Physiological implications of COVID-19 in reproduction: angiotensin-converting enzyme 2 a key player
G. Taru Sharma

A Department of Physiology, Indian Council of Agricultural Research (ICAR) – Indian Veterinary Research Institute, Izatnagar, Bareilly, Uttarpradesh, India.
B Department of Physiology, Kerala Veterinary and Animal Sciences University, Lakkidi, Pookode, Kerala, India.
C Department of Physiology, UP Pandit Deen Dayal Upadhyaya Pashu Chikitsa Vigyan Vishwavidyalaya Evam Go Anusandhan Sansthan, Mathura, Uttarpradesh, India.
D Animal Husbandry Department, Government of Kerala, Kerala, India.
E Department of Pathology, Indian Council of Agricultural Research (ICAR) – Indian Veterinary Research Institute, Izatnagar, Bareilly, Uttarpradesh, India.
F Corresponding author. Email: gts553@gmail.com
Reproduction, Fertility and Development 33(6) 381-391 https://doi.org/10.1071/RD20274
Submitted: 16 October 2020 Accepted: 19 January 2021 Published: 18 March 2021
Journal Compilation © CSIRO 2021 Open Access CC BY
Abstract
The COVID-19 outbreak, caused by severe acute respiratory syndrome coronavirus-2 (SARS-CoV-2), was first identified in China, and it has quickly become a global threat to public health due to its rapid rate of transmission and fatalities. Angiotensin-converting enzyme 2 (ACE2) has been identified as a receptor that mediates the entry of SARS-CoV-2 into human cells, as in the case of severe acute respiratory syndrome coronavirus (SARS-CoV). Several studies have reported that ACE2 expression is higher in Leydig, Sertoli and seminiferous ductal cells of males, as well as in ovarian follicle cells of females, suggesting possible potential pathogenicity of the coronavirus in the reproductive system. Higher ACE2 expression in the human placenta and reports of vertical transmission of SARS-CoV-2 among clinical cases have increased the relevance of further studies in this area. This review focuses on the interaction between SARS-CoV-2 and the ACE2 receptor and speculates on the mechanistic interplay in association with male and female reproductive physiology. In addition, based on the available literature, we discuss the alleged sex differences in terms of the infectivity of SARS-CoV-2, which is claimed greater among males, and further explore the physiological role of ACE2 and 17β-oestradiol for the same.
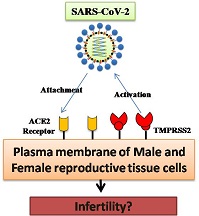
Keywords: COVID-19, SARS-CoV-2, ACE2 receptors, reproductive physiology, gender bias.
Introduction
The ongoing global COVID-19 pandemic first emerged in Wuhan, China, in December 2019. COVID-19 is caused by a novel strain of coronavirus, namely severe acute respiratory syndrome coronavirus-2 (SARS-CoV-2), which belongs to Family Coronaviridae. Coronaviruses are known to cause illness in both animals and humans. From the physiological point of view, the study of SARS-CoV-2 infection is interesting because the virus uses angiotensin converting enzyme (ACE) 2 receptors to enter host cells after priming of the spike protein of SARS-CoV-2 by transmembrane protease serine-2 (TMPRSS2; Hoffmann et al. 2020). The versatile nature of ACE2 receptors and their widespread distribution in the host body have perplexed the research community while addressing the pathogenesis of SARS-CoV-2 inside the body. However, the extensive distribution of ACE2 in the testes, ovary, placenta, and associated cells has drawn the attention of many researchers in recent times to gain an understanding of their role in changes in a host’s fertilising competence during infection with SARS-CoV-2.
Epidemiological data pertaining to infections with severe acute respiratory syndrome coronavirus (SARS-CoV) and Middle East respiratory syndrome coronavirus (MERS-CoV) indicate that the frequency of cases and deaths are higher among men than women (Karlberg et al. 2004; Channappanavar et al. 2017). The reason for male vulnerability to coronavirus may be explained by a clear sex prejudice (as detailed in the ‘Sex differences in COVID-19 infections’ section below) connected to scientific, social, and cultural roots, lifestyle differences and reproductive hormones. COVID-19 impact-related sex-disaggregated data is important for reducing health inequities in reproductive medicine. Although the global data show sex differences in COVID-19 susceptibility and mortality, systematic studies are lacking. Thus, this review discusses the physiological and biochemical effects of COVID-19 on reproductive physiology and explores the possible role of ACE2 receptors and reproductive hormones in the alleged sex differences in infection. An understanding of these issues may provide novel insights into the treatment of COVID-19 patients, with a special emphasis on reproductive health issues.
Possible effects of COVID-19 on the male reproductive system
The importance of studying the effects of COVID-19 on male reproduction arises from the fact that ACE2 is highly expressed in the testis, in both germ and somatic cells (Shen et al. 2020; Wang and Xu 2020). The expression of furin domains in the epididymis of men supports the risk of localisation of SARS-CoV-2 in the male reproductive tract (Thimon et al. 2006). Moreover, the transmembrane protein TMPRSS2, used for S-protein priming by the virus, is highly expressed in the epithelial cells of the prostate lumen (Chen et al. 2010) and orchitis was reported as a postinfection complication of the previous SARS-CoV outbreak in China in 2002 (Xu et al. 2006). Although feline coronavirus was reported to cause orchitis in cats (Sigurðardóttir et al. 2001), there are no reports regarding the association between orchitis and SARS-CoV-2.
Paoli et al. (2020) reported the absence of viral RNA in the seminal fluid of a man with a history of COVID-19 infection. However, the latest research letter from Li et al. (2020a) drew attention to the reporting of positive SARS-CoV-2 semen samples in severe COVID-19 cases, which signifies the possible infection of the testes and other reproductive structures by COVID-19. A viral breach of the immune-privileged environment of the testis may cause inflammation and an autoimmune attack of sperm cells, leading to infertility. A recent study reported a significant increase in serum LH concentrations and a marked decrease in the serum testosterone to LH ratio, thus resulting in subclinical or compensated hypogonadism in male COVID-19 patients (Pozzilli and Lenzi 2020). It is also possible that although a COVID-19 infection may no longer appear active, the virus may continue its replication silently, finally breaking through the blood-testes barrier, leading to the establishment of pathogenesis in the otherwise secure and controlled microenvironment of the testes.
Testicular ACE2
ACE2 is a versatile enzyme that is mostly associated with cardiovascular and renal functions; however, the distribution of ACE2 in the testes and reproductive cells indicates its substantial involvement in the regulation of the fertilising competence of spermatozoa. Gianzo et al. (2018) demonstrated the presence of ACE2 receptors located on the human sperm equatorial segment using immunofluorescence. ACE2 activity was found to be 13-fold higher in the sperm plasma membrane than in seminal plasma (Shibahara et al. 2001); in addition, ACE2 activity is higher in post- than prepubertal testes (Douglas et al. 2004). The wider distribution of the ACE2 receptors in testicular tissues may be used by SARS-CoV-2, and may result in serious outcomes in terms of the regulation of sperm fertilising competence. Furthermore, the involvement of the local renin–angiotensin system (RAS) in male reproduction was identified by the presence of several RAS components (i.e. prorenin, renin, angiotensinogen, angiotensin (Ang) I, AngII, ACE and ACE2) in the testes and epididymides of humans and other mammals (Langford et al. 1993; Fuchs et al. 2005; Deguchi et al. 2007).
There are two ACE isoenzymes in the testis, namely the somatic (sACE) and testicular (tACE) isoforms, whereas the testicular and somatic isoforms of ACE2 are identical. Among all the tissues in the body, the testes have nearly the highest levels of ACE2 mRNA and protein expression (Fan et al. 2021). Interestingly, the testicular expression of ACE2 is age related and is highest in men in their 30s and lowest in men in their 60s. These findings suggest a higher susceptibility of younger men to COVID-19, probably due to higher ACE2 expression.
Proposed mechanistic pathways of SARS-COV-2 and testicular disruption of spermatogenesis
Even though no studies have been reported regarding the impairment of spermatogenesis during COVID-19 infection, an interplay of different cascades that may be involved in disrupting the spermatogenic cycle inside the testes during COVID-19 infection can be proposed.
The most likely mechanism is disruption of the normal spermatogenesis pathway by SARS-COV-2, facilitated by ACE2 receptors, by targeting the RAS and its associated pathways (Fig. 1). ACE2 is the main Ang-(1–7)-forming enzyme, either through the hydrolysis of the potent vasoconstrictor AngII (Vickers et al. 2002) or the hydrolysis of AngI to inactive Ang-(1–9), which is subsequently converted to Ang-(1–7) by ACE (Donoghue et al. 2000; Burrell et al. 2004; Rice et al. 2004). Ang-(1–7) is reported to act on the Mas-related G-protein-coupled receptors (MRGPRS), thereby antagonising the biological effects of AngII. Bradykinin (BK), one of the most important peptides regulating water and ion balance in the body, is a part of the kinin–kallikrein system. In the testes, BK has been reported to have roles in prespermatogonial cell proliferation in vitro (Atanassova et al. 1998), anion secretion in the epididymis and vas deferens (Cheuk et al. 2002; Pierucci-Alves and Schultz 2008), smooth muscle contraction and prostaglandin production in the vas deferens (Peredo and Celuch 2001), as well as to affect the motility and vitality of ejaculated spermatozoa (Somlev and Subev 1998). The effects of ACE2 in the male reproductive system of humans have been demonstrated by the severe impairment of spermatogenesis in infertile subjects who have lower levels of ACE2, Ang-(1–7) and MRGPRS compared with fertile subjects (Reis et al. 2010). Further, the localisation of ACE2 in Leydig cells and a marked reduction in testes weight in Mas-deficient mice (Leal et al. 2009) provide substantial evidence for a role of ACE2 in regulating spermatogenesis.
Another mechanism is based on the interplay between SARS-CoV-2 and ACE2, along with changes in the intratesticular environment. Because SARS-COV-2 uses the ACE2 receptors to enter testicular cells, there are four possibilities for the testicular disruption of spermatogenesis (Fig. 2). First, the virus may attack the Sertoli and Leydig cells, abruptly stopping spermatogenic events and causing a marked reduction in the sperm count. Second, SARS-COV-2 can target the seminiferous epithelium containing spermatogonial cells, resulting in immune and inflammatory reactions that result in cytokine-mediated bursts inside the tubules and abrupt damage to the testicular epithelium. Third, the entry of SARS-CoV-2 into Sertoli cells may lead to a disturbance in the blood–testis barrier (BTB), immunological and inflammatory responses and indirect impairment of the functional dynamics of developing spermatozoa. The BTB may not constitute a perfect barrier to viruses, as evidenced by active viral replication found in human semen during infections with the Zika (Mead et al. 2018), Ebola (Fischer and Wohl 2016) and Marburg (Brainard et al. 2016) viruses. Finally, the virus may attack the myoid cells, thereby affecting the contractile properties of tubular cells.
![]() |
The third possible mechanism involves the interaction of two major cells of the testes, namely Leydig and Sertoli cells, with SARS-CoV-2. The interaction of the virus with Leydig cells may hinder the synthesis of testosterone, which is required for signalling and the overall functioning of the testes and sperm cells. Impaired FSH and LH signalling may result in aberrant functions of the testes and lead to infertility.
The proposed mechanisms above are corroborated by a recent study that reported the effect of SARS-CoV-2 infection on gonad function (Ma et al. 2020). That study provided the first direct evidence suggesting that SARS-CoV-2 may target the testis as the major organ of surveillance, replication, and pathogenesis (i.e. the testis is highly prone to infection, probably due to high cellular replication and turn over rate and so as under surveillance by major pathogens).
Implications of COVID-19 infection for testicular function
Patients with classical SARS had massive germ cell destruction, few or no spermatozoa in the seminiferous tubules and fibrotic basement membrane in the testes indicating testicular damage and hyperplasia (Xu et al. 2006). Furthermore, the Gene Set Enrichment Analysis (GSEA) method used in a recent study (Wang and Xu 2020) revealed that gene ontologies (GOs) related to the generation of male gametes, mitochondria and reproduction are downregulated, whereas cell–cell junction, immunity and immunity-related GOs are upregulated in Leydig and Sertoli cells after SARS-CoV-2 infection. Recently, Çayan et al. (2020) reported that serum total testosterone concentrations decreased significantly in COVID-19 patients from baseline levels. These observations were based on a cohort study conducted among COVID-19 patients categorised into three groups: Group I, asymptomatic patients; Group II, symptomatic patients admitted to an internal medicine unit; and Group III, symptomatic patients admitted to an intensive care unit (Çayan et al. 2020). These authors found that serum total testosterone concentrations in males are an important risk factor that significantly affect the severity of and likelihood of mortality due to COVID-19. Çayan et al. (2020) hypothesised that because testosterone is related to the immune system of respiratory organs, hypogonadism may contribute to the higher risk of respiratory infection. These findings suggest that COVID-19 infection dysregulates ACE2-mediated signalling in sperm cells and the testes, predisposing these subjects to the development of infertility and the production of incompetent germ cells (Wang and Xu 2020).
COVID-19 effects in the female reproductive system
Ovarian ACE2 receptors and RAS
ACE2 expression has been reported in primordial, primary, secondary and antral follicles, as well as the stroma and corpora lutea of human ovaries. ACE2 mRNA transcripts were detected in the ovaries of reproductive-age and postmenopausal women (Reis et al. 2011). Thus far, there is no evidence of ACE2 expression in human oocytes. However, it has been reported that ACE2 is present in the stroma and granulosa cells and oocytes of immature rat ovaries, with ACE2 expression enhanced in antral and preovulatory follicles subjected to equine chorionic gonadotrophin treatment (Pereira et al. 2009). In rats, ACE2 expression was upregulated during follicle development and further increased after gonadotrophin stimulation (Pereira et al. 2009) and human chorionic gonadotrophin (hCG) administration (Honorato-Sampaio et al. 2012). As Jing et al. 2020 recently reviewed, ACE2 mRNA is widely expressed in the human ovary, uterus, vagina and placenta.
Members of the local RAS in the ovary, including ACE2, are known to be involved in ovarian physiology and the regulation of follicle development, steroidogenesis, ovulation and atresia. AngII induces steroid secretion, facilitates follicle development and oocyte maturation, contributes to follicle atresia and influences ovulation (Domińska 2020). Ang-(1–7) promotes the production of oestradiol and progesterone and enhances ovulation, the resumption of meiosis (Jing et al. 2020) and the maturation of oocytes in women (Cavallo et al. 2017). The balance between AngII and Ang-(1–7) could regulate the regeneration of the endometrium (Vaz-Silva et al. 2009) and the activity of the myometrium (Vaz-Silva et al. 2012).
Binding of the SARS-CoV spike protein causes downregulation of ACE2 expression in the ovary, which remains low with persistent viral infection (Kuba et al. 2005; Dijkman et al. 2012). It can be hypothesised that, in the ovary, a decrease in ACE2 expression following SARS-CoV-2 infection may disturb the local RAS in the ovary. Disturbances in the local RAS in the ovary are associated with reproductive disorders, such as polycystic ovary syndrome (PCOS), ovarian hyperstimulation syndrome (OHS), ovarian tumours and ectopic pregnancy (Yoshimura 1997). It is unknown whether disturbances to the RAS caused by SARS-CoV-2 infection have any adverse effects on oocyte maturation and ovarian reserve.
Effects of COVID-19 in pregnant women
SARS-CoV-2 infection poses a significant risk to pregnant women and fetuses, causing premature birth (20.8%), fetal distress (26.7%), premature rupture of fetal membranes (13.0%) and Caesarean section (92.6%; Chen et al. 2020b; Ferrazzi et al. 2020; Li et al. 2020b; Liu et al. 2020; Zeng et al. 2020; Zhu et al. 2020). Moreover, approximately 2% of fetal deaths and 0.4% of neonatal deaths have been attributed to COVID-19 in South Korea (Yee et al. 2020). However, Islam et al. (2020) recently reported that, in general, the neonatal mortality due to COVID-19 ranges between 0.5% and 2.5% worldwide. It has also been reported that ACE2 is widely expressed in the human placenta (Valdés et al. 2006), mainly in the syncytiotrophoblast, cytotrophoblast, endothelium and vascular smooth muscle of primary and secondary villi. During early gestation, ACE2 is expressed in the primary and secondary decidual zone, as well as in luminal and glandular epithelial cells, which further increased with advancing gestational age (Pringle et al. 2011). Another study suggested that pregnant mice were susceptible to SARS- CoV infection-induced renal injury because ACE2 levels in the renal tubules of pregnant mice increase by 117% compared with non-pregnant mice (Brosnihan et al. 2003). Thus, in terms of fertility, SARS-CoV-2-infected pregnant females are equally at risk as males.
Pregnant women are more likely to get the disease with a more severe infection than non-pregnant women. During pregnancy, fetal development increases the abdominal volume and pushes the diaphragm upwards, leading to decreased compliance of the chest wall, which eventually leads to a 20–30% reduction in functional residual capacity. To compensate for this, there is an increased tidal volume and hyperventilation. In addition, changes to the nasal mucosa mediated by progesterone during pregnancy may lead to the adhesion of the virus in the upper respiratory tract, making it difficult to clear (Toppozada et al. 1982; Bende and Gredmark 1999). Further, viral infection may lead to increased pulmonary vascular resistance, which may lead to pulmonary hypertension and heart failure (Nelson et al. 1983). According to the current statistics, a significant proportion of COVID-19 deaths are due to dyspnoea (Shi et al. 2020). The incidence of physical dyspnoea in the third trimester of pregnancy is 50–70% (LoMauro and Aliverti 2015). During pregnancy, the immune system also changes to T helper (Th) 2 rather than Th1 dominated, thus making the mother more susceptible to respiratory virus infection (Muallem et al. 2017) and autoantigen attack (Danza et al. 2016). Thus, it can be predicted that the SARS-CoV-2 infection will undoubtedly worsen the degree of breathing difficulties in pregnant women. Further, the expression of ACE2 mRNA in the kidney, placenta and uterus is increased significantly during pregnancy as an adaptive feature in women to regulate blood pressure, but this can also increase the risk of contracting COVID-19 (Zhao et al. 2020). In this context, upregulation of ACE2 during gestation and its effect on pregnancy needs to be further investigated.
Trans-placental and -mammary transmission of SARS-CoV-2
There is an obvious concern about the effects of COVID-19 on fetal development during pregnancy as well as the manifestation of clinical symptoms in newborns after delivery. Initial studies reported negative results for the presence of the virus in babies born to pregnant women diagnosed with severe and non-severe COVID-19 infections (Chen et al. 2020a, 2020b). Some studies detected elevated levels of SARS-CoV-2-specific IgG and IgM, but not the viral particles, in samples collected from infants, suggesting the possibility of transplacental transfer of IgG and the presence of IgM due to possible in utero infection (Dong et al. 2020; Zeng et al. 2020). Similarly, Shek et al. (2003) reported that perinatal transmission of SARS-CoV was not detected in any of the five live-born infants who were born to pregnant women with SARS during the community outbreak in Hong Kong. However, the putative surface receptor ACE2 has been reported to be expressed in the human placenta and its expression is higher than in the lungs, suggesting the possibility of SARS-CoV-2 transmission through the transplacental route (Li et al. 2020c). However, it is unknown whether and how SARS-CoV-2 can be transmitted from the mother to the fetus. A recent comprehensive case report confirmed the vertical transmission of infection in a pregnant woman infected with COVID-19 in the third trimester of pregnancy (Vivanti et al. 2020). The viral load was found to be higher side in placental tissue (11.15 log10) than in amniotic fluid (2.09 log10) and maternal blood (4.87 log10) and was associated with inflammatory lesions found on the placenta. Further, the consequent viraemia and neurological manifestations in the neonate accompanied by virus-positive clinical samples confirmed the transplacental transmission of SARS-CoV-2 during the last week of pregnancy (Vivanti et al. 2020). Further studies are required to provide solid evidence for the vertical transmission of SARS-CoV-2 infection.
It remains unclear whether SARS-CoV-2 can be shed into breast milk and transmitted to a child through breastfeeding. Based on the available data, SARS-CoV-2 transmission may not occur through breast milk (Chen et al. 2020b; Groß et al. 2020; Wu et al. 2020). Even if there is no virus in the milk, contact transmission during breastfeeding should be taken into account and, to minimise the risk of transmission to the neonate, proper quarantine measures need to be enforced for the mother. The United Nations International Children’s Emergency Fund (UNICEF) suggests that COVID-19-positive mothers may continue breastfeeding while using precautions, such as wearing a mask and washing their hands before and after feeding (UNICEF 2020).
Sex differences in COVID-19 infections
Global data suggest that there is a sex difference in mortality from COVID-19. It has been reported that men have been more severely affected than women during the COVID-19 pandemic globally (Chamekh and Casimir 2020). According to statistics available in an open dataset, 2.4-fold more men have died during the recent pandemic than women (Jin et al. 2020). Possible biological reasons for this sex difference that have been proposed by various researchers are summarised in Table 1.
![]() |
Sex-disaggregated data for COVID-19 infections
As per the report from Global Health 50/50 (2020), total sex-disaggregated data for COVID-19 infections are available for 75 countries (Fig. 3). The data indicate that the number of confirmed cases and fatality rates are higher for males, mostly from Asia, America and Africa. In males, the proportion of highest confirmed cases (80%) has been reported in Cambodia and the highest fatality rate (77%) has been reported in Chad. Other major countries with higher fatality in males include Bangladesh (77%), Thailand (76%), Malawi (76%), Nigeria (75%), Afghanistan (75%), Burkina Faso (75%) and Pakistan (74%). In the US, although the number of confirmed cases is higher (52%) in females, the mortality rate is higher (54%) among males. The data from India indicated that the mortality rate is higher in males than females (64% vs 36%).
![]() |
In most European countries, a greater number of females have tested positive for COVID-19 than males. The highest numbers of COVID-19-positive females were reported from Ukraine (60%), Moldova (59%), Wales (56%), Latvia (56%), Scotland (55%), Belgium (55%) and Portugal (55%), and the highest female mortality was reported in Vietnam (63%), Slovenia (54%), Canada (53%), Estonia (53%), Australia (52%), Finland (51%) and Belgium (51%). In European countries, the number of confirmed cases and mortality rates are rising in the female rather than male population, which indicates the need for the collection of more relevant data, more detailed analysis and a greater understanding of the virus.
Differential expression of ACE2 in males and females
The greater susceptibility of males towards COVID-19 infection than females may be due to increased expression of ACE2 receptors in the male reproductive system (Robinson et al. 2020). Higher ACE2 expression in males may be attributed to the virus membrane fusion protein TMPRSS2 being an androgen-responsive gene (Kron et al. 2017; Asselta et al. 2020), in line with previous studies on H1N1 and H7N9 influenza (Cheng et al. 2015). Moreover, only gonadotrophin-dependent expression of ACE2 has been reported in the ovaries (Robinson et al. 2020). Furthermore, some reports suggest that the presence of ACE2 receptors in ovarian and uterine tissues did not facilitate SARS-CoV infection (Ding et al. 2004). There are also reports proving the cardiovascular protective effect of circulating ACE2 and explain the relative protection of females against heart diseases compared with males. Together, this evidence seems to indicate that the putative sex predisposition to COVID-19 infection, with men being more susceptible, may be reflective of a peculiar ACE plasma profile (Ciaglia et al. 2020).
In a study in rats, Dalpiaz et al. (2015) reported that ACE and ACE2 activity was greater in male than female rats. Further gonadectomy in both sexes promoted opposite responses in haemodynamic and cardio vascular parameters which are under the influence of ACE2. Thus, ACE2 activity is said to be sex steroid dependent. There is ample evidence that the expression of AngII and the angiotensin AT1 receptor (classical RAS components) are higher in males, whereas expression of the AT2 receptor and Ang(1–7), non-classical RAS components, is higher in females (Sullivan et al. 2015). In another study in rats, ACE2 expression was markedly reduced with aging in both sexes; although there was no sex-related difference in ACE2 in young adult and middle-aged groups, ACE2 content was significantly higher in old female than male rats (Sullivan et al. 2010).
Hypothetical role of 17β-oestradiol in curbing COVID-19 infection
The primary female sex hormone oestrogen, in both natural and synthetic forms, has an immune-suppressive effect at high concentrations and immunostimulatory activity at low concentrations (Klein and Flanagan 2016). 17β-Oestradiol (E2) is a potent anti-inflammatory hormone and the treatment of female mice with E2 reduces morbidity due to Influenza A virus infection compared with experimentally infected placebo-treated ovariectomised female C57BL/6 mice (Robinson et al. 2014). Oestrogen replacement treatment for 3 weeks in ovariectomised transgenic hypertensive (mRen2) rats resulted in reductions in plasma and tissue ACE activity (aorta and kidney), as well as circulating AngII concentrations, whereas circulating Ang-(1–7) concentrations were increased (Brosnihan et al. 1999). Oestrogen administration increases the expression a disintegrin and metalloproteinase (ADAM) 17 and ADAM10, two putative ectodomain shedders in atherosclerosis (Zhou et al. 2020). This suggests a protective role for oestrogen against cardiovascular events in females, a mechanism potentially accounting for the observed COVID-19 infection sex disparity. Conversely, the decrease in oestrogen in postmenopausal women affects TMPRSS2 expression, the gene being responsive to oestrogens (Baena et al. 2013).
The presence of E2 does not affect virus replication but rather varies the production of chemokines, the pulmonary recruitment of neutrophils and the cytokine responses of virus-specific CD8 T cells to protect females against severe influenza (Robinson et al. 2011). An in vivo study using a mouse model of SARS-CoV infection (Channappanavar et al. 2017) was used to demonstrate that female mice are less susceptible to SARS-CoV infection than male mice. Moreover, Channappanavar et al. (2017) found that the removal of the testes did not affect disease outcomes in male mice, whereas ovariectomy or treating female mice with the oestrogen receptor antagonists ICI-182 and ICI-780 resulted increased mortality to SARS-CoV infection, signifying that oestrogen signalling protects female mice from lethal SARS-CoV infection. In light of the recent pandemic situation, the same research team has stated that this could also be the reason for the male susceptibility to COVID-19 (Iyer et al. 2020).
SARS-CoV-2 and SARS-CoV attack cells via ACE2, and it has been shown that circulating ACE2 levels are higher in men than in women and in patients with diabetes or cardiovascular diseases (Patel et al. 2013). In a study conducted recently using differentiated normal human bronchial epithelial (NHBE) cells treated with E2 in vitro, ACE2 expression was downregulated but the underlying molecular mechanisms have yet to be elucidated (Fig. 4; Stelzig et al. 2020). Conversely, administration of exogenous oestradiol increased ACE2 mRNA expression and activity in the kidney and uterus of pregnant animals (Neves et al. 2008), indicating oestrogen could be a regulator of ACE2, although other hormones could also be involved. In atrial tissue slices prepared from male donors, oestrogen increased the amount of ACE2 mRNA (Brosnihan et al. 2008). These studies reveal the tissue-specific regulation of ACE2 receptor genes by E2.
![]() |
Conclusion
COVID-19 affects the reproductive system of both males and females. The mechanisms and pathways by which the virus affects gonad function, and the consequences, are not yet clear. The robust and widespread distribution of ACE2 in the male reproductive system predisposes males to the entry, localisation, replication and pathogenesis of the virus. SARS-CoV-2 may infect the ovary, uterus, vagina and placenta through the ubiquitous expression of ACE2, and can disturb female reproductive function, resulting in infertility, menstrual disorder and fetal distress. COVID-19 is likely to have long-term effects on male and female reproductive systems, as well as fertility status. Integration of mass data, genomics, proteomics and metabolomics should be used to understand the deleterious effects of this virus. Therefore, future studies are warranted to elucidate the different pathways and cellular responses to virus infection in both the male and female reproductive system. Understanding the key mechanistic pathways will help design suitable strategies for the effective management and treatment of COVID-19 and its associated reproductive impairment.
Conflicts of interest
The authors declare that there is no conflict of interest regarding the publication of this article.
Declaration of funding
No funding was availed for the work.
References
Asselta, R., Paraboschi, E. M., Mantovani, A., and Duga, S. (2020). ACE2 and TMPRSS2 variants and expression as candidates to sex and country differences in COVID-19 severity in Italy. Aging (Albany NY) 12, 10087–10098.| ACE2 and TMPRSS2 variants and expression as candidates to sex and country differences in COVID-19 severity in Italy.Crossref | GoogleScholarGoogle Scholar | 32501810PubMed |
Atanassova, N., Kancheva, L., and Somlev, B. (1998). Bradykinin stimulates prepubertal rat germ cell proliferation in vitro. Immunopharmacology 40, 173–178.
| Bradykinin stimulates prepubertal rat germ cell proliferation in vitro.Crossref | GoogleScholarGoogle Scholar | 9858060PubMed |
Baena, E., Shao, Z., Linn, D. E., Glass, K., Hamblen, M. J., Fujiwara, Y., Kim, J., Nguyen, M., Zhang, X., Godinho, F. J., Bronson, R. T., Mucci, L. A., Loda, M., Yuan, G. C., Orkin, S. H., and Li, Z. (2013). ETV1 directs androgen metabolism and confers aggressive prostate cancer in targeted mice and patients. Genes Dev. 27, 683–698.
| ETV1 directs androgen metabolism and confers aggressive prostate cancer in targeted mice and patients.Crossref | GoogleScholarGoogle Scholar | 23512661PubMed |
Bende, M., and Gredmark, T. (1999). Nasal stuffiness during pregnancy. Laryngoscope 109, 1108–1110.
| Nasal stuffiness during pregnancy.Crossref | GoogleScholarGoogle Scholar | 10401850PubMed |
Bertakis, K. D., Azari, R., Helms, L. J., Callahan, E. J., and Robbins, J. A. (2000). Gender differences in the utilization of health care services. J. Fam. Pract. 49, 147–152.
| 10718692PubMed |
Brainard, J., Pond, K., Hooper, L., Edmunds, K., and Hunter, P. (2016). Presence and Persistence of Ebola or Marburg Virus in Patients and Survivors: A Rapid Systematic Review. PLoS Negl. Trop. Dis. 10, e0004475.
| Presence and Persistence of Ebola or Marburg Virus in Patients and Survivors: A Rapid Systematic Review.Crossref | GoogleScholarGoogle Scholar | 27926934PubMed |
Brosnihan, K. B., Senanayake, P. S., Li, P., and Ferrario, C. M. (1999). Bi-directional actions of estrogen on the renin-angiotensin system. Braz J Med Biol Res 32, 373–381.
| Bi-directional actions of estrogen on the renin-angiotensin system.Crossref | GoogleScholarGoogle Scholar | 10347798PubMed |
Brosnihan, K. B., Neves, L. A., Joyner, J., Averill, D. B., Chappell, M. C., Sarao, R., Penninger, J., and Ferrario, C. M. (2003). Enhanced renal immunocytochemical expression of ANG-(1–7) and ACE2 during pregnancy. Hypertension (Dallas, Tex.: 1979 42, 749–753.
| Enhanced renal immunocytochemical expression of ANG-(1–7) and ACE2 during pregnancy.Crossref | GoogleScholarGoogle Scholar |
Brosnihan, K. B., Hodgin, J. B., Smithies, O., Maeda, N., and Gallagher, P. (2008). Tissue-specific regulation of ACE/ACE2 and AT1/AT2 receptor gene expression by oestrogen in apolipoprotein E/oestrogen receptor-alpha knock-out mice. Exp. Physiol. 93, 658–664.
| Tissue-specific regulation of ACE/ACE2 and AT1/AT2 receptor gene expression by oestrogen in apolipoprotein E/oestrogen receptor-alpha knock-out mice.Crossref | GoogleScholarGoogle Scholar | 18192335PubMed |
Burrell, L. M., Johnston, C. I., Tikellis, C., and Cooper, M. E. (2004). ACE2, a new regulator of the renin-angiotensin system. Trends in endocrinology and metabolism. Trends Endocrinol. Metab. 15, 166–169.
| ACE2, a new regulator of the renin-angiotensin system. Trends in endocrinology and metabolism.Crossref | GoogleScholarGoogle Scholar | 15109615PubMed |
Cavallo, I. K., Dela Cruz, C., Oliveira, M. L., Del Puerto, H. L., Dias, J. A., Lobach, V. N., Casalechi, M., Camargos, M. G., Reis, A. M., Santos, R. A., and Reis, F. M. (2017). Angiotensin-(1–7) in human follicular fluid correlates with oocyte maturation. Hum. Reprod. 32, 1318–1324.
| Angiotensin-(1–7) in human follicular fluid correlates with oocyte maturation.Crossref | GoogleScholarGoogle Scholar | 28402544PubMed |
Çayan, S., Uğuz, M., Saylam, B., and Akbay, E. (2020). Effect of serum total testosterone and its relationship with other laboratory parameters on the prognosis of coronavirus disease 2019 (COVID-19) in SARS-CoV-2 infected male patients: a cohort study. Aging Male , 1–11.
| Effect of serum total testosterone and its relationship with other laboratory parameters on the prognosis of coronavirus disease 2019 (COVID-19) in SARS-CoV-2 infected male patients: a cohort study.Crossref | GoogleScholarGoogle Scholar | 32883151PubMed |
Chamekh, M., and Casimir, G. (2020). Understanding gender-bias in critically ill patients with COVID-19. Front. Med. 7, 564117.
| Understanding gender-bias in critically ill patients with COVID-19.Crossref | GoogleScholarGoogle Scholar |
Channappanavar, R., Fett, C., Mack, M., Ten Eyck, P. P., Meyerholz, D. K., and Perlman, S. (2017). Sex-Based Differences in Susceptibility to Severe Acute Respiratfory Syndrome Coronavirus Infection. Journal of immunology (Baltimore, Md.: 1950) 198, 4046–4053.
| Sex-Based Differences in Susceptibility to Severe Acute Respiratfory Syndrome Coronavirus Infection.Crossref | GoogleScholarGoogle Scholar |
Chen, Y. W., Lee, M. S., Lucht, A., Chou, F. P., Huag, W., Havighurst, T. C., Kim, K., Wang, J. K., Antalis, T. M., Johnson, M. D., and Lin, C. Y. (2010). TMPRSS2, a serine protease expressed in the prostate on the apical surface of luminal epithelial cells and released into semen in prostasomes, is misregulated in prostate cancer cells. Am. J. Pathol. 176, 2986–2996.
| TMPRSS2, a serine protease expressed in the prostate on the apical surface of luminal epithelial cells and released into semen in prostasomes, is misregulated in prostate cancer cells.Crossref | GoogleScholarGoogle Scholar | 20382709PubMed |
Chen, Y., Peng, H., Wang, L., Zhao, Y., Zeng, L., Gao, H., and Liu, Y. (2020a). Infants Born to Mothers With a New Coronavirus (COVID-19). Front Pediatr. 8, 104.
| Infants Born to Mothers With a New Coronavirus (COVID-19).Crossref | GoogleScholarGoogle Scholar | 32266184PubMed |
Chen, H., Guo, J., Wang, C., Luo, F., Yu, X., Zhang, W., Li, J., Zhao, D., Xu, D., Gong, Q., Liao, J., Yang, H., Hou, W., and Zhang, Y. (2020b). Clinical characteristics and intrauterine vertical transmission potential of COVID-19 infection in nine pregnant women: a retrospective review of medical records. Lancet 395, 809–815.
| Clinical characteristics and intrauterine vertical transmission potential of COVID-19 infection in nine pregnant women: a retrospective review of medical records.Crossref | GoogleScholarGoogle Scholar | 32151335PubMed |
Cheng, Z., Zhou, J., To, K. K., Chu, H., Li, C., Wang, D., Yang, D., Zheng, S., Hao, K., Bossé, Y., Obeidat, M., Brandsma, C. A., Song, Y. Q., Chen, Y., Zheng, B. J., Li, L., and Yuen, K. Y. (2015). Identification of TMPRSS2 as a Susceptibility Gene for Severe 2009 Pandemic A(H1N1) Influenza and A(H7N9) Influenza. J. Infect. Dis. 212, 1214–1221.
| Identification of TMPRSS2 as a Susceptibility Gene for Severe 2009 Pandemic A(H1N1) Influenza and A(H7N9) Influenza.Crossref | GoogleScholarGoogle Scholar | 25904605PubMed |
Cheuk, B. L., Ko, W. H., and Wong, P. Y. (2002). COX-dependent and -independent pathways in bradykinin-induced anion secretion in rat epididymis. J. Cell. Physiol. 191, 217–226.
| COX-dependent and -independent pathways in bradykinin-induced anion secretion in rat epididymis.Crossref | GoogleScholarGoogle Scholar | 12064465PubMed |
Ciaglia, E., Vecchione, C., and Puca, A. A. (2020). COVID-19 Infection and Circulating ACE2 Levels: Protective Role in Women and Children. Front Pediatr. 8, 206.
| COVID-19 Infection and Circulating ACE2 Levels: Protective Role in Women and Children.Crossref | GoogleScholarGoogle Scholar | 32391299PubMed |
Dalpiaz, P. L., Lamas, A. Z., Caliman, I. F., Ribeiro, R. F., Abreu, G. R., Moyses, M. R., Andrade, T. U., Gouvea, S. A., Alves, M. F., Carmona, A. K., and Bissoli, N. S. (2015). Sex Hormones Promote Opposite Effects on ACE and ACE2 Activity, Hypertrophy and Cardiac Contractility in Spontaneously Hypertensive Rats. PLoS One 10, e0127515.
| Sex Hormones Promote Opposite Effects on ACE and ACE2 Activity, Hypertrophy and Cardiac Contractility in Spontaneously Hypertensive Rats.Crossref | GoogleScholarGoogle Scholar | 26010093PubMed |
Danza, Á., Ruiz-Irastorza, G., and Khamashta, M. (2016). Pregnancy in systemic autoimmune diseases: Myths, certainties and doubts. Med. Clin. (Barc.) 147, 306–312.
| Pregnancy in systemic autoimmune diseases: Myths, certainties and doubts.Crossref | GoogleScholarGoogle Scholar | 27143525PubMed |
Deguchi, E., Tani, T., Watanabe, H., Yamada, S., and Kondoh, G. (2007). Dipeptidase-inactivated tACE action in vivo: selective inhibition of sperm-zona pellucida binding in the mouse. Biol. Reprod. 77, 794–802.
| Dipeptidase-inactivated tACE action in vivo: selective inhibition of sperm-zona pellucida binding in the mouse.Crossref | GoogleScholarGoogle Scholar | 17634445PubMed |
Dijkman, R., Jebbink, M. F., Deijs, M., Milewska, A., Pyrc, K., Buelow, E., van der Bijl, A., and van der Hoek, L. (2012). Replication-dependent downregulation of cellular angiotensin-converting enzyme 2 protein expression by human coronavirus NL63. J. Gen. Virol. 93, 1924–1929.
| Replication-dependent downregulation of cellular angiotensin-converting enzyme 2 protein expression by human coronavirus NL63.Crossref | GoogleScholarGoogle Scholar | 22718567PubMed |
Ding, Y., He, L., Zhang, Q., Huang, Z., Che, X., Hou, J., Wang, H., Shen, H., Qiu, L., Li, Z., Geng, J., Cai, J., Han, H., Li, X., Kang, W., Weng, D., Liang, P., and Jiang, S. (2004). Organ distribution of severe acute respiratory syndrome (SARS) associated coronavirus (SARS-CoV) in SARS patients: implications for pathogenesis and virus transmission pathways. J. Pathol. 203, 622–630.
| Organ distribution of severe acute respiratory syndrome (SARS) associated coronavirus (SARS-CoV) in SARS patients: implications for pathogenesis and virus transmission pathways.Crossref | GoogleScholarGoogle Scholar | 15141376PubMed |
Domińska, K. (2020). Involvement of ACE2/Ang-(1–7)/MAS1 Axis in the Regulation of Ovarian Function in Mammals. Int. J Mol. Sci. 21, 4572.
| Involvement of ACE2/Ang-(1–7)/MAS1 Axis in the Regulation of Ovarian Function in Mammals.Crossref | GoogleScholarGoogle Scholar |
Dong, L., Tian, J., He, S., Zhu, C., Wang, J., Liu, C., and Yang, J. (2020). Possible Vertical Transmission of SARS-CoV-2 From an Infected Mother to Her Newborn. JAMA 323, 1846–1848.
| Possible Vertical Transmission of SARS-CoV-2 From an Infected Mother to Her Newborn.Crossref | GoogleScholarGoogle Scholar | 32215581PubMed |
Donoghue, M., Hsieh, F., Baronas, E., Godbout, K., Gosselin, M., Stagliano, N., Donovan, M., Woolf, B., Robison, K., Jeyaseelan, R., Breitbart, R. E., and Acton, S. (2000). A novel angiotensin-converting enzyme-related carboxypeptidase (ACE2) converts angiotensin I to angiotensin 1–9. Circ. Res. 87, E1–E9.
| A novel angiotensin-converting enzyme-related carboxypeptidase (ACE2) converts angiotensin I to angiotensin 1–9.Crossref | GoogleScholarGoogle Scholar | 10969042PubMed |
Douglas, G. C., O’Bryan, M. K., Hedger, M. P., Lee, D. K., Yarski, M. A., Smith, A. I., and Lew, R. A. (2004). The novel angiotensin-converting enzyme (ACE) homolog, ACE2, is selectively expressed by adult Leydig cells of the testis. Endocrinology 145, 4703–4711.
| The novel angiotensin-converting enzyme (ACE) homolog, ACE2, is selectively expressed by adult Leydig cells of the testis.Crossref | GoogleScholarGoogle Scholar | 15231706PubMed |
Fan, C., Li, K., Ding, Y., Lu, W. L., and Wang, J. (2021). ACE2 Expression in Kidney and Testis May Cause Kidney and Testis Infection in COVID-19 Patients. Front. Med. 7, 563893.
| ACE2 Expression in Kidney and Testis May Cause Kidney and Testis Infection in COVID-19 Patients.Crossref | GoogleScholarGoogle Scholar |
Ferrazzi, E., Frigerio, L., Savasi, V., Vergani, P., Prefumo, F., Barresi, S., Bianchi, S., Ciriello, E., Facchinetti, F., Gervasi, M. T., Iurlaro, E., Kustermann, A., Mangili, G., Mosca, F., Patanè, L., Spazzini, D., Spinillo, A., Trojano, G., Vignali, M., Villa, A., and Cetin, I. (2020). Vaginal delivery in SARS-CoV-2-infected pregnant women in Northern Italy: a retrospective analysis. BJOG , .
| Vaginal delivery in SARS-CoV-2-infected pregnant women in Northern Italy: a retrospective analysis.Crossref | GoogleScholarGoogle Scholar | 32339382PubMed |
Fischer, W. A., and Wohl, D. A. (2016). Confronting Ebola as a Sexually Transmitted Infection. Clin Infect Dis 62, 1272–1276.
| Confronting Ebola as a Sexually Transmitted Infection.Crossref | GoogleScholarGoogle Scholar | 26936667PubMed |
Fuchs, S., Frenzel, K., Hubert, C., Lyng, R., Muller, L., Michaud, A., Xiao, H. D., Adams, J. W., Capecchi, M. R., Corvol, P., Shur, B. D., and Bernstein, K. E. (2005). Male fertility is dependent on dipeptidase activity of testis ACE. Nat. Med. 11, 1140–1142.
| Male fertility is dependent on dipeptidase activity of testis ACE.Crossref | GoogleScholarGoogle Scholar | 16270063PubMed |
Gianzo, M., Urizar-Arenaza, I., Muñoa-Hoyos, I., Larreategui, Z., Garrido, N., Casis, L., Irazusta, J., and Subirán, N. (2018). Human sperm testicular angiotensin-converting enzyme helps determine human embryo quality. Asian journal of andrology 20, 498–504.
| Human sperm testicular angiotensin-converting enzyme helps determine human embryo quality.Crossref | GoogleScholarGoogle Scholar | 29873314PubMed |
Global Health 50/50 (2020) COVID-19 Sex-Disaggregated Data Tracker. https://globalhealth5050.org/the-sex-gender-and-covid-19-project/the-data-tracker/
Groß, R., Conzelmann, C., Müller, J. A., Stenger, S., Steinhart, K., Kirchhoff, F., and Münch, J. (2020). Detection of SARS-CoV-2 in human breastmilk. Lancet 395, 1757–1758.
| Detection of SARS-CoV-2 in human breastmilk.Crossref | GoogleScholarGoogle Scholar | 32446324PubMed |
Hoffmann, M., Kleine-Weber, H., Schroeder, S., Krüger, N., Herrler, T., Erichsen, S., Schiergens, T. S., Herrler, G., Wu, N. H., Nitsche, A., Müller, M. A., Drosten, C., and Pöhlmann, S. (2020). SARS-CoV-2 Cell Entry Depends on ACE2 and TMPRSS2 and Is Blocked by a Clinically Proven Protease Inhibitor. Cell 181, 271–280.e8.
| SARS-CoV-2 Cell Entry Depends on ACE2 and TMPRSS2 and Is Blocked by a Clinically Proven Protease Inhibitor.Crossref | GoogleScholarGoogle Scholar | 32142651PubMed |
Honorato-Sampaio, K., Pereira, V. M., Santos, R. A., and Reis, A. M. (2012). Evidence that angiotensin-(1–7) is an intermediate of gonadotrophin-induced oocyte maturation in the rat preovulatory follicle. Exp. Physiol. 97, 642–650.
| Evidence that angiotensin-(1–7) is an intermediate of gonadotrophin-induced oocyte maturation in the rat preovulatory follicle.Crossref | GoogleScholarGoogle Scholar | 22247282PubMed |
Islam, M. M., Poly, T. N., Walther, B. A., Yang, H. C., Wang, C. W., Hsieh, W. S., Atique, S., Salmani, H., Alsinglawi, B., Lin, M. C., Jian, W. S., and Jack Li, Y. C. (2020). Clinical Characteristics and Neonatal Outcomes of Pregnant Patients With COVID-19: A Systematic Review. Front. Med. 7, 573468.
| Clinical Characteristics and Neonatal Outcomes of Pregnant Patients With COVID-19: A Systematic Review.Crossref | GoogleScholarGoogle Scholar |
Iyer, S. P., Ensor, J., Anand, K., Hwu, P., Subbiah, V., Flowers, C., and Channappanavar, R. (2020). Higher mortality in men from COVID19 infection-understanding the factors that drive the differences between the biological sexes. medRxiv , .
| Higher mortality in men from COVID19 infection-understanding the factors that drive the differences between the biological sexes.Crossref | GoogleScholarGoogle Scholar |
Jaillon, S., Berthenet, K., and Garlanda, C. (2019). Sexual Dimorphism in Innate Immunity. Clin. Rev. Allergy Immunol. 56, 308–321.
| Sexual Dimorphism in Innate Immunity.Crossref | GoogleScholarGoogle Scholar | 28963611PubMed |
Jin, J. M., Bai, P., He, W., Wu, F., Liu, X. F., Han, D. M., Liu, S., and Yang, J. K. (2020). Gender Differences in Patients With COVID-19: Focus on Severity and Mortality. Front. Public Health 8, 152.
| Gender Differences in Patients With COVID-19: Focus on Severity and Mortality.Crossref | GoogleScholarGoogle Scholar | 32411652PubMed |
Jing, Y., Run-Qian, L., Hao-Ran, W., Hao-Ran, C., Ya-Bin, L., Yang, G., and Fei, C. (2020). Potential influence of COVID-19/ACE2 on the female reproductive system. Mol. Hum. Reprod. 26, 367–373.
| Potential influence of COVID-19/ACE2 on the female reproductive system.Crossref | GoogleScholarGoogle Scholar | 32365180PubMed |
Johnson, H. D., Sholcosky, D., Gabello, K., Ragni, R., and Ogonosky, N. (2003). Sex differences in public restroom handwashing behavior associated with visual behavior prompts. Percept. Mot. Skills 97, 805–810.
| Sex differences in public restroom handwashing behavior associated with visual behavior prompts.Crossref | GoogleScholarGoogle Scholar | 14738345PubMed |
Karlberg, J., Chong, D. S., and Lai, W. Y. (2004). Do men have a higher case fatality rate of severe acute respiratory syndrome than women do? Am. J. Epidemiol. 159, 229–231.
| Do men have a higher case fatality rate of severe acute respiratory syndrome than women do?Crossref | GoogleScholarGoogle Scholar | 14742282PubMed |
Klein, S. L., and Flanagan, K. L. (2016). Sex differences in immune responses. Nat. Rev. Immunol. 16, 626–638.
| Sex differences in immune responses.Crossref | GoogleScholarGoogle Scholar | 27546235PubMed |
Kron, K. J., Murison, A., Zhou, S., Huang, V., Yamaguchi, T. N., Shiah, Y. J., Fraser, M., van der Kwast, T., Boutros, P. C., Bristow, R. G., and Lupien, M. (2017). TMPRSS2-ERG fusion co-opts master transcription factors and activates NOTCH signaling in primary prostate cancer. Nat. Genet. 49, 1336–1345.
| TMPRSS2-ERG fusion co-opts master transcription factors and activates NOTCH signaling in primary prostate cancer.Crossref | GoogleScholarGoogle Scholar | 28783165PubMed |
Kuba, K., Imai, Y., Rao, S., Gao, H., Guo, F., Guan, B., Huan, Y., Yang, P., Zhang, Y., Deng, W., Bao, L., Zhang, B., Liu, G., Wang, Z., Chappell, M., Liu, Y., Zheng, D., Leibbrandt, A., Wada, T., Slutsky, A. S., et al. (2005). A crucial role of angiotensin converting enzyme 2 (ACE2) in SARS coronavirus-induced lung injury. Nat. Med. 11, 875–879.
| A crucial role of angiotensin converting enzyme 2 (ACE2) in SARS coronavirus-induced lung injury.Crossref | GoogleScholarGoogle Scholar | 16007097PubMed |
Langford, K. G., Zhou, Y., Russell, L. D., Wilcox, J. N., and Bernstein, K. E. (1993). Regulated expression of testis angiotensin-converting enzyme during spermatogenesis in mice. Biol Reprod 48, 1210–1218.
| Regulated expression of testis angiotensin-converting enzyme during spermatogenesis in mice.Crossref | GoogleScholarGoogle Scholar | 8391328PubMed |
Leal, M. C., Pinheiro, S. V., Ferreira, A. J., Santos, R. A., Bordoni, L. S., Alenina, N., Bader, M., and França, L. R. (2009). The role of angiotensin-(1–7) receptor Mas in spermatogenesis in mice and rats. J. Anat. 214, 736–743.
| The role of angiotensin-(1–7) receptor Mas in spermatogenesis in mice and rats.Crossref | GoogleScholarGoogle Scholar | 19438767PubMed |
Li, D., Jin, M., Bao, P., Zhao, W., and Zhang, S. (2020a). Clinical Characteristics and Results of Semen Tests Among Men With Coronavirus Disease 2019. JAMA Netw Open 3, e208292.
| Clinical Characteristics and Results of Semen Tests Among Men With Coronavirus Disease 2019.Crossref | GoogleScholarGoogle Scholar | 32990740PubMed |
Li, N., Han, L., Peng, M., Lv, Y., Ouyang, Y., Liu, K., Yue, L., Li, Q., Sun, G., Chen, L., and Yang, L. (2020b). Maternal and neonatal outcomes of pregnant women with COVID-19 pneumonia: a case-control study. Clinical infectious diseases: an official publication of the Infectious Diseases Society of America , 2035–2041.
| Maternal and neonatal outcomes of pregnant women with COVID-19 pneumonia: a case-control study.Crossref | GoogleScholarGoogle Scholar |
Li, M., Chen, L., Zhang, J., Xiong, C., and Li, X. (2020c). The SARS-CoV-2 receptor ACE2 expression of maternal-fetal interface and fetal organs by single-cell transcriptome study. PloS One 15, e0230295.
| The SARS-CoV-2 receptor ACE2 expression of maternal-fetal interface and fetal organs by single-cell transcriptome study.Crossref | GoogleScholarGoogle Scholar | 33370368PubMed |
Liu, W., Wang, J., Li, W., Zhou, Z., Liu, S., and Rong, Z. (2020). Clinical characteristics of 19 neonates born to mothers with COVID-19. Front. Med. 14, 193–198.
| Clinical characteristics of 19 neonates born to mothers with COVID-19.Crossref | GoogleScholarGoogle Scholar | 32285380PubMed |
LoMauro, A., and Aliverti, A. (2015). Respiratory physiology of pregnancy. Breathe 11, 297–301.
| Respiratory physiology of pregnancy.Crossref | GoogleScholarGoogle Scholar | 27066123PubMed |
Ma, L., Xie, W., Li, D., Shi, L., Mao, Y., Xiong, Y., Zhang, Y., and Zhang, M. 2020. Effect of SARS-CoV-2 infection upon male gonadal function: A single center-based study. MedRxiv
Mead, P. S., Hills, S. L., and Brooks, J. T. (2018). Zika virus as a sexually transmitted pathogen. Curr. Opin. Infect. Dis. 31, 39–44.
| Zika virus as a sexually transmitted pathogen.Crossref | GoogleScholarGoogle Scholar | 29176348PubMed |
Muallem, G., Wagage, S., Sun, Y., DeLong, J. H., Valenzuela, A., Christian, D. A., Harms Pritchard, G., Fang, Q., Buza, E. L., Jain, D., Elloso, M. M., López, C. B., and Hunter, C. A. (2017). IL-27 Limits Type 2 Immunopathology Following Parainfluenza Virus Infection. PLoS Pathog. 13, e1006173.
| IL-27 Limits Type 2 Immunopathology Following Parainfluenza Virus Infection.Crossref | GoogleScholarGoogle Scholar | 28129374PubMed |
Nelson, D. M., Main, E., Crafford, W., and Ahumada, G. G. (1983). Peripartum heart failure due to primary pulmonary hypertension. Obstet. Gynecol. 62, 58s–63s.
| 6877714PubMed |
Neves, L. A., Stovall, K., Joyner, J., Valdés, G., Gallagher, P. E., Ferrario, C. M., Merrill, D. C., and Brosnihan, K. B. (2008). ACE2 and ANG-(1–7) in the rat uterus during early and late gestation. Am. J. Physiol. Regul. Integr. Comp. Physiol. 294, R151–R161.
| ACE2 and ANG-(1–7) in the rat uterus during early and late gestation.Crossref | GoogleScholarGoogle Scholar | 17977916PubMed |
Paoli, D., Pallotti, F., Colangelo, S., Basilico, F., Mazzuti, L., Turriziani, O., Antonelli, G., Lenzi, A., and Lombardo, F. (2020). Study of SARS-CoV-2 in semen and urine samples of a volunteer with positive naso-pharyngeal swab. J. Endocrinol. Invest. 43, 1819–1822.
| Study of SARS-CoV-2 in semen and urine samples of a volunteer with positive naso-pharyngeal swab.Crossref | GoogleScholarGoogle Scholar | 32329026PubMed |
Patel, S. K., Velkoska, E., and Burrell, L. M. (2013). Emerging markers in cardiovascular disease: where does angiotensin-converting enzyme 2 fit in? Clin. Exp. Pharmacol. Physiol. 40, 551–559.
| Emerging markers in cardiovascular disease: where does angiotensin-converting enzyme 2 fit in?Crossref | GoogleScholarGoogle Scholar | 23432153PubMed |
Peredo, H. A., and Celuch, S. M. (2001). Bradykinin and electrical stimulation increase prostaglandin production in the rat vas deferens. Prostaglandins Leukot. Essent. Fatty Acids 65, 9–14.
| Bradykinin and electrical stimulation increase prostaglandin production in the rat vas deferens.Crossref | GoogleScholarGoogle Scholar | 11487302PubMed |
Pereira, V. M., Reis, F. M., Santos, R. A., Cassali, G. D., Santos, S. H., Honorato-Sampaio, K., and dos Reis, A. M. (2009). Gonadotropin stimulation increases the expression of angiotensin-(1–7) and MAS receptor in the rat ovary. Reprod. Sci. 16, 1165–1174.
| Gonadotropin stimulation increases the expression of angiotensin-(1–7) and MAS receptor in the rat ovary.Crossref | GoogleScholarGoogle Scholar | 19703990PubMed |
Pierucci-Alves, F., and Schultz, B. D. (2008). Bradykinin-stimulated cyclooxygenase activity stimulates vas deferens epithelial anion secretion in vitro in swine and humans. Biol. Reprod. 79, 501–509.
| Bradykinin-stimulated cyclooxygenase activity stimulates vas deferens epithelial anion secretion in vitro in swine and humans.Crossref | GoogleScholarGoogle Scholar | 18480467PubMed |
Pozzilli, P., and Lenzi, A. (2020). Commentary: Testosterone, a key hormone in the context of COVID-19 pandemic. Metabolism 108, 154252.
| Commentary: Testosterone, a key hormone in the context of COVID-19 pandemic.Crossref | GoogleScholarGoogle Scholar | 32353355PubMed |
Pringle, K. G., Tadros, M. A., Callister, R. J., and Lumbers, E. R. (2011). The expression and localization of the human placental prorenin/renin-angiotensin system throughout pregnancy: roles in trophoblast invasion and angiogenesis? Placenta 32, 956–962.
| The expression and localization of the human placental prorenin/renin-angiotensin system throughout pregnancy: roles in trophoblast invasion and angiogenesis?Crossref | GoogleScholarGoogle Scholar | 22018415PubMed |
Reis, A. B., Araújo, F. C., Pereira, V. M., Dos Reis, A. M., Santos, R. A., and Reis, F. M. (2010). Angiotensin (1–7) and its receptor Mas are expressed in the human testis: implications for male infertility. J. Mol. Histol. 41, 75–80.
| Angiotensin (1–7) and its receptor Mas are expressed in the human testis: implications for male infertility.Crossref | GoogleScholarGoogle Scholar | 20361351PubMed |
Reis, F. M., Bouissou, D. R., Pereira, V. M., Camargos, A. F., dos Reis, A. M., and Santos, R. A. (2011). Angiotensin-, its receptor Mas, and the angiotensin-converting enzyme type 2 are expressed in the human ovary. Fertil. Steril. 95, 176–181.
| Angiotensin-, its receptor Mas, and the angiotensin-converting enzyme type 2 are expressed in the human ovary.Crossref | GoogleScholarGoogle Scholar | 20674894PubMed |
Rice, G. I., Thomas, D. A., Grant, P. J., Turner, A. J., and Hooper, N. M. (2004). Evaluation of angiotensin-converting enzyme (ACE), its homologue ACE2 and neprilysin in angiotensin peptide metabolism. Biochem. J. 383, 45–51.
| Evaluation of angiotensin-converting enzyme (ACE), its homologue ACE2 and neprilysin in angiotensin peptide metabolism.Crossref | GoogleScholarGoogle Scholar | 15283675PubMed |
Robinson, D. P., Huber, S. A., Moussawi, M., Roberts, B., Teuscher, C., Watkins, R., Arnold, A. P., and Klein, S. L. (2011). Sex chromosome complement contributes to sex differences in coxsackievirus B3 but not influenza A virus pathogenesis. Biol. Sex Differ. 2, 8.
| Sex chromosome complement contributes to sex differences in coxsackievirus B3 but not influenza A virus pathogenesis.Crossref | GoogleScholarGoogle Scholar | 21806829PubMed |
Robinson, D. P., Hall, O. J., Nilles, T. L., Bream, J. H., and Klein, S. L. (2014). 17β-estradiol protects females against influenza by recruiting neutrophils and increasing virus-specific CD8 T cell responses in the lungs. J. Virol. 88, 4711–4720.
| 17β-estradiol protects females against influenza by recruiting neutrophils and increasing virus-specific CD8 T cell responses in the lungs.Crossref | GoogleScholarGoogle Scholar | 24522912PubMed |
Robinson, J. L., Kocabaş, P., Wang, H., Cholley, P. E., Cook, D., Nilsson, A., Anton, M., Ferreira, R., Domenzain, I., Billa, V., Limeta, A., Hedin, A., Gustafsson, J., Kerkhoven, E. J., Svensson, L. T., Palsson, B. O., Mardinoglu, A., Hansson, L., Uhlén, M., and Nielsen, J. (2020). An atlas of human metabolism. Science signaling 13, eaaz1482.
| An atlas of human metabolism.Crossref | GoogleScholarGoogle Scholar | 32209698PubMed |
Schurz, H., Salie, M., Tromp, G., Hoal, E. G., Kinnear, C. J., and Möller, M. (2019). The X chromosome and sex-specific effects in infectious disease susceptibility. Hum. Genomics 13, 2.
| The X chromosome and sex-specific effects in infectious disease susceptibility.Crossref | GoogleScholarGoogle Scholar | 30621780PubMed |
Shek, C. C., Ng, P. C., Fung, G. P., Cheng, F. W., Chan, P. K., Peiris, M. J., Lee, K. H., Wong, S. F., Cheung, H. M., Li, A. M., Hon, E. K., Yeung, C. K., Chow, C. B., Tam, J. S., Chiu, M. C., and Fok, T. F. (2003). Infants born to mothers with severe acute respiratory syndrome. Pediatrics 112, e254.
| Infants born to mothers with severe acute respiratory syndrome.Crossref | GoogleScholarGoogle Scholar | 14523207PubMed |
Shen, Q., Xiao, X., Aierken, A., Yue, W., Wu, X., Liao, M., and Hua, J. (2020). The ACE2 expression in Sertoli cells and germ cells may cause male reproductive disorder after SARS-CoV-2 infection. J. Cell. Mol. Med. 24, 9472–9477.
| The ACE2 expression in Sertoli cells and germ cells may cause male reproductive disorder after SARS-CoV-2 infection.Crossref | GoogleScholarGoogle Scholar | 32594644PubMed |
Shi, L., Wang, Y., Wang, Y., Duan, G., and Yang, H. (2020). Dyspnea rather than fever is a risk factor for predicting mortality in patients with COVID-19. J Infect. 81, 647–679.
| Dyspnea rather than fever is a risk factor for predicting mortality in patients with COVID-19.Crossref | GoogleScholarGoogle Scholar | 32417316PubMed |
Shibahara, H., Kamata, M., Hu, J., Nakagawa, H., Obara, H., Kondoh, N., Shima, H., and Sato, I. (2001). Activity of testis angiotensin converting enzyme (ACE) in ejaculated human spermatozoa. Int. J. Androl. 24, 295–299.
| Activity of testis angiotensin converting enzyme (ACE) in ejaculated human spermatozoa.Crossref | GoogleScholarGoogle Scholar | 11554987PubMed |
Sigurðardóttir, O. G., Kolbjørnsen, O., and Lutz, H. (2001). Orchitis in a cat associated with coronavirus infection. J. Comp. Pathol. 124, 219–222.
| Orchitis in a cat associated with coronavirus infection.Crossref | GoogleScholarGoogle Scholar |
Somlev, B., and Subev, M. (1998). Effect of kininase II inhibitors on bradykinin-stimulated bovine sperm motility. Theriogenology 50, 651–657.
| Effect of kininase II inhibitors on bradykinin-stimulated bovine sperm motility.Crossref | GoogleScholarGoogle Scholar | 10732155PubMed |
Stelzig, K. E., Canepa-Escaro, F., Schiliro, M., Berdnikovs, S., Prakash, Y. S., and Chiarella, S. E. (2020). Estrogen regulates the expression of SARS-CoV-2 receptor ACE2 in differentiated airway epithelial cells. Am. J. Physiol. Lung Cell. Mol. Physiol. 318, L1280–L1281.
| Estrogen regulates the expression of SARS-CoV-2 receptor ACE2 in differentiated airway epithelial cells.Crossref | GoogleScholarGoogle Scholar | 32432918PubMed |
Sullivan, J. C., Bhatia, K., Yamamoto, T., and Elmarakby, A. A. (2010). Angiotensin (1–7) receptor antagonism equalizes angiotensin II-induced hypertension in male and female spontaneously hypertensive rats. Hypertension (Dallas, Tex.: 1979) 56, 658–666.
| Angiotensin (1–7) receptor antagonism equalizes angiotensin II-induced hypertension in male and female spontaneously hypertensive rats.Crossref | GoogleScholarGoogle Scholar |
Sullivan, J. C., Rodriguez-Miguelez, P., Zimmerman, M. A., and Harris, R. A. (2015). Differences in angiotensin (1–7) between men and women. Am. J Physiol. Heart Circ. Physiol. 308, H1171–H1176.
| Differences in angiotensin (1–7) between men and women.Crossref | GoogleScholarGoogle Scholar | 25659489PubMed |
Thimon, V., Belghazi, M., Dacheux, J. L., and Gatti, J. L. (2006). Analysis of furin ectodomain shedding in epididymal fluid of mammals: demonstration that shedding of furin occurs in vivo. Reproduction 132, 899–908.
| Analysis of furin ectodomain shedding in epididymal fluid of mammals: demonstration that shedding of furin occurs in vivo.Crossref | GoogleScholarGoogle Scholar | 17127750PubMed |
Toppozada, H., Michaels, L., Toppozada, M., El-Ghazzawi, I., Talaat, M., and Elwany, S. (1982). The human respiratory nasal mucosa in pregnancy. An electron microscopic and histochemical study. J. Laryngol. Otol. 96, 613–626.
| The human respiratory nasal mucosa in pregnancy. An electron microscopic and histochemical study.Crossref | GoogleScholarGoogle Scholar | 7086277PubMed |
UNICEF (2020). Breastfeeding during the COVID-19 pandemic. https://www.unicef.org/eap/breastfeeding-during-covid-19
Valdés, G., Neves, L. A., Anton, L., Corthorn, J., Chacón, C., Germain, A. M., Merrill, D. C., Ferrario, C. M., Sarao, R., Penninger, J., and Brosnihan, K. B. (2006). Distribution of angiotensin-(1–7) and ACE2 in human placentas of normal and pathological pregnancies. Placenta 27, 200–207.
| Distribution of angiotensin-(1–7) and ACE2 in human placentas of normal and pathological pregnancies.Crossref | GoogleScholarGoogle Scholar | 16338465PubMed |
Vaz-Silva, J., Carneiro, M. M., Ferreira, M. C., Pinheiro, S. V., Silva, D. A., Silva-Filho, A. L., Witz, C. A., Reis, A. M., Santos, R. A., and Reis, F. M. (2009). The vasoactive peptide angiotensin-, its receptor Mas and the angiotensin-converting enzyme type 2 are expressed in the human endometrium. Reprod. Sci. 16, 247–256.
| The vasoactive peptide angiotensin-, its receptor Mas and the angiotensin-converting enzyme type 2 are expressed in the human endometrium.Crossref | GoogleScholarGoogle Scholar | 19164480PubMed |
Vaz-Silva, J., Tavares, R. L., Ferreira, M. C., Honorato-Sampaio, K., Cavallo, I. K., Santos, R. A., dos Reis, A. M., and Reis, F. M. (2012). Tissue specific localization of angiotensin-(1–7) and its receptor Mas in the uterus of ovariectomized rats. J. Mol. Histol. 43, 597–602.
| Tissue specific localization of angiotensin-(1–7) and its receptor Mas in the uterus of ovariectomized rats.Crossref | GoogleScholarGoogle Scholar | 22684246PubMed |
Vickers, C., Hales, P., Kaushik, V., Dick, L., Gavin, J., Tang, J., Godbout, K., Parsons, T., Baronas, E., Hsieh, F., Acton, S., Patane, M., Nichols, A., and Tummino, P. (2002). Hydrolysis of biological peptides by human angiotensin-converting enzyme-related carboxypeptidase. J. Biol. Chem. 277, 14838–14843.
| Hydrolysis of biological peptides by human angiotensin-converting enzyme-related carboxypeptidase.Crossref | GoogleScholarGoogle Scholar | 11815627PubMed |
Vivanti, A. J., Vauloup-Fellous, C., Prevot, S., Zupan, V., Suffee, C., Do Cao, J., Benachi, A., and De Luca, D. (2020). Transplacental transmission of SARS-CoV-2 infection. Nat. Commun. 11, 3572.
| Transplacental transmission of SARS-CoV-2 infection.Crossref | GoogleScholarGoogle Scholar | 32665677PubMed |
Wang, Z., and Xu, X. (2020). scRNA-seq Profiling of Human Testes Reveals the Presence of the ACE2 Receptor, A Target for SARS-CoV-2 Infection in Spermatogonia, Leydig and Sertoli Cells. Cells 9, 920.
| scRNA-seq Profiling of Human Testes Reveals the Presence of the ACE2 Receptor, A Target for SARS-CoV-2 Infection in Spermatogonia, Leydig and Sertoli Cells.Crossref | GoogleScholarGoogle Scholar |
Wu, Z., and McGoogan, J. M. (2020). Characteristics of and Important Lessons From the Coronavirus Disease 2019 (COVID-19) Outbreak in China: Summary of a Report of 72 314 Cases From the Chinese Center for Disease Control and Prevention. JAMA 323, 1239–1242.
| Characteristics of and Important Lessons From the Coronavirus Disease 2019 (COVID-19) Outbreak in China: Summary of a Report of 72 314 Cases From the Chinese Center for Disease Control and Prevention.Crossref | GoogleScholarGoogle Scholar | 32091533PubMed |
Wu, Y. T., Liu, C., Dong, L., Zhang, C. J., Chen, Y., and Liu, J. (2020) Viral Shedding of COVID-19 in Pregnant Women [cited 25 May 2020]. Available at SSRN: https://ssrn.com/abstract=3562059 or
Xu, J., Qi, L., Chi, X., Yang, J., Wei, X., Gong, E., Peh, S., and Gu, J. (2006). Orchitis: a complication of severe acute respiratory syndrome (SARS). Biol. Reprod. 74, 410–416.
| Orchitis: a complication of severe acute respiratory syndrome (SARS).Crossref | GoogleScholarGoogle Scholar | 16237152PubMed |
Yee, J., Kim, W., Han, J. M., Yoon, H. Y., Lee, N., Lee, K. E., and Gwak, H. S. (2020). Clinical manifestations and perinatal outcomes of pregnant women with COVID-19: a systematic review and meta-analysis. Sci. Rep. 10, 18126.
| Clinical manifestations and perinatal outcomes of pregnant women with COVID-19: a systematic review and meta-analysis.Crossref | GoogleScholarGoogle Scholar | 33093582PubMed |
Yoshimura, Y. (1997). The ovarian renin-angiotensin system in reproductive physiology. Front. Neuroendocrinol. 18, 247–291.
| The ovarian renin-angiotensin system in reproductive physiology.Crossref | GoogleScholarGoogle Scholar | 9237079PubMed |
Zeng, H., Xu, C., Fan, J., Tang, Y., Deng, Q., Zhang, W., and Long, X. (2020). Antibodies in Infants Born to Mothers With COVID-19 Pneumonia. JAMA 323, 1848–1849.
| Antibodies in Infants Born to Mothers With COVID-19 Pneumonia.Crossref | GoogleScholarGoogle Scholar | 32215589PubMed |
Zhao, X., Jiang, Y., Zhao, Y., Xi, H., Liu, C., Qu, F., and Feng, X. (2020). Analysis of the susceptibility to COVID-19 in pregnancy and recommendations on potential drug screening. Eur J Clin Microbiol Infect Dis 39, 1209–1220.
| Analysis of the susceptibility to COVID-19 in pregnancy and recommendations on potential drug screening.Crossref | GoogleScholarGoogle Scholar | 32328850PubMed |
Zhou, M., Dai, W., Cui, Y., and Li, Y. (2020). Estrogen downregulates gp130 expression in HUVECs by regulating ADAM10 and ADAM17 via the estrogen receptor. Biochem. Biophys. Res. Commun. 523, 753–758.
| Estrogen downregulates gp130 expression in HUVECs by regulating ADAM10 and ADAM17 via the estrogen receptor.Crossref | GoogleScholarGoogle Scholar | 31952790PubMed |
Zhu, H., Wang, L., Fang, C., Peng, S., Zhang, L., Chang, G., Xia, S., and Zhou, W. (2020). Clinical analysis of 10 neonates born to mothers with 2019-nCoV pneumonia. Transl. Pediatr. 9, 51–60.
| Clinical analysis of 10 neonates born to mothers with 2019-nCoV pneumonia.Crossref | GoogleScholarGoogle Scholar | 32154135PubMed |