Maternal undernutrition during pregnancy and lactation increases transcription factors, ETV5 and GDNF, and alters regulation of apoptosis and heat shock proteins in the testis of adult offspring in the rat
Graciela Pedrana
A Facultad de Veterinaria, Universidad de la República, Montevideo, 11600, Uruguay.
B Liggins Institute, University of Auckland, Auckland, 1142, New Zealand.
C UWA School of Agriculture and Environment and UWA Institute of Agriculture, University of Western Australia, Perth, WA 6009, Australia.
D Department of Biochemistry and Biomedical Sciences, McMaster University, Hamilton, L8S 4L8, Canada.
E Department of Pediatrics, McMaster University, Hamilton, L8S 4L8, Canada, and Farncombe Family Digestive Health Research Institute, McMaster University, Hamilton, L8S 4L8, Canada.
F Corresponding author. Email: gpedrana@gmail.com
Reproduction, Fertility and Development 33(7) 484-496 https://doi.org/10.1071/RD20260
Submitted: 30 September 2020 Accepted: 24 March 2021 Published: 22 April 2021
Journal Compilation © CSIRO 2021 Open Access CC BY-NC-ND
Abstract
We tested whether changes in Sertoli cell transcription factors and germ cell heat shock proteins (HSPs) are linked to the effects of maternal undernutrition on male offspring fertility. Rats were fed ad libitum with a standard diet (CONTROL) throughout pregnancy and lactation or with 50% of CONTROL intake throughout pregnancy (UNP) or lactation (UNL) or both periods (UNPL). After postnatal Day 21, 10 male pups per group were fed a standard diet ad libitum until postnatal Day 160 when testes were processed for histological, mRNA and immunohistochemical analyses. Compared with CONTROL: caspase-3 was increased in UNP and UNPL (P = 0.001); Bax was increased in UNL (P = 0.002); Bcl-2 (P < 0.0001) was increased in all underfed groups; glial cell line-derived neurotrophic factor (P = 0.002) was increased in UNP and UNL; E twenty-six transformation variant gene 5 and HSP70 were increased, and HSP90 was diminished in all underfed groups (P < 0.0001). It appears that maternal undernutrition during pregnancy and lactation disrupts the balance between proliferation and apoptosis in germ cells, increasing germ cell production and perhaps exceeding the support capacity of the Sertoli cells. Moreover, fertility could be further compromised by changes in meiosis and spermiogenesis mediated by germ cell HSP90 and HSP70.
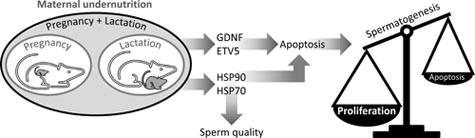
Keywords: nutrition, fetal programming, transcription factor, testis, Sertoli cell, apoptosis.
Introduction
The term ‘fetal and developmental programming’ refers to the effects of a stressor during a specific period of pre- or postnatal development that cause morphological and functional changes in cells and tissues, with consequences that can manifest later in life, including a range of cardiometabolic and reproductive disorders (Langley-Evans 2015). For example, maternal undernutrition during pre- and postnatal life can lead to metabolic syndrome and cardiovascular disease in adult offspring (Wadhwa et al. 2009; Bell and Greenwood 2016; Kereliuk et al. 2017; Jazwiec and Sloboda 2019). Conversely, under other conditions, normal development can be disturbed by changes in the pathways that control cell proliferation, apoptosis and differentiation (Langley-Evans and McMullen 2010), but the cells and tissues then adapt through a process known as developmental plasticity (Bateson et al. 2004; Langley-Evans 2015).
In humans, pioneering studies of health history by DJP Barker gave rise to the concept of suboptimal developmental conditions in utero predisposing the offspring to chronic diseases in later life, including cancer. ‘The Barker Hypothesis’ is now commonly referred to as the ‘Developmental origins of health and disease’ or DOHaD (review: Sinclair et al. 2016). In animals, several studies have proposed associations between nutrition during prenatal and early postnatal life, birthweight and postnatal reproductive development, as explanations of the variation in reproductive performance observed in adult animals under field conditions (reviews: Martin et al. 2010; Sinclair et al. 2016). In male rats, for example, nutrient restriction during late gestation is associated with delayed puberty, reductions in circulating concentrations of leptin and FSH, and a decrease in the diameter of seminiferous tubules (Léonhardt et al. 2003). Similarly, protein restriction in pregnant rats has several deleterious effects on male offspring, including reductions in Sertoli cell number, sperm count, sperm motility and daily sperm production, as well as altered oxidative stress in the testis (Toledo et al. 2011; Khorram et al. 2015). We recently reported on the effects of maternal undernutrition in the rat, during pregnancy and/or lactation, on the testis IGF-I (insulin-like growth factor I) system and germ cell proliferation in male offspring (Pedrana et al. 2020). In the testis of adult offspring from mothers that were undernourished during pregnancy and lactation, there were increases in IGF-I, Sertoli cell numbers and spermatogonial cell proliferation. These changes were associated with a reduction in the number of seminiferous tubules at Stage VII of the spermatogenic cycle, before spermiogenesis, suggesting effects on spermatogenesis. The present study used this cohort to extend on our previous observations to investigate the cellular mechanisms involved.
In many reports, maternal metabolic challenge appears to affect the development of Sertoli cell function in the offspring, suggesting that other factors produced by Sertoli cells could be involved in the programming of the testis, with subsequent effects on adult fertility. Sertoli cells are somatic cells with well-controlled transcriptional systems for the many roles they play throughout the spermatogenic cycle (Figueiredo et al. 2016; França et al. 2016). For example, studies in rodent models (Cooke et al. 2006) have shown that Sertoli cells produce two transcription factors that are members of the ETS family: ETV5 (E twenty-six transformation variant gene 5; also called ETS-related molecule, ERM) and GDNF (glial cell line-derived neurotrophic factor). Both factors are involved in paracrine regulation of the renewal of spermatogonial stem cells (SSCs), a multifaceted process that includes cell proliferation, prevention of apoptosis and maintenance of stem cell phenotype (Meng et al. 2000; Ishii et al. 2012; Singh et al. 2017). Disruption of the Etv5 gene allows the first wave of spermatogenesis, but all SSCs are lost during that wave, leading to a Sertoli cell-only phenotype (Schlesser et al. 2008; Tyagi et al. 2009).
The ETS family of proteins regulates cellular invasion and migration, cell survival and entry into the S phase of the cell cycle in the testis (Simon et al. 2007; Hofmann 2008). In thyroid tissue tumours, ETV5 has been associated with metastatic progression (Puli et al. 2018). In the testis, self-renewal and differentiation of SSCs initiates meiosis, a highly organised process targeted by factors within the stem cell niche produced by Sertoli cells. If the ability of SSCs to undergo self-renewal is modified, the SSC population could be rapidly depleted, resulting in male infertility.
In normal tissue development as well as pathological situations, cell proliferation is a balance between two processes, cell division and apoptosis, and the heat shock proteins (HSPs) are involved in maintaining that balance (Sarge and Cullen 1997; Selim et al. 2012; Domingos et al. 2013). This same balance applies to the testis (Kennedy et al. 2014), where HSP90 is expressed (Grad et al. 2010; Au et al. 2015) and seems to be indispensable for disassembly and/or progression through the late pachytene or the pachytene checkpoint, when meiotic spermatocytes are undergoing apoptosis. Thus, in the absence of functional HSP90, germ cells fail to complete meiosis, leading to spermatogenic arrest and subsequent infertility (Grad et al. 2010). In mammalian cells, there are two HSP90 homologues: HSP90α is known to be critical for the first wave of spermatogenesis before puberty, but the role of HSP90β is not yet clear, although it is expressed in Sertoli and germ cells (Jha et al. 2013).
Members of the HSP family are involved in cell thermotolerance, a critical aspect of testis function, and in sensitivity to a broad range of cytotoxic stimuli (Selim et al. 2012). For example, HSP70 is considered to be cytoprotective, particularly under stress conditions, as it modifies the antioxidant potential of cells. It translocates to the nucleus and nucleolus during the S phase or during a heat shock and participates in pathways regulating the integrity and accuracy of DNA replication, and in the inhibition of breaks in nucleoplasmic/nucleolic single-stranded sDNA, one of the final stages of apoptosis (Kotoglou et al. 2009).
These considerations led us to test whether ETV5 and GDNF in Sertoli cells, through their roles as regulators of HSPs and apoptosis in germ cells, are involved in the programming of testicular function in the context of maternal undernutrition during pregnancy and/or lactation.
Materials and methods
Animal model
All animal work was approved under the guidelines of the Animal Ethics Committee of the University of Auckland (R402). In the present study, we used an established model of developmental programming based on manipulation of maternal nutrition (Howie et al. 2012). Female Wistar rats (120 days old; n = 24) were sourced from the Vernon Jansen Unit at the University of Auckland, where the animal model had been developed. They were time-mated using a rat oestrous cycle monitor (Fine Science Tools, USA) and thereafter housed individually in standard rat cages in the same room, with free access to water, a constant temperature of 25°C, and a 12:12 light:dark cycle. Pregnant dams were randomly allocated to one of four dietary groups (6 per group): (1) CONTROL in which dams were fed ad libitum throughout pregnancy and lactation with a standard diet (protein 18%, fat 5%; digestible energy 3.4 kcal/g; Teklad Global 18% Protein Diet, Diet 2018); (2) UNPL in which dams were fed 50% of CONTROL intake throughout both pregnancy and lactation; (3) UNP in which dams were fed 50% of CONTROL intake throughout pregnancy, then fed ad libitum throughout lactation; and (4) UNL in which dams were fed ad libitum throughout pregnancy but then fed 50% of CONTROL intake throughout lactation.
Body mass and age at puberty
After birth, pups were weighed and litter size was adjusted to four males and four females per litter to ensure standardised nutrition until weaning on Day 22 postpartum. After weaning, male offspring were housed in pairs and fed the standard chow diet ad libitum until the end of the study (160 days).
Testis sampling and processing
On postnatal Day 160, male offspring (n = 40; 10 per dietary group) were fasted overnight, weighed, anaesthetised with pentobarbitone (60 mg kg−1 i.p.) and then killed by decapitation. Blood was collected into heparinised vacutainers tubes and centrifuged (2400g, 20 min) and plasma was separated and stored at −20°C until analysis. The values for body mass and total testis mass were used to calculate relative testis mass and the gonadosomatic index (ratio of testis mass to body mass). The left testes were frozen in liquid nitrogen and stored at –80°C in preparation for molecular analyses. Right testes were immersed in fixative (4% formaldehyde phosphate buffer, pH 7.4) for 24 h.
Histology and immunohistochemistry
Fixed testes were progressively dehydrated by immersion in increasing concentrations of ethanol (70%, 95%, 100%), embedded in paraffin resin (Historesin; Leica, Germany) and sectioned at 5 µm by microtome (Leica 149 Reichert Jung Biocut 2030) in preparation for staining and immunohistochemistry. Slides were processed for the detection of immunoexpression for active caspase-3, Bax, Bcl-2, ETV5, GDNF, HSP90 and HSP70 using the streptavidin–biotin–peroxidase protocol, described previously (Pedrana et al. 2020). In brief, tissue sections were deparaffinised, rehydrated by heat-induced epitope retrieval for 5 min in a microwave oven, by immersion in 0.01 M citrate buffer (pH 6.0) and Tween (5 mL); slides were washed with distilled water and phosphate-buffered saline (PBS, pH 7.4); endogenous peroxidases were inactivated with 3% hydrogen peroxide 200 (20 min), followed by washing in PBS and incubation with normal rat serum to block non-specific binding proteins; sections were then incubated for 30 min with each primary antibody on different slides (Table 1), for 30 min with biotinylated secondary antibody (mouse specific HRP/DAB, ab 64259, Abcam, USA; or rabbit specific HRP/DAB, ab 64261; Abcam, USA; depending on the origin of the primary antibody); slides were then incubated with streptavidin–peroxidase enzyme complex for 30 min; incubation with diaminobenzidine (DAB) chromogen solution (1.5 mL hydrogen peroxide + 30 μL DAB) for 5 min to visualise the antigen; sections were counterstained with Mayer’s hematoxylin, dehydrated, and mounted with Entellan® (Merck) on glass coverslips. The specificity of the antibodies was verified by substitution of the primary antibody with normal serum, diluted 1:100 or 1:200 in PBS, according to the dilution of the primary antibody. All assays contained a negative control. Positive controls were ovine testis and mammary gland tumours.
Image analysis
Digital images were obtained using a light microscope (Olympus CX23) equipped with a digital camera (Dino-Eyepiece, AM-7023X; AnMo Electronics Corp., Taiwan) and then analysed with DinoCapture 2.0 software (AnMo Electronics Corp.). Digital images of all seminiferous tubules within a cross-section of the testis were captured and analysed at ×400 and ×600 magnification. For each protein (Table 1), the immunostained area (IA) was quantified by digital image analysis using ImageJ software (Version 1.52b 6 May 2018; Wayne Rasband, NIH, USA; http://rsb.info.nih.gov/). All proteins analysed were measured in seminiferous tubules sections, considering only the IA occupied by seminiferous tubule were described and quantified by ImageJ. Areas of immunopositivity were measured using a locally written macro that included colour threshold values from RGB images and conversion to binary images and IA measurement. This process provided quantitative values for the percentage of IA. A batch mode was performed to apply the macro to all images.
Immunoexpression of GDNF was analysed in 200 digital images for each testis. The tubules were classified according to stage of cycle (Stages I–XIV as described previously for the rat) (Johnston et al. 2011). All stages were assessed but, for the present study, there was a focus on the period before spermiation (up to Stage VII). To reduce systematic random variation, a consensus decision was made by two observers simultaneously examining each section to define the stage of spermatogenic cycle, as suggested in previous studies (Hess et al. 1990).
Gene expression analysis by qPCR
Total RNA was extracted using commercially available kits (AllPrep DNA/RNA mini kit; cat 80204; Qiagen). Genomic DNA contamination was removed from each sample by treatment with RNase-free DNase (Invitrogen Life Technologies, NZ) according to the manufacturer’s instructions. RNA quantity and purity were analysed using a NanoDrop spectrophotometer (ND-1000; BioLab Ltd) and NanoDrop software (version 3.1.2). All RNA samples were stored at −80°C until required. For first-strand cDNA synthesis we used 5 µg of total RNA with Moloney Murine Leukaemia Virus Reverse Transcriptase (M-MLV-RT; Promega Corp., WI, USA) and a standard thermocycler (GeneAmp® PCR System 9700; Applied Biosystems). A master mix was prepared containing 5 μL M-MLV 5 × buffer (cat M531A; In Vitro Technologies), 0.5 μL M-MLV-RT (cat. M170B; In Vitro Technologies) and 1.25 μL 10 μM deoxynucleoside-triphosphates (cat. R0181; Global Science). We used the following cycling conditions: an initial denaturation stage of 5 min at 96°C followed by 30 cycles (30 s each) of 96°C (denaturation), 60°C (annealing stage) and 72°C (extension stage). The cDNA was stored at –20°C until assay by quantitative polymerase chain reaction (qPCR).
Testicular gene expression for caspase-3, Bax, Bcl-2 and the endogenous reference (β-actin) was measured by qPCR using the ABI PRISM® 7900HT Sequence Detection System (Applied Biosystems, NZ). All primers were designed using Primer 3 software (version 0.4.0, Whitehead Institute for Biomedical Research) manufactured by Invitrogen (Invitrogen Life Technologies). Primer set controls were β-actin forward primer CACCAACTGGGACGATATGGA and β-actin reverse primer CAGCCTGGATGGCTACGTACAT, 188 bp (Table 2). Optimal primer conditions were adjusted to the cycling conditions: length 20 bp (range 17–23 bp); Tm 63°C (range 60–65°C); amplicon length 100–300 bp. Dissociation analyses were performed to ensure specificity and only samples producing a single peak in the dissociation curves were used. Amplified products were visualised on an agarose gel using the E-Gel® CloneWell 0.8% SYBR Safe gel (cat. G6618-08; Invitrogen), run on the E-Gel® iBase™ Power System (cat. G6400; Invitrogen) and sequenced by spectrophotometry (Allan Wilson Centre, Massey University, NZ). The resulting sequences were evaluated using NCBI BLAST to ensure specificity.
Transcript levels were quantified by qPCR under the following conditions: an initial 2-min hold period at 50°C for normalisation (Stage 1), followed by enzyme activation at 95°C for 2 min (Stage 2); amplification of the gene product through 40 successive cycles of 95°C for 15 s and then 60°C for 1 min (Stage 3); followed by a dissociation stage of 15 s at 95°C, 15 s at 60°C, and 2 min at 99°C (Stage 4). A standard curve was generated from the mean cycle threshold (Ct) of eight standards (1:5 serial dilution) of a known concentration in triplicate and amplification and dissociation curves were generated for all standards and samples (Applied Biosystems). Each sample was run in triplicate.
Statistical analysis
Effects of treatment on the expression of caspase-3, Bax and Bcl-2 and on IA (%) for caspase-3, Bax, Bcl-2, GDNF, ETV5, HSP70 and HSP90 were analysed using a one-way ANOVA in a general linear model GLM (SAS for Windows, USA) and post hoc Tukey’s tests. All data are reported as mean ± s.e.m. In all cases, the level of significance was P < 0.05.
Results
Caspase-3 immunolocalisation, immunostained area and mRNA levels
Immunostaining for caspase-3 was clearly evident in the testis and the distribution and intensity of staining varied with the type of cells present and therefore with the stage of the spermatogenic cycle. In the UNPL group, seminiferous tubules at Stage VII of the spermatogenic cycle showed caspase-3 apoptotic protein in round spermatids localised near the basal lamina of the seminiferous tubules, but not in spermatocytes and spermatogonia (Fig. 1a, b). In all four treatments groups, staining was notable in the cytoplasm and nuclei of round spermatids in the ad-luminal region of the seminiferous tubules (Figs 1, 3a). In the UNPL group, caspase-3 was also detected in the middle and basal compartments of the seminiferous tubules, in the spermatogonia, spermatocytes and Sertoli cells (Figs 1, 3a). The IA for caspase-3 in the seminiferous tubules was greater in the UNPL (P = 0.001) than in the CONTROL group (Fig. 3b). In contrast, caspase-3 mRNA levels in the testis were greater in the UNP group compared with the CONTROL group (Fig. 3c).
![]() |
![]() |
Bax immunolocalisation, immunostained area and mRNA
In the CONTROL group, Sertoli cells showed Bax immunostaining in the cytoplasm and elongated spermatids’ nuclei, although it was absent in spermatogonia. In contrast, in all three undernutrition groups (UNP, UNL UNPL), intense Bax immunostaining was evident in Sertoli cell cytoplasm and elongated spermatids, and spermatogonia were stained in both the cytoplasm and nuclei (Fig. 3d). The Bax IA was greater (P = 0.002) in UNL than in any other group (Fig. 3e). On the other hand, levels of Bax mRNA did not differ among treatments (Fig. 3f).
Bcl-2 immunolocalisation, immunostained area and mRNA
In the CONTROL group, Bcl-2 was immunolocalised in the Sertoli cell cytoplasm and in the nuclei of elongated spermatids (Fig. 3g). In both the UNPL and UNL groups, intense immunostaining for Bcl-2 was evident in degenerating pachytene spermatocytes and round spermatids at Stage VII of the spermatogenic cycle (Figs 2, 3g). In the UNPL group, the lumen of the seminiferous tubules at cycle Stage VII contained detached, round spermatids and only a few spermatozoa (Fig. 2). In the round spermatids, Bcl-2 was localised in the acrosome perinuclear region whereas, in elongated spermatids, it was localised in the nuclear region (Figs 2, 3g). The IA for Bcl-2 was greater in all undernutrition groups (P < 0.0001) compared with the CONTROL group (Fig. 3h). As with Bax, Bcl-2 mRNA levels did not differ among groups (Fig. 3i).
GDNF and ETV5 immunolocalisation and immunostained areas
In the CONTROL group, moderate GDNF immunostaining was detected in the cytoplasm of Sertoli cells, but there was minimal immunoexpression in the cytoplasm or nuclei of spermatogonia. In contrast, in all three undernutrition groups (UNP, UNL, UNPL), GDNF staining was clearly evident in the cytoplasm of spermatogonia and leptotene spermatocytes and in the nuclei of elongated spermatids. The strongest GDNF immunostaining was detected in spermatids in the UNP and UNL groups (Fig. 4a) and the IA was greater in the UNP (P < 0.01) and UNL groups (P < 0.009) compared with the CONTROL group (Fig. 4b). In Stage VII of the spermatogenic cycle, the IA for GDNF was greater in the UNP (P < 0.0036), UNL (P < 0.0001) and UNPL (P < 0.0561) than in the CONTROL (Fig. 4c).
ETV5 immunostaining was detected in the Sertoli cell cytoplasm, with the most intense expression observed in the undernutrition groups (Fig. 4d). The IA for ETV5 was greater (P < 0.0001) in all undernutrition groups (UNP, UNL, UNPL) compared with the CONTROL group (Fig. 4f).
HSP70 and HSP90 immunolocalisation and immunostained area
In the CONTROL group, HSP70 immunostaining was localised to the interstitial tissue between the seminiferous tubules; inside the seminiferous tubules, only a few spermatogonia showed slight immunostaining in the cytoplasm or nucleus. In the undernutrition groups (UNP, UNL, UNPL), on the other hand, HSP70 was detected in the cytoplasm of spermatogonia, primary spermatocytes (pachytene stage) and elongated spermatids, where it was also evident in the nucleus (Fig. 5a). The IA for HSP70 was greater (P < 0.0001) in the undernutrition groups (UNP, UNL, UNPL) compared with the CONTROL group (Fig. 5a).
In all groups, HSP90 immunostaining was detected in the nuclei of spermatogonia, in the cytoplasm of pachytene spermatocytes and round spermatids, and in the nuclei of elongated spermatids. Immunostaining was intense in the nuclei of elongated spermatids in the UNP group (Fig. 5b). The IA for HSP90 was greater (P < 0.0001) in CONTROL group compared with the undernutrition groups (UNP, UNL, UNPL; Fig. 5b).
Discussion
Maternal undernutrition, during pregnancy or lactation, affects testis development in the offspring through metabolic signals in the developing tissue such as those provided by the insulin-like growth factor system (Pedrana et al. 2020). We now know that important markers of testis function in adult offspring are also affected, including transcription factors (GDNF, ETV5), pro- and anti-apoptotic proteins (caspase 3, Bax, Bcl-2) and heat shock proteins (HSP90, HSP70). These observations need to be interpreted in the context of changes in cell populations that we have reported previously in this rat cohort (Pedrana et al. 2020) and in the context of spermatogenesis being a controlled process. It is important that proliferation is balanced against apoptosis so that the number of germ cells does not exceed the maximum that the Sertoli cells can support (Russell et al. 2002). The balance of proliferation and apoptosis will be affected by changes in GDNF and ETV5 because these transcription factors control the activity of pro- and anti-apoptotic proteins. Moreover, these outcomes will be exacerbated by changes in the expression of HSP90 and HSP70 because of their important roles in meiosis and spermiogenesis. Undernutrition during pregnancy and lactation (UNPL) had greater effects than undernutrition during only pregnancy (UNP) or only lactation (UNL), as would be expected, although we observed some specific differences between UNP and UNL in the responses of Sertoli cells and germ cells, perhaps reflecting the sequence of developmental processes in the testis. In Fig. 6, we have combined the present observations with those presented previously (Pedrana et al. 2020) to summarise our current hypothesis about how maternal undernutrition affects the testis of adult offspring in the rat.
![]() |
Transcription factors GDNF, ETV5
The effects of maternal undernutrition on GNDF would be transmitted via receptors in type A spermatogonia, with consequences for production of members of the anti-apoptotic Bcl family of proteins (Jijiwa et al. 2008; Johnston et al. 2011; Rossi and Dolci 2013; Takashima et al. 2015). Importantly, we observed an increase in GDNF in Stage VII of the spermatogenic cycle, at a time when it would be decreasing during normal development (Johnston et al. 2011). An excess of GDNF promotes the proliferation of clusters of undifferentiated type A spermatogonia and these clusters can evolve into testicular tumours that resemble the human seminoma (Tadokoro et al. 2002). Similarly, ETS proteins, such as ETV5, are also thought to control production of the Bcl family in the testis (Hofmann 2008; Schmidt et al. 2009; Ishii et al. 2012) and therefore play a role in regulating cell survival and entry into the S phase of the cell cycle (Puli et al. 2018). ETV5 appears to be one of the few transcription factors expressed by Sertoli cells that controls SSC self-renewal through either CXCL12/CXCR4 or CCL9/CCR1 signalling (Chen and Liu 2015). Therefore, increased production of GNDF and ETV5 by Sertoli cells is consistent with the increase in germ cell number that we previously observed in the same model of maternal undernutrition (Pedrana et al. 2020). With an overproduction of germ cells, the capacity of the Sertoli cells to support germ cell development would be exceeded, potentially leading to defects in spermatogenesis.
Pro-apoptotic (caspase-3, Bax) and anti-apoptotic (Bcl-2) proteins
We assessed the concentration as well as the localisation of these proteins using immunohistochemistry only because, to understand the process of apoptosis, we needed information about concentration as well as localisation. Moreover, the activities of Bcl-2 and Bax are modulated by various post-translational modifications, including folding and conformation, stability, half-life, subcellular localisation and protein–protein interactions (Letai and Kutuk 2008). If caspase-3 is localised within the nucleus, it is probably playing a critical role in the breakdown of that cell compartment and in the progression of apoptosis (Kamada et al. 2005; Prokhorova et al. 2018).
These issues are important in the context of the present study because apoptosis is integral to the control of spermatogenesis; that is, it is needed to limit the number of germ cells that a Sertoli cell must support. It is thus no surprise that the Sertoli cells themselves control germ cell apoptosis through paracrine signalling that balances pro-apoptotic factors (caspase-3, Bax) and anti-apoptotic factors such as Bcl-2 (Murphy and Richburg 2014). Maternal undernutrition during pregnancy and lactation increased caspase-3 production in the detached spermatids seen in the lumen of the seminiferous tubules, suggesting a disruption of the elaborate network of cell junctions between Sertoli cells and spermatids that confers structural, communication and signalling support for the survival of developing germ cells (Gao et al. 2015). However, maternal undernutrition only during lactation led to a decrease in active caspase-3, mostly in spermatocytes and spermatids, and also an increase in Bax, mostly in spermatocytes, suggesting differential consequences in testicular cells according to the timing of undernutrition.
Interestingly, our results showed an increase Blc-2 in offspring’s testes from all maternal undernutrition groups, consistent with the inhibitory effects of Bcl-2 overexpression on normal spermatogonial apoptosis, which leads to accumulation of spermatogonia, forcing them to survive with consequences for differentiation during spermiogenesis (Furuchi et al. 1996; Shaha et al. 2010). As Bcl-2 is a pro-survival protein (Shamas-Din et al. 2013) and considered to be a ‘rheostat’ in Sertoli cells and germ cells (Yamamoto et al. 2001), upregulation of Bcl-2 genes causes severe attenuation of germ cell apoptosis (Wright et al. 2007). This situation is consistent with our observation of an increase in Bcl-2 proteins in the maternal undernutrition groups.
As mentioned before, the present observations need to be considered alongside our previous report, in which we described the effects of maternal undernutrition on the proliferation of germ cells in the same rat offspring cohort (Pedrana et al. 2020). Taken together, these observations suggest that in the testis of offspring of nutritionally compromised mothers, there is an imbalance between germ cell proliferation and apoptosis. The increase in proliferation is explained by increases in transcription factors (ETV5, GDNF) and in promotion of anti-apoptotic processes (Bcl-2, HSP70), leading to potentially harmful deregulation of spermatogenesis. The overall outcome is excessive proliferation, although the response to undernutrition seems to vary among the developmental windows, an observation requiring further investigation. Interestingly, the testicular anti-apoptotic mechanisms activated by maternal undernutrition seem to parallel other pathologies such as cancer (Montero and Letai 2018).
Heat shock protein 70
HSP70 is known to be a molecular chaperon for both meiosis (Scieglinska and Krawczyk 2015) and spermiogenesis (Govin et al. 2006), so these processes would be expected to be impaired by increased expression of HSP70 in the testis of offspring from mothers underfed during pregnancy and lactation (e.g. our UNPL group). Furthermore, overexpression of HSP70 is associated with resistance to apoptosis in other tissues, blocking apoptosome formation (Beere 2004) and increasing Bcl-2 expression in cerebral ischaemia (FitzGerald et al. 2009). Moreover, HSP70 expression is activated by hypoxia, a condition frequently associated with tumour progression (Scieglinska and Krawczyk 2015). On the other hand, an increase in HSP70 production has been seen as a protective mechanism in germ cells, preventing cellular damage induced by stress, elevated environmental temperatures or toxicants such as 2,5-hexanedione (Sertoli cell toxicant), ethylene glycol monomethyl ether (spermatocyte toxicant), cyclophosphamide (spermatogonia toxicant) and sulfasalazine (Fukushima et al. 2005) or lindane organochlorine pesticide (Saradha et al. 2008). The increase in HSP70 in the germ cells of adult offspring from undernourished mothers, detected by immunohistochemistry, could reflect activation of a protective mechanism.
Heat shock protein 90
Because HSP90 also plays a role as a chaperone protein (Dixit and Verkhivker 2012; Verba and Agard 2017), it is interesting that its expression was decreased by maternal undernutrition during pregnancy and lactation, in contrast with HSP70. However, HSP90 promotes the stability and activation of post-meiotic kinases in spermatids (Jha et al. 2013) during spermiogenesis, so a decrease or a complete absence of the protein would lead to testis atrophy and azoospermia (Grad et al. 2010), an increased sensitivity to apoptotic stimuli and a decrease in spermatid kinases (Jha et al. 2013) and oligoasthenozoospermia, as seen in men (Sagare-Patil et al. 2017). Moreover, the effects of maternal undernutrition on HSP90 expression are consistent with the decreases that we observed, in the same animals, in the frequency of Stage VII in the spermatogenic cycle, before spermiation begins (Pedrana et al. 2020). Overall, the testicular HSP90 response to maternal undernutrition would help explain changes in the process of spermiogenesis, as HSP90 is a determinant of the concentration of protamine, a factor necessary for DNA packaging during spermiation.
Conclusion
We conclude that maternal undernutrition during pregnancy and lactation modifies the processes that control the production of fertile spermatozoa in the testis of adult offspring (Fig. 6). The combination of increases in the transcription factors, ETV5 and GDNF, both promoters of proliferation, and Bcl-2 and HSP70, both anti-apoptotic and meiotic proteins, would accelerate stem cell renewal and thus lead to excessive proliferation of germ cells, while reducing the rate of apoptosis of germ cells. Finally, maternal undernutrition decreases the amount of HSP90 in the developing germ cells, probably leading to a reduction in the concentration of protamine, an essential factor in DNA packaging, so the final step of spermatogenesis, spermiogenesis, could also be compromised. These results are consistent with our previous observations, in the same animals, of increases in testis IGF-I and the proliferation of spermatogonia, and a reduction in the number of seminiferous tubules at Stage VII of the spermatogenic cycle, as would be expected if spermiogenesis was also altered.
Overall, our data suggest that maternal undernutrition alters the equilibrium between apoptosis and proliferation in spermatogonia and spermatocytes, and the differentiation of spermatids, in adult male rat offspring of undernourished mothers. This equilibrium is essential in the control of spermatogenesis, so maternal undernutrition is likely to have major and persisting effects on the regulation of fertility of male offspring.
Conflicts of interest
Graeme Martin is the Editor-in-Chief of Reproduction, Fertility and Development, but was blinded from the peer-review process for this paper.
Declaration of funding
This research was supported by Health Research Council of New Zealand, Gravida: National Centre for Growth and Development, and the Comisión de Investigación y Desarrollo Científico (CSIC) Foundation (CSIC Financial Support Grant 2016), and Universidad de la República, Uruguay. DMS holds a Canada Research Chair in Perinatal Programming.
Acknowledgements
We thank Mónica Viqueira, technician in the Histology Laboratory, for her help in microtome procedure of samples of rat testis, and Vanesa Pereira and Ronell Bologna for their kind help in the scanning of images.
References
Au, C. E., Hermo, L., Byrne, E., Smirle, J., Fazel, A., Simon, P. H. G., Kearney, R. E., Cameron, P. H., Smith, C. E., Vali, H., Fernandez-Rodriguez, J., Ma, K., Nilsson, T., and Bergeron, J. J. M. (2015). Expression, sorting, and segregation of Golgi proteins during germ cell differentiation in the testis. Mol. Biol. Cell 26, 4015–4032.| Expression, sorting, and segregation of Golgi proteins during germ cell differentiation in the testis.Crossref | GoogleScholarGoogle Scholar | 25808494PubMed |
Bateson, P., Barker, D., Clutton-Brock, T., Deb, D., D’Udine, B., Foley, R. A., Gluckman, P., Godfrey, K., Kirkwood, T., Lahr, M. M., McNamara, J., Metcalfe, N. B., Monaghan, P., Spencer, H. G., and Sultan, S. E. (2004). Developmental plasticity and human health. Nature 430, 419–421.
| Developmental plasticity and human health.Crossref | GoogleScholarGoogle Scholar | 15269759PubMed |
Beere, H. M. (2004). ‘The stress of dying’: the role of heat shock proteins in the regulation of apoptosis. J. Cell Sci. 117, 2641–2651.
| ‘The stress of dying’: the role of heat shock proteins in the regulation of apoptosis.Crossref | GoogleScholarGoogle Scholar | 15169835PubMed |
Bell, A. W., and Greenwood, P. L. (2016). Prenatal origins of postnatal variation in growth, development and productivity of ruminants. Anim. Prod. Sci. 56, 1217–1232.
| Prenatal origins of postnatal variation in growth, development and productivity of ruminants.Crossref | GoogleScholarGoogle Scholar |
Chen, S.-R., and Liu, Y.-X. (2015). Regulation of spermatogonial stem cell self-renewal and spermatocyte meiosis by Sertoli cell signaling. Reproduction 149, R159–R167.
| Regulation of spermatogonial stem cell self-renewal and spermatocyte meiosis by Sertoli cell signaling.Crossref | GoogleScholarGoogle Scholar | 25504872PubMed |
Cooke, P. S., Hess, R. A., Simon, L., Schlesser, H. N., Carnes, K., Tyagi, G., Hofmann, M.-C., and Murphy, K. M. (2006). The transcription factor Ets-Related Molecule (ERM) is essential for spermatogonial stem cell maintenance and self-renewal. Anim. Reprod. 3, 98–107.
Dixit, A., and Verkhivker, G. M. (2012). Probing Molecular Mechanisms of the Hsp90 Chaperone: Biophysical Modeling Identifies Key Regulators of Functional Dynamics. PLoS One 7, e37605.
| Probing Molecular Mechanisms of the Hsp90 Chaperone: Biophysical Modeling Identifies Key Regulators of Functional Dynamics.Crossref | GoogleScholarGoogle Scholar | 23284721PubMed |
Domingos, F. F. T., Thomé, R. G., Martinelli, P. M., Sato, Y., Bazzoli, N., and Rizzo, E. (2013). Role of HSP70 in the regulation of the testicular apoptosis in a seasonal breeding teleost Prochilodus argenteus from the São Francisco River, Brazil. Microsc. Res. Tech. 76, 350–356.
| Role of HSP70 in the regulation of the testicular apoptosis in a seasonal breeding teleost Prochilodus argenteus from the São Francisco River, Brazil.Crossref | GoogleScholarGoogle Scholar |
Figueiredo, A. F., França, L. R., Hess, R. A., and Costa, G. (2016). Sertoli cells are capable of proliferation into adulthood in the transition region between the seminiferous tubules and the rete testis in Wistar rats. Cell Cycle 15, 2486–2496.
| Sertoli cells are capable of proliferation into adulthood in the transition region between the seminiferous tubules and the rete testis in Wistar rats.Crossref | GoogleScholarGoogle Scholar | 27420022PubMed |
FitzGerald, U., Gorman, A. M., and Samali, A. (2009). Heat Shock Proteins and the Regulation of Apoptosis. In ‘Heat Shock Proteins in Neural Cells. Neuroscience Intelligence Unit’. (Ed. C. Richter-Landsberg.) pp. 53–66. (Springer New York: New York, NY)
França, L. R., Hess, R. A., Dufour, J. M., Hofmann, M. C., and Griswold, M. D. (2016). The Sertoli cell: One hundred fifty years of beauty and plasticity. Andrology 4, 189–212.
| The Sertoli cell: One hundred fifty years of beauty and plasticity.Crossref | GoogleScholarGoogle Scholar | 26846984PubMed |
Fukushima, T., Yamamoto, T., Kikkawa, R., Hamada, Y., Komiyama, M., Mori, C., and Horii, I. (2005). Effects of male reproductive toxicants on gene expression in rat testes. J. Toxicol. Sci. 30, 195–206.
| Effects of male reproductive toxicants on gene expression in rat testes.Crossref | GoogleScholarGoogle Scholar | 16141653PubMed |
Furuchi, T., Masuko, K., Nishimune, Y., Obinata, M., and Matsui, Y. (1996). Inhibition of testicular germ cell apoptosis and differentiation in mice misexpressing Bcl-2 in spermatogonia. Development 122, 1703–1709.
| 8674410PubMed |
Gao, Y., Mruk, D. D., and Cheng, C. Y. (2015). Sertoli cells are the target of environmental toxicants in the testis – a mechanistic and therapeutic insight. Expert Opin. Ther. Targets 19, 1073–1090.
| Sertoli cells are the target of environmental toxicants in the testis – a mechanistic and therapeutic insight.Crossref | GoogleScholarGoogle Scholar | 25913180PubMed |
Govin, J., Caron, C., Escoffier, E., Ferro, M., Kuhn, L., Rousseaux, S., Eddy, E. M., Garin, J., and Khochbin, S. (2006). Post-meiotic shifts in HSPA2/HSP70.2 chaperone activity during mouse spermatogenesis. J. Biol. Chem. 281, 37888–37892.
| Post-meiotic shifts in HSPA2/HSP70.2 chaperone activity during mouse spermatogenesis.Crossref | GoogleScholarGoogle Scholar | 17035236PubMed |
Grad, I., Cederroth, C. R., Walicki, J., Grey, C., Barluenga, S., Winssinger, N., De Massy, B., Nef, S., and Picard, D. (2010). The molecular chaperone Hsp90α is required for meiotic progression of spermatocytes beyond pachytene in the mouse. PLoS One 5, e15770.
| The molecular chaperone Hsp90α is required for meiotic progression of spermatocytes beyond pachytene in the mouse.Crossref | GoogleScholarGoogle Scholar | 21209834PubMed |
Hess, R. A., Schaeffer, D. J., Eroschenko, V. P., and Keen, J. E. (1990). Frequency of the stages in the cycle of the seminiferous epithelium in the rat. Biol. Reprod. 43, 517–524.
| Frequency of the stages in the cycle of the seminiferous epithelium in the rat.Crossref | GoogleScholarGoogle Scholar | 2271733PubMed |
Hofmann, M.-C. (2008). Gdnf signaling pathways within the mammalian spermatogonial stem cell niche. Mol. Cell. Endocrinol. 288, 95–103.
| Gdnf signaling pathways within the mammalian spermatogonial stem cell niche.Crossref | GoogleScholarGoogle Scholar | 18485583PubMed |
Howie, G. J., Sloboda, D. M., and Vickers, M. H. (2012). Maternal undernutrition during critical windows of development results in differential and sex-specific effects on postnatal adiposity and related metabolic profiles in adult rat offspring. Br. J. Nutr. 108, 298–307.
| Maternal undernutrition during critical windows of development results in differential and sex-specific effects on postnatal adiposity and related metabolic profiles in adult rat offspring.Crossref | GoogleScholarGoogle Scholar | 22018052PubMed |
Ishii, K., Kanatsu-Shinohara, M., Toyokuni, S., and Shinohara, T. (2012). FGF2 mediates mouse spermatogonial stem cell self-renewal via upregulation of Etv5 and Bcl6b through MAP2K1 activation. Development 139, 1734–1743.
| FGF2 mediates mouse spermatogonial stem cell self-renewal via upregulation of Etv5 and Bcl6b through MAP2K1 activation.Crossref | GoogleScholarGoogle Scholar | 22491947PubMed |
Jazwiec, P. A., and Sloboda, D. M. (2019). Nutritional adversity, sex and reproduction: 30 years of DOHaD and what have we learned? J. Endocrinol. 242, T51–T68.
| Nutritional adversity, sex and reproduction: 30 years of DOHaD and what have we learned?Crossref | GoogleScholarGoogle Scholar | 31013473PubMed |
Jha, K. N., Coleman, A. R., Wong, L., Salicioni, A. M., Howcroft, E., and Johnson, G. R. (2013). Heat Shock Protein 90 Functions to Stabilize and Activate the Testis-specific Serine/Threonine Kinases, a Family of Kinases Essential for Male Fertility. J. Biol. Chem. 288, 16308–16320.
| Heat Shock Protein 90 Functions to Stabilize and Activate the Testis-specific Serine/Threonine Kinases, a Family of Kinases Essential for Male Fertility.Crossref | GoogleScholarGoogle Scholar | 23599433PubMed |
Jijiwa, M., Kawai, K., Fukihara, J., Nakamura, A., Hasegawa, M., Suzuki, C., Sato, T., Enomoto, A., Asai, N., Murakumo, Y., and Takahashi, M. (2008). GDNF-mediated signaling via RET tyrosine 1062 is essential for maintenance of spermatogonial stem cells. Genes Cells 13, 365–374.
| GDNF-mediated signaling via RET tyrosine 1062 is essential for maintenance of spermatogonial stem cells.Crossref | GoogleScholarGoogle Scholar | 18363967PubMed |
Johnston, D. S., Olivas, E., DiCandeloro, P., and Wright, W. W. (2011). Stage-Specific Changes in GDNF Expression by Rat Sertoli Cells: A Possible Regulator of the Replication and Differentiation of Stem Spermatogonia. Biol. Reprod. 85, 763–769.
| Stage-Specific Changes in GDNF Expression by Rat Sertoli Cells: A Possible Regulator of the Replication and Differentiation of Stem Spermatogonia.Crossref | GoogleScholarGoogle Scholar | 21653894PubMed |
Kamada, S., Kikkawa, U., Tsujimoto, Y., and Hunter, T. (2005). Nuclear translocation of caspase-3 is dependent on its proteolytic activation and recognition of a substrate-like protein(s). J. Biol. Chem. 280, 857–860.
| Nuclear translocation of caspase-3 is dependent on its proteolytic activation and recognition of a substrate-like protein(s).Crossref | GoogleScholarGoogle Scholar | 15569692PubMed |
Kennedy, D., Jäger, R., Mosser, D. D., and Samali, A. (2014). Regulation of apoptosis by heat shock proteins. IUBMB Life 66, 327–338.
| Regulation of apoptosis by heat shock proteins.Crossref | GoogleScholarGoogle Scholar | 24861574PubMed |
Kereliuk, S., Brawerman, G., Dolinsky, V., Kereliuk, S. M., Brawerman, G. M., and Dolinsky, V. W. (2017). Maternal Macronutrient Consumption and the Developmental Origins of Metabolic Disease in the Offspring. Int. J. Mol. Sci. 18, 1451.
| Maternal Macronutrient Consumption and the Developmental Origins of Metabolic Disease in the Offspring.Crossref | GoogleScholarGoogle Scholar |
Khorram, O., Keen-Rinehart, E., Der Chuang, T., Ross, M. G., and Desai, M. (2015). Maternal undernutrition induces premature reproductive senescence in adult female rat offspring. Fertil. Steril. 103, 291–298.
| Maternal undernutrition induces premature reproductive senescence in adult female rat offspring.Crossref | GoogleScholarGoogle Scholar | 25439841PubMed |
Kotoglou, P., Kalaitzakis, A., Vezyraki, P., Tzavaras, T., Michalis, L. K., Dantzer, F., Jung, J. U., and Angelidis, C. (2009). Hsp70 translocates to the nuclei and nucleoli, binds to XRCC1 and PARP-1, and protects HeLa cells from single-strand DNA breaks. Cell Stress Chaperones 14, 391–406.
| Hsp70 translocates to the nuclei and nucleoli, binds to XRCC1 and PARP-1, and protects HeLa cells from single-strand DNA breaks.Crossref | GoogleScholarGoogle Scholar | 19089598PubMed |
Langley-Evans, S. C. (2015). Nutrition in early life and the programming of adult disease: a review. J. Hum. Nutr. Diet. 28, 1–14.
| Nutrition in early life and the programming of adult disease: a review.Crossref | GoogleScholarGoogle Scholar | 24479490PubMed |
Langley-Evans, S. C., and McMullen, S. (2010). Developmental origins of adult disease. Med. Princ. Pract. 19, 87–98.
| Developmental origins of adult disease.Crossref | GoogleScholarGoogle Scholar | 20134170PubMed |
Léonhardt, M., Lesage, J., Croix, D., Dutriez-Casteloot, I., Beauvillain, J. C., and Dupouy, J. P. (2003). Effects of perinatal maternal food restriction on pituitary-gonadal axis and plasma leptin level in rat pup at birth and weaning and on timing of puberty. Biol. Reprod. 68, 390–400.
| Effects of perinatal maternal food restriction on pituitary-gonadal axis and plasma leptin level in rat pup at birth and weaning and on timing of puberty.Crossref | GoogleScholarGoogle Scholar | 12533401PubMed |
Letai, A., and Kutuk, O. (2008). Regulation of Bcl-2 Family Proteins by Posttranslational Modifications. Curr. Mol. Med. 8, 102–118.
| Regulation of Bcl-2 Family Proteins by Posttranslational Modifications.Crossref | GoogleScholarGoogle Scholar | 18336291PubMed |
Martin, G. B., Blache, D., Miller, D. W., and Vercoe, P. E. (2010). Interactions between nutrition and reproduction in the management of the mature male ruminant. Animal 4, 1214–1226.
| Interactions between nutrition and reproduction in the management of the mature male ruminant.Crossref | GoogleScholarGoogle Scholar | 22444618PubMed |
Meng, X., Lindahl, M., Hyvönen, M. E., Parvinen, M., de Rooij, D. G., Hess, M. W., Raatikainen-Ahokas, A., Sainio, K., Rauvala, H., Lakso, M., Pichel, J. G., Westphal, H., Saarma, M., and Sariola, H. (2000). Regulation of cell fate decision of undifferentiated spermatogonia by GDNF. Science 287, 1489–1493.
| Regulation of cell fate decision of undifferentiated spermatogonia by GDNF.Crossref | GoogleScholarGoogle Scholar | 10688798PubMed |
Montero, J., and Letai, A. (2018). Why do BCL-2 inhibitors work and where should we use them in the clinic? Cell Death Differ. 25, 56–64.
| Why do BCL-2 inhibitors work and where should we use them in the clinic?Crossref | GoogleScholarGoogle Scholar | 29077093PubMed |
Murphy, C. J., and Richburg, J. H. (2014). Implications of Sertoli cell induced germ cell apoptosis to testicular pathology. Spermatogenesis 4, e979110.
| Implications of Sertoli cell induced germ cell apoptosis to testicular pathology.Crossref | GoogleScholarGoogle Scholar | 26413394PubMed |
Pedrana, G., Viotti, H., Lombide, P., Cavestany, D., Martin, G. B., Vickers, M. H., and Sloboda, D. M. (2020). Maternal undernutrition during pregnancy and lactation affects testicular morphology, the stages of spermatogenic cycle, and the testicular IGF-I system in adult offspring. J. Dev. Orig. Health Dis. 11, 473–483.
| Maternal undernutrition during pregnancy and lactation affects testicular morphology, the stages of spermatogenic cycle, and the testicular IGF-I system in adult offspring.Crossref | GoogleScholarGoogle Scholar | 32340648PubMed |
Prokhorova, E. A., Kopeina, G. S., Lavrik, I. N., and Zhivotovsky, B. (2018). Apoptosis regulation by subcellular relocation of caspases. Sci. Rep. 8, 12199.
| Apoptosis regulation by subcellular relocation of caspases.Crossref | GoogleScholarGoogle Scholar | 30111833PubMed |
Puli, O. R., Danysh, B. P., McBeath, E., Sinha, D. K., Hoang, N. M., Powell, R. T., Danysh, H. E., Cabanillas, M. E., Cote, G. J., and Hofmann, M. C. (2018). The Transcription Factor ETV5 Mediates BRAFV600E-Induced Proliferation and TWIST1 Expression in Papillary Thyroid Cancer Cells. Neoplasia 20, 1121–1134.
| The Transcription Factor ETV5 Mediates BRAFV600E-Induced Proliferation and TWIST1 Expression in Papillary Thyroid Cancer Cells.Crossref | GoogleScholarGoogle Scholar | 30265861PubMed |
Rossi, P., and Dolci, S. (2013). Paracrine Mechanisms Involved in the Control of Early Stages of Mammalian Spermatogenesis. Front. Endocrinol. 4, 181.
| Paracrine Mechanisms Involved in the Control of Early Stages of Mammalian Spermatogenesis.Crossref | GoogleScholarGoogle Scholar |
Russell, L. D., Chiarini-garcia, H., Korsmeyer, S. J., and Knudson, C. M. (2002). Bax-Dependent Spermatogonia Apoptosis Is Required for Testicular Development. Biol. Reprod. 66, 950–958.
| Bax-Dependent Spermatogonia Apoptosis Is Required for Testicular Development.Crossref | GoogleScholarGoogle Scholar | 11906913PubMed |
Sagare-Patil, V., Bhilawadikar, R., Galvankar, M., Zaveri, K., Hinduja, I., and Modi, D. (2017). Progesterone requires heat shock protein 90 (HSP90) in human sperm to regulate motility and acrosome reaction. J. Assist. Reprod. Genet. 34, 495–503.
| Progesterone requires heat shock protein 90 (HSP90) in human sperm to regulate motility and acrosome reaction.Crossref | GoogleScholarGoogle Scholar | 28236106PubMed |
Saradha, B., Vaithinathan, S., and Mathur, P. P. (2008). Lindane alters the levels of HSP70 and clusterin in adult rat testis. Toxicology 243, 116–123.
| Lindane alters the levels of HSP70 and clusterin in adult rat testis.Crossref | GoogleScholarGoogle Scholar | 17997001PubMed |
Sarge, K. D., and Cullen, K. E. (1997). Regulation of hsp expression during rodent spermatogenesis. Cell. Mol. Life Sci. 53, 191–197.
| Regulation of hsp expression during rodent spermatogenesis.Crossref | GoogleScholarGoogle Scholar | 9118007PubMed |
Schlesser, H. N., Simon, L., Hofmann, M. C., Murphy, K. M., Murphy, T., Hess, R. A., and Cooke, P. S. (2008). Effects of ETV5 (Ets variant gene 5) on testis and body growth, time course of spermatogonial stem cell loss, and fertility in mice. Biol. Reprod. 78, 483–489.
| Effects of ETV5 (Ets variant gene 5) on testis and body growth, time course of spermatogonial stem cell loss, and fertility in mice.Crossref | GoogleScholarGoogle Scholar | 18032421PubMed |
Schmidt, J. A., Avarbock, M. R., Tobias, J. W., and Brinster, R. L. (2009). Identification of glial cell line-derived neurotrophic factor-regulated genes important for spermatogonial stem cell self-renewal in the rat. Biol. Reprod. 81, 56–66.
| Identification of glial cell line-derived neurotrophic factor-regulated genes important for spermatogonial stem cell self-renewal in the rat.Crossref | GoogleScholarGoogle Scholar | 19339709PubMed |
Scieglinska, D., and Krawczyk, Z. (2015). Expression, function, and regulation of the testis-enriched heat shock HSPA2 gene in rodents and humans. Cell Stress Chaperones 20, 221–235.
| Expression, function, and regulation of the testis-enriched heat shock HSPA2 gene in rodents and humans.Crossref | GoogleScholarGoogle Scholar | 25344376PubMed |
Selim, M. E., Rashed, E. H., Aleisa, N., and Daghestani, M. H. (2012). The protection role of heat shock protein 70 (HSP-70) in the testes of cadmium-exposed rats. Bioinformation 8, 58–64.
| The protection role of heat shock protein 70 (HSP-70) in the testes of cadmium-exposed rats.Crossref | GoogleScholarGoogle Scholar | 22359436PubMed |
Shaha, C., Tripathi, R., and Prasad Mishra, D. (2010). Male germ cell apoptosis: Regulation and biology. Philos. Trans. R. Soc. Lond. B Biol. Sci. 365, 1501–1515.
| Male germ cell apoptosis: Regulation and biology.Crossref | GoogleScholarGoogle Scholar | 20403866PubMed |
Shamas-Din, A., Kale, J., Leber, B., and Andrews, D. W. (2013). Mechanisms of action of Bcl-2 family proteins. Cold Spring Harb. Perspect. Biol. 5, a008714.
| Mechanisms of action of Bcl-2 family proteins.Crossref | GoogleScholarGoogle Scholar | 23545417PubMed |
Simon, L., Ekman, G. C., Tyagi, G., Hess, R. A., Murphy, K. M., and Cooke, P. S. (2007). Common and distinct factors regulate expression of mRNA for ETV5 and GDNF, Sertoli cell proteins essential for spermatogonial stem cell maintenance. Exp. Cell Res. 313, 3090–3099.
| Common and distinct factors regulate expression of mRNA for ETV5 and GDNF, Sertoli cell proteins essential for spermatogonial stem cell maintenance.Crossref | GoogleScholarGoogle Scholar | 17574550PubMed |
Sinclair, K. D., Rutherford, K. M. D., Wallace, J. M., Brameld, J. M., Stöger, R, Alberio, R, Sweetman, D, Gardner, D. S., Perry, V. E. A., Adam, C. L., Ashworth, C. J., Robinson, J. E., and Dwyer, C. M. (2016). Epigenetics and developmental programming of welfare and production traits in farm animals. Reprod., Fertil. Dev. 28, 1443–1478.
| Epigenetics and developmental programming of welfare and production traits in farm animals.Crossref | GoogleScholarGoogle Scholar |
Singh, D., Paduch, D. A., Schlegel, P. N., Orwig, K. E., Mielnik, A., Bolyakov, A., and Wright, W. W. (2017). The production of glial cell line-derived neurotrophic factor by human sertoli cells is substantially reduced in sertoli cell-only testes. Hum. Reprod. 32, 1108–1117.
| The production of glial cell line-derived neurotrophic factor by human sertoli cells is substantially reduced in sertoli cell-only testes.Crossref | GoogleScholarGoogle Scholar | 28369535PubMed |
Tadokoro, Y., Yomogida, K., Ohta, H., Tohda, A., and Nishimune, Y. (2002). Homeostatic regulation of germinal stem cell proliferation by the GDNF/FSH pathway. Mech. Dev. 113, 29–39.
| Homeostatic regulation of germinal stem cell proliferation by the GDNF/FSH pathway.Crossref | GoogleScholarGoogle Scholar | 11900972PubMed |
Takashima, S., Kanatsu-Shinohara, M., Tanaka, T., Morimoto, H., Inoue, K., Ogonuki, N., Jijiwa, M., Takahashi, M., Ogura, A., and Shinohara, T. (2015). Functional differences between GDNF-dependent and FGF2-dependent mouse spermatogonial stem cell self-renewal. Stem Cell Reports 4, 489–502.
| Functional differences between GDNF-dependent and FGF2-dependent mouse spermatogonial stem cell self-renewal.Crossref | GoogleScholarGoogle Scholar | 25684228PubMed |
Toledo, F. C., Perobelli, J. E., Pedrosa, F. P. C., Anselmo-Franci, J. A., and Kempinas, W. D. G. (2011). In utero protein restriction causes growth delay and alters sperm parameters in adult male rats. Reprod. Biol. Endocrinol. 9, 94.
| In utero protein restriction causes growth delay and alters sperm parameters in adult male rats.Crossref | GoogleScholarGoogle Scholar | 21702915PubMed |
Tyagi, G., Carnes, K., Morrow, C., Kostereva, N. V., Ekman, G. C., Meling, D. D., Hostetler, C., Griswold, M., Murphy, K. M., Hess, R. A., Hofmann, M. C., and Cooke, P. S. (2009). Loss of Etv5 decreases proliferation and RET levels in neonatal mouse testicular germ cells and causes an abnormal first wave of spermatogenesis. Biol. Reprod. 81, 258–266.
| Loss of Etv5 decreases proliferation and RET levels in neonatal mouse testicular germ cells and causes an abnormal first wave of spermatogenesis.Crossref | GoogleScholarGoogle Scholar | 19369650PubMed |
Verba, K. A., and Agard, D. A. (2017). How Hsp90 and Cdc37 Lubricate Kinase Molecular Switches. Trends Biochem. Sci. 42, 799–811.
| How Hsp90 and Cdc37 Lubricate Kinase Molecular Switches.Crossref | GoogleScholarGoogle Scholar | 28784328PubMed |
Wadhwa, P. D., Buss, C., Entringer, S., and Swanson, J. M. (2009). Developmental origins of health and disease: Brief history of the approach and current focus on epigenetic mechanisms. Semin. Reprod. Med. 27, 358–368.
| Developmental origins of health and disease: Brief history of the approach and current focus on epigenetic mechanisms.Crossref | GoogleScholarGoogle Scholar | 19711246PubMed |
Wright, A., Reiley, W. W., Chang, M., Jin, W., Lee, A. J., Zhang, M., and Sun, S. C. (2007). Regulation of Early Wave of Germ Cell Apoptosis and Spermatogenesis by Deubiquitinating Enzyme CYLD. Dev. Cell 13, 705–716.
| Regulation of Early Wave of Germ Cell Apoptosis and Spermatogenesis by Deubiquitinating Enzyme CYLD.Crossref | GoogleScholarGoogle Scholar | 17981138PubMed |
Yamamoto, C. M., Hikim, P., Lue, Y., Portugal, M., Guo, T. B., Hsu, S. Y., Salameh, W., Wang, C., Hsueh, J., and Swerdloff, R. S. (2001). Impairment of spermatogenesis in transgenic mice with selective overexpression of Bcl-2 in the somatic cells of the testis. J. Androl. 22, 981–991.
| Impairment of spermatogenesis in transgenic mice with selective overexpression of Bcl-2 in the somatic cells of the testis.Crossref | GoogleScholarGoogle Scholar | 11700863PubMed |