Annual response patterns in activity and demographic parameters of reptile species occupying a predictable Mediterranean climate
Richard A. How
A
B
Abstract
Annual patterns in behaviour and demography define longer-term changes in reptile populations, probability of species detection and risks of local extinction.
To determine seasonal activity and demographic parameters of species in an isolated assemblage adapted to a seasonally predictable climate.
A 37-year pitfall trapping, capture–release study in a 338-ha inner-city bushland experiencing a Mediterranean climate. Pit trap methodology changed after 7 years.
Reproductive activity of 29 species peaked during austral spring to early summer with hatching or births occurring from late summer to early autumn. Growth, as determined from size changes over demi-monthly periods, indicated sexual maturity varied from <1 year to 5 years. Five of eight uniquely marked species had longevity exceeding a decade while individuals within six species moved over a kilometre. Dimorphism in adult length and mass was significantly greater in females for 15 of the 18 species with adequate data. Adult male activity peaked earlier in spring than adult females’ over the latter 30 years; hatchlings had different species-specific activity and annual cohort survival patterns. Activity declined in adults through summer and autumn before brumation in late autumn and winter in all species and age-size categories. Capture frequency in most species changed between methodologies.
Species had broadly similar seasonal activity but different demographic parameters within an isolated reptile assemblage currently experiencing increasing temperature and declining rainfall.
Comprehending seasonal variation is essential for interpreting decadal-long changes in reptile numbers experiencing a drying climate that alters activity, reproduction and survival to inform species detectability and likely extinction risk.
Keywords: activity, demographic parameters, detectability, local extinction risk, longevity, movement, reproduction, reptile populations, seasonal variation.
Introduction
Abundant species define assemblage structure and function (Gaston and Fuller 2008) and best indicate the realised and probable impacts to changes in habitat and climate over time and space to biota in any region. To interpret the likely consequences of those changes, long-term data are fundamental to understanding population dynamics and assessing extinction risk of species (Clutton-Brock and Sheldon 2010; Hero et al. 2010; Lindenmayer et al. 2012; White 2019), both criteria that form a basis for the IUCN Red List Category criterion of species’ population declines over a decade (IUCN 2012). The extensive review of long-term individual-based and population-based studies by Clutton-Brock and Sheldon (2010) illustrated the contribution of both approaches to ecology but highlighted the additional benefits of individual-based long term studies to understanding evolutionary processes. Examining 822 time series population studies, of longer than 35 years, White (2019) determined that, on average, nearly 16 years of continuous monitoring were required to identify significant long-term changes in species abundance; none of those studies included ectothermic terrestrial vertebrates such as reptiles. Long-term data are also valuable to documentation and management of biodiversity under regimes where anthropogenic changes in landscape structure and climate may be obfuscated by short-term seasonal impacts on demography (Magurran et al. 2010).
The rich biotic diversity reported on the landforms of south-western Western Australia (WA) for both flora (Lamont et al. 1977; Beard et al. 2000; Hopper and Gioia 2004) and vertebrate fauna (Kitchener et al. 1980a, 1980b, 1982; Recher and Serventy 1991) informed the later recognition of south-western WA as one of the global biodiversity ‘hotspots’ by Myers et al. (2000); who also later noted that conservation of all hotspots had the potential to reduce global extinction by a third (Myers 2003). The south-western WA region encompasses the Swan Coastal Plain (SCP) and Perth Metropolitan area where biodiversity surveys documented the historic and the then recent vertebrate faunas, to conclude that nearly half the natural vegetation, over a third of native mammals, several birds, but no reptiles or frogs, had become locally extinct over 150 years of European occupation (Kitchener et al. 1978; Storr et al. 1978a, 1978b; Western Australian Museum 1978; Davis et al. 2013). The entire south-western WA experiences a Mediterranean climate, characterised by cool wet winters following hot dry summers with a predictability in that annual cycle in both rainfall and temperature (Di Castri 1991).
Informative to those earlier intensive biodiversity appraisals in south-western WA was the significant international research on reptile fauna of WA that highlighted its global importance (Pianka and Pianka 1976; Pianka 1981, 1986; Bradshaw 1986, also references in Aplin and How 1993). Decadal long studies by Pianka (1986) on desert reptile assemblages documented WA’s rich and diverse reptilian fauna and this was complemented by eco-physiological work undertaken by the University of WA (Baverstock 1978; Bradshaw 1986). The SCP contains a highly diverse reptile fauna (Storr et al. 1978b; Davidge 1979; Bush et al. 1995) and later intensive biodiversity surveys in Perth Metropolitan’s smaller urban and peri-urban remnant bushlands (How and Dell 1994, 2000, also references in Li et al. 2023) indicated that numerous local extinctions had occurred in those remnants as a result of fragmentation, habitat loss, predation and fire frequency across a landscape experiencing rapid alteration from development.
A long-term study of the herpetofauna in Bold Park, an inner urban bushland remnant of Perth, was initiated in 1986 to document reptile species occurrence, their seasonal activity, habitat preferences, relative abundance, and assemblage structure within a biodiverse but isolated environment (How 1998). That study continues and illustrated that total relative abundance of reptiles in the assemblage was highly correlated with residual rainfall pattern from 1 to 4 years preceding annual sampling over a 28-year period (How et al. 2022). They postulated that the observed lag-response in abundance could be explained by variation in reproduction and survival of the composite species to altered resource states under changed rainfall; the 16 most regularly trapped accounting for over 97% of all captures. They also documented that three, possibly four, species had become locally extinct over that 35-year study.
Essential to understanding the observed decadal changes in total abundance is the interpretation of the annual activity patterns and age structure of in situ reptile species populations since variation in their breeding parameters and survival responses determines total assemblage abundance. Those demographic parameters are documented by this study through both population-based and individual-based assessments of reptile species comprising the Bold Park assemblage.
Materials and methods
Sample sites
Bold Park encompasses 437 ha of coastal bushland within the Perth Metropolitan Area of Western Australia, the largest area of intact native bushland in the central metropolitan area (How and Dell 2000). It comprises a contiguous central section of 338 ha circumscribed by dual-carriageway and double-lane bitumen roads some 9 km west of Perth and <1 km east of the Indian Ocean. Elevated to A-Class Reserve (A45409) on the 10 August 1998, its administration and management for passive recreation and conservation has operated for over 60 years, first by the Perth City Council then later by the Botanic Gardens and Parks Authority. Reabold Hill, at 85 m elevation within the Park, is the highest point on the SCP.
The Park supports a diverse array of native vegetation communities and a rich flora of 310 native and 232 non-native plant species. Tuart and Banksia woodlands, both declared Threatened Communities, as well as heaths on limestone predominate (Keighery et al. 1990). Twenty-nine species of reptile, three native and five introduced mammals, 91 bird, and four amphibian species have been recorded in Bold Park (How and Dell 1990), none of which are listed as threatened taxa under State or Commonwealth legislation. Fires have been very infrequent in Bold Park during the study period (How and Dell 1994; Dell and How 1995; Dunstan 2013).
Four sites were selected for sampling in 1986 that comprised different principal vegetation formations, covering the greatest variability of the Park’s heterogeneity: (1) BP1, mixed coastal heath; (2) BP2, Banksia sessilis shrubland; (3) BP3, Banksia attenuata/Banksia menziesii low woodland; and (4) BP4, Eucalyptus gomphocephala (Tuart) woodland. In 1993, Banksia shrubland (BP2) was substituted by mixed inland heath (BP5) to expand the geographic coverage and diversity of soil and habitats sampled.
Dunstan (2013) appraised historical panchromatic photographs of Bold Park, taken after 1963, and documented an absence of fires on the selected sampling sites since well before that time; a notable exception being the intense fire that burnt all the Tuart Woodland (BP4) site and nearly 100 ha adjacent in December 2000.
Monitoring regime and duration
The monitoring regime utilised three different sampling protocols (Table 1) during the decadal history of the program (How et al. 2022). A pilot sampling (Regime I) commenced at each site during December 1986 and involved trapping for four consecutive days and nights during five different months – December 1986; and February, March, May, and June 1987 – terminating on probable hibernation of individuals over winter. Each site consisted of six pitfall traps, comprising PVC pipes of 15-cm diameter and 60-cm depth, at 8-m spacing along a 50 m (long) and 30 cm (high) fly-wire fence line (How 1998). Sampling was refined and expanded in September 1987 (Regime II) to encompass trapping for at least 50 days over 9 months (September–May) for each of the next 6 years (from 1987–1988 to 1992–1993, inclusive); those months were defined as the annual sampling period (Table 1).
Month (demi-month) | August B | September A | September B | October A | October B | November A | November B | December A | December B | January A | January B | February A | February B | March A | March B | April A | April B | May A | May B | June A | June B | Total | |
---|---|---|---|---|---|---|---|---|---|---|---|---|---|---|---|---|---|---|---|---|---|---|---|
Demi-month | 8B | 9A | 9B | 10A | 10B | 11A | 11B | 12A | 12B | 1A | 1B | 2A | 2B | 3A | 3B | 4A | 4B | 5A | 5B | 6A | 6B | ||
Regime I | 96 | 96 | 96 | 96 | 96 | 480 | |||||||||||||||||
Regime II | 192 | 72 | 600 | 576 | 504 | 1344 | 984 | 816 | 528 | 432 | 360 | 816 | 264 | 720 | 576 | 120 | 192 | 9096 | |||||
Regime III | 216 | 432 | 1008 | 4758 | 11,157 | 14,850 | 14,484 | 15,900 | 9621 | 3615 | 6723 | 9192 | 9225 | 9177 | 3846 | 1029 | 144 | 115,377 | |||||
Species | |||||||||||||||||||||||
Ctenotus fallens | 3 | 3 | 65 | 149 | 340 | 234 | 246 | 94 | 63 | 482 | 720 | 386 | 340 | 85 | 18 | 1 | 3229 | ||||||
Strophurus spinigerus | 2 | 3 | 24 | 188 | 439 | 524 | 737 | 522 | 196 | 165 | 94 | 99 | 60 | 9 | 3 | 3065 | |||||||
Hemiergis quadrilineatus | 1 | 2 | 128 | 354 | 677 | 772 | 763 | 189 | 25 | 27 | 19 | 33 | 49 | 13 | 4 | 3056 | |||||||
Lerista elegans | 4 | 8 | 130 | 263 | 234 | 226 | 272 | 120 | 48 | 114 | 141 | 152 | 104 | 26 | 7 | 1 | 1850 | ||||||
Lerista lineopunctulata | 1 | 20 | 63 | 209 | 420 | 374 | 161 | 50 | 53 | 62 | 30 | 18 | 2 | 1 | 1 | 1465 | |||||||
Simoselaps bertholdi | 1 | 4 | 23 | 121 | 148 | 85 | 74 | 42 | 30 | 40 | 55 | 51 | 40 | 13 | 1 | 728 | |||||||
Menetia greyii | 2 | 3 | 53 | 101 | 87 | 68 | 52 | 19 | 7 | 14 | 6 | 10 | 3 | 3 | 1 | 429 | |||||||
Anilios australis | 2 | 35 | 72 | 111 | 57 | 57 | 36 | 11 | 13 | 1 | 7 | 5 | 407 | ||||||||||
Pogona minor | 1 | 1 | 1 | 13 | 27 | 46 | 54 | 41 | 12 | 8 | 19 | 29 | 37 | 56 | 15 | 360 | |||||||
Cryptoblepharus buchananii | 2 | 5 | 22 | 35 | 45 | 47 | 24 | 6 | 30 | 19 | 38 | 34 | 8 | 2 | 317 | ||||||||
Lialis burtonis | 7 | 24 | 32 | 40 | 38 | 33 | 5 | 12 | 18 | 11 | 12 | 3 | 235 | ||||||||||
Lerista praepedita | 1 | 7 | 15 | 99 | 85 | 36 | 14 | 4 | 8 | 9 | 8 | 3 | 2 | 1 | 292 | ||||||||
Tiliqua rugosa | 1 | 3 | 25 | 27 | 41 | 30 | 22 | 4 | 4 | 3 | 2 | 2 | 2 | 166 | |||||||||
Brachyurophis semifasciatus | 20 | 47 | 63 | 31 | 17 | 5 | 10 | 1 | 194 | ||||||||||||||
Aprasia repens | 2 | 24 | 61 | 24 | 23 | 22 | 7 | 1 | 3 | 4 | 8 | 179 | |||||||||||
Narophis bimaculatus | 4 | 19 | 40 | 19 | 26 | 10 | 11 | 4 | 4 | 9 | 1 | 147 | |||||||||||
Ctenotus australis | 1 | 3 | 9 | 23 | 5 | 4 | 5 | 13 | 12 | 8 | 83 | ||||||||||||
Ctenophorus chapmani | 1 | 2 | 1 | 14 | 17 | 11 | 15 | 6 | 3 | 3 | 3 | 1 | 4 | 1 | 82 | ||||||||
Varanus gouldii | 1 | 1 | 2 | 28 | 34 | 6 | 1 | 1 | 74 | ||||||||||||||
Cyclodomorphus celatus | 1 | 1 | 9 | 11 | 11 | 7 | 4 | 2 | 6 | 4 | 4 | 1 | 1 | 62 | |||||||||
Neelaps calonotos | 1 | 3 | 2 | 2 | 8 | 3 | 4 | 5 | 3 | 3 | 7 | 5 | 9 | 3 | 58 | ||||||||
Christinus marmoratus | 1 | 2 | 2 | 7 | 6 | 5 | 1 | 6 | 7 | 9 | 5 | 1 | 52 | ||||||||||
Pseudonaja affinis | 1 | 2 | 3 | 5 | 1 | 2 | 9 | 9 | 3 | 1 | 36 | ||||||||||||
Brachyurophis fasciolatus | 3 | 1 | 5 | 1 | 1 | 11 | |||||||||||||||||
Heternotia binoei | 2 | 1 | 2 | 6 | 3 | 2 | 16 | ||||||||||||||||
Morethia obscura | 1 | 3 | 2 | 2 | 1 | 1 | 10 | ||||||||||||||||
Lucasium alboguttatum | 1 | 2 | 2 | 5 | |||||||||||||||||||
Pletholax gracilis | 2 | 1 | 3 | ||||||||||||||||||||
Echiopsis curta | 1 | 1 | 2 |
The numbers of captures of the 29 lizard species caught during Regime III for each demi-month are indicated. Demi-monthly periods A = days 1–15; B = days 16–28/31.
From September 1993 to April 2023 (Regime III – now 30 years), pitfall trap type and configuration at each monitoring site was altered to nine traps, comprising 20-L buckets (29 cm diameter, 39 cm deep), spaced 10 m apart and arranged in a 3 × 3 grid. Individual traps were overlain by a 7 m long 30 cm high fly-wire fence placed across the buckets. The altered trapping regime was implemented to standardise with the broader study of the ground fauna in bushland remnants across the Perth Metropolitan Region over the period 1993–1997 (How and Dell 2000). A fifth site (BP5) in inland heath vegetation replaced the Banksia shrubland site (BP2) at the commencement of this regime, while trapping effort increased from around 1500 trap days to over 3000 trap days, annually, for the subsequent 30 years.
Handling and interpretation of biological data
The nomenclature for all reptile species and families follows the Western Australian Museum (2023) – Checklist-Terrestrial-Vertebrates.
Captured individuals were identified (to species level) and measured for snout-vent length (SVL), tail length and tail regeneration to the nearest 0.5 mm using Vernier callipers. Body mass was recorded to the nearest 0.1 g using Pesola balances (5 g, 10 g) and nearest 1.0 g for balances (50 g, 100 g and 1 kg). Additional comments pertaining to an individual (e.g. body and reproductive condition, scars etc.) were also recorded. Individuals were released immediately post measurement within 2 m of the pitfall trap of capture. Species with pentadactyl limbs were individually marked by toe-clipping before release. All identification, handling and measurements were undertaken by the author.
Sex of individuals was determined by cloacal examination with males having visible hemipenal sheaths, while eversion of hemipenes was routinely attempted on most species. Determination of sex was routine after 1992–1993, while accurate sex determination in hatchlings and juveniles became reliable only after 2009.
Size at maturity in females was determined from the SVL of females recorded as gravid and usually documented from the smallest reproductively active individual, unless that was a noticeable outlier to the distribution of ‘active size’ category. Gravid females were conservatively determined through abdominal palpation and/or observation through the ventral skin to determine if eggs or large follicles were present. In live-bearing taxa (Hemiergis, Cyclodomorphus, Tiliqua) embryos, if detected, were recorded. Maturity in males was conservatively determined from the smallest male extruding seminal (hemipenal) plugs during cloacal examination for sex determination.
Hatching time was determined from the demi-month of peak captures of the smallest individuals (SVL) in each species. This occurred in most reptiles from late summer to early autumn months – February to mid-March under Perth’s strongly seasonal Mediterranean-type climate. However, smallest SVLs of infrequently captured or live-bearing species often occurred outside that period and may not reflect actual hatching size or time.
Growth in early age cohorts was assessed from SVL measurements made on a demi-monthly basis. In uniquely marked and recaptured individuals, growth and age were accurately determined. However, growth and early age cohorts were inferred for other species from the increasing SVL in each species from mean demi-monthly measurements after peak hatching times. Age-year cohorts were determined for up to 2 years and occasionally 3 years, and were more accurately determined for abundantly captured species. Age categories were defined as ‘hatchlings’ during their first year and ‘juveniles’ until reproductive maturity was attained. Adult size was designated from the minimum SVL length at reproductive maturity and from inferred and assigned age-size categories in the years following hatching.
Analyses
Variation was inherent in all behavioural and demographic parameters examined on a demi-monthly basis over the 37 years study period. However, the intent of this study was to document broad seasonal patterns in parameters under Perth’s predictable Mediterranean climate. Between year differences will be evaluated in a subsequent publication to determine species specific responses to decadal change in environmental factors.
Size at reproductive maturity, growth parameters and longevity of individuals were evaluated over the entire 37 years. All metrics involving seasonal activity and reproductive parameters were constrained to the latter 30 years (i.e. Regime III – post September 1993) of standardised methodology when adult individuals were routinely categorised for sex and reproductive condition and closer examination was undertaken to document sex of hatchlings and yearlings up to 2 years of age.
Comparisons between SVL and mass in reproductive-status categories in adults of both sexes were tested for significance using a standard t-test.
Results
A total of 29 species of reptiles in eight families were captured in Bold Park during the study. Earlier analysis of this reptile assemblage (How et al. 2022) suggested that altered trap type and configuration between Regime II and Regime III potentially impacted assemblage structure during the different regimes. Consequently, they modelled relative abundance response to rainfall variation only for Regime III. The current study confirms variable responses of species to changed trapping design (Fig. 1). Five of the six most abundant taxa, and the great majority of the commonest 24 species, were captured more frequently during Regimes I and II. Those altered capture rates within the same species between different trapping regimes, necessitated that species activity be evaluated only with the consistent methodology of Regime III.
Capture rates (captures/100 trap days) of 24 common reptile species in Regimes I + II (hatched columns) and Regime III (solid columns) in Bold Park.
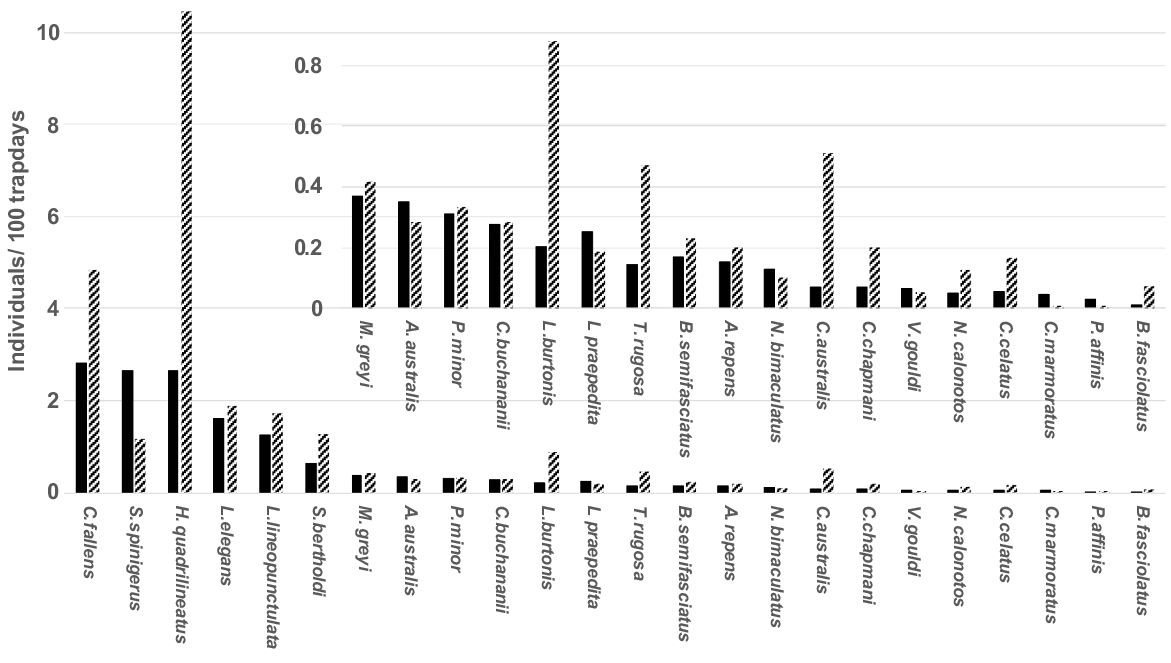
Activity
Over the 30 years of Regime III, 29 species were captured on 16,613 individual occasions (Table 1). During the first 7 years of Regimes I and II combined, 26 species were captured on 2477 individual occasions (from Table 2). The three species not captured during the earlier regimes were the snake, Echiopsis curta, the legless lizard, Pletholax gracilis and the gecko, Heteronotia binoei; the former two were first captured in February 1994 and November 1998, respectively, while the gecko was first captured in February 2016. Captures of H. binoei in every subsequent year indicates the species has been a recent coloniser that is now established in Bold Park.
Species FAMILY | Total no. | Smallest (mm) (Demi-month) | Hatchling (<1 year) Size (mm) | No. reproductively active (two smallest (mm)) | Adult minimum size (mm) | Inferred maturity (Indicative year) | Clutch range (No.) | ||||
---|---|---|---|---|---|---|---|---|---|---|---|
F | M | F | M | F | M | ||||||
Hemiergis quadrilineatus SCINCIDAE | 4056 | 23.5 (10B) | <43.5 | 235 (35.5, 42.5) | 4 (47,48.5) | ≥43.5 | ≥43 | Second year | Second year | 1–4 (43) | |
Ctenotus fallens SCINCIDAE | 3692 | 30.32 (2B) | <75.8 | 106 (80.5,82) | 89 (75,75.5) | ≥84 | ≥75 | Third year | Second year | 1–6 (8) | |
Strophurus spinigerus DIPLODACTYLIDAE | 3179 | 26 (3B) | <52 | 263 (56,58 × 2) | ≥60 | ≥57 | Third year | Third year | 1–2 (34) | ||
Lerista elegans SCINCIDAE | 2025 | 17.5 (1B) | <31.5 | 103 (33 × 2,35.5) | 115 (33, 34 × 4) | ≥35.5 | ≥34 | Third year | Second year | 1–4 (37) | |
Lerista lineopunctulata SCINCIDAE | 1630 | 35.5 (12B) | <54 | 162 (72,73.5) | 280 (72.5,73.5) | ≥74 | ≥74 | Third year | Third year | 1–5 (99) | |
Simoselaps bertholdi ELAPIDAE | 850 | 82 (2B) | <105 | 25 (193,201) | 39 (180 × 2,182) | ≥200 | ≥180 | Fifth +year | Third year | 2–4 (10) | |
Menetia greyii SCINCIDAE | 469 | 11 (1A) | <26 | 147 (23,26 × 2) | 0 | ≥25 | Second year | 2–3 (24) | |||
Anilios australis TYPHLOPODIDAE | 434 | 70 (2B) | <125 | 52 (145,157) | 3 (155,167) | ≥144 | ≥144 | Third year | Third year | 2–6 (27) | |
Pogona minor AGAMIDAE | 392 | 31 (2A) | <85 | 35 (79,85.5) | 7 (70.5,79) | ≥85 | ≥85 | First year | First year | 2–8 (12) | |
Cryptoblepharus buchananii SCINCIDAE | 344 | 19 (2B) | <37 | 49 (37,39 × 2) | 9 (36 × 2,39 × 2) | ≥37 | ≥37 | Second year | Second year | 1–3 (9) | |
Lialis burtonis PYGOPODIDAE | 319 | 54.5 (3A) | <120 | 64 (108,132) | 23 (120,125) | ≥140 | ≥120 | Third year | Second year | 1–2 (47) | |
Lerista praepedita SCINCIDAE | 310 | 32 (3A) | <48 | 24 (54.5,57) | 71 (49.5 × 2,50) | ≥54 | ≥54 | Second year | Second year | 1–3 (16) | |
Brachyurophis semifasciatus ELAPIDAE | 216 | 96 (11A) | <185 | 25 (185,255) | 11 (242,245) | ≥250 | ≥235 | Fifth +year | Fourth year | 1–6 (17) | |
Tiliqua rugosa SCINCIDAE | 211 | 160 (12A) | <240 | 23 (270 × 2,275 × 2) | ≥270 | Second +year | |||||
Aprasia repens PYGOPODIDAE | 198 | 48 (3A) | <64 | 10 (84,95) | 23 (77,78 × 2) | ≥84 | ≥75 | Fourth year | Second year | 1–2 (4) | |
Narophis bimaculatus ELAPIDAE | 157 | 135 (12A) | <185 | 13 (195,205) | 8 (245,285 × 3) | ≥240 | ≥240 | Fourth year | Fourth year | 2–6 (9) | |
Ctenotus australis SCINCIDAE | 132 | 40.5 (2A) | <80 | 1 (96.5) | 2 (93,94) | ≥90 | ≥90 | Third+ year | Third+ year | ||
Ctenophorus chapmani AGAMIDAE | 101 | 26 (2B) | <42 | 2 (46 × 2) | 1 (42) | ≥46 | ≥42 | Second year | First year | ||
Varanus gouldii VARANIDAE | 79 | 95 (12B) | |||||||||
Cyclodomorphus celatus SCINCIDAE | 74 | 38.5 (2A) | <50 | 2 (92,104) | 13 (80,82) | ≥92 | ≥80 | Fourth year | Third year | ||
Neelaps calonotos ELAPIDAE | 74 | 95 (2B) | <140 | ||||||||
Christinus marmoratus GEKKONIDAE | 53 | 25 (3B) | 3 (52,57) | 2–3 (3) | |||||||
Pseudonaja affinis ELAPIDAE | 37 | 260 (3A) | |||||||||
Brachyurophis fasciolatus ELAPIDAE | 18 | 152 (3B) | |||||||||
Heteronotia binoei GEKKONIDAE | 16 | 40 (2A) | 1 (50) | 2 (1) | |||||||
Morethia obscura SCINCIDAE | 13 | 31 (2B) | 3 (42.5,46 × 2) | ||||||||
Lucasium alboguttatum DIPLODACTYLIDAE | 6 | 40.5 (10B) | |||||||||
Pletholax gracilis PYGOPODIDAE | 3 | 61 (11A) | |||||||||
Echiopsis curta ELAPIDAE | 2 | 245 (2A) |
Total numbers captured during study, smallest individual and demi-month of capture, hatchling size at the end of their first year, number reproductively active and their smallest sizes, minimum adult size, inferred maturity based on indicative age-size categories and clutch number and range.
F, females; M, males.
The six most abundant species comprised 80.6% of captures in Regime III and 24 species, generally captured over numerous years, comprised 99.7% of total captures (Table 1). The capture rates of those 24 species were averaged over annual demi-monthly periods for the 30 annual sampling periods in Regime III (Fig. 2), illustrating the annual activity profile for the total reptile assemblage.
Demi-monthly capture rates, expressed as captures/100 trap days (mean + s.e.), averaged for all species in the Bold Park assemblage during the 30 trapping seasons of Regime III.
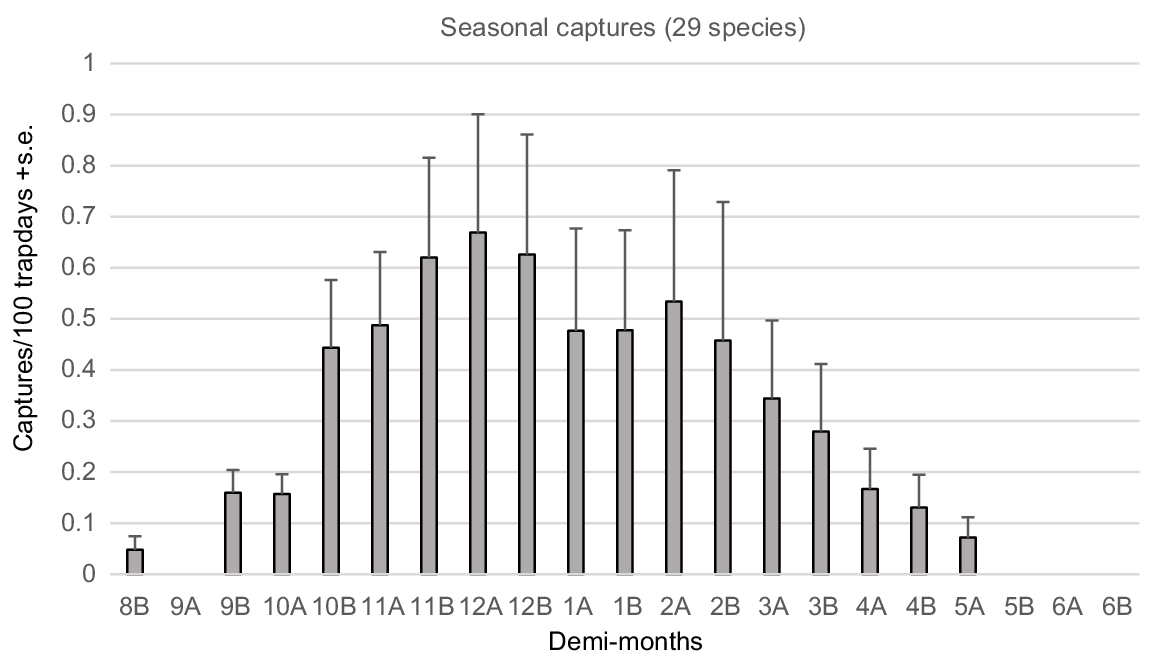
Highest activity within the reptile assemblage occurs during the spring and summer months between late October and late December (Fig. 2). However, large standard errors associated with mean demi-monthly capture rate indicates significant variation exists between the 24 species regularly trapped. That variance is best explained by variable emergence times of species’ hatchlings (Fig. 3), generally occurring from late January to late March, and different seasonal activity patterns within adults.
Activity patterns for adults and hatchlings (<1 year) in 16 species, expressed as captures/100 trap days (left axis), over an annual demi-monthly timescale (females, solid line; males, dashed line). Hatchling activity over their first year (grey columns), also expressed as captures/100 trap days (right axis), over the same annual demi-monthly timescale. Menetia greyii is functionally parthenogenetic.
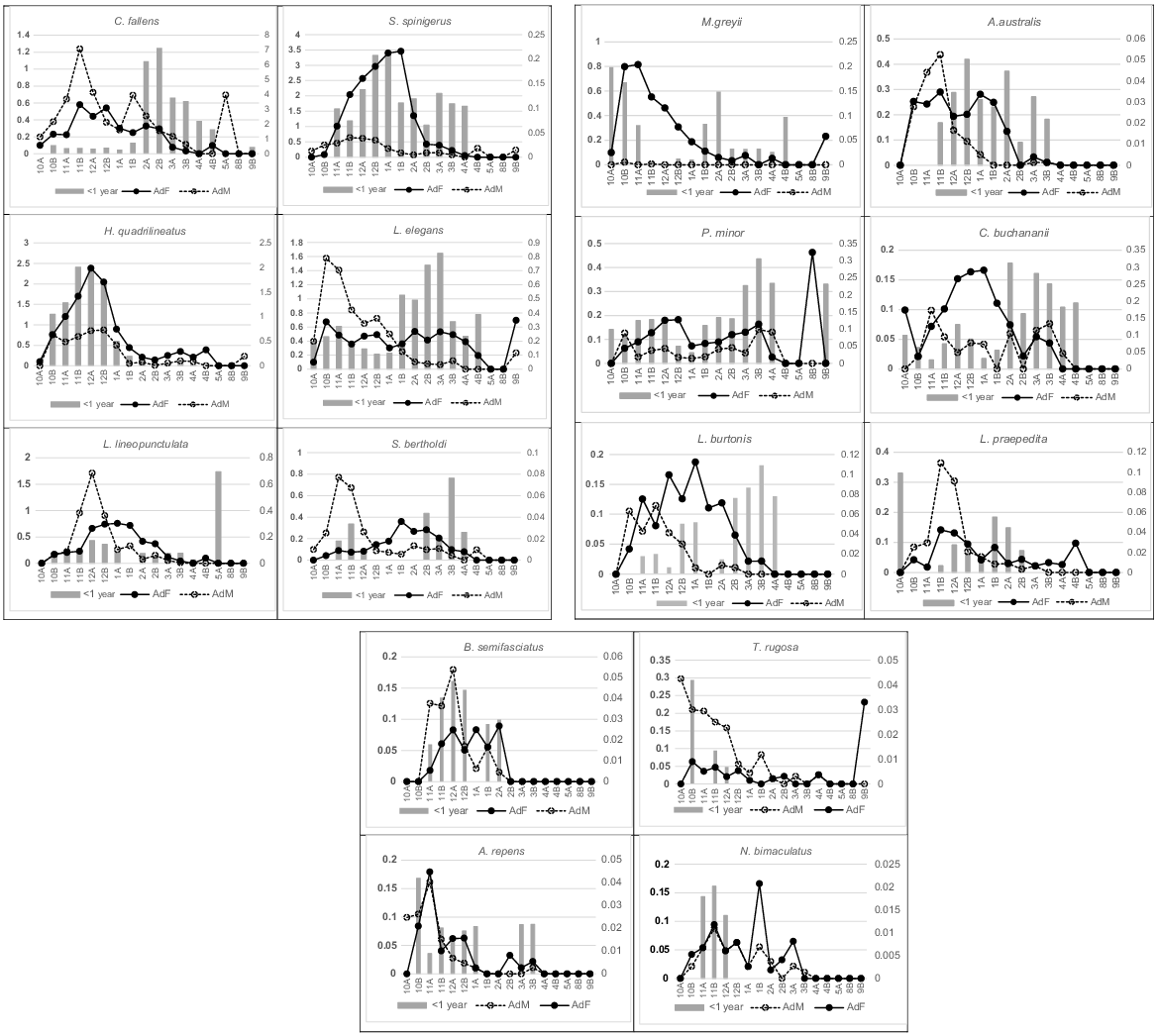
The five additional species that contribute to the total assemblage, were the recent colonist, H. binoei, the rare habitat specialist, P. gracilis, and three species that have become locally extinct over the course of the study. The snake, E. curta, the ground gecko, Lucasium alboguttatum and the fossorial snake Brachyurophis fasciolatus have not been captured for the past 29, 27 and 23 years, respectively. Additionally, P. gracilis and the heath dragon, Ctenophorus chapmani, have only been captured once during the past 20 years, making them likely contenders to become the next local extinctions. Two species, the snake, Pseudonaja affinis, and monitor, Varanus gouldii, have large adult individuals, not amenable to pitfall capture, and were poorly represented as only their hatchlings and juveniles were captured.
The six commonest species strongly influence activity pattern in the total assemblage.
Emergence of hatchlings occurred between the end of January to early March in Ctenotus fallens, Strophurus spinigerus, Lerista elegans and Simoselaps bertholdi (Fig. 3). Hatchlings first appear in December for Lerista lineopunctulata and live young may be born before winter in Hemiergis quadrilineatus but are mostly captured during the following late October and November (see Table 2). Few hatchlings of S. spinigerus were caught in autumn but many more during the spring and summer months of their first year. The late summer early autumn emergence of hatchlings appears to be a consistent pattern for most of the 16 species with adequate data that account for 97% of all captures (Fig. 3).
Adults also show variable activity patterns but, almost universally, there is a marked decline over the hotter months from mid-January and through the hatching season of February and March. Adult female C. fallens were active slightly later in the spring than adult males; however, both had similar spring-dominated abundances then showed a marked decline in activity from early January onwards through summer and into autumn. Both adult sexes in S. spinigerus had similar activity patterns although adult females greatly outnumber adult males in all demi-months and decreased activity later than males. The decline in H. quadrilineatus adult activity from early January was marked in both adult sexes, despite fewer males recorded than females. The decline in female activity occurred when they are carrying late-stage clutches. Adults in the two fossorial Lerista species shared similar peak activity patterns in spring and early summer with males active slightly earlier than females and females remaining active longer (Fig. 3). Males of S. bertholdi were more active from November to early December than females, the latter peaking during January–February after depositing eggs; a pattern previously documented by How and Shine (1999) for this population. Patterns of adult activity amongst these commonest species were broadly mirrored in adults of the remaining species in Fig. 3 that had peak activity between November and early January but slightly contrasting patterns over those demi-months.
Species demographics
Growth, inferred size-age (based on SVL) categories, individual longevity and maximum movement were evaluated over species’ complete capture record covering 37 years (Regime I to III). However, reproductive seasonality and size and mass of adults was evaluated only over the 30 years of Regime III when mandatory documentation of sex and reproductive status occurred.
Size-age categories were appraised from the time of peak demi-month emergence of hatchlings for all species and their subsequent size (SVL, mm) changes in following demi-monthly periods. Examination of those plots illustrated inferred age of cohorts at the end of each sampling (yearly) period and the size attained by species by the end of their first (hatchling), second and, occasionally, third years. Infrequently captured species, resulting from either their rarity (L. alboguttatum, P. gracilis) or trap size limitations (V. gouldii, P. affinis), had insufficient data for age category assessment. Viviparous species (H. quadrilineatus, Tiliqua rugosa, Cyclodomorphus celatus) had young at the end of autumn and juveniles that generally appeared in the trap record in early spring (Table 2).
C. fallens and Pogona minor, two regularly trapped species with uniquely marked individuals, had growth patterns determined from recaptured, known-aged individuals. Both sexes in C. fallens showed similar patterns of growth over demi-months through their first, second and third years and, despite size dimorphism between adults (see Supplementary material Table S1), there was no clear indication from known-age individuals when that dimorphism commenced (Fig. 4). Growth patterns in both C. fallens and P. minor hatchlings and yearlings indicated continuing increases in size and mass between late autumn and early spring over both their first and second years, despite a complete absence of capture-activity throughout that period.
Growth patterns for Ctenotus fallens (above) and Pogona minor (below) for demi-months over successive years determined from mark–recaptured individuals first caught during their hatchling and first years. SVL (mm) (lines; left axis) and mass (g) (columns; right axis) for females (filled + s.d.) and males (open −s.d.). Hatchlings (year 0) are followed by age in years (digits).
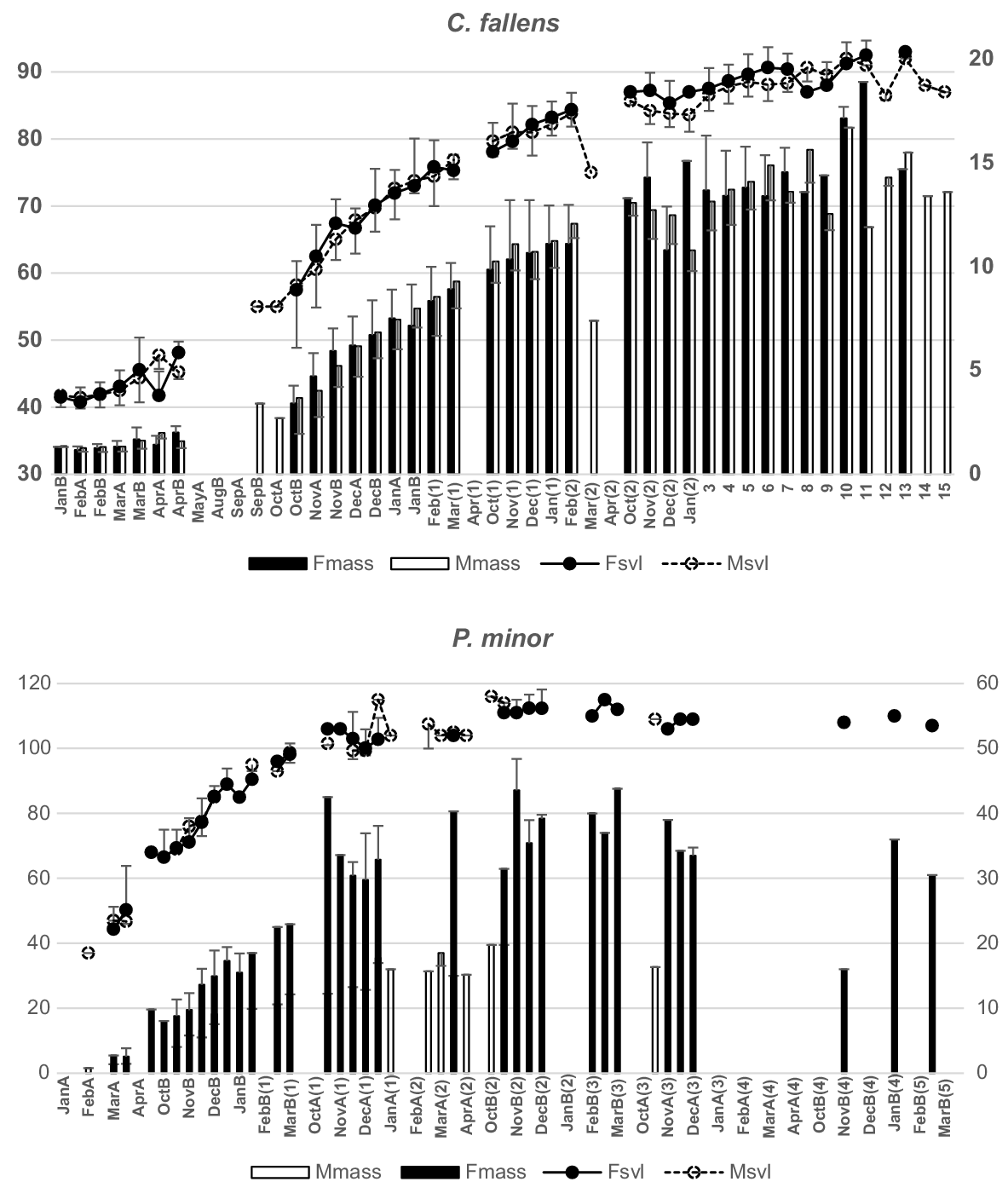
Only 26 individual hatchling S. spinigerus individuals were captured over their first autumn and just 149 within their hatchling year. Of those, only nine were recaptured in later months, insufficient to allow known-age growth rates to be determined. P. minor, Cryptoblepharus buchananii, Ctenotus australis and C. chapmani individuals did not show clear evidence of sex dimorphism in growth over their early years, while both T. rugosa and C. celatus individuals were too infrequently recaptured to determine growth pattern from size-age categories.
The size at maturity (adults) was evaluated from the combination of the minimum size of individuals illustrating reproductive activity and size determined growth category over demi-monthly periods. The smallest reproductively active individuals are documented (Table 2). However, occasionally those individuals were ‘outliers’ in a species maturity-size distribution and probably unlikely to represent routine onset of maturity for that species.
Age at reproductive maturity varied greatly between species and occasionally between sexes. The agamids, P. minor and C. chapmani, matured by the end of their first year as did some male C. fallens. However, it appears that in most species both sexes do not become reproductively active until the end of their second or usually, in their third years. In snake species (S. bertholdi, Brachyurophis semifasciatus, Narophis bimaculatus) reproductive activity commenced a year or two later than the lizard species, based on their size-age category (Table 2).
Reproductive pattern was conservatively determined from the proportion of adult individuals in each demi-month (Fig. 5) having either yolky follicles or eggs (females) or seminal plugs (males).
Numbers in 18 species of adult females (filled columns) and adult males (open columns) captured during each demi-month (left axis) and the percentage of females gravid (solid lines) and males reproductively active (dotted line) during those periods (right axis). Male H. quadrilineatus and S. spinigerus were not categorised for reproductive status.
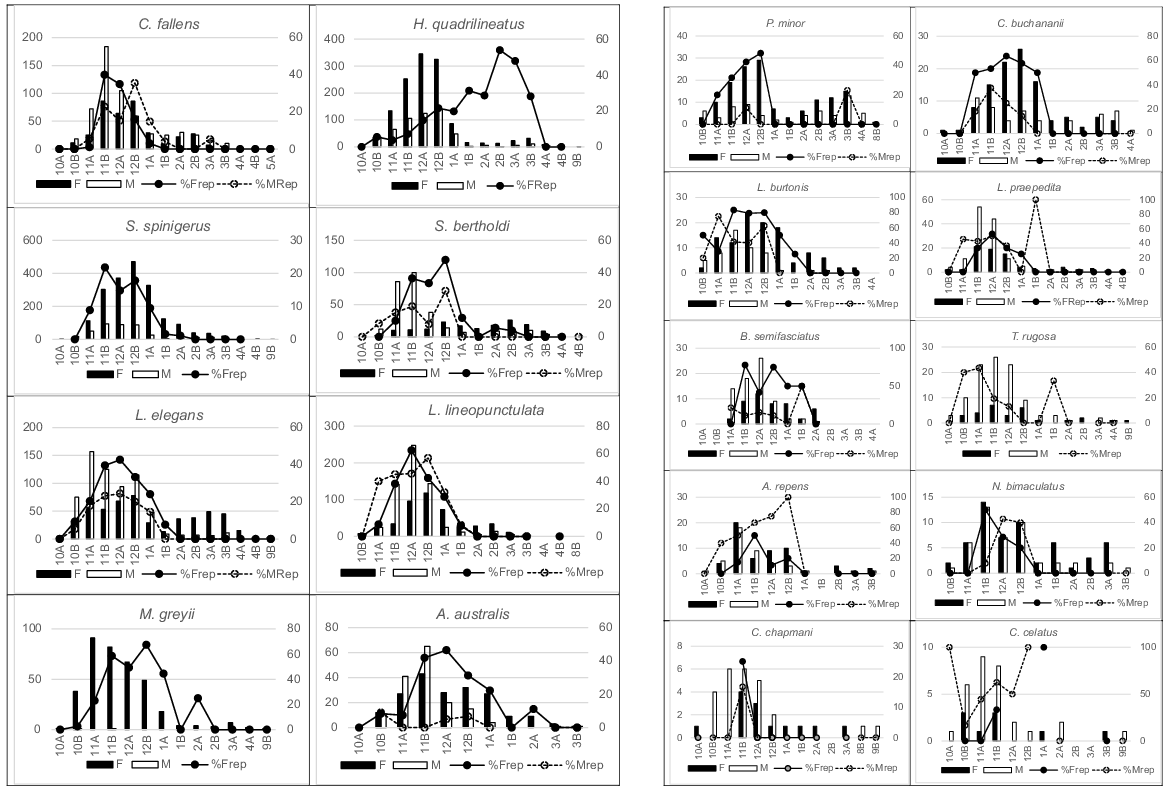
Reproduction occurred, almost universally, from late spring to early summer months in the 18 most abundant species (Fig. 5). Information for other species suggested a similar pattern despite limited sample sizes and/or temporal coverage. The viviparous species, H. quadrilineatus, T. rugosa and C. celatus, produced young at the end of autumn but determination of reproductively active or gravid females in those species was difficult given the robust opaqueness of the ventral scales.
The upper range in clutch size recorded (Table 2) indicated the likely maximum individual fecundity in Bold Park species populations. One or two eggs are only ever produced by all gecko and legless lizard species, consequently that represents the maximum clutch size in those taxonomic groups; no evidence for double clutching during a year was recorded in marked S. spinigerus individuals. The maximum clutch recorded in skinks, agamids and all snakes varied considerably but could reflect the observational inconsistencies mentioned in the Material and Methods section. For the nine species, where 10 or more clutches were counted (Table 2), larger females carried larger clutches, notably in H. quadrilineatus, L. lineopunctulata, Anilios australis, C. fallens and N. bimaculatus. In C. fallens clutches of six were recorded in the largest females, but many large gravid individuals had only two to four eggs, probably reflecting egg deposition prior to examination.
Captures of hatchlings, based on their demi-monthly SVL classes, inferred that high mortality occurs during the first year in all species (Fig. 3), specifically during autumn and winter following hatching. Few individuals survive to the yearling category. For marked individuals of C. fallens and P. minor, the low survival of hatchlings was illustrated by lower captures and few marked individuals reaching spring with continuing low captures through the following year. In S. spinigerus, very few hatchlings were captured before winter and their capture rates diminished rapidly over the following spring and summer. In other species, survival of hatchlings also appeared low with decreasing capture rates over autumn and few surviving to spring.
Indicative longevity (Table 3), determined from the longest period between recaptures of uniquely identified individuals (measured in sampling years), illustrated large variation between species. Most re-trapped individuals occurred within their hatchling year, but numerous survived for several years afterwards to infer a longevity greater than a decade for two viviparous (T. rugosa, C. celatus) and two oviparous (C. fallens, C. buchananii) skink species as well as the oviparous gecko, S. spinigerus (Table 2). Another oviparous skink, C. australis, was documented to live at least 6 years, an estimate probably curtailed by the rapid decline of the species population over the study period. Both oviparous agamid species, P. minor and C. chapmani, were relatively short-lived with only one female P. minor known to survive 4 years (Table 3). These estimates of longevity are conservative as they do not consider size-age category at first capture.
Species | Sampling seasons (years) between recaptures | Total | |||||||||||||||||||||||
---|---|---|---|---|---|---|---|---|---|---|---|---|---|---|---|---|---|---|---|---|---|---|---|---|---|
0 | 1 | 2 | 3 | 4 | 5 | 6 | 7 | 8 | 9 | 10 | 11 | 12 | 13 | 14 | 15 | 16 | 17 | 18 | 19 | 20 | 21 | 22 | |||
Ctenotus fallens | 221 | 100 | 55 | 42 | 25 | 17 | 6 | 3 | 7 | 3 | – | – | 2 | – | – | 1 | – | – | – | – | – | – | – | 482 | |
Strophurus spinigerus | 211 | 131 | 46 | 22 | 20 | 8 | 8 | 8 | 4 | – | 1 | 3 | – | 2 | – | – | – | – | 1 | – | – | – | 1 | 466 | |
Pogona minor | 64 | 11 | 5 | 3 | 1 | – | – | – | – | – | – | – | – | – | – | – | – | – | – | – | – | – | – | 84 | |
Cryptoblepharus buchananii | 10 | 5 | 1 | – | 1 | – | 1 | 1 | 1 | 2 | 1 | – | – | – | 1 | – | 1 | – | – | – | – | – | – | 25 | |
Tiliqua rugosa | 3 | 1 | 2 | 8 | 1 | 2 | 1 | 2 | 1 | 3 | 1 | 1 | – | 1 | – | 1 | 2 | – | 1 | 1 | – | 1 | – | 33 | |
Ctenotus australis | 4 | 5 | 3 | 2 | 1 | – | 1 | – | – | – | – | – | – | – | – | – | – | – | – | – | – | – | – | 16 | |
Ctenophorus chapmani | 13 | 3 | 1 | – | – | – | – | – | – | – | – | – | – | – | – | – | – | – | – | – | – | – | – | 17 | |
Cyclodomorphus celatus | 1 | 1 | – | 2 | – | – | – | – | – | – | – | – | – | – | 1 | – | – | – | – | – | – | – | – | 5 |
Most recaptures of uniquely marked individuals occurred within traps at the same sampling site and year as their previous capture (Table 3), particularly among hatchlings. Those trap-indicated movements within the same site restrict the maximum distance moved between recaptures to less than 43 m.
Some individuals of all marked species traversed between different sampling sites throughout Bold Park. In ‘straight line’ distances these movements varied from 150 m to 1.7 km (Table 4), indicating individuals may move extensively through the study area. The skinks C. fallens and T. rugosa had recorded movements between all sites, while the gecko, S. spinigerus, had recorded movements almost exclusively between sites BP1, BP5 and BP3, sites dominated by proteaceous heath understoreys. The arboreal skink, C. buchananii, had recorded movements only between the Banksia and Tuart woodland sites of BP3 and BP4, respectively.
Between sites (BP) | 2–3 | 2–4 | 3–4 | 1–5 | 2–5 | 3–5 | 4–5 | 1–2 | 1–4 | 1–3 | Total | Mean ± s.d. | |
---|---|---|---|---|---|---|---|---|---|---|---|---|---|
Distance (m) | 150 | 280 | 400 | 750 | 1410 | 1450 | 1565 | 1565 | 1610 | 1665 | |||
Ctenotus fallens | 3 | 1 | 3 | 7 | 4 | 2 | 2 | 10 | 32 | 1126 ± 567 | |||
Strophurus spinigerus | 11 | 4 | 1 | 6 | 22 | 1164 ± 420 | |||||||
Pogona minor | 1 | 1 | 2 | 1207 ± 458 | |||||||||
Cryptoblepharus buchananii | 4 | 4 | 400 ± 0 | ||||||||||
Tiliqua rugosa | 2 | 2 | 1 | 4 | 1 | 2 | 12 | 1414 ± 308 | |||||
Ctenotus australis | 1 | 1 | 2 | 1615 ± 50 | |||||||||
Ctenophorus chapmani | 1 | 1 | 2 | 1180 ± 430 | |||||||||
Cyclodomorphus celatus | 1 | 1 | 750 |
Direct-line distances between sites, number of recorded movements and average inter-site distance in metres (mean ± s.d.) moved for each species.
Most movements between sites were recorded over one to several sampling years. However, one adult C. fallens moved 150 m between sites BP3 and BP2 over a period of 15 days, while two hatchlings moved 1665 m between sites BP1 and BP3 and 1565 m between sites BP5 and BP4 over periods of 7 and 5 days, respectively. A gravid female S. spinigerus moved between sites BP1, BP3 and BP5 over a 28-day period, distances of 1665 m and 1450 m, while another adult female moved 750 m between site BP1 and BP5 over 5 days.
The mass (g) and size (SVL, mm) of adult females and males in different reproductive categories for the dominant 18 species are in Table S1. Sample sizes were limited in the other less frequently captured species.
Gravid females in all species were significantly heavier (P < 0.01) than other adult females determined as not reproductively active. Similarly significant differences in female length occurred, except for C. buchananii, B. semifasciatus and N. bimaculatus. Reproductively active males were of similar mass to other males, except for being significantly lighter (P < 0.05) in A. australis and significantly heavier in L. elegans and Lialis burtonis (both P < 0.05), and L. lineopunctulata (P < 0.01). Lengths of adult males were significantly longer in L. lineopunctulata (P < 0.01) and N. bimaculatus (P < 0.05) but significantly shorter (P < 0.01) in A. australis for adults determined as not reproductively active. Female T. rugosa were not reliably categorised for reproductive condition and no seminal plugs were recorded from any adult male S. spinigerus and only four in H. quadrilineatus. Two Menetia greyii males were captured, a skink known to have parthenogenic populations.
Sexual dimorphism in length was significantly greater (P < 0.01) in non-gravid females than non-reproductive males in all species, except M. greyii, P. minor and T. rugosa. Dimorphism in mass was also significant greater (P < 0.01) for adult females, except in C. fallens, M. greyii (only two males captured), P. minor, C. buchananii, and T. rugosa, and significantly less (P < 0.01) in H. quadrilineatus. Only four male H. quadrilineatus extruded seminal plugs, which likely accounts for non-reproductive males being significantly heavier than their female counterparts.
The extensive SVL range in adults in several species suggests extended individual longevity, since many reptiles show continuous growth with age. In most species individuals were not uniquely marked, and therefore not permitting accurate documentation of longevity. However, two exceptionally large female A. australis (SVL 360 mm) were captured in Tuart woodland at BP4, and a large female B. fasciatus (SVL, 390 mm) in Banksia woodland at BP3; those measurements were greater than 2 s.d. above the means for adults of their sex.
Discussion
Species activity
This study focused on seasonal activity and demographic parameters of reptile species that are numerically dominant by combining response patterns from a long-term capture-release program of in situ populations experiencing a predictable Mediterranean climate. A previous study (How et al. 2022) implicated changeable rainfall pattern across south-western Australia as a key correlate in determining total reptile abundance and suggested variation in reproductive and survival patterns of common species would account for lagged response documented in total numbers; that variation resulting from altered resource states under variable annual rainfall.
Adult individuals are key to defining behavioural and demographic parameters of populations, including variation in activity, fecundity, size and mass that determine species performance through time and over space. During the 30-year period of standard methodology for Bold Park, adult male activity in most species preceded that of adult females during the spring-summer reproductive period. A further generalisation was a marked decline in adult activity from January to April that preceded an almost complete cessation of activity over the late autumn and winter; indicating a late summer aestivation period as well as supporting known brumation in reptiles from late autumn through winter. Activity of hatchlings and juveniles within the study populations, however, showed different species-specific seasonal patterns.
Evaluating the ecological traits of the five fossorial elapid snakes occurring in Bold Park, How and Shine (1999) also reported overall low abundance and reduced activity through mid-summer, as well as winter, and noted male S. bertholdi were more active in spring, during the mating season, while females were more active in autumn, after oviposition. That broad pattern was also reflected by B. semifasciatus and the blind snake, A. australis, in this study, but in N. bimaculatus seasonal activity was similar in both adult sexes. Two other fossorial snakes (B. fasciolatus, Neelaps calonotos) had limited adult data to interpret general patterns, although increased activity was noted in N. calonotos during both late autumn and early spring.
Comprehending seasonal variation in activity patterns of age cohorts in species provides insights into their longer-term demographic performance, probability of detection and potential for local extinction.
Three issues are inherent in detectability – variable demographic factors and activity responses of populations though time as well as suitability of capture methods employed. Those issues are particularly relevant within the isolated reptile populations in Bold Park.
Several species had unreliable detectability using pitfall trapping due to their large adult sizes (P. affinis, V. gouldii) or their potential climbing abilities (Christinus marmoratus, C. buchananii). Additionally, marked changes in annual activity were also noted in capture rates of S. spinigerus and H. quadrilineatus throughout the study (How et al. 2022) while variable seasonal activity patterns of species hatchlings and adults also impacts detectability, particularly if sampling duration is constrained to certain times of the year.
The great majority of species in Bold Park showed altered capture rates between Regime III and Regime I + II combined, necessitating caution in interpreting activity and relative abundance patterns even when using only slightly different pitfall trapping methodologies. The methodological study by Bamford and Calver (2015), contrasting pitfall trapping with active foraging through habitat destruction on the SCP, showed few species were equally detectable using those differing techniques. Environmental impact assessments are mandated by regulatory authorities for development projects and require use of various methodologies to focus on biotic richness, particularly threatened species (Western Australian Government 2018; Thompson and Thompson 2020). Inherent in those assessments is an understanding of seasonal variation within individual species activity that would impact both the design and temporal sequence of biodiversity surveys. This is relevant to Guidance Statements, Technical guidance and Standard Operating Procedures proposed by regulatory authorities (Thompson and Thompson 2024).
While acknowledging methodological differences between pitfall trapping studies, comparison of species activity could still provide evidence of regional differences in relative abundance of otherwise cryptic species. Pitfall trapping of four fossorial snake species, in common between this study and in WA deserts (Goodyear and Pianka 2008), indicated that higher activity (captures/100 pit trap days) occurred in Bold Park for S. bertholdi (0.46 vs 0.21) and N. bimaculatus (0.13 vs 0.05), similar activity in B. semifasciatus (0.17 vs 0.17) but lower activity in B. fasciolatus (0.01 vs 0.07), a species now locally extinct in isolated Bold Park.
Species demography
Many studies of population parameters in WA reptiles have been generated either from the dissection of individuals removed from the population (Davidge 1980; Pianka 1986) that potentially impacts in situ demographic parameters, or from museum specimens (Chapman and Dell 1985; How et al. 1986; Strahan et al. 1998) that cover large spatial and broad temporal scales that likely obfuscates variation. Fewer studies involve demographic parameters from in situ individuals of naturally occurring populations under known environmental conditions (Bradshaw 1971; Baverstock 1975; Kitchener et al. 1988; Sarre 1995, 1998). The review of reptile studies in WA by Aplin and How (1993) supported the proposal by Bradshaw (1986) that field studies of species demography, in both space and time, should be a focus for future herpetofaunal research, particularly given the rapid expansion of agriculture, forestry, mining and urbanisation in the south-west of the State.
Size is crucial to defining reptile size-age demographic categories and essential to determining parameters in naturally occurring populations over extended timeframes – despite recent studies showing that indeterminate growth is not universal among ectothermic reptiles (Frýdlová et al. 2020). Growth patterns were determined for both C. fallens and P. minor from marked, known-aged hatchlings and juveniles that were followed through subsequent demi-months or monthly intervals for up to 3 years. There was no indication of variable growth rates in P. minor hatchlings or juveniles as has been documented for the saxiphilous agamid, Ctenophorus ornatus, that occurs on granite outcrops adjacent to Perth (Baverstock 1975). Growth in both C. fallens and P. minor hatchlings and yearlings increased in size and mass between captures in late autumn and early spring, providing a conundrum because that is a period of supposed brumation and during which there was a complete absence of capture-activity.
However, in most species size-age categories were inferred from changes in SVL over demi-months after peak hatchling emergence and then through juvenile categories for their first 2 years, and occasionally 3 years. Growth was maximal during those early-age cohorts and pulses in size classes were traced to provide inferred age categories and probable age at reproductive maturity.
Reproduction occurred between late spring and early summer in both lizards and snakes while hatching, or birth, of young occurred from late summer to early autumn. Spring reproduction and hatchling emergence in the late autumn for snakes accords with earlier findings, determined through dissected specimens (Shine 1984, Strahan et al. 1998; Goodyear and Pianka 2008), while the reproductive parameters in lizards was in broad agreement with other populations in the Mediterranean climate of the WA wheatbelt region (Chapman and Dell 1985) and the SCP (Davidge 1980). Informatively, Bradshaw (1981) indicated that in some lizard species there was variation in reproductive onset, with breeding commencing earlier in more northerly latitudes. Reproductive parameters in two arboreal gecko species, Gehyra variegata and Hesperoedura reticulata, in the WA wheatbelt region (Kitchener et al. 1988) had fixed clutch sizes of one and two eggs, respectively, in spring but the former reached sexual maturity in its third, while the latter only reproduced after their fifth year.
Age at maturity, based on size-age categories and assuming continuous growth, varied between most lizards and snakes, although it was generally acquired between 2 and 4 years of age. Marked P. minor individuals achieved sexual maturity toward the end of their first year, as did some male C. fallens, but in the latter both male and female maturity usually occurred a year later. The granite rock-inhabiting dragon, C. ornatus, in localities nearby to Perth (Baverstock 1975, 1978), was relatively short-lived and had a high fecundity with populations being either slow or fast growing and age at sexual maturity occurring in less than 1 year for the fast growers and between 1.5 years and 2 years for slow growers.
Survival of hatchlings appeared low in all species in Bold Park with few individuals reaching spring of their hatchling year and even fewer the yearling age category, as inferred from capture rates within size-age classes over those periods. For two species with marked individuals, low survival of hatchlings was informed by few surviving to spring and through to the following year. High mortality among hatchling and juveniles has been documented for both gecko species studied by Kitchener et al. (1988) in the wheatbelt region of WA, although other studies of natural populations seldom address low survival in early-age cohorts. Adult survival in Bold Park was more difficult to assess due to infrequent recaptures of marked individuals and their potential for movement throughout the larger study area.
Longevity was determined only for species with uniquely marked individuals. Several of those species had individuals that survived for over a decade and longevity reached at least 22 years in S. spinigerus. Extensive longevity was recorded for the arboreal geckos G. variegata and H. reticulata, (How and Kitchener 1983; Kitchener et al. 1988) and a continuation of that study showed individual Gehyra survived at least 13 years and Hesperoedura at least 18 years (J. Dell, unpubl. data); both individuals being adult when first captured. Agamids have relatively short longevity with individuals surviving for only a few years (Bradshaw 1971; Baverstock 1975), such as both Bold Park agamids, P. minor and C. chapmani.
Movement of individuals occurred regularly within trapping sites and most recaptures occurred there. However, movements by marked individuals of several species of nearly 2 km occurred between trapping sites, suggesting they utilise the entire contiguous study area of 338 ha. In contrast, two arboreal geckos studied in the wheatbelt region of WA showed exceptionally strong site fidelity; in the case of H. reticulata some individuals were confined to a single tree for the entirety of their capture record over 18 years (J. Dell, pers. comm.).
Reproductively active females were larger and heavier than their non-reproductive counterparts, lending support to the observation that larger females are probably more fecund. Adult males were rarely different between their reproductive categories. The comparison of non-reproductively active categories between the sexes supports sexual dimorphism in size and mass with females being larger and heavier than males for most species. However, the reverse situation occurred in H. quadrilineatus, a probable consequence of very few males extruding plugs that potentially reflects sperm storage in that live-bearing taxon, as previously demonstrated in its congener Hemiergis peronii (Smyth and Smith 1968).
Populations of the same reptile species on individual islands of the Houtman Abrolhos archipelago, isolated for the past 6K years and also experiencing a Mediterranean climate, showed significant differences in adult size (How et al. 2020). They postulated that differing relative abundances and adult size in populations probably reflected divergent resource states on islands of differing size and biological heterogeneity. Resource states were also indicated as the principal driver of different population abundances in G. variegata populations across vegetated isolates of the WA wheatbelt (Sarre 1998).
Demographic considerations
Knowledge of activity and demographic parameters are fundamental to understanding and interpreting a species population performance; particularly among species within isolates where presence does not necessarily equate to persistence. Many vertebrate taxa in south-western Australia are exceptionally long lived, have long generation times, and remain on isolates long after populations are no longer viable, with a consequence that many species may still disappear (Saunders et al. 1991). Small populations of long-lived species also comprise the most likely candidates for defining the extinction debt inherent in any anthropogenically created isolate (Hanski and Ovaskainen 2002).
The population time series appraisal by White (2019) indicated that none of the life history traits examined (generation time, litter size, adult mass, maximum longevity or incubation) in over 540 bird species population studied were significant predictors of minimum time required to accurately document a long-term population trend. That review suggested using a minimum threshold time was unwise and, consequently, implied that species specific knowledge was essential.
Storr et al. (1978b) indicated that reptile species persisted on the SCP for 150 years of European colonisation without known losses, despite the many associated anthropogenic perturbations that initiated regional extinctions in many mammals and several bird species. The bushland remnants remaining on the SCP, however, showed a strong positive relationship between isolate area and reptile species richness (How and Dell 2000); a relationship that was highly significant for snakes and non-skink lizards but non-significant for skink taxa that can persist in very small isolates for long periods of time after fragmentation. None of those remnants retained all reptile species known to occur on the SCP, giving support to zoogeographic partitioning of species aligned to variable geomorphological elements of the Plain.
In Bold Park, the largest of the inner urban Perth bushland remnants, three relatively recent local extinctions have occurred, and another two species are unrecorded for over a decade; all are snakes or non-skink lizards with initially low or decreasing relative abundances. Those latter two species, P. gracilis and C. chapmani, have declined markedly during the study period and are potential candidates for local extinction. The former is a semi-arboreal legless lizard preferring dense proteaceous shrub layers for movement and occupied an isolated patch of heath not sampled by this survey (W. G. Jiang, unpubl. data) the latter is a short-lived agamid showing large pulses in relative abundances during the earlier years of the study. These observations of the reptiles in Bold Park suggest that numerous additional local extinctions are probable and indicate that an extinction debt is still inherent in the assemblage studied (How et al. 2022). In the wheatbelt region of south-western Australia, numerous local extinctions among reptiles were inferred from the regional surveys of small remnant vegetation reserves isolated for less than 100 years (Kitchener et al. 1980b; Smith et al. 1996).
The significance of understanding eco-physiological and population life-history parameters to interpreting species responses under different seasonal or climatic conditions has been shown to occur elsewhere in south-western WA. Different growth parameters in populations of the rock-dragon (C. ornatus), correlated with predictability of environmental conditions, highlights the demographic plasticity inherent in a species occupying a highly specialised habitat (Bradshaw 1971; Baverstock 1975). In the nocturnal and arboreal gecko, H. reticulata, body temperatures were higher than ambient temperatures between 23°C and 16°C but were the same below 16°C, when activity became greatly reduced. These physiologically induced behavioural shifts influenced activity patterns both during the night and over different seasons (How and Kitchener 1983) directly affecting detection of individuals within the population.
Short-term studies may document numerical changes in populations but are unlikely to measure changes in demographic parameters that underpin those numerical changes. This study’s initial objectives were to evaluate population persistence and habitat preferences of reptiles occupying a biodiverse inner urban bushland isolate (How 1998), its extended duration has also allowed decadal variation in reptile relative abundance to be documented and likely factors influencing that variation to be proposed (How et al. 2022). However, an understanding in situ species activity patterns, breeding success and survival in different age cohorts are central to interpreting population numbers through time, particularly in a reptile assemblage experiencing a marked decline in rainfall and increase in temperatures (BoM 2022) within its Mediterranean climate.
Data availability
This study remains a work in progress. The data analysed for the current publication is available on request from the author.
Declaration of funding
Equipment was funded by the Western Australian Museum with all travel costs provided by the author.
Acknowledgements
Permission to research in Bold Park and assistance was initially provided by Perth City Council, then Town of Cambridge and latterly the Botanic Gardens and Parks Authority. Peter Luff and Barry Connell generously shared their extensive local knowledge and experience of Bold Park during early years. Several Bushland Managers, Customer Service Officers and staff from Botanic Gardens and Parks Authority along with numerous colleagues assisted the program, while my family was exceptional in understanding and supporting the field program over decades. The project was supported logistically by the Western Australian Museum and its directors, particularly John Bannister and Paddy Berry. John Dell provided unique natural history insights over decades of collaborative work while Roy Teale, Mark Cowan, Mike Calver and reviewers made comments that improved an earlier draft. The Western Australian Museum, most recently, operated under DBCA Collecting Permits FO25000006-26e to collect fauna while this project was granted ethics approval through the DBCA Animal Ethics Committee (License to Use Animals for Scientific Purposes No. U18/2006).
References
Bamford MJ, Calver MC (2015) A comparison of measures of abundance of reptiles in Kwongan vegetation of the South-West of Australia, determined through systematic searching and pitfall trapping. Australian Zoologist 37, 472-484.
| Crossref | Google Scholar |
Baverstock PR (1975) Effect of variations in rate of growth on physiological parameters in the lizard Amphibolurus ornatus. Comparative Biochemistry and Physiology Part A: Physiology 51, 619-631.
| Crossref | Google Scholar |
Baverstock PR (1978) The probable basis of the relationship between growth rate and winter mortality in the lizard Amphibolurus ornatus (Agamidae). Oecologia 37, 101-107.
| Crossref | Google Scholar | PubMed |
Beard JS, Chapman AR, Gioia P (2000) Species richness and endemism in the Western Australian flora. Journal of Biogeography 27, 1257-1268.
| Crossref | Google Scholar |
Bradshaw SD (1971) Growth and mortality in a field population of Amphibolurus lizards exposed to seasonal cold and aridity. Journal of Zoology 165, 1-25.
| Crossref | Google Scholar |
Chapman A, Dell J (1985) Biology and zoogeography of the amphibians and reptiles of the Western Australian wheatbelt. Records of Western Australian Museum 12, 1-46.
| Google Scholar |
Clutton-Brock T, Sheldon BC (2010) Individuals and populations: the role of long-term, individual-based studies of animals in ecology and evolutionary biology. Trends in Ecology & Evolution 25, 562-573.
| Crossref | Google Scholar | PubMed |
Davidge C (1979) A census of a community of small terrestrial vertebrates. Australian Journal of Ecology 4, 165-170.
| Crossref | Google Scholar |
Davidge C (1980) Reproduction in the herpetofaunal community of a Banksia woodland near Perth, W.A. Australian Journal of Zoology 28, 435-443.
| Crossref | Google Scholar |
Davis RA, Gole C, Roberts JD (2013) Impacts of urbanisation on the native avifauna of Perth, Western Australia. Urban Ecosystems 16, 427-452.
| Crossref | Google Scholar |
Frýdlová P, Mrzílková J, Šeremeta M, Křemen J, Dudák J, Žemlička J, Minnich B, Kverková K, Němec P, Zach P, Frynta D (2020) Determinate growth is predominant and likely ancestral in squamate reptiles. Proceedings of the Royal Society B: Biological Sciences 287, 20202737.
| Crossref | Google Scholar |
Gaston KJ, Fuller RA (2008) Commonness, population depletion and conservation biology. Trends in Ecology & Evolution 23, 14-19.
| Crossref | Google Scholar | PubMed |
Goodyear SE, Pianka ER (2008) Sympatric ecology of five species of fossorial snakes (Elapidae) in Western Australia. Journal of Herpetology 42, 279-285.
| Crossref | Google Scholar |
Hanski I, Ovaskainen O (2002) Extinction debt at extinction threshold. Conservation Biology 16, 666-673.
| Crossref | Google Scholar |
Hero J-M, Castley J, Malone M, Lawson B, Magnusson W (2010) Long-term ecological research in Australia: innovative approaches for future benefits. Australian Zoologist 35, 216-228.
| Crossref | Google Scholar |
Hopper SD, Gioia P (2004) The southwest Australian floristic region: evolution and conservation of a global hot spot of biodiversity. Annual Review of Ecology, Evolution, and Systematics 35, 623-650.
| Crossref | Google Scholar |
How RA (1998) Long-term sampling of a herpetofaunal assemblage on an isolated urban bushland remnant, Bold Park, Perth. Journal of the Royal Society of Western Australia 81, 143-148.
| Google Scholar |
How RA, Dell J (1990) Vertebrate fauna of Bold Park. Western Australian Naturalist 18, 122-131.
| Google Scholar |
How RA, Dell J (1994) The zoogeographic significance of urban bushland remnants to reptiles in the Perth region, Western Australia. Pacific Conservation Biology 1, 132-140.
| Crossref | Google Scholar |
How RA, Dell J (2000) Ground vertebrate fauna of Perth’s vegetation remnants: impact of 170 years of urbanization. Pacific Conservation Biology 6, 198-217.
| Crossref | Google Scholar |
How RA, Kitchener DJ (1983) The biology of the gecko Oedura reticulata Bustard, in a small habitat isolate in the Western Australian wheatbelt. Australian Wildlife Research 10, 543-556.
| Crossref | Google Scholar |
How RA, Shine R (1999) Ecological traits and conservation biology of five fossorial ‘sand-swimming’ snake species (Simoselaps: Elapidae) in south-western Australia. Journal of Zoology 249, 269-282.
| Crossref | Google Scholar |
How RA, Dell J, Wellington BD (1986) Comparative biology of eight species of Diplodactylus gecko in Western Australia. Herpetolgica 42, 471-482.
| Google Scholar |
How RA, Cowan MA, Teale RJ, Schmitt LH (2020) Environmental correlates of reptile variation on the Houtman Abrolhos archipelago, eastern Indian Ocean. Journal of Biogeography 47, 2017-2028.
| Crossref | Google Scholar |
How RA, Cowan MA, How JR (2022) Decadal abundance patterns in an isolated urban reptile assemblage: monitoring under a changing climate. Ecology and Evolution 12, e9081.
| Crossref | Google Scholar |
IUCN (2012) IUCN red list categories and criteria. Version 3.1. Available at https://www.iucn.org/resources/publication/iucn-red-list-categories-and-criteria-version-31
Keighery GJ, Harvey J, Keighery BJ (1990) Vegetation and flora of Bold Park, Perth. Western Australian. Naturalist 18, 100-121.
| Google Scholar |
Kitchener DJ, Chapman A, Dell J, Muir BG, Palmer M (1980a) Lizard assemblage and reserve size and structure in the Western Australian wheatbelt – some implications for conservation. Biological Conservation 17, 25-62.
| Crossref | Google Scholar |
Kitchener DJ, Chapman A, Muir BG, Palmer M (1980b) The conservation value for mammals of reserves in the western Australian wheatbelt. Biological Conservation 18, 179-207.
| Crossref | Google Scholar |
Kitchener DJ, Dell J, Muir BG, Palmer M (1982) Birds in Western Australian Wheatbelt reserves – implications for conservation. Biological Conservation 22, 127-163.
| Crossref | Google Scholar |
Kitchener DJ, How RA, Dell J (1988) Biology of Oedura reticulata and Gehyra variegata (Gekkonidae) in an isolated woodland of Western Australia. Journal of Herpetology 22, 401-412.
| Crossref | Google Scholar |
Lamont BB, Downes S, Fox JED (1977) Importance–value curves and diversity indices applied to a species-rich heathland in Western Australia. Nature 265, 438-441.
| Crossref | Google Scholar |
Li Y, Hopkins AJM, Davis RA (2023) Going, going, gone the diminishing capacity of museum specimen collections to address global change research: a case study on urban reptiles. Animals 13, 1078.
| Crossref | Google Scholar | PubMed |
Lindenmayer DB, Likens GE, Andersen A, Bowman D, Bull CM, Burns E, Dickman CR, Hoffman AA, Keith DA, Liddell MJ, Lowe AJ, Metcalf DJ, Phinn SR, Russell-Smith J, Thurgate N, Wardle GM (2012) Value of long-term ecological studies. Austral Ecology 37, 745-757.
| Crossref | Google Scholar |
Magurran AE, Baillie SR, Buckland ST, Dick JMP, Elston DA, Scott EM, Smith RI, Somerfield PJ, Watt AD (2010) Long-term datasets in biodiversity research and monitoring: assessing change in ecological communities through time. Trends in Ecology & Evolution 25, 574-582.
| Crossref | Google Scholar | PubMed |
Myers N (2003) Biodiversity hotspots revisited. BioScience 53, 916-917.
| Crossref | Google Scholar |
Myers N, Mittermeier RA, Mittermeier CG, da Fonseca GAB, Kent J (2000) Biodiversity hotspots for conservation priorities. Nature 403, 853-858.
| Crossref | Google Scholar | PubMed |
Pianka ER, Pianka HD (1976) Comparative ecology of twelve species of nocturnal lizards (Gekkonidae) in the Western Australian deserts. Copeia 1976, 125-142.
| Crossref | Google Scholar |
Recher HF, Serventy DL (1991) Long term changes in the relative abundances of birds in Kings Park, Perth, Western Australia. Conservation Biology 5, 90-102.
| Crossref | Google Scholar |
Sarre S (1995) Size and structure of populations of Oedura reticulata (Reptilia: Gekkonidae) in woodland remnants: implications for the future regional distribution of a currently common species. Australian Journal of Ecology 20, 288-298.
| Crossref | Google Scholar |
Sarre SD (1998) Demographics and population persistence of Gehyra variegata (Gekkonidae) following habitat fragmentation. Journal of Herpetology 32, 153-162.
| Crossref | Google Scholar |
Saunders DA, Hobbs RJ, Margules CR (1991) Biological consequences of ecosystem fragmentation: a review. Conservation Biology 5, 18-32.
| Crossref | Google Scholar |
Shine R (1984) Ecology of small fossorial Australian snakes of the genera Neelaps and Simoselaps (Serpentes, Elapidae). In ‘Vertebrate ecology and systematics; a tribute to Henry S. Fitch’. (Eds RA Seigel, LE Hunt, L Malaret, NL Zuschlag) pp. 173–183. (Mus. Nat. Hist., Univ. Kansas. Spec. Publ.: Kansas)
Smith GT, Arnold GW, Sarre S, Abensperg-Traun M, Steven DE (1996) The effect of habitat fragmentation and livestock grazing on animal communities in remnants of gimlet Eucalyptus salubris Woodland in the Western Australian Wheatbelt. II. Lizards. Journal of Applied Ecology 33, 1302-1310.
| Crossref | Google Scholar |
Smyth M, Smith MJ (1968) Obligatory sperm storage in the skink Hemiergis peronii. Science 161, 575-576.
| Crossref | Google Scholar | PubMed |
Storr GM, Harold G, Barron G (1978b) Amphibians and reptiles of the Northern Swan Coastal Plain. In ‘Faunal studies of the Northern Swan Coastal Plain – a consideration of past and future changes’. pp. 172–203. (Western Australian Museum Report to Department of Conservation & Environment: WA, Perth)
Strahan N, How RA, Dell J (1998) Reproduction and diet in four species of burrowing snakes (Simoselaps spp.) from southwestern Western Australia. Records Western Australian Museum 19, 57-63.
| Google Scholar |
Thompson GG, Thompson SA (2020) A comparison of an environmental impact assessment (EIA) vertebrate fauna survey with a post-approval fauna salvage program: consequences of not adhering to EIA survey guidelines, a Western Australian example. Pacific Conservation Biology 26, 412-419.
| Crossref | Google Scholar |
Thompson GG, Thompson SA (2024) Compliance is not necessarily good science. The Environmental Protection Authority’s vertebrate fauna survey guidelines should be revised. Pacific Conservation Biology 30, PC23040.
| Crossref | Google Scholar |
Western Australian Museum (2023) Checklist of the Terrestrial Vertebrate Fauna of Western Australia. Available at https://museum.wa.gov.au/research/departments/terrestrial-zoology/checklist-terrestrial-vertebrate-fauna-western-australia
White ER (2019) Minimum time required to detect population trends: the need for long-term monitoring programs. BioScience 69, 40-46.
| Crossref | Google Scholar |