Endocrine changes induced by GnRH immunisation and subsequent early re-stimulation of testicular function with a GnRH agonist in stallions
Camille Gautier


A
B
C
D
Abstract
Resumption of testicular function after gonadotrophin-releasing hormone (GnRH) immunisation varies among individual animals and some stallions regain fertility only after a prolonged time.
This study evaluated endocrine effects of GnRH immunisation and early subsequent re-stimulation with a GnRH agonist. We hypothesised that GnRH agonist treatment advances resumption of normal endocrine function in GnRH-vaccinated stallions.
Shetland stallions were assigned to an experimental and a control group (n = 6 each). Experimental stallions were GnRH-immunised twice, 4 weeks apart. Each experimental stallion was hemicastrated together with an age-matched control animal when testosterone concentration decreased below 0.3 ng/mL. Three weeks later, daily treatment with the GnRH agonist buserelin was initiated (4 μg/day for 4 weeks followed by 8 μg/day). The remaining testicle was removed when testosterone concentration exceeded 0.5 ng/mL in vaccinated stallions. Blood was collected for LH, FSH, oestradiol and anti-müllerian hormone (AMH) analyses, and testicular and epididymal tissue were conserved for real-time qPCR and histology.
GnRH vaccination reduced blood concentrations of LH and FSH, with a structural deterioration of testicular tissue and disruption of spermatogenesis. Daily buserelin treatment for approximately 60 days partially restored gonadotropin secretion and induced a recovery of the functional organisation of the testicular tissue with effective spermatogenesis.
Endocrine testicular function can be restored in GnRH-vaccinated stallions by daily low-dose buserelin treatment. The buserelin treatment protocol may potentially be improved regarding the dose, interval and duration.
Daily buserelin treatment can be recommended for treatment of GnRH-vaccinated stallions with prolonged inhibition of testicular function.
Keywords: buserelin, CYP19A1, FSH, GnRH vaccination, horse, LH, reproduction, testis.
Introduction
Vaccination against gonadotrophin-releasing hormone (GnRH) temporarily suppresses testicular function in male animals. Besides the wide-spread use in male pigs raised for pork production in some countries (Einarsson et al. 2011), GnRH vaccine is also used in male horses with the aim to reduce androgen-related behaviours. The GnRH vaccination suppresses the stimulus for LH and FSH secretion, which decreases steroidogenesis, spermatogenesis and sexual behaviour (Thompson 2000; Malmgren et al. 2001; Clement et al. 2005; Turkstra et al. 2005; Janett et al. 2009). Because it reduces testosterone synthesis, vaccination against GnRH has also been suggested as a treatment in stallions persistently infected with equine arteritis virus (Vidament et al. 2010). Testicular function is reduced transiently by GnRH immunisation but the time until complete resumption varies considerably among individual stallions. Occasionally, endocrine characteristics in stallions have not returned to the pre-vaccination state within 10–12 months (Burger et al. 2006; Janett et al. 2009; Miszczak et al. 2020). The reasons for such individual variations so far are poorly understood. Although we have recently shown that a daily, low-dose GnRH agonist treatment after GnRH immunisation can enhance the restoration of spermatogenesis (Gautier et al. 2022), there is no accepted treatment for accelerating resumption of testicular function in GnRH-vaccinated stallions. Furthermore, the GnRH-vaccinated, downregulated stallion may provide a model for endocrine disruption of spermatogenesis and potential treatments for reversal in other species.
Testicular function in stallions is regulated by positive and negative feedback mechanisms that involve the hypothalamus, pituitary and testes (reviewed by Roser 2008 and Aurich 2018). The pituitary function is reflected by LH and FSH secretion, while the peripheral concentrations of testicular steroid hormones are indicators of testicular endocrine function. The inactivation of GnRH by antibody binding reduces the production and release of LH and FSH. This will result in a decreased stimulation of steroidogenesis by Leydig cells (Rabb et al. 1990; Amann 1993), impaired Sertoli cell function and reduced spermatogenesis (Saez et al. 1989).
The objective of this study was to evaluate acute endocrine effects and histological changes in testicular parenchyma induced by GnRH immunisation and re-stimulation of testicular function with a GnRH agonist. We hypothesised that daily low-dose GnRH agonist treatment will normalise endocrine homeostasis, and thus enhance testicular function. Concentrations of LH, FSH and AMH plasma concentrations were evaluated. Protein expression and mRNA abundance for the LH receptor (LH-R), FSH receptor (FSH-R) and CYP19A1, and histomorphology were analysed in testicular and epididymal tissue of GnRH-vaccinated and control stallions.
Materials and methods
Animals
The study was approved by the Austrian Federal Ministry for Education, Science and Research (license number 2020-0.328.742) and was conducted in Vienna, Austria, continuing recent work on the effects of the anti-GnRH vaccine Improvac and subsequent GnRH-agonist treatment on semen characteristics (Gautier et al. 2022). Twelve fertile Shetland stallions aged between 3 and 14 years (8.8 ± 1.2 years, mean ± s.e.m.) with body weights between 99 and 207 kg (144 ± 8 kg) were included in the study as described previously (Gautier et al. 2022).
Experimental design
The experiment consisted of a 4-week pre-vaccination phase to determine basal endocrine and testicular parameters. This was followed by an experimental phase of 25 weeks. Stallions were ranked by age and assigned in alternate order to an experimental and a control group, thus creating pairs of experimental and control stallions of similar ages. Animals of the experimental group (n = 6) were immunised against GnRH with the Improvac vaccine (Zoetis, Vienna, Austria; 400 μg per animal injected into the pectoral musculature in weeks 0 and 4) whereas control stallions (n = 6) received 1 mL of saline as described previously (Gautier et al. 2022). Blood was collected once a week throughout the study for determination of plasma testosterone, oestradiol and AMH, and serum LH and FSH concentration.
After testosterone concentrations had decreased to <0.3 ng/mL for two consecutive weeks, each stallion in the experimental group and age-matched control stallion was hemicastrated. The first hemicastration was performed in weeks 9 (n = 1), 10 (n = 2), 11 (n = 2) and 12 (n = 1) after the first GnRH vaccination (numbers in brackets refer to the treatment group, the same number of control stallions was castrated simultaneously).
The different parts of the epididymal and testicular parenchyma were immediately dissected and fixed in 4% (v/v) buffered formaldehyde for histology and immunohistochemistry or placed in RNAlater (Qiagen, Vienna, Austria) and stored at −80°C for real-time quantitative polymerase chain reaction (qPCR). Three weeks after hemicastration, treatment with the GnRH agonist buserelin (Veterelin, Laboratorios Callier, Barcelona, Spain) was initiated (4 μg/day for 4 weeks followed by 8 μg/day intramuscularly until castration) to stimulate testicular function in vaccinated stallions, while control stallions received saline. The remaining testicles were removed from the matched control and experimental stallions when testosterone concentrations in the GnRH-vaccinated stallions exceeded 0.5 ng/mL for two consecutive weeks. The second hemicastration was performed in weeks 9 (n = 1) and 10 (n = 5) after initiation of buserelin treatment (numbers in brackets refer to the treatment group, the same number of control stallions was castrated simultaneously). Testicular and epididymal samples were processed as described above for analyses.
Endocrine analyses
Blood samples were collected from the jugular vein, alternating sides, in heparinised and clot activator tubes (Vacuette, Greiner, Kremsmünster, Austria). The tubes were centrifuged within 30 min after collection at 1200g and 5°C for 10 min. Plasma and serum samples were immediately frozen and stored at −20°C until analysis was carried out. Plasma testosterone concentrations were analysed as described and data have been published previously (Gautier et al. 2022). Analysis of plasma oestradiol concentration was performed by direct enzyme immunoassay (Estradiol ELISA DE2693; Demeditec Diagnostics, Kiel, Germany) validated in our laboratory. Recovery of oestradiol standard added to plasma was close to 100% and serial dilution of plasma resulted in concentrations decreasing in parallel to the standard curve. The sensitivity of the assay was 1.6 pg/mL. The intra-assay and inter-assay coefficients of variation were 7.2% and 4.2%, respectively. Serum FSH and LH concentrations were analysed by radioimmunoassay at Endolytics (Fort Collins, CO, USA), previously validated for use in the horse (Nett et al. 1975, 1979). The intra-assay coefficients of variation were 4.9% and 7.9%, and the inter-assay coefficients of variation were 11.0% and 18.7% for LH and FSH, respectively. The minimal detectable concentration was 1.3 ng/mL for LH and 3.1 ng/mL for FSH. Plasma AMH concentrations were determined with an enzyme-linked immunosorbent assay (AL-115, Ansh Labs, Webster, TX, USA) as described previously and validated for equine plasma in our laboratory (Scarlet et al. 2018). The intra- and inter-assay coefficients of variation were 3.2% and 2.1%, respectively, and the minimal detectable concentration was 0.03 ng/mL.
Real-time qPCR
Testicular and epididymal tissue from both hemicastrations was analysed. For RNA extraction, samples were mechanically homogenised on a MagNA Lyser instrument (Roche, Rotkreuz, Switzerland) using 1.4-mm ceramic beads (VWR, Vienna, Austria) at 6500g for 30 s. The RNA was extracted with the RNeasy Mini Kit and the RNase-Free DNase Set (Qiagen) in accordance with the manufacturer’s protocol. The RNA integrity values were assessed on the 2100 Bioanalyser using the RNA Nano 6000 Kit (Agilent, Santa Clara, CA, USA). Only samples with an RNA integrity number (RIN) ≥ 5 were used for subsequent real-time qPCR. Reverse transcription was performed with the high-capacity cDNA Reverse Transcription Kit (Thermo Fisher, Waltham, MA, USA). No reverse transcription (RT) controls (without RT enzyme) were included to monitor for contaminating DNA. Assay details are listed in Table 1 (Scarlet et al. 2015; Herrera-Luna et al. 2016). The assays were validated by the generation of standard curves to calculate the PCR amplification efficiencies (Kenkel et al. 2011). The real-time qPCR was performed in 20-μL reaction volumes including 1× HOT FIREPol EvaGreen qPCR Mix Plus ROX (Solis BioDyne, Tartu, Estonia), 200 nM of each primer and 20 ng cDNA. All samples were analysed in triplicate on the qTOWER3 84 Real-time PCR cycler (Analytik Jena, Jena, Germany) using the following temperature profile: initial denaturation at 95°C for 12 min, 40 cycles of 95°C for 15 s, and 60°C for 1 min, followed by a melting curve analysis over a temperature range of 60–95°C. Four candidate reference genes (RGs): GAPDH, PSMB4, SNRPD3 and VCP, were included as described (Scarlet et al. 2015). The RG stability was assessed with the BestKeeper tool and GAPDH as the most stably expressed gene was selected for normalisation (Pfaffl et al. 2004). Mean Cq values were corrected for PCR reaction efficiencies (E), normalised to the RG, and relative expression changes were calculated using the comparative 2−ΔΔCT method (Livak and Schmittgen 2001).
Accession number A | Gene symbol | Gene name | Oligo | Sequence (5′−3′) | Amplicon size (bp) | E | Reference | |
---|---|---|---|---|---|---|---|---|
XM_005602588.3, XM_005602587.3, XM_023620997.1, XM_023640810.1, XM_023650771.1 | CYP19A1 | Equus caballus cytochrome P450 family 19 subfamily A member 1 | Forward Reverse | GAGAAACGTGGTGAGCTGAC CGCAATGAGACATAGCATGAAGA | 114 | 1.04 | Herrera-Luna et al. (2016) | |
XM_005599992.3, XM_023619110.1, XM_023619111.1 | LHCGR | Equus caballus luteinising hormone/choriogonadotropin receptor | Forward Reverse | CCACAACTGATAGCTACCAACAAAG TGCAGGTGAAATCGGTGAAG | 79 | 1 | Herrera-Luna et al. (2016) | |
XM_023618416.1, XM_023618413.1, XM_023618414.1, XM_005599769.3 | FSHR | Equus caballus follicle stimulating hormone receptor | Forward Reverse | CCACATCTCCCATCCAAGGA TGTGCAATTGGCACCATTG | 70 | 1.04 | Herrera-Luna et al. (2016) | |
NM_001163856.1 XM_008513936.1 | GAPDH | Equus caballus glyceraldehyde-3-phosphate dehydrogenase | Forward Reverse | GGCAAGTTCCATGGCACAGT GGCAAGTTCCATGGCACAGT | 129 | 0.9 | Scarlet et al. (2015) |
E, amplification efficiency.
Histomorphology
Testicular and epididymal tissue were embedded in paraffin wax. The 3-μm sections were mounted on 3-aminopropyltriethoxysilane glutaraldehyde-coated slides, dried overnight at 37°C and then stained with hematoxylin–eosin. Measurements were always performed by the same evaluator who was blinded regarding treatments. Testis slides were evaluated under an Axio Imager Z2 light microscope (Carl Zeiss, Jena, Germany) at 100×, 200× and 400× magnification using software Zeiss Zen blue edition (Carl Zeiss, Munich, Germany), and the images recorded in tiff format. Ten fields of 100× magnification per animal were analysed with the Fiji script software (Schindelin et al. 2012). The seminiferous epithelial area and mean feret diameter (MFD = mean (max feret + min feret)) of seminiferous tubules were measured. After assessment of 10 random fields, a mean value of both parameters was calculated. The seminiferous tubule epithelium area was calculated as the percentage of the area of seminiferous tubule epithelium present in the 10 fields divided by the total area evaluated using 100× magnification.
Epididymal sections were digitalised with the Fritz Microscopy Slide Scanner (PreciPoint, Freising, Germany) at 40× magnification and were subsequently analysed with the ViewPoint software (PreciPoint). Per animal, 10 random circular epididymal sections in the head and the body of the epididymis were analysed for tubule perimeter, lumen diameter, tubule diameter, epithelial height and muscle wall thickness. The mean of each parameter was calculated from the 10 tubules analysed.
Immunohistochemistry
Formaldehyde-fixed testis samples were embedded in paraffin wax and 3-μm sections were cut. Endogenous peroxidase activity was blocked by incubation of sections in 0.6% H2O2 in methanol for 15 min at room temperature. Antigen retrieval was performed by heating tissue sections in citrate buffer (10 mM) at pH 6 for 30 min. Incubation in 1.5% normal goat serum diluted in phosphate-buffered saline (PBS) was used to minimise non-specific antibody binding. Subsequently, slides were incubated overnight with primary rabbit anti-FSH-R (LS-A4004; LSBio, Shirley, MA, USA; dilution 1:800), anti-CYP19A1 (3599; Biovision, Waltham, MA, USA; dilution 1:1000) or anti-LH-R (LS C312710; LSBio; dilution 1:100; Herrera-Luna et al. 2016) antibodies. The sections were rinsed with PBS and incubated with biotin-free anti-rabbit secondary antibody (BrightVison Poly-HRP; ImmunoLogic Technologies, Duiven, Netherlands) for 30 min at room temperature. Immunostaining was visualised using diaminobenzidine chromogen (DAB Quanto, Richard Allan Scientific, Kalamazoo, MI, USA; TA-125-QHDX) over 5 min at room temperature, followed by washing in distilled water and counterstaining with Mayer’s hemalaun for 3 min at room temperature. Finally, sections were washed with tap water for 10 min, dehydrated in an increasing series of alcohol and mounted with dibutyl phthalate in xylene (DPX; Fluka, Buchs, Switzerland). As a negative control, the primary antibodies were substituted with PBS. Horse tissue samples were immunostained as positive controls (CYP19A1 and FSH-R: horse testis; LH-R: horse pituitary).
The expression of LH-R, FSH-R and CYP19A1 were evaluated under a light microscope Axio Imager Z2 at 200× magnification and images were recorded in tiff format. The LH-R, FSH-R and CYP19A1 staining in testis samples were measured with the Fiji software applying colour deconvolution. The 3,3′-diaminobenzidine (DAB) channel was separated as described previously (Ruifrok and Johnston 2001) using a Fiji plugin (Landini et al. 2021). Particles were defined as positive if they showed intensity values within a previously determined threshold range (80–100). Positive regions were allocated to the total tissue area. The same person, blinded for animal groups, analysed all slides.
Statistical analysis
The mRNA abundance of all target genes was normalised against the geometric mean of mRNA abundance of GAPDH. ‘Fold changes’ refer to the sample with the lowest mRNA abundance (set to 1) of the respective gene. Statistical analysis was performed with the IBM SPSS statistics software (ver. 27; IBM, Armonk, NY, USA). Data were tested for normal distribution by Shapiro–Wilk test. Data for LH and FSH were not normally distributed and therefore were log-transformed for further analysis. Homogeneity of variances was asserted by Levene’s test. For all parameters determined except mRNA abundance, statistical comparisons were made by repeated measures ANOVA with group as between subject factor and time as within subject factor. Data for mRNA abundance were compared by non-parametric tests (Wilcoxon signed-rank test for comparison between times in the same stallions and Mann–Whitney U-test for comparison between GnRH vaccinated and control stallions). For all statistical analyses a P-value of <0.05 was considered significant.
Results
Gonadotropins, AMH and steroid hormones
The experimental procedures were based on changes in plasma testosterone concentrations which have been published previously (Gautier et al. 2022). Testosterone concentration decreased after GnRH vaccination and the first hemicastration was performed when testosterone concentration decreased below 0.3 ng/mL for two consecutive weeks in vaccinated stallions. During buserelin treatment, testosterone concentration increased again in GnRH-vaccinated stallions (Fig. 1). The second hemicastration was performed when testosterone concentration exceeded 0.5 ng/mL for two consecutive weeks in vaccinated stallions. In GnRH-vaccinated stallions, plasma LH concentration decreased and was undetectable after the second vaccination, slightly increased in response to buserelin treatment but remained lower than observed in control stallions (time P < 0.001; time P < 0.05, time × group P < 0.001, group P < 0.05; Fig. 2a). The concentration of FSH decreased after GnRH vaccination and increased to pre-vaccination baseline concentrations with buserelin treatment after the first hemicastration. In control stallions, FSH concentration increased stepwise after both hemicastrations and was higher than in GnRH-vaccinated stallions (time P < 0.001, time × group P < 0.001, group P < 0.05; Fig. 2b).
Plasma testosterone concentration in GnRH-vaccinated (V) and control (C) stallions. The left segment of the x-axis is aligned to the first GnRH vaccination (time 0) and to the 2 weeks before the first hemicastration (separated by the interrupted vertical line); the right segment of the x-axis is aligned to the start of buserelin treatment and to the 2 weeks before the second hemicastration (separated by interrupted vertical line). V1 and V2 indicate the first and second GnRH vaccination in weeks 0 and 4, respectively, and C1 and C2 the time of the first and second hemicastration. The time of buserelin treatment is indicated by the interrupted line parallel to the x-axis. Data represents mean ± s.e.m. *, significant differences between groups at individual time points (P < 0.05, Mann–Whitney U-test), from Gautier et al. (2022).
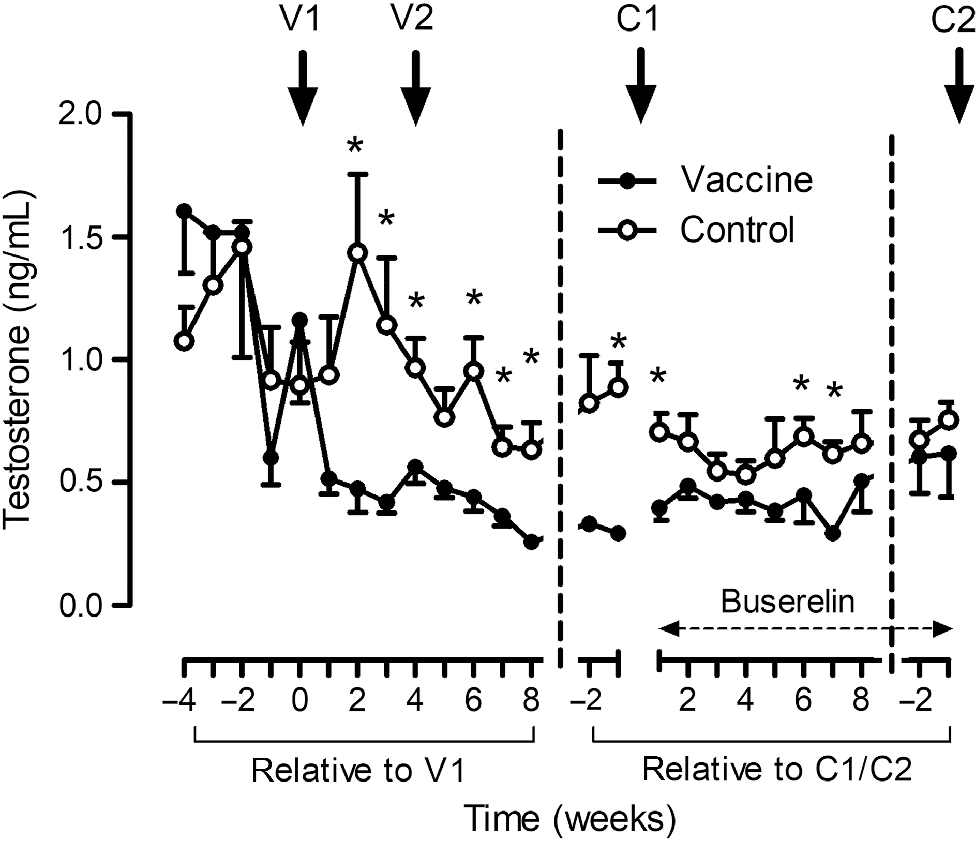
Concentration of (a) LH and (b) FSH in serum of GnRH-vaccinated () and control (
) stallions (n = 6 per group). The left segment of the x-axis is aligned to the first GnRH vaccination (time 0) and to the 2 weeks before the first hemicastration (separated by interrupted vertical line); the right segment of the x-axis is aligned to the start of buserelin treatment and to the 2 weeks before the second hemicastration (separated by interrupted vertical line). V1 and V2 indicate the first and second GnRH vaccination in weeks 0 and 4, respectively, and C1 and C2 the time of the first and second hemicastration. The period of buserelin treatment is indicated by the interrupted line parallel to the x-axis. Data represents mean ± s.e.m.
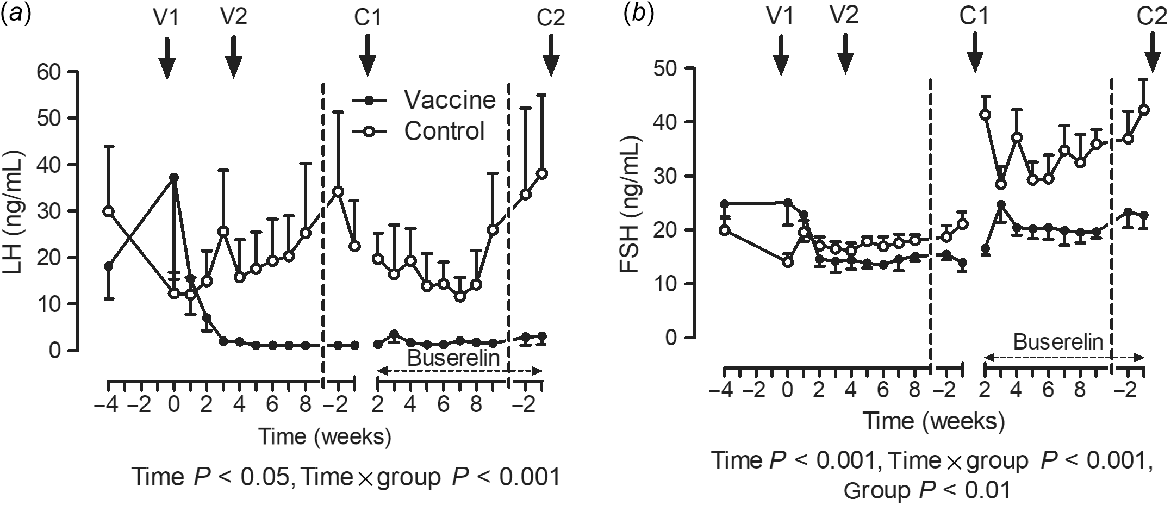
Plasma oestradiol concentration decreased after GnRH vaccination and increased progressively during buserelin treatment to the concentration of control stallions after the first hemicastration. In control stallions, plasma oestradiol concentration decreased after the first hemicastration (time P < 0.001, time × group P < 0.01, group P < 0.05; Fig. 3).
Concentration of oestradiol in plasma of GnRH-vaccinated () and control (
) stallions (n = 6 per group). The left segment of the x-axis is aligned to the first GnRH vaccination (time 0) and to the 2 weeks before the first hemicastration (separated by interrupted vertical line); the right segment of the x-axis is aligned to the start of buserelin treatment and to the 2 weeks before the second hemicastration (separated by interrupted vertical line). V1 and V2 indicate the first and second GnRH vaccination in weeks 0 and 4, respectively, and C1 and C2 the time of the first and second hemicastration. The period of buserelin treatment is indicated by the interrupted line parallel to the x-axis. Data represents mean ± s.e.m.
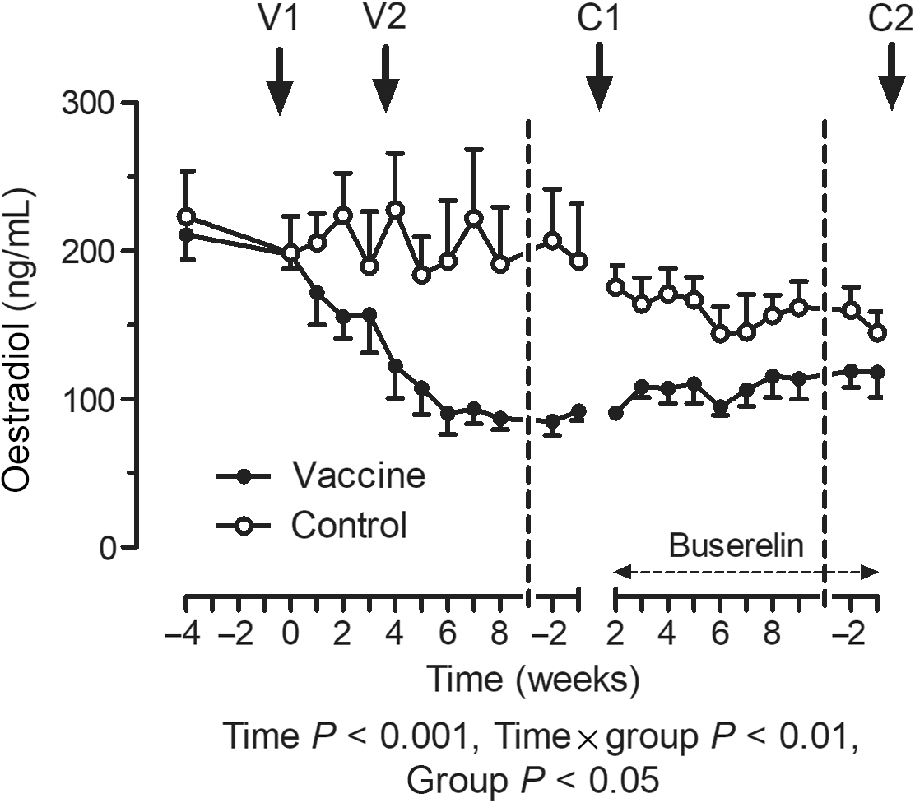
Plasma AMH concentration was not affected by GnRH immunisation but decreased after the first hemicastration in both groups, remained constant until the second hemicastration, and decreased further thereafter (P < 0.001; Fig. 4).
Concentration of AMH in plasma of GnRH-vaccinated () and control (
) stallions (n = 6 per group). The left segment of the x-axis is aligned to the first GnRH vaccination (time 0) and to the 2 weeks before the first hemicastration (separated by interrupted vertical line); the right segment of the x-axis is aligned to the start of buserelin treatment and to the 2 weeks before the second hemicastration (separated by interrupted vertical line). V1 and V2 indicate the first and second GnRH vaccination in weeks 0 and 4, respectively, and C1 and C2 the time of the first and second hemicastration. The period of buserelin treatment is indicated by the interrupted line parallel to the x-axis. Data represents mean ± s.e.m.
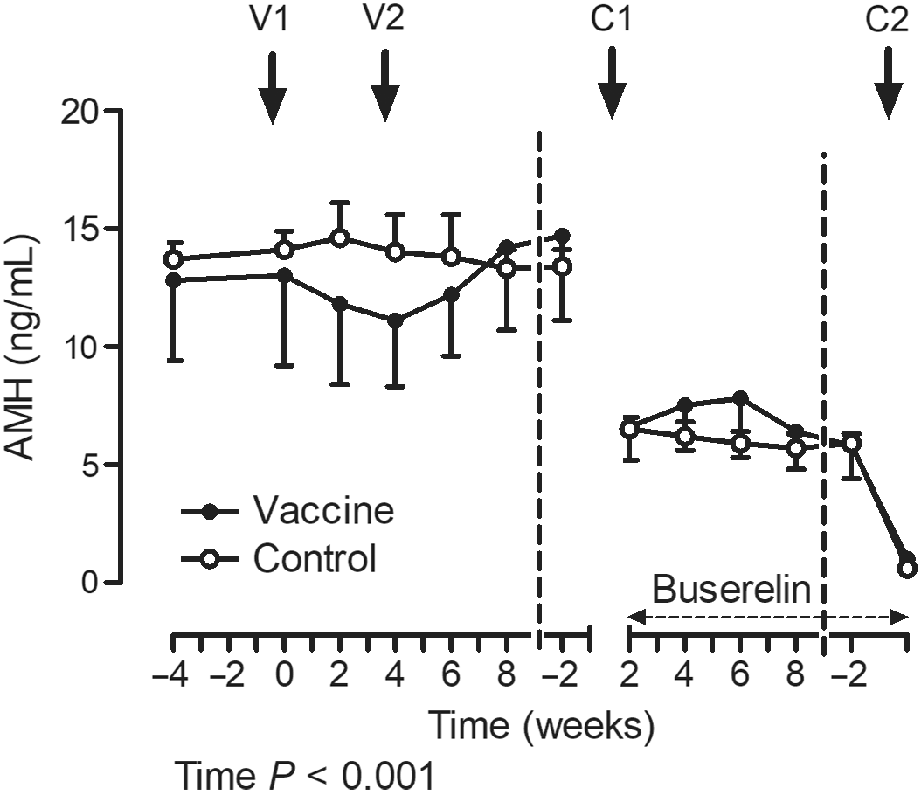
Histomorphology
The GnRH-vaccination induced a disorganisation of the seminiferous tubule structure; in testes collected after the first hemicastration, elongated spermatids were entirely missing or only present in small numbers in the tubular lumen. After buserelin treatment of GnRH-vaccinated stallions, nearly all seminiferous tubules presented a well-organised germinal epithelium with the presence of elongated spermatids in the lumen (Fig. 5).
Morphometry of seminiferous tubule sections stained with hematoxylin–eosin after GnRH vaccination (hemicastration 1) and after buserelin treatment (hemicastration 2) for all vaccinated stallions (#1 to #6). Scale bars = 50 μm.
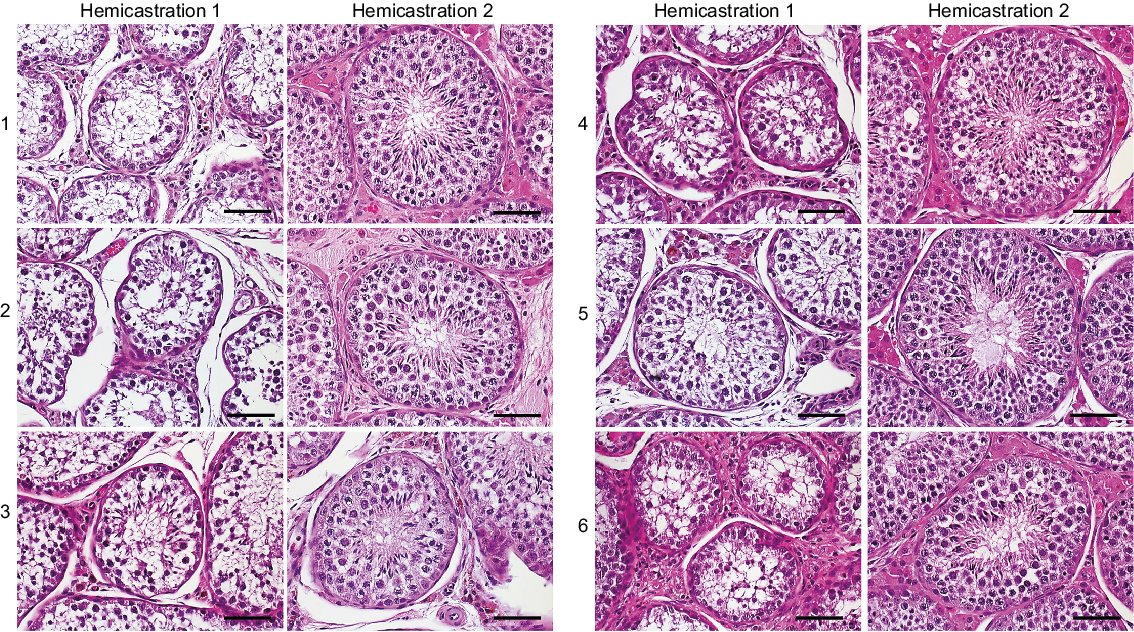
Relative area of the seminiferous tubules and average tubule diameter were smaller in GnRH-vaccinated stallions compared to control stallions at the first hemicastration and both increased again in response to buserelin treatment, reaching similar values to the control stallions after the second hemicastration (for relative tubule area time P < 0.001, time × group P < 0.01, group P < 0.05; for tubule diameter time P < 0.001, time × group P < 0.05, group P < 0.01; Fig. 6a, b).
(a) Mean percentage (+ s.e.m.) of seminiferous tubule area and (b) mean diameter (+ s.e.m.) of seminiferous tubules in GnRH-vaccinated and control stallions (n = 6 per group) at the first and second hemicastration.
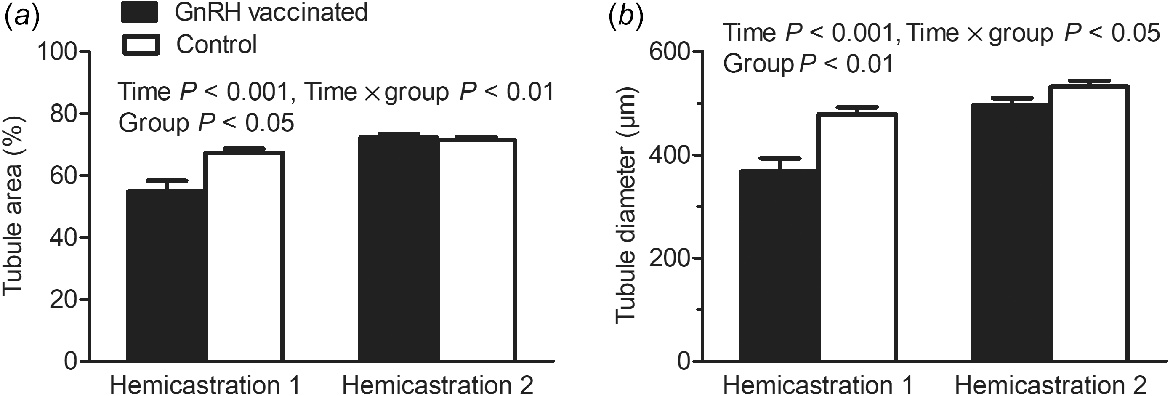
In the epididymis, both in the corpus and the caput, the luminal tubular diameter was reduced by GnRH vaccination but did not increase following buserelin treatment (P < 0.001 between groups; Fig. 7a, c). The same trend was observed for height of the tubular epithelium (caput P < 0.01 and corpus P < 0.05 between groups; Fig. 7b, d). At the first hemicastration, no or very few spermatozoa were observed along the epididymal duct in all GnRH-vaccinated stallions. At the second hemicastration, spermatozoa were present in the epididymal lumen of all six GnRH vaccinated stallions (Fig. 8).
(a) Mean diameter (+ s.e.m.) of the epididymal tubule lumen and (b) mean height (+ s.e.m.) of the tubular epithelium in the head (caput) of the epididymis and (c) diameter of the epididymal tubule lumen and (d) height of the tubular epithelium in the body (corpus) of the epididymis (n = 6 tissue samples per group).
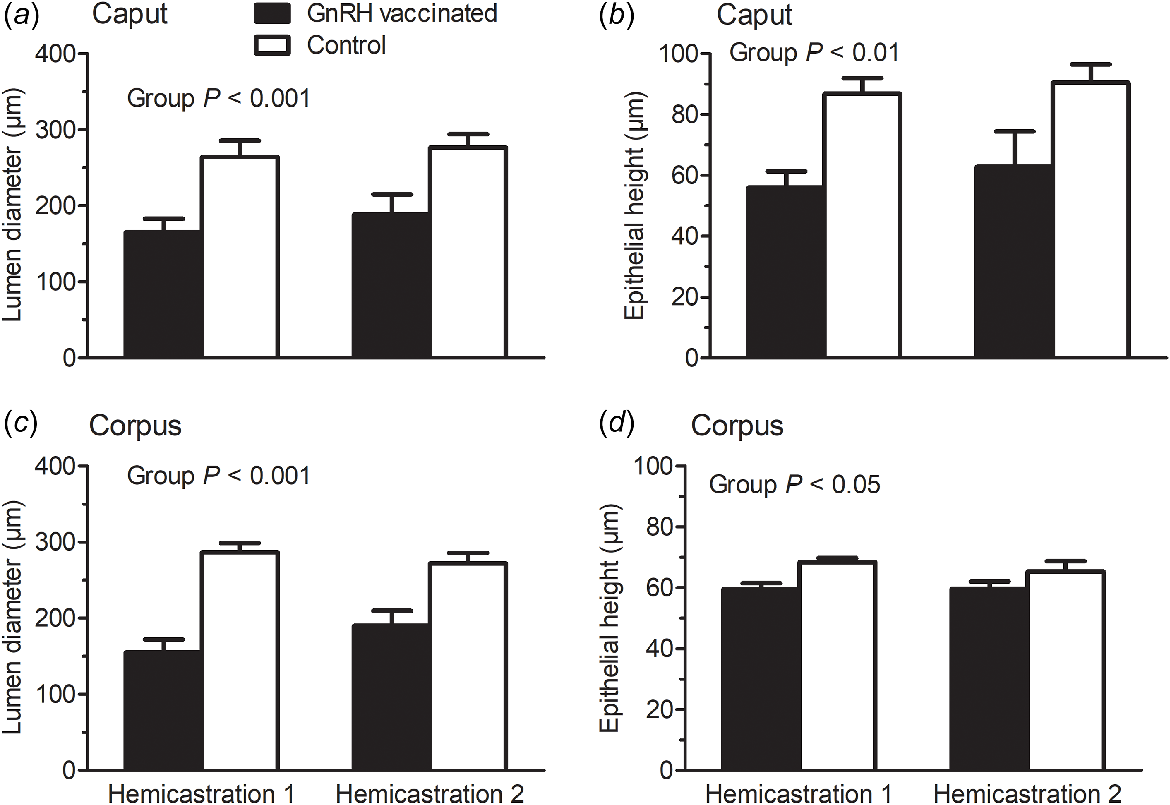
Hematoxylin staining of the epididymal corpus from (a, b) a 4-year-old GnRH-vaccinated stallion and (c, d) his age-matched control stallion at (a, c) the first and (b, d) second hemicastration; and from (e, f) a 12-year-old vaccinated stallion with (g, h) his age-matched control stallion at (e, g) the first and (f, h) second hemicastration. Scale bars = 500 μm.
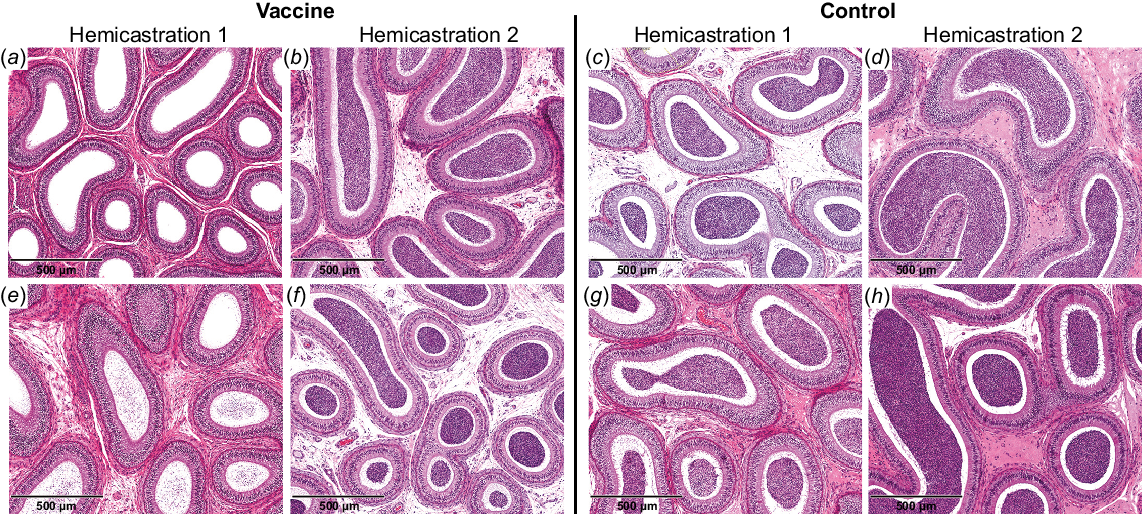
Abundance of mRNA and protein expression
Relative mRNA abundance of LHR and CYP19A1 were lower in testicular tissue of GnRH-vaccinated than control stallions (P < 0.001). After buserelin treatment of GnRH-vaccinated stallions, LHR mRNA abundance no longer differed between groups, whereas CYP19A1 mRNA remained lower in vaccinated than in control stallions (P < 0.001). Relative FSHR mRNA abundance was higher in GnRH-vaccinated than in control stallions at the first hemicastration (P < 0.001) and similar in both groups at the second hemicastration (Fig. 9a–c).
Relative mRNA abundance of (a) LH receptor (LHR), (b) FSH receptor (FSHR) and (c) CYP19A1 in testicular tissue of GnRH-vaccinated () and control (
) stallions (n = 6 per group) at the first and second hemicastration. Symbols show data for individual stallions, with the horizontal line indicating the mean and the whiskers showing the standard error (± s.e.m.). Asterisks above bars indicate statistically significant differences (*P < 0.05, **P < 0.01).
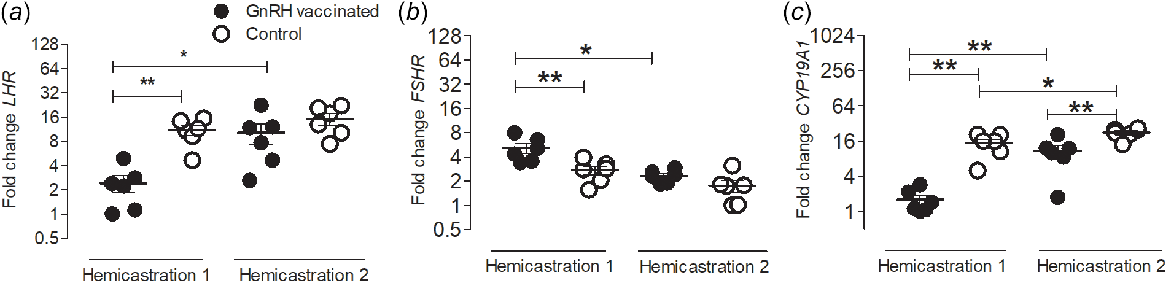
Expression of LHR protein was detected in the Leydig cells of GnRH-vaccinated and control stallions (Fig. 10). The quantification of LHR protein positive areas was lower in testes of GnRH-vaccinated compared to control stallions at the first hemicastration and was not affected by buserelin treatment (group P < 0.01; Fig. 11a). The FSH-R protein was expressed in the spermatocytes and Sertoli cells in both stallion groups (Fig. 10) and quantitative expression neither differed between groups nor changed significantly from the first to the second hemicastration (Fig. 11b). Immunostaining for CYP19A1 protein was detected in the cytoplasm of Leydig cells (Fig. 10) with a reduced positively stained area in GnRH-vaccinated stallions at the first hemicastration and an increase following the buserelin treatment (time P < 0.05, time × group P < 0.05, group P < 0.001; Fig. 11c).
Immunohistochemical staining for LH receptor (LH-R), FSH receptor (FSH-R) and CYP19A1 in the testis from GnRH-vaccinated and control stallions at the first and second hemicastration. Insets in 4, 8, and 12 represent negative controls. Scale bars = 50 μm. L, Leydig cell; Spc, spermatocyte; S, Sertoli cell.
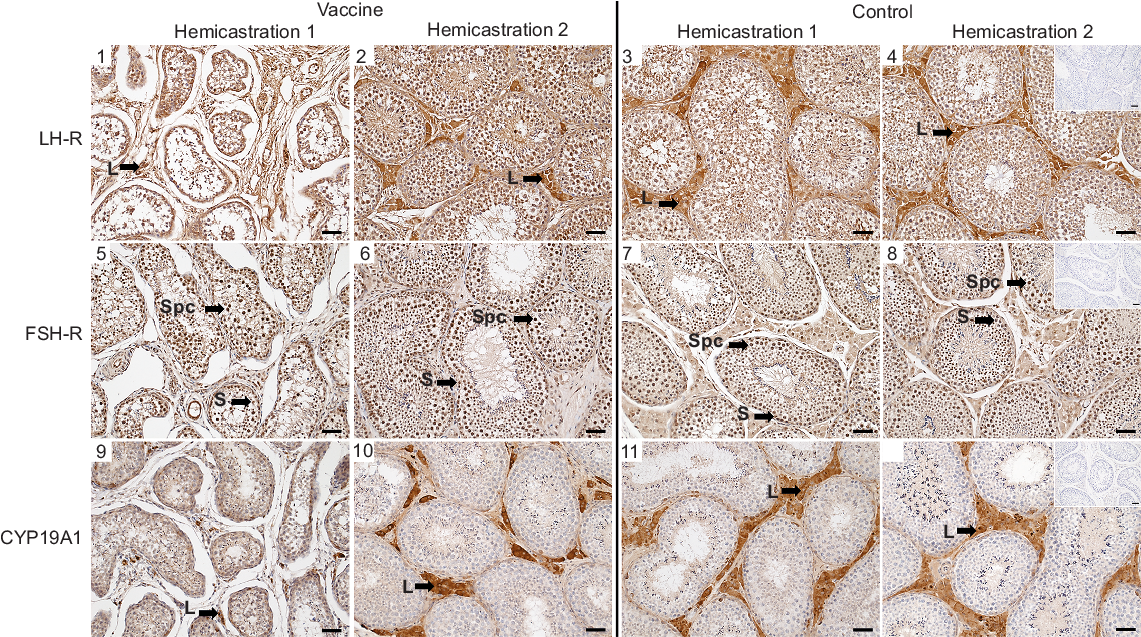
Discussion
Results of this study improve our knowledge of GnRH vaccine-induced testicular downregulation in stallions and, furthermore, demonstrate that subsequent testicular function can be prematurely restored with a daily low-dose GnRH agonist treatment. Daily buserelin treatment for approximately 60 days starting 12 weeks after GnRH immunisation stimulated the secretion of gonadotropins sufficiently to restore basal gonadal steroid synthesis. This stimulation induced the recovery of testicular tissue with functional seminiferous tubules, accompanied by complete spermatogenesis.
Immunisation against GnRH disrupts testosterone biosynthesis for several weeks, but the duration can vary markedly among individual stallions with gonadal function being suppressed for at least 6 months (Dowsett et al. 1993, 1996; Malmgren et al. 2001; Clement et al. 2005; Turkstra et al. 2005; Burger et al. 2006; Janett et al. 2009; Ponthier et al. 2020; Birrell et al. 2021; Gautier et al. 2022). Complete recovery in stallions not stimulated with GnRH agonist lasts on average 12 months after the initial GnRH vaccination (Clement et al. 2005; Burger et al. 2006; Janett et al. 2009). The decrease in blood testosterone concentrations is a consequence of inhibited gonadotropin, particularly LH secretion. Concentrations of LH in GnRH-immunised stallions were completely suppressed for 5 weeks after the first vaccination. At the same time, FSH concentrations decreased but were still detectable throughout the study. Similar observations were made in GnRH-immunised mares (Garza et al. 1986). Both LH and FSH synthesis are stimulated by GnRH, but gonadotropin synthesis continues to some extent, when GnRH stimulation is markedly reduced. This assumption is further supported by a previous study where slow-release implants containing the GnRH agonist deslorelin, although inducing a downregulation of GnRH receptors did not suppress testicular function in stallions. This suggests that despite suppressive GnRH, the release of LH and FSH is sufficient to maintain testicular function (Gautier et al. 2018).
In this study, although buserelin treatment of GnRH-vaccinated stallions stimulated a small increase in LH secretion, it was already efficient in re-stimulating testosterone secretion (Gautier et al. 2022). At the same time, FSH concentrations increased markedly in response to buserelin in GnRH-vaccinated stallions. Interestingly, FSH concentration in the blood increased even more in the non-treated control stallions after the first hemicastration, indicating a marked reduction of the testicular negative feedback by inhibin secretion produced in the Sertoli cells (Stewart and Roser 1998). Although inhibin secretion was not determined in the present study, it can be assumed that hemicastration resulted in a considerable decrease of inhibin secretion.
Plasma oestradiol concentration was reduced by GnRH vaccination but increased progressively during buserelin treatment to concentrations only slightly lower than in control stallions. This is in agreement with the finding of low oestrone sulfate concentrations and disturbed sperm production in subfertile stallions (Roser and Hughes 1992). Stallion testes produce considerable amounts of oestrogens (Raeside 1979). Reduced oestrogen concentrations can be caused by lower testosterone availability for conversion into oestrogens in the testes (Dorrington et al. 1978) or reduced steroidogenic activity of the Leydig cells (Raeside and Renaud 1983; Roser 1995).
In this study, the concentration of AMH was neither affected by GnRH vaccination nor by buserelin treatment. In a previous study, plasma AMH concentrations in stallions were also not affected by GnRH receptor downregulation with the GnRH agonist deslorelin, indicating that the endocrine function of Sertoli cells does not depend on GnRH (Gautier et al. 2018). In the testes, AMH is exclusively released from Sertoli cells (Josso et al. 1993) but its regulation appears to be independent from GnRH and also gonadotropins.
Disruption of testicular stimulation by GnRH immunisation caused a severe disorganisation of the seminiferous tubules, disruption of spermatogenesis, and the absence of elongated spermatids. Indeed, spermatogenesis requires a local environment with high concentrations of testosterone (Sharpe 1994). In the present study, the relative distribution of seminiferous tubules to interstitial testicular tissue was reduced in GnRH-vaccinated stallions. This resembles the situation in prepubertal stallions (Dowsett et al. 1991, 1996; Botha et al. 2022). Vaccination against GnRH also induced a decrease in seminiferous tubule diameter, which is in agreement with previous studies on GnRH vaccination of prepubertal stallions (Malmgren et al. 2001; Turkstra et al. 2005; Botha et al. 2022). In the present investigation, vaccination also induced a decrease in lumen diameter and epithelial height of the epididymis. Furthermore, very few or no mature sperm were present in the lumen of the epididymal duct in vaccinated stallions. Buserelin treatment restored physiological histomorphology in the testes of GnRH-vaccinated stallions, although group differences in epididymal histology were still clearly evident at the time of the second hemicastration. This indicates a delayed restoration of epididymal function in comparison to testicular function after GnRH vaccination and buserelin re-stimulation. This could negatively affect epididymal sperm maturation and therefore fertility.
The histological deterioration of testicular tissue after GnRH vaccination was associated with altered expression of steroidogenic markers. Both mRNA abundance and protein expression of LH-R and CYP19A1 were reduced by GnRH vaccination, whereas FSH-R mRNA and protein were not changed. After buserelin treatment, testicular LHR mRNA abundance was similar in stallions of both groups, whereas protein expression increased but was still lower in GnRH-vaccinated compared to control stallions at the time of the second hemicastration. These results suggest that GnRH vaccination downregulates the LH-R both at the transcriptional and translational levels. The LH-R downregulation explains the decrease of testosterone concentration in GnRH-vaccinated stallions (Gautier et al. 2022) because testosterone synthesis depends on activation of Leydig cell LH-Rs (Amann 1993). The GnRH agonist treatment re-stimulated transcription of the gene but did not fully restore the efficiency of the translation step, suggesting that a longer buserelin treatment period is probably more effective.
In the present study, the increased expression of FSHR mRNA in the testes of GnRH-vaccinated compared to control stallions is in agreement with findings in deslorelin-implanted male cats (Mehl et al. 2016). These authors suggested a compensatory mechanism after suppression of the endogenous release of GnRH and/or FSH. In the present study, FSHR mRNA abundance was similar in both groups at the second hemicastration. At no stage were differences noted in FSH-R protein expression, thus agreeing with observations described in deslorelin-treated cats (Mehl et al. 2016). In prepubertal mammals, FSH is well-known for a major role in the initiation of the first wave of spermatogenesis, whereas in pubertal mammals, spermatogenesis is mainly androgen-dependent (Russell et al. 1987; Schteingart et al. 1995; Roser 2008; O’Shaughnessy 2014). The disruption of spermatogenesis in GnRH-vaccinated stallions of the present study is therefore most likely a result of decreased testosterone synthesis, induced by the absence of LH stimulation in Leydig cells. Independent from testosterone, however, a role of FSH for the maintenance of spermatogenesis via paracrine mechanism has been suggested (Johnson et al. 1997).
Both CYP19A1 mRNA and protein abundance were markedly reduced by GnRH vaccination. The GnRH agonist treatment increased CYP19A1 mRNA and protein abundance again, but both remained lower than in the control group. This downregulation and restimulation of CYP19A1 is of particular importance in male horses, because of the large amounts of oestrogens produced in the testes (Raeside and Christie 1997; Lemazurier et al. 2002).
The main objective of this study was not to investigate the effects of hemicastration on testicular function in stallions; however, some interesting observations can be addressed. After hemicastration, testis volume increased in controls stallions and plasma testosterone concentration was not reduced, indicating compensatory hypertrophy of the remaining testis (Gautier et al. 2022) and confirming previous findings (Hoagland et al. 1986; Jung et al. 2020). In contrast, testicular volume and gonadosomatic index decreased in GnRH-vaccinated stallions (Gautier et al. 2022). Similar to testosterone, oestradiol concentration in the control stallions was also not affected by hemicastration. These results clearly suggest that testicular steroid synthesis required for normal reproductive function is maintained by the remaining testis. On the contrary, plasma AMH concentration decreased to approximately 50% of the original concentration in stallions of both groups after hemicastration, indicating that there is no immediate compensation for AMH synthesis by the Sertoli cells. In stallions during the breeding season, testicular weight and daily sperm production per testis increase due to an increase in Sertoli cell numbers (Johnson and Thompson 1983). This suggests that proliferation of the Sertoli cells was not yet detectable in the stallions of our study. An increase in testicular volume and the gonadosomatic index became, however, apparent in the control stallions as early as 40 days after the first hemicastration (Gautier et al. 2022). Although it was not yet associated with an increase in total sperm count, compensatory hypertrophy of the remaining testis was already initiated. The second hemicastration occurred too early to allow for a more pronounced outcome.
In conclusion, a daily low-dose GnRH agonist treatment for approximately 60 days in stallions vaccinated against GnRH approximately 12 weeks earlier, re-stimulates gonadotropin secretion sufficiently to induce a premature recovery of testicular tissue with functional organisation and effective spermatogenesis. A longer period, however, apparently is required for complete recovery of all testicular functions. With further improvements in the protocol, daily buserelin treatment may be recommended for treatment of the GnRH-vaccinated stallions with persistent inhibition in testicular function and fertility.
Data availability
The data that support this study will be shared upon reasonable request to the corresponding author.
Acknowledgements
This research was supported using resources of the VetCore Facility (VetBioBank) of the University of Veterinary Medicine Vienna.
References
Aurich C (2018) Horse overview. In ‘Encyclopedia of reproduction, Vol. 1’. (Ed. MK Skinner) pp. 508–514. (Academic Press). 10.1016/B978-0-12-809633-8.20635-3
Birrell JR, Schulman ML, Botha AE, Ganswindt A, Fosgate GT, Bertschinger HJ (2021) Vaccination against GnRH as a prelude to surgical castration of horses. Equine Veterinary Journal 53, 1141-1149.
| Crossref | Google Scholar | PubMed |
Botha AE, Schulman ML, Birrell J, du Plessis L, Laver PN, Soley J, Colenbrander B, Bertschinger HJ (2022) Effects of an anti-gonadoliberin releasing hormone vaccine on testicular, epididymal and spermatogenic development in the horse. Reproduction in Domestic Animals 57, 919-927.
| Crossref | Google Scholar | PubMed |
Burger D, Janett F, Vidament M, Stump R, Fortier G, Imboden I, Thun R (2006) Immunization against GnRH in adult stallions: effects on semen characteristics, behaviour, and shedding of equine arteritis virus. Animal Reproduction Science 94, 107-111.
| Crossref | Google Scholar |
Clement F, Vidament M, Daels P, van der Meer F, Larry JL, Colenbrander B, Turkstra J (2005) Immunocastration in stallions: effect on spermatogenesis and behaviour. Animal Reproduction Science 89, 230-233.
| Google Scholar | PubMed |
Dorrington JH, Fritz IB, Armstrong DT (1978) Control of testicular estrogen synthesis. Biology of Reproduction 18, 55-64.
| Crossref | Google Scholar | PubMed |
Dowsett KF, Pattie WA, Knott LM, Jackson AE, Hoskinson RM, Rigby RP, Moss BA (1991) A preliminary study of immunological castration in colts. Journal of Reproduction and Fertility Supplement 44, 183-190.
| Google Scholar | PubMed |
Dowsett KF, Tshewang U, Knott LM, Jackson AE, Trigg TE (1993) Immunocastration of colts and immunospeying of fillies. Immunology & Cell Biology 71, 501-508.
| Crossref | Google Scholar | PubMed |
Dowsett KF, Knott LM, Tshewang U, Jackson AE, Bodero D, Trigg TE (1996) Suppression of testicular function using two dose rates of a reversible water soluble gonadotrophin releasing hormone (GnRH) vaccine in colts. Australian Veterinary Journal 74, 228-235.
| Crossref | Google Scholar | PubMed |
Einarsson S, Brunius C, Wallgren M, Lundström K, Andersson K, Zamaratskaia G, Rodriguez-Martinez H (2011) Effects of early vaccination with Improvac® on the development and function of reproductive organs of male pigs. Animal Reproduction Science 127, 50-55.
| Crossref | Google Scholar | PubMed |
Garza F, Jr., Thompson DL, Jr., French DD, Wiest JJ, George RLS, Ashley KB, Jones LS, Mitchell PS, Mcneill DR (1986) Active immunization of intact mares against gonadotropin-releasing hormone: differential effects on secretion of luteinizing hormone and follicle-stimulating hormone. Biology of Reproduction 35, 347-352.
| Crossref | Google Scholar | PubMed |
Gautier C, Schmidt K, Aurich J, Aurich C (2018) Effects of implants containing the GnRH agonist deslorelin on testosterone release and semen characteristics in Shetland stallions. Animal Reproduction Science 195, 230-241.
| Crossref | Google Scholar | PubMed |
Gautier C, Aurich J, Kaps M, Okada CTC, Wagner L-H, Melchert M, Aurich C (2022) Re-stimulation of testicular function in GnRH-vaccinated stallions by daily GnRH agonist treatment. Theriogenology 194, 27-34.
| Crossref | Google Scholar | PubMed |
Herrera-Luna CV, Scarlet D, Walter I, Aurich C (2016) Effect of stallion age on the expression of LH and FSH receptors and aromatase P450 in equine male reproductive tissues. Reproduction, Fertility and Development 28, 2016-2026.
| Crossref | Google Scholar | PubMed |
Hoagland TA, Ott KM, Dinger JE, Mannen K, Woody CO, Riesen JW, Daniels W (1986) Effects of unilateral castration on morphologic characteristics of the testis in one-, two-, and three-year-old stallions. Theriogenology 26, 397-405.
| Crossref | Google Scholar | PubMed |
Janett F, Stump R, Burger D, Thun R (2009) Suppression of testicular function and sexual behavior by vaccination against GnRH (Equity™) in the adult stallion. Animal Reproduction Science 115, 88-102.
| Crossref | Google Scholar | PubMed |
Johnson L, Thompson DL, Jr. (1983) Age-related and seasonal variation in the Sertoli cell population, daily sperm production and serum concentrations of follicle-stimulating hormone, luteinizing hormone and testosterone in stallions. Biology of Reproduction 29, 777-789.
| Crossref | Google Scholar | PubMed |
Johnson L, Blanchard TL, Varner DD, Scrutchfield WL (1997) Factors affecting spermatogenesis in the stallion. Theriogenology 48, 1199-1216.
| Crossref | Google Scholar | PubMed |
Josso N, Cate RL, Picard J-Y, Vigier B, di Clemente N, Wilson C, Imbeaud S, Pepinsky RB, Guerrier D, Boussin L, Legeai L, Carré-Eusèbe D (1993) Anti-müllerian hormone: the Jost factor. In ‘Recent progress in hormone research, Vol. 48’. (Ed. CW Bardin) pp. 1–59. (Academic Press) doi:10.1016/b978-0-12-571148-7.50005-1
Jung H, Lee G, Kim J, Lee J-W, Yoon M (2020) Effects of hemicastration on testes and testosterone concentration in stallions. Journal of Equine Veterinary Science 92, 103166.
| Crossref | Google Scholar | PubMed |
Kenkel CD, Aglyamova G, Alamaru A, Bhagooli R, Capper R, Cunning R, deVillers A, Haslun JA, Hédouin L, Keshavmurthy S, Kuehl KA, Mahmoud H, McGinty ES, Montoya-Maya PH, Palmer CV, Pantile R, Sánchez JA, Schils T, Silverstein RN, Squiers LB, Tang P-C, Goulet TL, Matz MV (2011) Development of gene expression markers of acute heat-light stress in reef-building corals of the genus Porites. PLoS ONE 6, e26914.
| Crossref | Google Scholar |
Landini G, Martinelli G, Piccinini F (2021) Colour deconvolution: stain unmixing in histological imaging. Bioinformatics 37, 1485-1487.
| Crossref | Google Scholar | PubMed |
Lemazurier E, Moslemi S, Sourdaine P, Desjardins I, Plainfosse B, Seralini G-E (2002) Free and conjugated estrogens and androgens in stallion semen. General and Comparative Endocrinology 125, 272-282.
| Crossref | Google Scholar | PubMed |
Livak KJ, Schmittgen TD (2001) Analysis of relative gene expression data using real-time quantitative PCR and the 2−ΔΔCT method. Methods 25, 402-408.
| Crossref | Google Scholar | PubMed |
Malmgren L, Andresen Ø, Dalin A-M (2001) Effect of GnRH immunisation on hormonal levels, sexual behaviour, semen quality and testicular morphology in mature stallions. Equine Veterinary Journal 33, 75-83.
| Crossref | Google Scholar | PubMed |
Mehl NS, Khalid M, Srisuwatanasagul S, Swangchan-uthai T, Sirivaidyapong S (2016) GnRH-agonist implantation of prepubertal male cats affects their reproductive performance and testicular LH receptor and FSH receptor expression. Theriogenology 85, 841-848.
| Crossref | Google Scholar | PubMed |
Miszczak F, Burger D, Ferry B, Legrand L, Fortier G, Lainé A-L, Vabret A, Pronost S, Vidament M (2020) Anti-GnRH vaccination of stallions shedding equine arteritis virus in their semen: a field study. Veterinarski Arhiv 90, 543-556.
| Crossref | Google Scholar |
Nett TM, Holtan DW, Estergreen VL (1975) Serum levels of estrogens, luteinizing hormone and prolactin in pregnant mares. Journal of Reproduction and Fertility Supplement 23, 457-462.
| Google Scholar |
Nett TM, Pickett BW, Squires EL (1979) Effects of Equimate (ICI-81008) on levels of luteinizing hormone, follicle-stimulating hormone and progesterone during the estrous cycle of the mare. Journal of Animal Science 48, 69-75.
| Crossref | Google Scholar | PubMed |
O’Shaughnessy PJ (2014) Hormonal control of germ cell development and spermatogenesis. Seminars in Cell & Developmental Biology 29, 55-65.
| Crossref | Google Scholar | PubMed |
Pfaffl MW, Tichopad A, Prgomet C, Neuvians TP (2004) Determination of stable housekeeping genes, differentially regulated target genes and sample integrity: BestKeeper – Excel-based tool using pair-wise correlations. Biotechnology Letters 26, 509-515.
| Crossref | Google Scholar | PubMed |
Ponthier J, Rigaux G, Parrilla-Hernandez S, Egyptien S, Gatez C, Carrasco Leroy C, Deleuze S (2020) Case report: suppression of harem stallion behavior and fertility following anti-gonadotropin-releasing hormone vaccination of a captive wild Przewalski’s horse (Equus ferus przewalskii). Frontiers in Veterinary Science 7, 569185.
| Crossref | Google Scholar | PubMed |
Rabb MH, Thompson DL, Barry BE, Colborn DR, Hehnke KE, Garza F (1990) Effects of active immunization against GnRH on LH, FSH and prolactin storage, secretion and response to their secretagogues in pony geldings. Journal of Animal Science 68, 3322-3329.
| Crossref | Google Scholar | PubMed |
Raeside JI (1979) Seasonal changes in the concentration of estrogens and testosterone in the plasma of the stallion. Animal Reproduction Science 1, 205-212.
| Crossref | Google Scholar |
Raeside JI, Christie HL (1997) Estrogen concentrations in semen of the stallion. Animal Reproduction Science 48, 293-300.
| Crossref | Google Scholar | PubMed |
Raeside JI, Renaud RL (1983) Estrogen and androgen production by purified Leydig cells of mature boars. Biology of Reproduction 28, 727-733.
| Crossref | Google Scholar | PubMed |
Roser JF (1995) Endocrine regulation of reproductive function in fertile, subfertile and infertile stallions. Reproduction in Domestic Animals 30, 245-250.
| Crossref | Google Scholar |
Roser JF (2008) Regulation of testicular function in the stallion: an intricate network of endocrine, paracrine and autocrine systems. Animal Reproduction Science 107, 179-196.
| Crossref | Google Scholar | PubMed |
Roser JF, Hughes JP (1992) Seasonal effects on seminal quality, plasma hormone concentrations, and GnRH-induced LH response in fertile and subfertile stallions. Journal of Andrology 13, 214-223.
| Crossref | Google Scholar | PubMed |
Ruifrok AC, Johnston DA (2001) Quantification of histochemical staining by color deconvolution. Analytical and Quantitative Cytology and Histology 23, 291-299.
| Google Scholar | PubMed |
Russell LD, Alger LE, Nequin LG (1987) Hormonal control of pubertal spermatogenesis. Endocrinology 120, 1615-1632.
| Crossref | Google Scholar | PubMed |
Saez JM, Avallet O, Naville D, Perrard-Sapori MH, Chatelain PG (1989) Sertoli-leydig cell communications. Annals of the New York Academy of Sciences 564, 210-231.
| Crossref | Google Scholar | PubMed |
Scarlet D, Ertl R, Aurich C, Steinborn R (2015) The orthology clause in the next generation sequencing era: novel reference genes identified by RNA-seq in humans improve normalization of neonatal equine ovary RT-qPCR data. PLoS ONE 10, e0142122.
| Crossref | Google Scholar | PubMed |
Scarlet D, Wulf M, Kuhl J, Köhne M, Ille N, Conley AJ, Aurich C (2018) Anti-Müllerian hormone profiling in prepubertal horses and its relationship with gonadal function. Theriogenology 117, 72-77.
| Crossref | Google Scholar | PubMed |
Schindelin J, Arganda-Carreras I, Frise E, Kaynig V, Longair M, Pietzsch T, Preibisch S, Rueden C, Saalfeld S, Schmid B, Tinevez J-Y, White DJ, Hartenstein V, Eliceiri K, Tomancak P, Cardona A (2012) Fiji: an open-source platform for biological-image analysis. Nature Methods 9, 676-682.
| Crossref | Google Scholar | PubMed |
Schteingart HF, Meroni SB, Pellizzari EH, Pérez AL, Cigorraga SB (1995) Regulation of Sertoli cell aromatase activity by cell density and prolonged stimulation with FSH, EGF, insulin and IGF-I at different moments of pubertal development. The Journal of Steroid Biochemistry and Molecular Biology 52, 375-381.
| Crossref | Google Scholar | PubMed |
Stewart BL, Roser JF (1998) Effects of age, season, and fertility status on plasma and intratesticular immunoreactive (IR) inhibin concentrations in stallions. Domestic Animal Endocrinology 15, 129-139.
| Crossref | Google Scholar | PubMed |
Thompson DL, Jr. (2000) Immunization against GnRH in male species (comparative aspects). Animal Reproduction Science 60–61, 459-469.
| Crossref | Google Scholar | PubMed |
Turkstra JA, van der Meer FJUM, Knaap J, Rottier PJM, Teerds KJ, Colenbrander B, Meloen RH (2005) Effects of GnRH immunization in sexually mature pony stallions. Animal Reproduction Science 86, 247-259.
| Crossref | Google Scholar | PubMed |
Vidament M, Ferry B, Laine AL, Wimel L, Miszczak F, Burger D, Briant C, Decourt C, Caraty A (2010) L’injection journalière d’un agoniste de gnRH restaure la fonction de reproduction chez des étalons castrés à long terme par une immunisation anti-GnRH. In ‘Proceedings 36ème Journée de la Recherche Equine’, Paris, pp. 71–80. (Institut Français de Cheval et de l’Equitation, IFCE) [In French]