as well as signal transducer and activator of transcription 3 (Stat-3), and peroxisome proliferator-activated receptor γ (PPARγ) (Estienne et al. 2019).
Adipocytes and macrophages are the main sources of resistin. Nonetheless, resistin is also expressed in structures of the hypothalamic–pituitary–ovarian (HPG) axis that regulates the reproductive tract. The presence of resistin was reported in the rodent hypothalami (Wilkinson et al. 2005). The adipokine is expressed in the anterior pituitary (AP) cells, and what is more, it modulates luteinising hormone (LH) secretion by AP cells in vitro (Maillard et al. 2017). In the case of ovaries, resistin is expressed in theca (Th) and granulosa (G) cells and oocytes of rodents as well as in the G and luteal (L) cells of cattle (Maillard et al. 2011). Moreover, Maillard et al. (2011) reported that resistin influences basal and insulin-like growth factor (IGF)-1-stimulated steroidogenesis and proliferation of G cells. In porcine ovaries, resistin is expressed in Th and G cells and influences the steroidogenesis and mitosis of ovarian cells (Rak-Mardyła et al. 2013). In the case of tissues related to the reproductive system outside the HPG axis, resistin expression was found in the human and ovine uterus, as well as in the human and rat placenta (Yura et al. 2003; Caja et al. 2005; Oh et al. 2017; Dall’Aglio et al. 2019). In addition, expression of the resistin gene and protein has also been found in syncytiotrophoblast and extravillous trophoblasts in early gestation and the syncytiotrophoblast in late gestation (Yura et al. 2003). Chen et al. (2005) showed that the concentration of resistin in the serum of pregnant women increases in the third trimester and is higher than in non-pregnant ones. Resistin modulates the expression and activity of glucose transporter 1 (GLUT-1), which is involved in the transplacental movement of glucose necessary for placenta growth and consequently for embryo development (Di Simone et al. 2009).
In the reproductive system, in addition to the ovary, the uterus can also be a source of steroid hormones. Since 2008, it has been known that the porcine uterus can produce steroid hormones, such as oestrone (E1), oestradiol (E2), androstenedione (A4), and testosterone (T) (Franczak 2008; Franczak and Kotwica 2008). Our earlier research established the impact of metabolism-linked hormones (adiponectin, chemerin, orexin A and B) on the uterine functioning in pigs including the process of steroid hormones’ production (Smolinska et al. 2016; Kiezun et al. 2017; Kaminski et al. 2018; Kisielewska et al. 2019; Rytelewska et al. 2020, 2021; Gudelska et al. 2022). It has been shown that resistin is involved in the process of ovarian steroidogenesis in pigs (Rak-Mardyła et al. 2014; Kurowska et al. 2021). The above inspired us to investigate if adipokine is present in the uterine tissues and how it affects steroidogenesis in the porcine uterus. Thus, we hypothesise that resistin is expressed in the porcine endometrium and myometrium during the oestrous cycle and early pregnancy and that the expression is influenced by the hormonal status of animals. We further postulated that the adipokine may be a potential uterine- or adipose-derived factor that regulates steroidogenesis in the uterus of pigs. This study aimed to determine the expression of the resistin gene and protein in the porcine endometrium and myometrium and the adipokine concentrations in the blood plasma and uterine luminal flushings (ULF) during the oestrous cycle and early pregnancy, as well as the effect of resistin on progesterone (P4) and E2 production and steroidogenic acute regulatory protein (StAR), P450 side-chain cleavage enzyme (P450SCC), cytochrome P450C17 (P450C17), cytochrome P450 aromatase (P450AROM; CYP19A1), and 3-hydroxysteroid dehydrogenase (3βHSD) protein abundances in the porcine endometrium collected during early pregnancy and the mid-luteal phase of the cycle. Furthermore, we wanted to ascertain whether the hormone’s mode of action involves the Akt signaling pathway.
Materials and methods
Fig. 1 shows the experimental design of this study.
The experimental design of this study. Akt, protein kinase B; P4, progesterone; E2, oestradiol; StAR, acute regulatory protein; P450SCC, P450 side-chain cleavage enzyme; 3βHSD, 3β-hydroxysteroid dehydrogenase; P450C17, cytochrome P450C17; P450AROM, cytochrome P450 aromatase. Created in BioRender https://BioRender.com/t00t230.
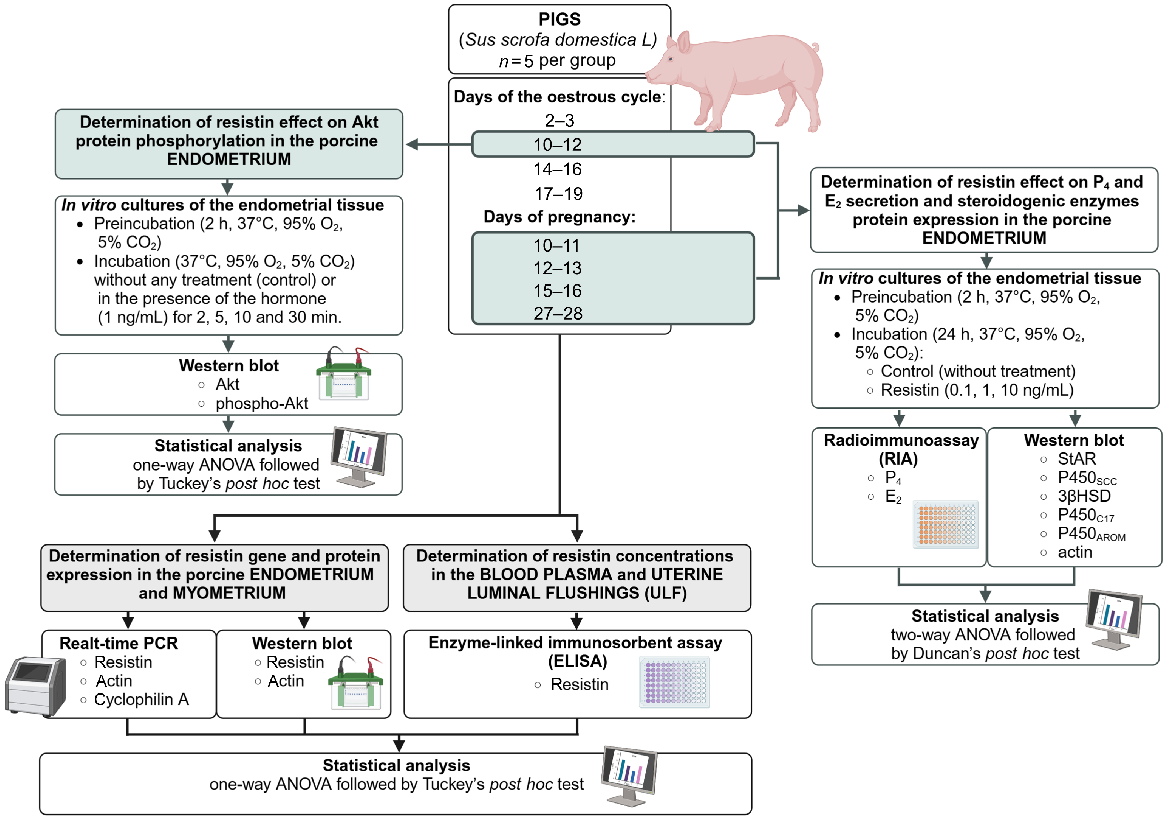
Experimental animals and samples’ collection
All experimental tissues were gained from mature cross-breed gilts (Polish Large White × Polish Landrace × Duroc × Pietrain) descended for commercial slaughter (7–8 months, 130–150 kg) according to the Polish Act on the Protection of Animals used for scientific or educational purposes of the 15 January 2015 (Polish Journal of Law of 2015 item 266) as well as directive 2010/63/EU of the European Parliament of the 22nd of September 2010 on the protection of animals used for a scientific purposes.
Animals were divided into eight groups (n = 5 per group) as follows: non-pregnant animals on days 2–3 (the early luteal phase), 10–12 (the mid-luteal phase), 14–16 (the late luteal phase), and 17–19 (the follicular phase) of the oestrous cycle; and pregnant ones on days 10–11 (the transuterine migration of embryos), 12–13 (the maternal recognition of pregnancy), 15–16 (the beginning of implantation), and 27–28 (the end of implantation). Early pregnancy stages were compared with the mid-luteal phase of the oestrus cycle (days 10–12) during which corpus luteum (CL) activity corresponds to that observed during pregnancy. Therefore, days 10–12 of the oestrous cycle are considered the reference control for the expression/concentration of resistin when embryos are not present in the uterus.
The day of the onset of the second oestrus was assigned as day 0 of the oestrous cycle. Ovarian morphology and P4 levels were used to verify the phase of the cycle (Akins and Morrissette 1968; Nitkiewicz et al. 2012; Dobrzyn et al. 2017). The P4 levels in blood plasma on days 2–3, 10–12, 14–16, and 17–19 of the oestrous cycle were as follows: 4 ± 2, 19 ± 3.4, 8 ± 2.2, and 0.2 ± 0.03 ng/mL, respectively (Nitkiewicz et al. 2012). In the blood plasma of pregnant pigs on days 10–11, 12–13, 15–16, and 27–28 the P4 concentrations were as follows: 16.3 ± 0.7, 14.7 ± 0.5, 12.9 ± 0.9, and 11.7 ± 0.5 ng/mL, respectively (Dobrzyn et al. 2017). Pigs were naturally inseminated by cross-breed boar on days 1–2 of the cycle and the day of pregnancy was confirmed by the presence and morphology of conceptuses in the uterine horns (Anderson 1978). Directly after slaughter, blood samples were collected into heparinised tubes (lithium heparin; Sarstedt, Germany). The uteri were placed into the ice-cold phosphate-buffered saline (PBS) supplemented with 1% antibiotic-antimycotic solution (Sigma-Aldrich, USA) and transported to the laboratory. The ULF samples were harvested by injection and recovering 40 mL of PBS (20 mL/uterine horn). Then, blood plasma and ULF samples were centrifuged (blood: 2500g, 15 min, 4°C; ULF: 3000g, 5 min, 4°C) and frozen at −20°C. After ULF collection, the uterine horns were cut longitudinally on the mesometrial surface and samples of the endometrium and myometrium were collected by dissecting the endometrial fragments with sterile scissors and retracting the myometrium so as not to contaminate the collected samples with other tissue. All tissue fragments were collected from the middle part of the uterine horns.
Proper separation of the endometrium and myometrium was verified using a dissecting microscope with high magnification. All fragments containing residual contamination with other tissue were subjected to additional cleaning by scraping with a sterile scalpel. The obtained tissue sections were then immediately placed in liquid nitrogen and stored at −80°C. The remaining endometrium was intended for in vitro studies.
In vitro cultures of the endometrial tissue
Endometrial tissue slices were in vitro cultured as described by Smolinska et al. (2016) in five independent experiments (n = 5 per group). The tissue explants were harvested from the middle part of the uterine horns of pigs during the above-mentioned days of pregnancy and the mid-luteal phase of the cycle. Endometrial fragments were cut from the remaining endometrium as described above and placed in fresh PBS (without antibiotic-antimycotic solution) on ice. The obtained fragments were then cut into irregular slices (90–110 mg) and put back in fresh PBS on ice. The explants were rinsed three times in phenol red-free M199 medium (Sigma Aldrich, USA) and preincubated (2 h, 37°C, 95% O2, 5% CO2) in 2 mL of phenol red-free M199 medium enriched with 5% new-born calf serum (FBS; Sigma-Aldrich, USA), 0.1% bovine serum albumin (BSA; MP Biomedicals, USA), and 1% antibiotic-antimycotic solution (Sigma-Aldrich, USA).
To determine the influence of resistin on the secretion of P4 and E2 as well as on StAR, P450SCC, 3βHSD, P450C17, and P450AROM protein expression, after preincubation, the explants were incubated for 24 h under the same conditions with the addition of resistin (0.1, 1, or 10 ng/mL; Phoenix Pharmaceuticals, USA) or without any treatment (control). Doses of the hormone used in this study are physiological concentrations observed in porcine blood plasma and ULF and ovarian follicular fluid (Rak-Mardyła et al. 2014). To determine the impact of resistin on the phosphorylation of Akt, endometrial explants harvested from pigs on days 10–12 of the oestrous cycle (n = 5) were incubated in phenol red-free M199 medium without any treatment (control) or in the presence of the hormone (1 ng/mL) for 2, 5, 10, and 30 min. After incubation, all tissue explants and media were collected and stored at −80°C and −20°C, respectively.
RNA isolation, cDNA synthesis and quantitative real-time polymerase chain reaction
Total RNA isolation from uterine tissues was executed using TRI Reagent® RNA Isolation Reagent (Sigma-Aldrich, USA) as described by the manufacturer. After isolation, the purity and quantity of RNA were assessed spectrophotometrically (wavelengths 260 and 280 nm; Infinite M200 PRO, Tecan, Switzerland). Only samples with A260/A280 of >1.8 were used for further analyses. Reverse transcription was carried out using Omniscript RT Kit (Qiagen, USA) with 1 μg of RNA and 0.5 μg oligo(dT)15 primers (Roche, Switzerland) at a final volume of 20 μL (1 h, 37°C; 5 min, 93°C).
Real-time quantitative polymerase chain reaction (RT-qPCR) was conducted using AriaMX (Applied Biosystems, USA). Briefly, cDNA (50 ng) was amplified with RT PCR Mix Probe (A&A Biotechnology, Poland) and specific, pre-designed TaqMan™ probes for RETN and reference genes: actin and cyclophilin A (Table 1) in a total volume of 20 μL. No template controls (NTC) were performed with water instead of cDNA. All samples were amplified in duplicates. Real-time PCR conditions were as follows: initial denaturation at 95° for 3 min, next 40 cycles of denaturation at 95°C for 15 s, and annealing at 60°C for 1 min. To confirm the specificity of RT-qPCR reaction, electrophoresis was performed. The comparative cycle threshold method (ΔΔCT) was used to determine the relative RETN gene expression in comparison to the geometric mean of reference genes’ Ct values (Livak and Schmittgen 2001).
Protein isolation and western blot
Total protein was isolated from tissues using T-PER™ Tissue Protein Extraction Reagent (Thermo Fisher Scientific, USA) with a mix of protease inhibitors (Sigma-Aldrich, USA). After homogenisation on ice, the lysates were centrifuged twice at 4°C, 10,000g for 5 min. Protein concentrations in the supernatants were determined using the Bradford dye-binding procedure with dilutions of BSA as the standards. Western blot analysis was conducted according to Kiezun et al. (2023) with modifications. In brief, the protein samples were separated by sodium dodecyl sulphate polyacrylamide gel electrophoresis (SDS-PAGE) in 12.5% polyacrylamide gels and then transferred onto polyvinylidene difluoride (PVDF) membranes (Merck Millipore, USA). After SEMI-DRY transfer, the membranes were blocked in Tris-buffered saline with Tween-20 (TBST) containing 5% BSA or skimmed milk (1 h, room temperature, details in Table 2). The membranes were then incubated in a specific primary antibody solution detailed in Table 2. Subsequently, the membranes were washed three times for 5 min in TBST and incubated for 1.5 h at room temperature with secondary antibodies conjugated with horseradish peroxidase (HRP; Table 2). The resulting immunoreactive products were visualised using a chemiluminescence HRP substrate (Merck Millipore, USA) according to the manufacturer’s protocol. The immunocomplexes were analysed and archived using Azure 280 Imaging System (Azure Biosystems, USA). The optical density (OD) was determined with Image Studio Lite v.5.2 (LI-COR, USA). Actin protein was used as a reference to determine the relative amounts of resistin, StAR, P450SCC, 3βHSD, P450C17, and P450AROM proteins. Total Akt protein abundances were used to normalise amounts of phosphorylated Akt protein.
Protein | Gels (%) | Host species | Catalogue number and supplier | Primary antibody dilution | Blocking agent | Secondary antibodies | Secondary antibody dilution | |
---|---|---|---|---|---|---|---|---|
Resistin | 12.5 | Mouse | sc-376336 (Santa Cruz Biotechnology, USA) | 1:100 | 5% BSA in TBST | Goat anti-mouse (115-035-003; Jackson ImmunoResearch, USA) | 1:5000 | |
StAR | Rabbit | ab96637 (Abcam, UK) | 1:250 | 5% milk in TBST | Goat anti-rabbit (AP156P; Merck Millipore, USA) | 1:2000 | ||
P450SCC | ab175408 (Abcam, UK) | 1:250 | 1:2000 | |||||
P450C17 | ab125022 (Abcam, UK) | 1:250 | 1:2000 | |||||
P450AROM | A2161 (ABclonal, USA) | 1:250 | 1:2000 | |||||
3βHSD | SAB2101086 (Sigma Aldrich, USA) | 1:200 | 1:2000 | |||||
Actin | A2066 (Sigma Aldrich, USA) | 1:2000 | 1:20,000 | |||||
Akt | #9272 (Cell Signaling Technology, USA) | 1:1000 | 1:20,000 | |||||
phospho-Akt | #9271 (Cell Signaling Technology, USA) | 1:500 | 1:20,000 |
StAR, steroidogenic acute regulatory protein; P450SCC, P450 side-chain cleavage enzyme; P450C17, cytochrome P450c17; P450AROM, cytochrome P450 aromatase; 3βHSD, 3β-hydroxysteroid dehydrogenase; Akt, protein kinase B.
Enzyme-linked immunosorbent assay (ELISA)
Resistin protein concentrations in the blood plasma and ULF were determined using a commercial ELISA kit (ELH-Resistin; RayBiotech Life, USA) as described by the manufacturer. The range of the standard curve was from 2 to 400 pg/mL. The sensitivity of the assay was identified as the lowest concentration that could be recognised from the zero sample (2 pg/mL). As described by the manufacturer, this ELISA kit shows no cross-reactivity with any homologous proteins assayed. The absorbances of obtained complexes were evaluated using Infinite M200 PRO reader with Tecan I-control software (Tecan, Switzerland) at 450 nm. The results were linearised by plotting the log of resistin concentrations in comparison with the log of the OD. Regression analysis was used to define the best-fit line. Intra-assay coefficients of variation of the assay were 4.02 ± 1.01% (the inter-assay coefficients of variations were not determined because the assays were done in one assay).
Radioimmunoassay
Steroids (P4 and E2) concentrations in the media were determined based on radioimmunoassay (Rytelewska et al. 2021). Levels of P4 were determined without extraction, whereas extraction efficiency for the E2 assay was 90.67 ± 0.73%. The standard curve range for P4 was from 1 to 1500 pg/mL, whereas for E2, from 0.5 to 200 pg/mL. The sensitivities of the test for P4 and E2 were 1 and 0.5 pg/mL, respectively. The intra-assay coefficients of variation were 2.82 ± 1.03% for P4 and 2.81 ± 0.66% for E2.
Statistical analysis
All statistical analyses were completed using Statistica software (StatSoft, USA). Differences in expression or concentrations of resistin in the endometrium, myometrium, blood, and ULF during the oestrous cycle and early pregnancy, as well as Akt protein phosphorylation under the impact of resistin, were evidenced using one-way ANOVA followed by Tuckey’s post hoc test. The results regarding the influence of resistin on the steroidogenesis process in the endometrium were analysed using two-way ANOVA with two independent factors: phase/stage (days of the cycle or pregnancy) and resistin (at the doses of 0.1, 1, and 10 ng/mL), and the interaction of main factors (phase/stage × resistin). The two-way ANOVA was followed by Duncan’s post hoc test. Values for P < 0.05 were considered statistically significant. All obtained results were presented as mean ± standard error of the mean (s.e.m.) from five independent observations (n = 5 per group).
Results
Gene and protein expression of resistin in the porcine endometrium
During the oestrous cycle, the RETN gene expression was higher on days 14–16 of the cycle compared to days 2–3 and 17–19 (Fig. 2a; P < 0.05). Higher amounts of resistin protein in the endometrial explants were observed on days 2–12 compared to days 14–16 of the cycle (Fig. 2b; P < 0.05).
Resistin gene and protein expression in the porcine endometrium during the oestrous cycle and early pregnancy. A comparison of resistin mRNA (quantitative real-time PCR; a, c, e) and protein (western blot; b, d, f) abundances in the porcine endometrium between days 2–3, 10–12, 14–16, and 17–19 of the oestrous cycle (a, b), between days 10–11, 12–13, 15–16, and 27–28 of pregnancy (c, d), and between days 10–12 of the oestrous cycle and days 10–11, 12–13, 15–16, and 27–28 of pregnancy (e, f). Upper panels – representative immunoblots; lower panels – densitometric analysis of resistin protein relative content normalised with the actin protein. Results are presented as means ± s.e.m. (n = 5). Statistically significant differences are presented by different superscripts (P < 0.05). RETN, resistin.
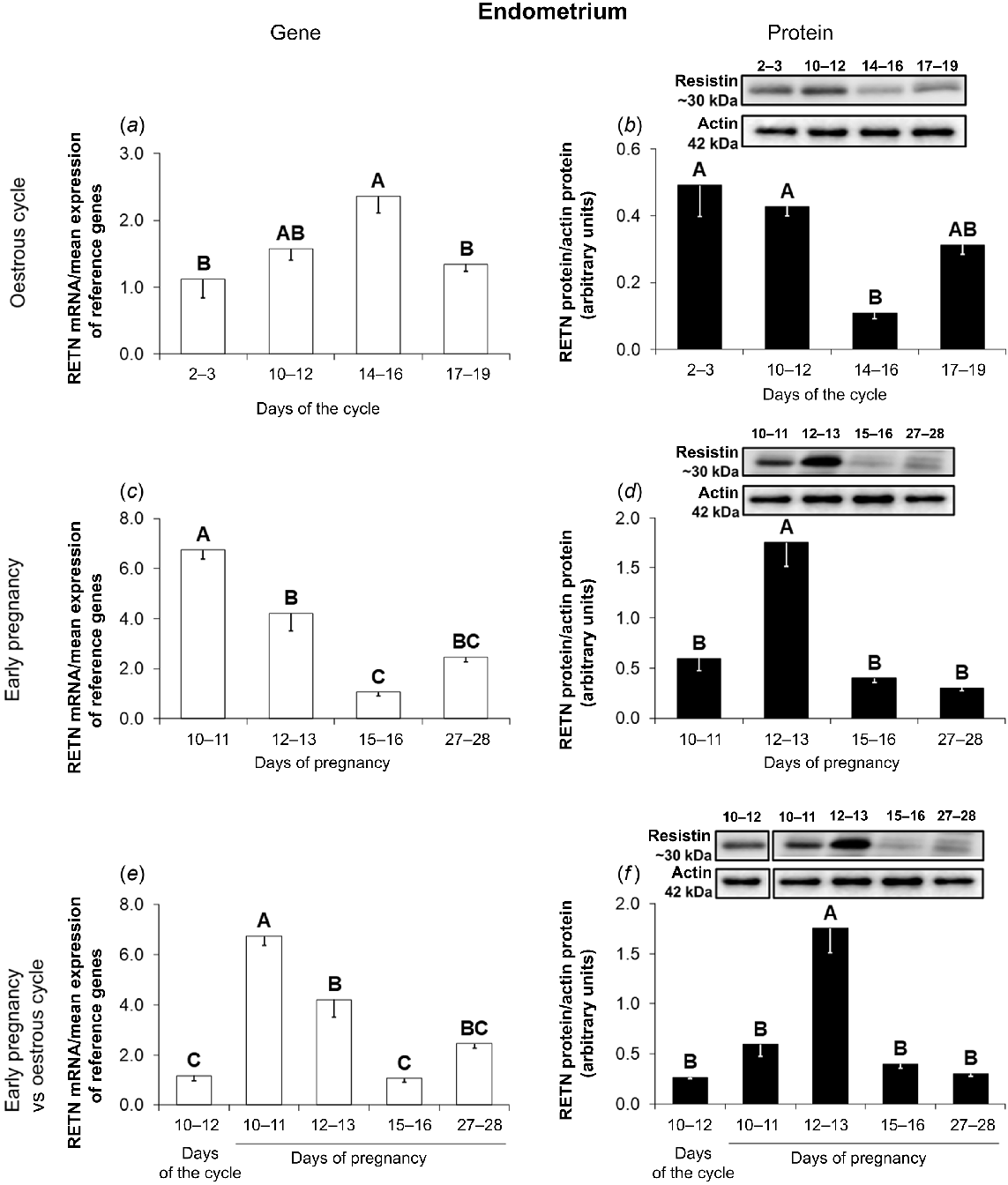
During early pregnancy, the endometrial expression of the RETN gene was the highest on days 10–11 and the lowest on days 15–16 (Fig. 2c; P < 0.05). The expression of resistin protein in the endometrium of pregnant pigs was higher on days 12–13 of gestation in comparison with other periods of pregnancy (Fig. 2d; P < 0.05).
The comparison of the RETN gene expression during early pregnancy with that observed on days 10–12 of the oestrous cycle shows higher abundances of resistin mRNA on days 10–13 of pregnancy in relation to the mid-luteal phase of the cycle (Fig. 2e; P < 0.05). Significantly higher amounts of resistin protein were noted in the endometrium on days 12–13 of pregnancy compared to days 10–12 of the oestrous cycle (Fig. 2f; P < 0.05).
Gene and protein expression of resistin in the porcine myometrium
In the myometrium during the oestrous cycle, the RETN mRNA content was the highest on days 10–12, lower on days 17–19, and the lowest on days 2–3 and 14–16 of the cycle (Fig. 3a; P < 0.05). The adipokine protein abundance was at the same level throughout the oestrous cycle (Fig. 3b; P < 0.05).
Resistin gene and protein expression in the porcine myometrium during the oestrous cycle and early pregnancy. A comparison of resistin mRNA (quantitative real-time PCR; a, c, e) and protein (western blot; b, d, f) abundances in the porcine myometrium between days 2–3, 10–12, 14–16, and 17–19 of the oestrous cycle (a, b), between days 10–11, 12–13, 15–16, and 27–28 of pregnancy (c, d), and between days 10–12 of the oestrous cycle and days 10–11, 12–13, 15–16, and 27–28 of pregnancy (e, f). Upper panels – representative immunoblots; lower panels – densitometric analysis of resistin protein relative content normalised with the actin protein. Results are presented as means ± s.e.m. (n = 5). Statistically significant differences are presented by different superscripts (P < 0.05). RETN, resistin.
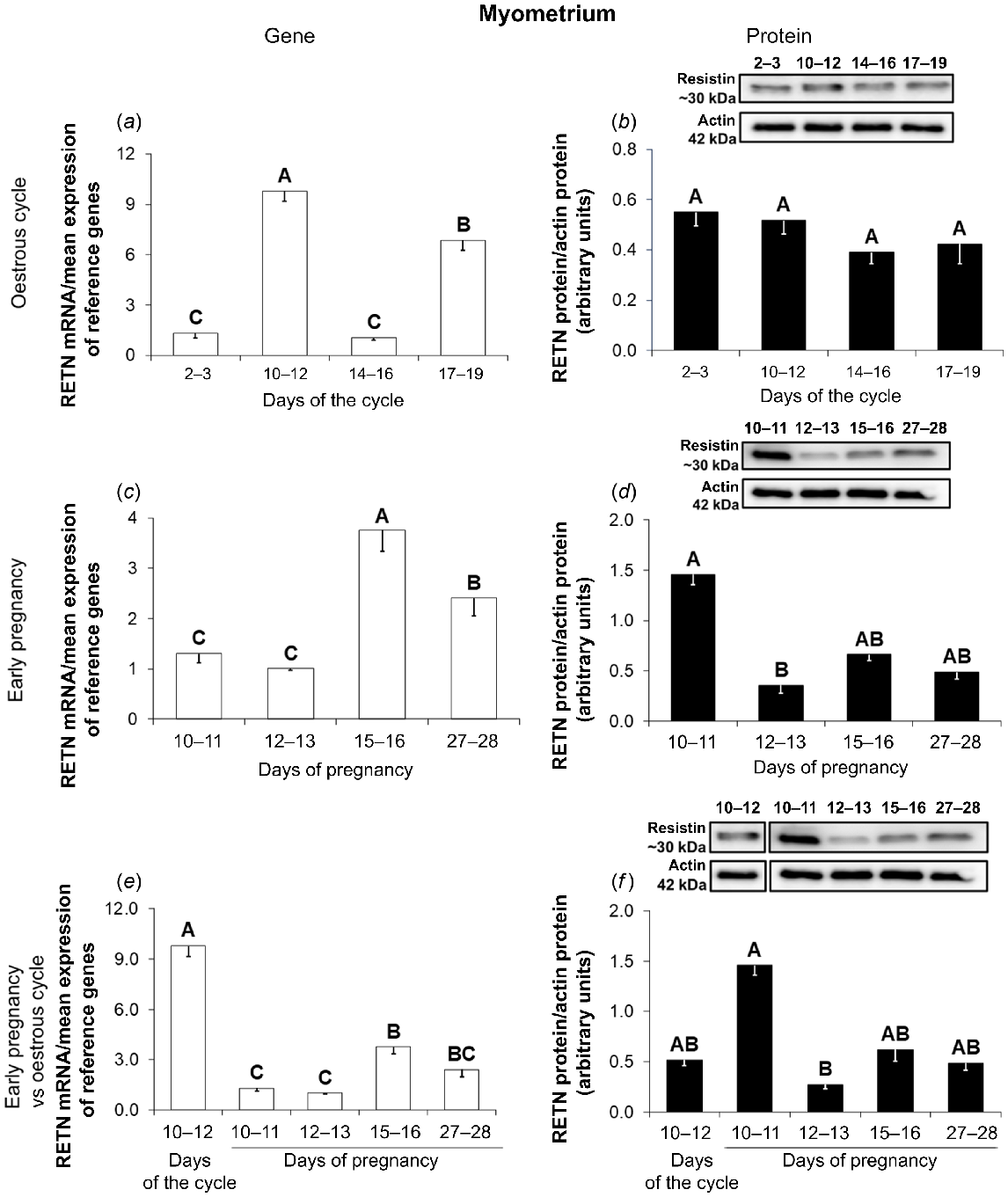
During gestation, the expression of the resistin gene was the highest on days 15–16, lower on days 27–28, and the lowest on days 10–13 of pregnancy (Fig. 3c; P < 0.05). A western blot analysis showed that the expression of the hormone protein was higher on days 10–11 compared to days 12–13 of pregnancy (Fig. 3d; P < 0.05).
Higher expression of the RETN gene was observed on days 10–12 of the oestrous cycle in comparison with all periods of early pregnancy (Fig. 3e; P < 0.05). In the case of resistin protein, there were no differences in the expression between pregnant and non-pregnant animals (Fig. 3f; P < 0.05).
Resistin concentrations in the porcine blood plasma
During the oestrous cycle, resistin concentrations in the porcine blood plasma were on the same level regardless of the cycle phase (Fig. 4a; P < 0.05). During early pregnancy, the highest hormone levels were observed on days 12–13, lower on days 15–16, and the lowest on days 10–11 and 27–28 (Fig. 4b; P < 0.05). A comparison of early pregnancy with the mid-luteal phase of the cycle showed differences in the hormone concentrations between pregnant and non-pregnant pigs. Higher levels of adipokine were noted on days 12–13 of pregnancy compared to days 10–12 of the oestrous cycle. In contrast, concentrations of resistin on days 10–11 and 27–28 of pregnancy were lower than that observed during the mid-luteal phase of the cycle (Fig. 4c; P < 0.05).
Resistin concentrations in the porcine blood plasma and uterine luminal flushings during the oestrous cycle and early pregnancy. A comparison of resistin levels (ELISA) in the blood plasma between days 2–3, 10–12, 14–16, and 17–19 of the oestrous cycle (a), between days 10–11, 12–13, 15–16, and 27–28 of pregnancy (b), and between days 10–12 of the oestrous cycle and days 10–11, 12–13, 15–16, and 27–28 of pregnancy (c), as well as in the uterine luminal flushings (ULF) between phases of the oestrous cycle (d), between periods of early pregnancy (e), and between days 10–12 of the oestrous cycle and periods of early pregnancy (f). Results are presented as means ± s.e.m. (n = 5). Statistically significant differences are presented by different superscripts (P < 0.05).
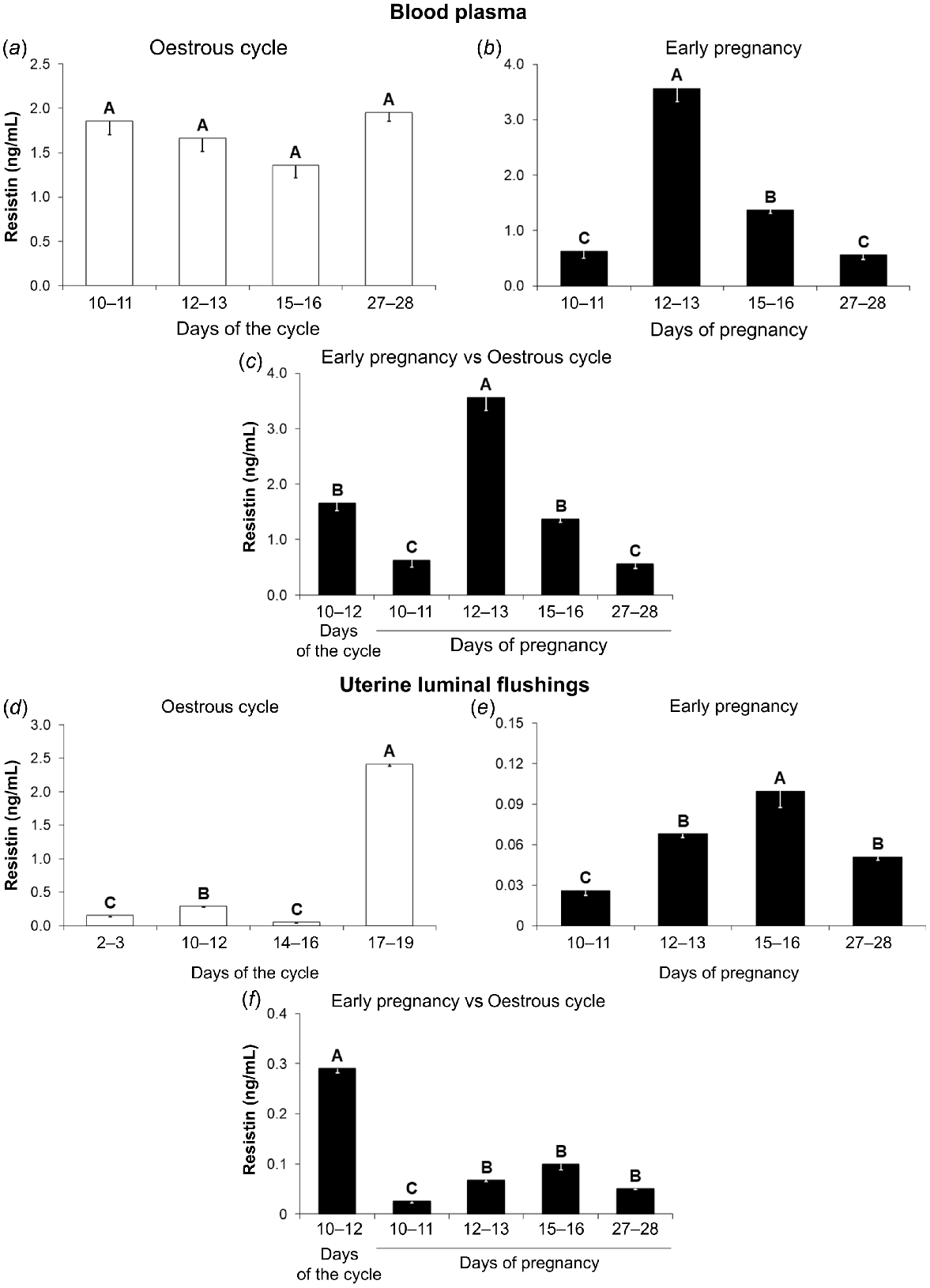
Resistin concentrations in the porcine ULF
During the oestrous cycle, the highest concentrations of resistin in ULF were observed on days 17–19, lower on days 10–12, and the lowest on days 2–3 and 14–16 (Fig. 4d; P < 0.05). Concentrations of resistin in ULF of pregnant animals were the highest on days 15–16, lower on days 12–13 and 27–28, and the lowest on days 10–11 of pregnancy (Fig. 4e; P < 0.05). A comparison of days 10–12 of the oestrous cycle with early pregnancy showed enhanced resistin concentrations in ULF during the cycle compared to all studied periods of pregnancy (Fig. 4f; P < 0.05).
The effect of resistin on P4 and E2 secretion by the porcine endometrium
Even though there was no independent effect of resistin on the P4 secretion, the interaction between both main factors was observed (Supplementary Table S1). Consequently, the post hoc test was used. On days 12–13 and 27–28 of gestation, concentrations of P4 were higher in media after incubation in the presence of resistin (1 and 0.1 ng/mL, respectively). The secretion of P4 was decreased under the influence of resistin on days 10–11 (1 ng/mL) and 15–16 (0.1 ng/mL) of gestation (Fig. 5a; Table S1; P < 0.05). Basal secretion of this hormone was lower on days 12–13 of gestation in comparison with other periods of pregnancy and days 10–12 of the cycle (Fig. 5a; Table S1; P < 0.05).
The impact of resistin on P4 and E2 secretion. The effect of resistin (0.1, 1, and 10 ng/mL) on the secretion of progesterone (P4; a) and oestradiol (E2; b) by the in vitro incubated endometrium from pigs during early pregnancy (days 10–11, 12–13, 15–16, and 27–28) and on days 10–12 of the oestrous cycle. Concentrations of P4 and E2 in media were measured using radioimmunoassay analysis. Results are presented as means ± s.e.m. (n = 5). Statistically significant differences are presented by different superscripts (P < 0.05). C, control; R0.1, resistin 0.1 ng/mL; R1, resistin 1 ng/mL; R10, resistin 10 ng/mL.
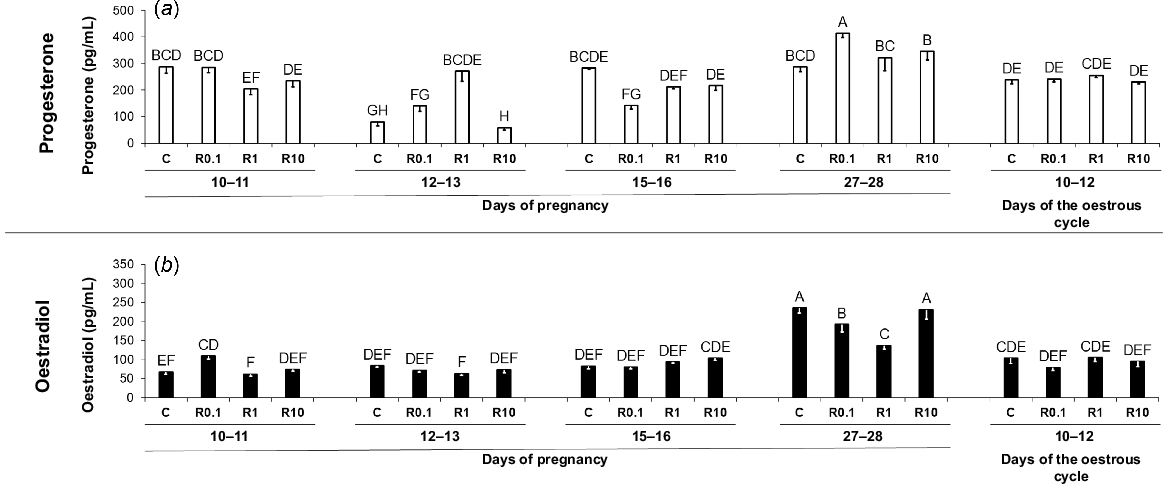
In the endometrial tissue explants incubated in the presence of resistin the production of E2 was increased on days 10–11 (0.1 ng/mL) of gestation. On days 27–28 of pregnancy, a lower secretion of E2 by endometrial tissue was observed under the impact of resistin (0.1 and 1 ng/mL; Fig. 5b; Table S2; P < 0.05). Basal production of this steroid was higher on days 27–28 of pregnancy compared to the other analysed periods (Fig. 5b; Table S2; P < 0.05).
The effect of resistin on StAR, P450SCC, 3βHSD, P450C17, and P450AROM proteins abundances and Akt protein phosphorylation in the porcine endometrium
On days 12–13 of gestation, as well as on days 10–12 of the cycle, resistin (all doses) decreased the amounts of StAR protein in the endometrium (Fig. 6a; Table S3; P < 0.05). Basal expression of StAR protein was the highest on days 10–12 of the oestrous cycle, lower on days 12–13 of pregnancy, and the lowest on days 10–11 and 15–28 of pregnancy (Fig. 6a; Table S3; P < 0.05).
The impact of resistin on StAR, P450SCC and 3βHSD protein expression. The effect of resistin (0.1, 1, and 10 ng/mL) on protein abundances (western blot) of steroidogenic acute regulatory protein (StAR; a), P450 side-chain cleavage enzyme (P450SCC; b), and 3β-hydroxysteroid dehydrogenase (3βHSD; c) in the in vitro-incubated endometrium from pigs during early pregnancy (days 10–11, 12–13, 15–16 and 27–28) and on days 10–12 of the oestrous cycle. Upper panels, representative immunoblots; lower panels, densitometric analysis of target proteins’ relative content normalised with the actin protein. Results are presented as means ± s.e.m. (n = 5). Statistically significant differences are presented by different superscripts (P < 0.05). C, control; R0.1, resistin 0.1 ng/mL; R1, resistin 1 ng/mL; R10, resistin 10 ng/mL.
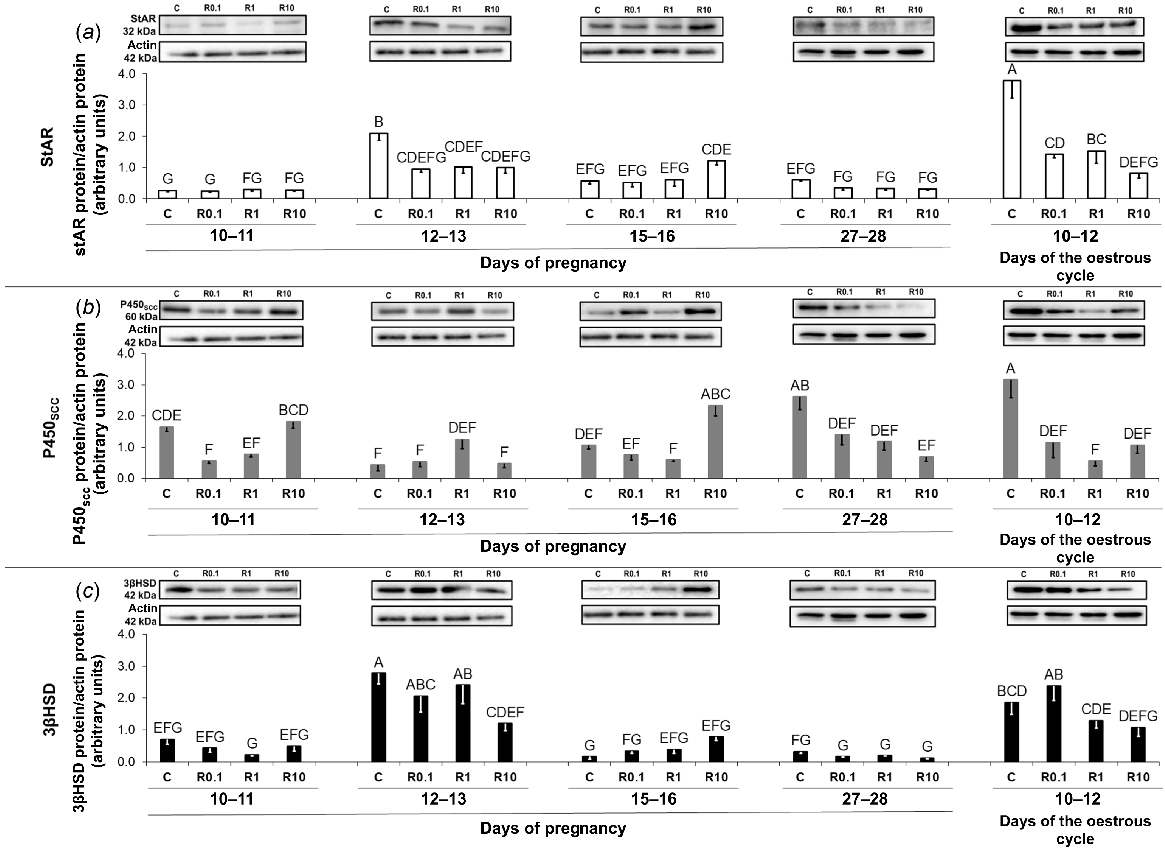
Amounts of P450SCC protein were higher under the impact of resistin (10 ng/mL) on days 15–16 of pregnancy. Expression of P450SCC protein was decreased by the adipokine in the endometrium harvested on days 10–11 (0.1 ng/mL) and 27–28 (all doses) of pregnancy, as well as on days 10–12 of the oestrous cycle (all doses; Fig. 6b; Table S4; P < 0.05). Basal P450SCC protein amounts were higher on days 10–12 of the cycle and 27–28 of pregnancy compared to the remaining periods of pregnancy (Fig. 6b; Table S4; P < 0.05).
Despite the absence of an independent effect of resistin on 3βHSD protein amounts, the interaction between both main factors was observed (Table S5), thus the post hoc test was conducted. The adipokine (10 ng/mL) reduced the enzyme protein abundance on days 12–13 of gestation (Fig. 6c; Table S5; P < 0.05). Basal 3βHSD protein expression was the highest on days 12–13 of pregnancy, lower on days 10–12 of the cycle, and the lowest on days 10–11 and 15–28 of gestation (Fig. 6c; Table S5; P < 0.05).
Similarly to P4 and 3βHSD, no independent effect of resistin on the amount of P450C17 protein was noted, but an interaction between both main factors was observed (Table S6), therefore a post hoc test was performed. The abundances of the enzyme protein were higher under the influence of resistin (10 ng/mL) on days 15–16 of pregnancy (Fig. 7a; Table S6; P < 0.05). During the mid-luteal phase of the cycle, resistin (all doses) decreased the amounts of P450C17 protein (Fig. 7a; Table S6; P < 0.05). Basal P450C17 protein expression was the highest on days 10–12 of the oestrous cycle, lower on days 15–16 of pregnancy, and the lowest during the remaining periods of pregnancy (Fig. 7a; Table S6; P < 0.05).
The impact of resistin on P450C17 and P450AROM protein expression. The effect of resistin (0.1, 1, and 10 ng/mL) on protein abundances (western blot) of cytochrome P450C17 (P450C17; a) and cytochrome P450 aromatase (P450AROM; b) in the in vitro-incubated endometrium from pigs during early pregnancy (days 10–11, 12–13, 15–16, and 27–28) and on days 10–12 of the oestrous cycle. Upper panels, representative immunoblots; lower panels, densitometric analysis of target proteins’ relative content normalised with the actin protein. Results are presented as means ± s.e.m. (n = 5). Statistically significant differences are presented by different superscripts (P < 0.05). C, control; R0.1, resistin 0.1 ng/mL; R1, resistin 1 ng/mL; R10, resistin 10 ng/mL.
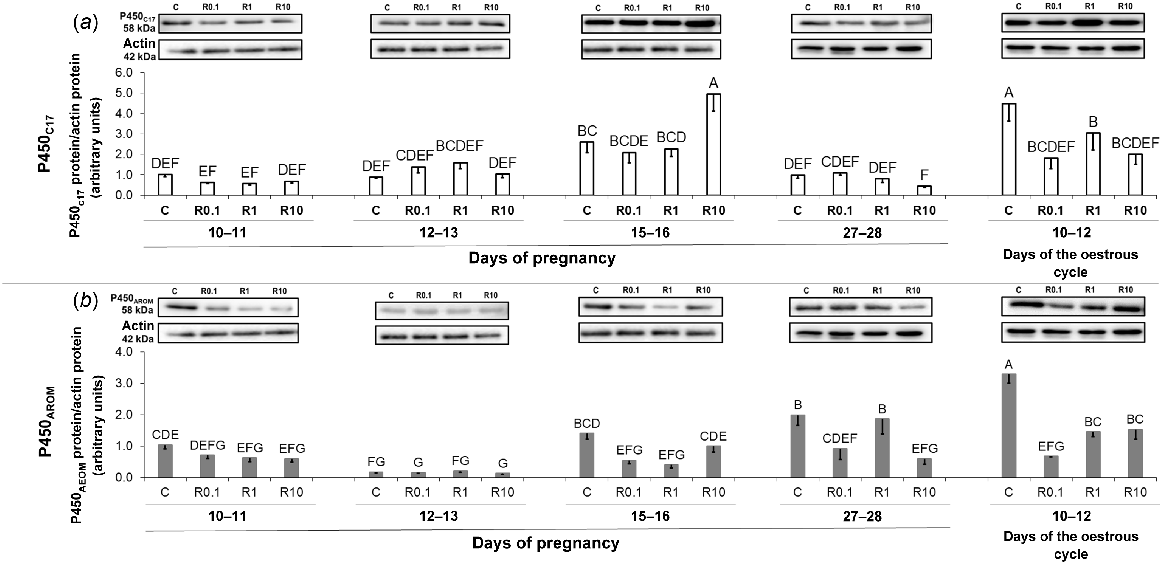
Resistin caused a drop in the P450AROM protein abundance on days 15–16 (0.1 and 1 ng/mL) and 27–28 (0.1 and 10 ng/mL) of gestation as well as during the oestrous cycle (all doses; Fig. 7b; Table S7; P < 0.05). Basal P450AROM protein abundances were the highest on days 10–12 of the oestrous cycle, whereas the lowest on days 12–13 of pregnancy (Fig. 7b; Table S7; P < 0.05).
Resistin increased the phosphorylation of Akt protein after 2, 5, and 10 min of incubation in comparison with the control group (Fig. 8; P < 0.05).
The impact of resistin on the activation of protein kinase B (Akt) signaling pathway. The effect of resistin (1 ng/mL) on phosphorylation of Akt protein in the in vitro-incubated endometrium from pigs on days 10–12 of the oestrous cycle. Upper panels, representative immunoblots; lower panels, densitometric analysis of phospho-Akt protein relative content normalised with the total Akt protein. Results are presented as means ± s.e.m. (n = 5). Statistically significant differences are presented by different superscripts (P < 0.05).
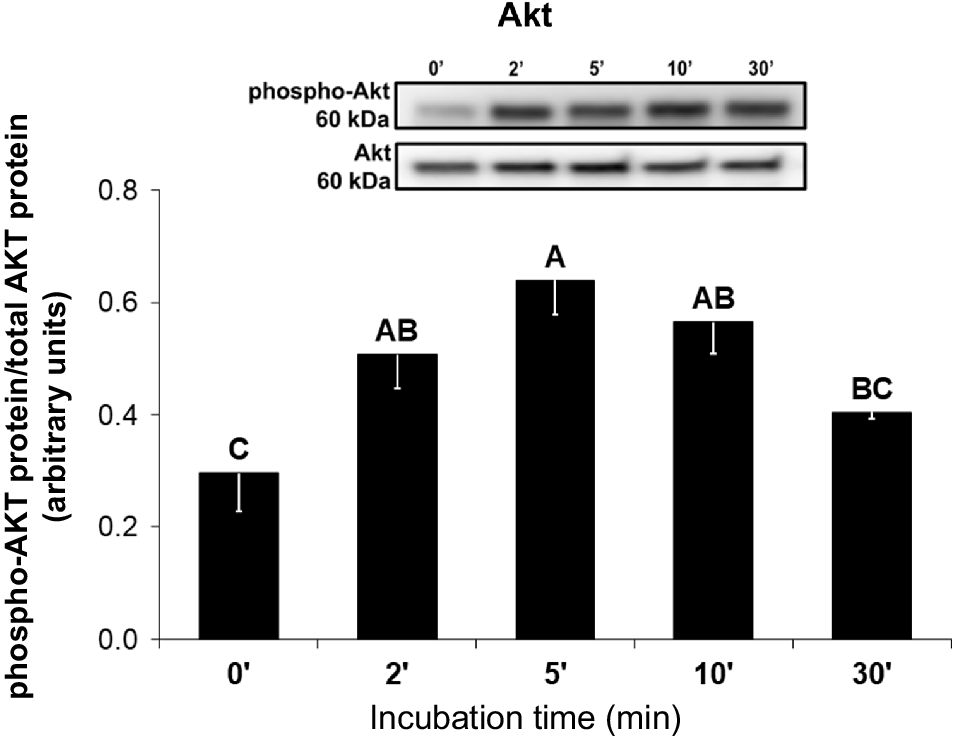
Discussion
The adipose tissue, the primary organ for storing energy, generates several hormones that impact uterine functioning. An overview of the literature on the expression of adipokines and their receptors in the endometrium of rodents, humans, and pigs can be found in Reverchon et al. (2014). To our knowledge, this is the first publication to demonstrate the presence of resistin mRNA and protein in the uterine tissues, as well as the concentrations of adipokine in the blood plasma and ULF of domestic pigs during the oestrous cycle and early pregnancy. For now, a few reports are showing the expression of resistin or its role in the functioning of the uterus. Resistin is an adipokine that has so far been found only in the uterus of women and sheep (Oh et al. 2017; Dall’Aglio et al. 2019). Dall’Aglio et al. (2019) found that the resistin protein abundance in the ovine uterus depends on the nutritional status of these animals, further supporting the relationship between nutritional status and reproductive activity. The presented study noted that the abundance of resistin mRNA and protein in the porcine uterine tissues and the hormone concentrations in the blood plasma and ULF vary during the cycle and early pregnancy. This indicates that the hormonal environment influences the adipokine levels during the cycle and early pregnancy. Rak et al. (2015a) noted that T and E2 increased RETN expression in the porcine ovarian follicles, whereas P4 had no impact on the hormone mRNA abundance. Moreover, the authors also observed a stimulatory effect of T, P4, and E2 on resistin protein expression in ovarian follicles and the hormone levels in the culture medium (Rak et al. 2015a). Results of this study show that the highest resistin concentrations during the oestrous cycle in ULF were during the follicular phase. This stage of the cycle is characterised by increasing levels of E2, prostaglandin F2α (PGF2α), and follicle-stimulating hormone (FSH) in circulation (Ka et al. 2018), as well as elevated E2 concentrations in porcine endometrial cytoplasmic extracts (Deaver and Guthrie 1980). Moreover, higher protein expression in the endometrial tissue and concentrations of resistin in blood plasma were observed during the maternal recognition of pregnancy. Concentrations of the hormone in ULF increased on days 12–13 of pregnancy and persisted in the further examined periods. In pigs, this period of pregnancy is characterised by higher P4, oestrogens, prostaglandins, calcium, and protein levels in ULF (Geisert et al. 1982; Dobrzyn et al. 2017). In addition to E2, elongating porcine embryos also secrete interleukin 1β2 (IL1B2), interferon δ (IFND), and interferon γ (IFNG) (Ka et al. 2018), which may also influence resistin levels. Increased resistin concentrations when those hormones levels are high supports the consideration that resistin expression is hormonally related. The overlapping pattern of resistin expression in the endometrium with its concentration in circulation and ULF during maternal recognition of pregnancy confirms the statement that the uterus is one of the sources of resistin. Both the oestrous cycle and early pregnancy involve significant immune system activity in the endometrium, with lymphocytes, neutrophils, macrophages, and plasma cells present in varying numbers depending on the phase of the cycle or period of gestation (Bischof et al. 1995; Kaeoket et al. 2002). This suggests that immune cells may serve as an additional source of resistin in the ULF. Previous studies have shown an increased number of endometrial leucocytes in pregnant pigs compared to non-pregnant animals (Bischof et al. 1995). Resistin is known to be secreted by immune cells (Li et al. 2021), including porcine alveolar macrophages (Hua et al. 2023). This could partially explain the observed differences in resistin concentrations in ULF between the oestrous cycle and early pregnancy, as well as between pregnant and non-pregnant animals. Notably, resistin concentrations in ULF during pregnancy were significantly lower than in the mid-luteal phase of the cycle. Since resistin functions as a proinflammatory factor (Estienne et al. 2019), it is plausible that the molecules produced by developing porcine embryos, such as IL1B2, IFND, IFNG, and E2, suppress resistin secretion to prevent embryonic rejection and ensure successful non-invasive implantation. Further research is necessary to determine if and how resistin expression depends on a pig’s endogenous hormone profile.
In the present study, the expression pattern of the resistin protein differs from the expression of the RETN gene. Other researchers also found variations in gene and corresponding protein expression patterns (Gygi et al. 1999; Gry et al. 2009). Although the exact causes of these differences are unknown, intracellular feedback that controls gene expression, variations in the stability of mRNAs and proteins, and the post-transcriptional processing of mRNA may be the reason (Gygi et al. 1999; Gry et al. 2009; Li et al. 2014). Changes in expression depending on the day of the cycle and/or pregnancy, and alternating high and low expression, particularly of a gene, may be related to the feedback effect of gene expression in response to high mRNA levels (Gry et al. 2009; Li et al. 2014). Gene expression levels indicate the tissue’s capacity to synthesise the protein rather than the absolute amount of protein.
The oestrous cycle and early pregnancy in pigs are characterised by dynamic changes, often occurring very suddenly (Oestrup et al. 2009; Soede et al. 2011). During the oestrous cycle, the uterus undergoes cyclical changes to properly prepare for the reception of embryos. The developing CL generates an increasing amount of P4 with a peak in concentration during the mid-luteal phase of the cycle (Anderson 2009; Ka et al. 2018) and inhibiting gonadotrophin secretion (Soede et al. 2011). This is all related to CL activity or, if its functioning is not maintained, to its luteolysis. Moreover, it is known that during the oestrous cycle the content of receptors for E2, P4, and T changes in both the endometrium and myometrium (Koziorowski et al. 1984). This may also be the reason for the variable influence of, in this case, steroid hormones on resistin expression in the porcine uterus. A similar phenomenon may apply to other hormones, not only steroids. It is possible that the aforementioned hormones can affect resistin mRNA abundances, but this requires more research.
The ability of porcine embryos to synthesise and secrete oestrogens is especially important because mainly E2 is a signal that informs maternal organisms about conceptuses’ presence in the uterus in pigs (Geisert et al. 1990). Recent studies conducted on CYP19A1 –/– knockout porcine embryos showed sustain of CL functioning and embryo survival until days 27–30 of pregnancy (Meyer et al. 2019). In addition to embryos, the endometrium may also be a compensating source of oestrogens released into the uterine environment (Franczak and Kotwica 2008; Kaczynski et al. 2023) and that may be related to CYP19A1 –/– knockout embryos subsistence pending the end of the implantation process. Resistin caused enhanced secretion of E2 by the porcine endometrium on days 10–11 of pregnancy, just before maternal recognition of pregnancy. However, on days 15–28 of pregnancy, resistin downregulates protein abundances of P450AROM, an enzyme catalysing the conversion of T to E2. This indicates that resistin may be one of the factors involved in the assurance of appropriate conditions for fertilisation by affecting E2 and P4 secretion from endometrial cells and by that, supporting pregnancy establishment.
Although the prevalence of uterine steroidogenesis has been established for a long time, its regulation remains unclear. Franczak and Kotwica (2008) demonstrated the ability of both porcine endometrium and myometrium to produce E1, E2, A4, and T (Franczak 2008; Franczak and Kotwica 2008). Production of steroid hormones by the uterus may support embryonic steroidogenesis, and thus uterine receptivity and development of uterine glands, as well as uterine responsiveness to other hormones (Tarleton et al. 1999; Hu et al. 2003; Blitek et al. 2007). In our previous study, we determined the expression of StAR, P450SCC, 3βHSD, P450C17, and P450AROM in the porcine endometrium (Gudelska et al. 2020). However, steroid hormones in the uterus were also demonstrated in other species. Mann et al. (2007) discovered that bovine endometrial tissue stores E2. The equine endometrium can metabolise P4 to E2 (Goff et al. 1993). Schiff et al. (1993) demonstrated that the P450SCC gene is also expressed in cells of the mouse uterus, the decidua. Furthermore, in the disease-free human endometrium, mRNA levels of aromatase and 17β-hydroxysteroid dehydrogenase (17βHSD) type 1, which catalyses the conversion of E1 to E2, were extremely low. In contrast, mRNA levels of enzymes involved in converting E2 into less active metabolites, such as 17βHSD types 2 and 4, oestrogen sulfotransferase (EST), and steroid sulfatase (STS), were high. Moreover, P4 enhanced the expression of 17βHSD type 2 and EST during the in vitro study (Dassen et al. 2007). Since resistin is expressed in the uterine tissues of pigs, we investigated the mechanism underlying resistin activity in the endometrium. We found that uterine steroid hormone production changed under the resistin impact. This study shows, for the first time, how resistin affects the secretion of P4 and E2, as well as the amount of StAR, P450SCC, 3βHSD, P450C17, and P450AROM proteins in the endometrium of pigs throughout the early pregnancy and mid-luteal phase of the oestrous cycle. In research conducted by Maillard et al. (2011), the adipokine decreased P4 and E2 secretion by bovine G cells. Studies by Spicer et al. (2011) showed inhibition of steroidogenesis in Th and G cells from cattle ovarian follicles under the impact of adipokine. Resistin reduced P4 and E2 production in human G cells in response to IGF-1 but not FSH (Reverchon et al. 2013). Furthermore, resistin increased basal P4 secretion in cultured rat G cells but did not affect E2 production (Maillard et al. 2011). Resistin enhanced P4, A4, and T secretion and levels of P450SCC, 3βHSD, P450c17, and 17βHSD mRNAs and proteins, whereas the hormone had no effects on E2 secretion and P450AROM expression in the prepubertal porcine ovarian follicles (Rak-Mardyła et al. 2013). In the porcine luteal cells, resistin inhibited P4 and stimulated E2 secretion by affecting steroidogenic enzymes’ expression (Kurowska et al. 2021). In brief, the effect of resistin on ovarian steroidogenesis seems to be tissue- and species-dependent. The action of resistin on the secretion of E2 and P4 may be associated with providing appropriate conditions for fertilisation, and subsequent establishment of pregnancy.
Most of the research shows the inhibitory effect of resistin on E2 production; however, the influence of the adipokine on P4 secretion varies between species (Maillard et al. 2011; Spicer et al. 2011; Rak-Mardyła et al. 2013; Reverchon et al. 2013; Kurowska et al. 2021). In the present study, uterine synthesis of P4 during the transuterine migration of embryos and at the beginning of implantation was reduced under the impact of resistin. In contrast, during the maternal recognition of pregnancy and at the end of implantation, P4 release was higher in tissues incubated in the presence of the adipokine. The effect of resistin on E2 production was opposed. The adipokine increased the steroid levels on days 10–11 of gestation, whereas on days 27–28 of pregnancy, E2 levels were decreased under the impact of resistin. This may depend on differences in the uterine environment that occur during these periods of pregnancy in the pig. Pregnancy is a complex process in which many factors are involved. Disruption in the production of any of them may lead to failure in pregnancy establishment. During the maternal recognition of pregnancy in pigs, the key role is played by E2 secreted by developing embryos. In the porcine uterus, resistin had no impact on endometrial E2 release during this stage of gestation. It can imply that resistin does not belong to factors, which can directly affect this very meaningful process. The development of endometrial receptivity for embryo implantation is under the strong influence of P4. Consistently, the exposition of the endometrium to P4 has a paradoxical effect of downregulating P4 receptor (PGR) expression in the luminal (LE) and glandular (GE) epithelium (Geisert et al. 1994). In the present study, we noted that basal production of P4 by endometrium was the lowest on days 12–13 of pregnancy and that resistin increased the secretion of P4 on those days, which could be related to supporting the ovarian production of P4, and by that, to maintaining proper levels of the steroid to prepare the uterus for implantation. Subsequently, on days 15–16 of gestation, resistin inhibited P4 secretion. This may be intended to prevent too high hormone concentrations because hormonal homeostasis during early pregnancy is crucial for reproductive performance.
Munir et al. (2005) noted that CYP17A1 expression and P450C17 activity – a measure of ovarian hyperandrogenism in PCOS females – were improved by resistin alone or by resistin, forskolin, and insulin together. In the porcine endometrium, resistin increased the amounts of P450C17 on days 15–16 of pregnancy and decreased amounts of the enzyme during the mid-luteal phase of the oestrous cycle. We propose that the resistin effect on the production of steroid hormones is species- and tissue-specific based on the findings of this study and earlier published investigations. The existence of distinct resistin isoforms could account for these contradicting results and the functional variety of resistin across species. Neither the resistin receptor nor the mechanism of the hormone activity in the porcine uterus are known. It can be suggested that local production of P4 and E2 in the porcine uterus might be inversely correlated, with uterine-derived P4 potentially providing endometrial protection against the development of pathological conditions in pigs. This may explain the differing effects of resistin on P4 and E2 production observed in this experiment. Resistin stimulates the secretion of P4, possibly by 17βHSD type 2 and EST, which inhibits the formation of E2. However, such a hypothesis requires more research. Steroid hormones are synthesised on an ongoing basis depending on demand and are not stored in the cell (Bremer and Miller 2014). Therefore, it can be assumed that resistin affects the de novo synthesis of steroid hormones.
In this study, the distinction in resistin effect on steroidogenic enzyme abundances and P4 and E2 concentration patterns could be explained by the difference between enzyme protein expression and its activity as well as post-translational modification of proteins. Moreover, increased protein expression does not always reflect its enzymatic activity. The expressed protein may have little or no ability to convert substrate into a suitable product. There is also a feedback loop that inhibits the expression or activity of steroidogenic enzymes when the concentration of the resulting hormone is too high. It is known that the activity of 3βHSD is reduced under the impact of P4 in rat ovaries (Tanaka et al. 1993) or by androgens in rat testicular Leydig cells (Fanjul et al. 1992). Furthermore, conceptuses could also be a source of other steroid hormones, not only E2, which may be transformed by enzymes present in the endometrium or they may influence the secretion of others. Kaczynski et al. (2021) determined that intrauterine administration of E2 provoked intensified levels of P4 observed in the porcine CLs. To exclude this possibility, research should be conducted on other steroid hormones.
The Akt signaling pathway is known to be involved in the process of steroidogenesis. It was convincingly shown that resistin at the dose of 10 ng/mL promoted the activation of many kinases, including Akt, MAPKs, p38, and ERK1/2, in cultured bovine and rat G cells (Maillard et al. 2011). Resistin (10 ng/mL) increased Stat-3, Akt, and ERK1/2 phosphorylation in cultured porcine ovarian follicular cells (Rak et al. 2015b). Moreover, the adipokine reduced indicators of apoptosis, such as gene expression of pro-apoptotic genes, caspase activity, and DNA fragmentation, most likely through the ERK1/2, JAK/STAT and PI3K signaling pathways (Rak et al. 2015b). The results obtained in this experiment are in agreement with those previously described. Resistin (1 ng/mL) enhanced the abundance of the phosphorylated Akt protein in the porcine endometrium. Although during the mid-luteal phase of the oestrous cycle resistin did not significantly affect the secretion of P4 and E2, it inhibited the expression of StAR, P450SCC, 3βHSD, P450C17, and P450AROM proteins. Thus, the influence of resistin on the Akt pathway might be connected to the adipokine’s influence on the steroidogenic enzyme activity or other steroid hormones’ production in the endometrial cells.
Conclusion
This study revealed that resistin is present in the porcine uterus, blood plasma, and ULF during the oestrous cycle and early pregnancy. Moreover, we demonstrated that resistin mRNA and protein abundances and concentrations of the hormone in blood plasma and ULF are dependent on the phase of the oestrous cycle and/or period of early pregnancy. These findings suggest that the hormonal status of pigs may be related to this phenomenon. Furthermore, resistin affects P4 and E2 production and expression of key steroidogenic enzymes (StAR, P450SCC, 3βHSD, P450C17, and P450AROM) depending on the examined period of pregnancy. Resistin stimulated Akt signaling pathway activation, suggesting the involvement of this pathway in the mechanism of that adipokine action. Given the circumstances, resistin may be one of the regulators of pregnancy establishment in pigs; however, further studies are necessary to find out more details.
Data availability
All raw data were generated at the Department of Animal Anatomy and Physiology, Faculty of Biology and Biotechnology, University of Warmia and Mazury in Olsztyn, Poland. All data supporting obtained results are available from the corresponding author upon reasonable request.
Declaration of funding
This research was supported by the Polish National Science Centre (grant no. 2018/31/N/NZ9/00783).
References
Akins EL, Morrissette MC (1968) Gross ovarian changes during estrous cycle of swine. American Journal of Veterinary Research 29(10), 1953-1957.
| Google Scholar | PubMed |
Anderson LL (1978) Growth, protein content and distribution of early pig embryos. The Anatomical Record 190(1), 143-153.
| Crossref | Google Scholar | PubMed |
Banerjee RR, Lazar MA (2001) Dimerization of resistin and resistin-like molecules is determined by a single cysteine. Journal of Biological Chemistry 276(28), 25970-25973.
| Crossref | Google Scholar | PubMed |
Bischof RJ, Brandon MR, Lee C-S (1995) Cellular immune responses in the pig uterus during pregnancy. Journal of Reproductive Immunology 29(2), 161-178.
| Crossref | Google Scholar | PubMed |
Blitek A, Mendrzycka AU, Bieganska MK, Waclawik A, Ziecik AJ (2007) Effect of steroids on basal and LH-stimulated prostaglandins F(2alpha) and E(2) release and cyclooxygenase-2 expression in cultured porcine endometrial stromal cells. Reproductive Biology 7(1), 73-88.
| Google Scholar | PubMed |
Caja S, Martínez I, Abelenda M, Puerta M (2005) Resistin expression and plasma concentration peak at different times during pregnancy in rats. Journal of Endocrinology 185(3), 551-559.
| Crossref | Google Scholar | PubMed |
Chen D, Dong M, Fang Q, He J, Wang Z, Yang X (2005) Alterations of serum resistin in normal pregnancy and pre-eclampsia. Clinical Science 108(1), 81-84.
| Crossref | Google Scholar | PubMed |
Dall’Aglio C, Scocco P, Maranesi M, Petrucci L, Acuti G, De Felice E, Mercati F (2019) Immunohistochemical identification of resistin in the uterus of ewes subjected to different diets: preliminary results. European Journal of Histochemistry 63(2), 3020.
| Crossref | Google Scholar |
Daquinag AC, Zhang Y, Amaya-Manzanares F, Simmons PJ, Kolonin MG (2011) An isoform of decorin is a resistin receptor on the surface of adipose progenitor cells. Cell Stem Cell 9(1), 74-86.
| Crossref | Google Scholar | PubMed |
Dassen H, Punyadeera C, Kamps R, Delvoux B, Van Langendonckt A, Donnez J, Husen B, Thole H, Dunselman G, Groothuis P (2007) Estrogen metabolizing enzymes in endometrium and endometriosis. Human Reproduction 22(12), 3148-3158.
| Crossref | Google Scholar | PubMed |
Deaver DR, Guthrie HD (1980) Cytoplasmic estrogen receptor, estradiol and progesterone concentrations in endometrium of nonpregnant and pregnant pigs. Biology of Reproduction 23(1), 72-77.
| Crossref | Google Scholar | PubMed |
Di Simone N, Di Nicuolo F, Marzioni D, Castellucci M, Sanguinetti M, D’lppolito S, Caruso A (2009) Resistin modulates glucose uptake and glucose transporter-1 (GLUT-1) expression in trophoblast cells. Journal of Cellular and Molecular Medicine 13(2), 388-397.
| Crossref | Google Scholar | PubMed |
Dobrzyn K, Smolinska N, Szeszko K, Kiezun M, Maleszka A, Rytelewska E, Kaminski T (2017) Effect of progesterone on adiponectin system in the porcine uterus during early pregnancy. Journal of Animal Science 95(1), 338-352.
| Crossref | Google Scholar | PubMed |
Estienne A, Bongrani A, Reverchon M, Ramé C, Ducluzeau P-H, Froment P, Dupont J (2019) Involvement of novel adipokines, chemerin, visfatin, resistin and apelin in reproductive functions in normal and pathological conditions in humans and animal models. International Journal of Molecular Sciences 20(18), 4431.
| Crossref | Google Scholar |
Fanjul LF, Quintana J, González J, Santana P, Estévez F, Ruiz de Galarreta CM (1992) Testicular 3β-hydroxysteroid dehydrogenase/Δ5-4 isomerase in the hypophysectomized rat: effect of treatment with 5α-dihydrotestosterone. Journal of Endocrinology 133(2), 237-243.
| Crossref | Google Scholar | PubMed |
Fasshauer M, Blüher M (2015) Adipokines in health and disease. Trends in Pharmacological Sciences 36(7), 461-470.
| Crossref | Google Scholar | PubMed |
Franczak A (2008) Endometrial and myometrial secretion of androgens and estrone during early pregnancy and luteolysis in pigs. Reproductive Biology 8(3), 213-228.
| Crossref | Google Scholar | PubMed |
Franczak A, Kotwica G (2008) Secretion of estradiol-17β by porcine endometrium and myometrium during early pregnancy and luteolysis. Theriogenology 69(3), 283-289.
| Crossref | Google Scholar | PubMed |
Geisert RD, Brookbank JW, Michael Roberts R, Bazer FW (1982) Establishment of pregnancy in the pig: II. Cellular remodeling of the porcine blastocyst during elongation on day 12 of pregnancy. Biology of Reproduction 27(4), 941-955.
| Crossref | Google Scholar | PubMed |
Geisert RD, Zavy MT, Moffatt RJ, Blair RM, Yellin T (1990) Embryonic steroids and the establishment of pregnancy in pigs. Journal of Reproduction and Fertility. Supplement 40, 293-305.
| Google Scholar | PubMed |
Geisert RD, Pratt TN, Bazer FW, Mayes JS, Watson GH (1994) Immunocytochemical localization and changes in endometrial progestin receptor protein during the porcine oestrous cycle and early pregnancy. Reproduction, Fertility and Development 6(6), 749-760.
| Crossref | Google Scholar | PubMed |
Goff AK, Leduc S, Poitras P, Vaillancourt D (1993) Steroid synthesis by equine conceptuses between days 7 and 14 and endometrial steroid metabolism. Domestic Animal Endocrinology 10(3), 229-236.
| Crossref | Google Scholar | PubMed |
Gry M, Rimini R, Strömberg S, Asplund A, Pontén F, Uhlén M, Nilsson P (2009) Correlations between RNA and protein expression profiles in 23 human cell lines. BMC Genomics 10, 365.
| Crossref | Google Scholar |
Gudelska M, Dobrzyn K, Kiezun M, Rytelewska E, Kisielewska K, Kaminska B, Kaminski T, Smolinska N (2020) The expression of chemerin and its receptors (CMKLR1, GPR1, CCRL2) in the porcine uterus during the oestrous cycle and early pregnancy and in trophoblasts and conceptuses. Animal 14(10), 2116-2128.
| Crossref | Google Scholar | PubMed |
Gudelska M, Dobrzyn K, Kiezun M, Kisielewska K, Rytelewska E, Kaminski T, Smolinska N (2022) Chemerin affects P4 and E2 synthesis in the porcine endometrium during early pregnancy. International Journal of Molecular Sciences 23(2), 945.
| Crossref | Google Scholar |
Gygi SP, Rochon Y, Franza BR, Aebersold R (1999) Correlation between protein and mRNA abundance in yeast. Molecular and Cellular Biology 19(3), 1720-1730.
| Crossref | Google Scholar | PubMed |
Hu J, Braileanu GT, Mirando MA (2003) Effect of ovarian steroids on basal and oxytocin-induced prostaglandin F2α secretion from pig endometrial cells. Reproduction, Fertility and Development 15(4), 197-205.
| Crossref | Google Scholar | PubMed |
Hua K, Li T, He Y, Guan A, Chen L, Gao Y, Xu Q, Wang H, Luo R, Zhao L, Jin H (2023) Resistin secreted by porcine alveolar macrophages leads to endothelial cell dysfunction during Haemophilus parasuis infection. Virulence 14(1), 2171636.
| Crossref | Google Scholar |
Ka H, Seo H, Choi Y, Yoo I, Han J (2018) Endometrial response to conceptus-derived estrogen and interleukin-1β at the time of implantation in pigs. Journal of Animal Science and Biotechnology 9, 44.
| Crossref | Google Scholar |
Kaczynski P, Baryla M, Goryszewska E, Waclawik A (2021) Estradiol-17β regulates expression of luteal DNA methyltransferases and genes involved in the porcine corpus luteum function in vivo. International Journal of Molecular Sciences 22(7), 3655.
| Crossref | Google Scholar |
Kaczynski P, Goryszewska-Szczurek E, Baryla M, Waclawik A (2023) Novel insights into conceptus-maternal signaling during pregnancy establishment in pigs. Molecular Reproduction and Development 90(7), 658-672.
| Crossref | Google Scholar | PubMed |
Kaeoket K, Dalin A-M, Magnusson U, Persson E (2002) Corrigendum to “The sow endometrium at different stages of the oestrous cycle: studies on the distribution of CD2, CD4, CD8 and MHC class II expressing” cells [Anim. Reprod. Sci. 68 (2001) 99-109]. Animal Reproduction Science 73(1-2), 109-119.
| Crossref | Google Scholar | PubMed |
Kaminski T, Smolinska N, Kiezun M, Dobrzyn K, Szeszko K, Maleszka A (2018) Effect of orexin B on CYP17A1 and CYP19A3 expression and oestradiol, oestrone and testosterone secretion in the porcine uterus during early pregnancy and the oestrous cycle. Animal 12(9), 1921-1932.
| Crossref | Google Scholar | PubMed |
Kiezun M, Smolinska N, Dobrzyn K, Szeszko K, Rytelewska E, Kaminski T (2017) The effect of orexin A on CYP17A1 and CYP19A3 expression and on oestradiol, oestrone and testosterone secretion in the porcine uterus during early pregnancy and the oestrous cycle. Theriogenology 90, 129-140.
| Crossref | Google Scholar | PubMed |
Kiezun M, Dobrzyn K, Kiezun J, Kaminski T, Smolinska N (2023) Chemerin affects the expression of angiogenesis-related factors in the porcine endometrium during early pregnancy and the oestrous cycle: an in vitro study. Reproduction, Fertility and Development 35(16), 692-707.
| Crossref | Google Scholar | PubMed |
Kisielewska K, Rytelewska E, Gudelska M, Kiezun M, Dobrzyn K, Szeszko K, Bors K, Wyrebek J, Kaminski T, Smolinska N (2019) The effect of orexin B on steroidogenic acute regulatory protein, P450 side-chain cleavage enzyme, and 3β-hydroxysteroid dehydrogenase gene expression, and progesterone and androstenedione secretion by the porcine uterus during early pregnancy and the estrous cycle. Journal of Animal Science 97(2), 851-864.
| Crossref | Google Scholar | PubMed |
Koziorowski M, Kotwica G, Stefanczyk S, Krzymowski T (1984) Estradiol, progesterone and testosterone receptors for pig endometrium and myometrium at various stages of the estrous cycle. Experimental and Clinical Endocrinology & Diabetes 84(3), 285-293.
| Crossref | Google Scholar |
Kurowska P, Sroka M, Dawid M, Mlyczyńska E, Respekta N, Jurek M, Klimczyk D, Grzesiak M, Dupont J, Rak A (2021) Expression and role of resistin on steroid secretion in the porcine corpus luteum. Reproduction 162(4), 237-248.
| Crossref | Google Scholar | PubMed |
Lee S, Lee H-C, Kwon Y-W, Lee SE, Cho Y, Kim J, Lee S, Kim J-Y, Lee J, Yang H-M, Mook-Jung I, Nam K-Y, Chung J, Lazar MA, Kim H-S (2014) Adenylyl cyclase-associated protein 1 is a receptor for human resistin and mediates inflammatory actions of human monocytes. Cell Metabolism 19(3), 484-497.
| Crossref | Google Scholar | PubMed |
Li JJ, Bickel PJ, Biggin MD (2014) System wide analyses have underestimated protein abundances and the importance of transcription in mammals. PeerJ 2, e270.
| Crossref | Google Scholar |
Li Y, Yang Q, Cai D, Guo H, Fang J, Cui H, Gou L, Deng J, Wang Z, Zuo Z (2021) Resistin, a novel host defense peptide of innate immunity. Frontiers in Immunology 12, 699807.
| Crossref | Google Scholar |
Livak KJ, Schmittgen TD (2001) Analysis of relative gene expression data using real-time quantitative PCR and the 2-ΔΔCT Method. Methods 25(4), 402-408.
| Crossref | Google Scholar | PubMed |
Maillard V, Froment P, Ramé C, Uzbekova S, Elis S, Dupont J (2011) Expression and effect of resistin on bovine and rat granulosa cell steroidogenesis and proliferation. Reproduction 141(4), 467-479.
| Crossref | Google Scholar | PubMed |
Maillard V, Elis S, Desmarchais A, Hivelin C, Lardic L, Lomet D, Uzbekova S, Monget P, Dupont J (2017) Visfatin and resistin in gonadotroph cells: expression, regulation of LH secretion and signalling pathways. Reproduction, Fertility and Development 29(12), 2479-2495.
| Crossref | Google Scholar | PubMed |
Mann GE, Scholey DV, Robinson RS (2007) Identification of elevated concentrations of estradiol in bovine uterine endometrium. Domestic Animal Endocrinology 33(4), 437-441.
| Crossref | Google Scholar | PubMed |
McTernan CL, McTernan PG, Harte AL, Levick PL, Barnett AH, Kumar S (2002) Resistin, central obesity, and type 2 diabetes. The Lancet 359(9300), 46-47.
| Crossref | Google Scholar |
Meyer AE, Pfeiffer CA, Brooks KE, Spate LD, Benne JA, Cecil R, Samuel MS, Murphy CN, Behura S, McLean MK, Ciernia LA, Smith MF, Whitworth KM, Wells KD, Spencer TE, Prather RS, Geisert RD (2019) New perspective on conceptus estrogens in maternal recognition and pregnancy establishment in the pig. Biology of Reproduction 101(1), 148-161.
| Crossref | Google Scholar | PubMed |
Munir I, Yen HW, Baruth T, Tarkowski R, Azziz R, Magoffin DA, Jakimiuk AJ (2005) Resistin stimulation of 17α-hydroxylase activity in ovarian theca cells in vitro: relevance to polycystic ovary syndrome. The Journal of Clinical Endocrinology & Metabolism 90(8), 4852-4857.
| Crossref | Google Scholar | PubMed |
Nitkiewicz A, Smolinska N, Maleszka A, Kiezun M, Kaminski T (2012) Localization of orexin A and orexin B in the porcine uterus. Reproductive Biology 12(2), 135-155.
| Crossref | Google Scholar | PubMed |
Oestrup O, Hall V, Petkov SG, Wolf XA, Hyldig S, Hyttel P (2009) From zygote to implantation: morphological and molecular dynamics during embryo development in the pig. Reproduction in Domestic Animals 44(Suppl 3), 39-49.
| Crossref | Google Scholar |
Oh YK, Ha YR, Yi KW, Park HT, Shin J-H, Kim T, Hur J-Y (2017) Increased expression of resistin in ectopic endometrial tissue of women with endometriosis. American Journal of Reproductive Immunology 78(5), e12726.
| Crossref | Google Scholar |
Rak A, Drwal E, Karpeta A, Gregoraszczuk EŁ (2015a) Regulatory role of gonadotropins and local factors produced by ovarian follicles on in vitro resistin expression and action on porcine follicular steroidogenesis. Biology of Reproduction 92(6), 142.
| Crossref | Google Scholar |
Rak A, Drwal E, Wróbel A, Gregoraszczuk EŁ (2015b) Resistin is a survival factor for porcine ovarian follicular cells. Reproduction 150(4), 343-355.
| Crossref | Google Scholar | PubMed |
Rak-Mardyła A, Durak M, Łucja Gregoraszczuk E (2013) Effects of resistin on porcine ovarian follicle steroidogenesis in prepubertal animals: an in vitro study. Reproductive Biology and Endocrinology 11, 45.
| Crossref | Google Scholar |
Rak-Mardyła A, Duda M, Gregoraszczuk EŁ (2014) A role for resistin in the ovary during the estrous cycle. Hormone and Metabolic Research 46(7), 493-498.
| Crossref | Google Scholar | PubMed |
Reverchon M, Cornuau M, Ramé C, Guerif F, Royère D, Dupont J (2013) Resistin decreases insulin-like growth factor I-induced steroid production and insulin-like growth factor I receptor signaling in human granulosa cells. Fertility and Sterility 100(1), 247-255.E3.
| Crossref | Google Scholar | PubMed |
Reverchon M, Ramé C, Bertoldo M, Dupont J (2014) Adipokines and the female reproductive tract. International Journal of Endocrinology 2014, 232454.
| Crossref | Google Scholar |
Rytelewska E, Kisielewska K, Gudelska M, Kiezun M, Dobrzyn K, Bors K, Wyrebek J, Kaminska B, Kaminski T, Smolinska N (2020) The effect of orexin a on the StAR, CYP11A1 and HSD3B1 gene expression, as well as progesterone and androstenedione secretion in the porcine uterus during early pregnancy and the oestrous cycle. Theriogenology 143, 179-190.
| Crossref | Google Scholar | PubMed |
Rytelewska E, Kiezun M, Kisielewska K, Gudelska M, Dobrzyn K, Kaminska B, Kaminski T, Smolinska N (2021) Chemerin as a modulator of ovarian steroidogenesis in pigs: an in vitro study. Theriogenology 160, 95-101.
| Crossref | Google Scholar | PubMed |
Sánchez-Solana B, Laborda J, Baladrón V (2012) Mouse resistin modulates adipogenesis and glucose uptake in 3T3-L1 preadipocytes through the ROR1 receptor. Molecular Endocrinology 26(1), 110-127.
| Crossref | Google Scholar | PubMed |
Schiff R, Arensburg J, Itin A, Keshet E, Orly J (1993) Expression and cellular localization of uterine side-chain cleavage cytochrome P450 messenger ribonucleic acid during early pregnancy in mice. Endocrinology 133(2), 529-537.
| Crossref | Google Scholar | PubMed |
Smolinska N, Dobrzyn K, Kiezun M, Szeszko K, Maleszka A, Kaminski T (2016) Effect of adiponectin on the steroidogenic acute regulatory protein, P450 side chain cleavage enzyme and 3β-hydroxysteroid dehydrogenase gene expression, progesterone and androstenedione production by the porcine uterus during early pregnancy. Journal of Physiology and Pharmacology 67(3), 443-456.
| Google Scholar | PubMed |
Soede NM, Langendijk P, Kemp B (2011) Reproductive cycles in pigs. Animal Reproduction Science 124(3-4), 251-258.
| Crossref | Google Scholar | PubMed |
Spicer LJ, Schreiber NB, Lagaly DV, Aad PY, Douthit LB, Grado-Ahuir JA (2011) Effect of resistin on granulosa and theca cell function in cattle. Animal Reproduction Science 124(1-2), 19-27.
| Crossref | Google Scholar | PubMed |
Steppan CM, Bailey ST, Bhat S, Brown EJ, Banerjee RR, Wright CM, Patel HR, Ahima RS, Lazar MA (2001) The hormone resistin links obesity to diabetes. Nature 409(6818), 307-312.
| Crossref | Google Scholar | PubMed |
Tanaka N, Iwamasa J, Matsuura K, Okamura H (1993) Effects of progesterone and anti-progesterone RU486 on ovarian 3β-hydroxysteroid dehydrogenase activity during ovulation in the gonadotrophin-primed immature rat. Journal of Reproduction and Fertility 97(1), 167-172.
| Crossref | Google Scholar | PubMed |
Tarkowski A, Bjersing J, Shestakov A, Bokarewa MI (2010) Resistin competes with lipopolysaccharide for binding to toll-like receptor 4. Journal of Cellular and Molecular Medicine 14(6b), 1419-1431.
| Crossref | Google Scholar | PubMed |
Tarleton BJ, Wiley AA, Bartol FF (1999) Endometrial development and adenogenesis in the neonatal pig: effects of estradiol valerate and the antiestrogen ICI 182,780. Biology of Reproduction 61(1), 253-263.
| Crossref | Google Scholar | PubMed |
Wilkinson M, Wilkinson D, Wiesner G, Morash B, Ur E (2005) Hypothalamic resistin immunoreactivity is reduced by obesity in the mouse: co-localization with α-melanostimulating hormone. Neuroendocrinology 81(1), 19-30.
| Crossref | Google Scholar | PubMed |
Yura S, Sagawa N, Itoh H, Kakui K, Nuamah MA, Korita D, Takemura M, Fujii S (2003) Resistin is expressed in the human placenta. The Journal of Clinical Endocrinology & Metabolism 88(3), 1394-1397.
| Crossref | Google Scholar | PubMed |