Effects of prolonged starvation on body mass and respiratory physiology of the New Zealand abalone or pāua, Haliotis iris
Islay D. Marsden
A
Abstract
Subtidal, adult pāua experience irregular food supply. The effects of prolonged starvation have not been examined.
To examine effects of 105-day starvation on body mass, respiration and haemolymph proteins.
Pāua, maintained at 15°C, were divided into groups that were either fed Macrocystis pyrifera, or starved; each group was further divided between pāua whose haemolymph was sampled at intervals and those sampled only at the beginning and end of the experimental period. Body mass, condition, oxygen uptake and gonad index were measured at the start and end.
Total mass of both fed and starved groups did not change significantly. The condition of the starved individuals decreased significantly. Haemocyanin accounts almost entirely for haemolymph proteins. The haemolymph haemocyanin concentration in fed abalone fell by 17–27% over 105 days, and by 49–63% in starved individuals. Final normoxic oxygen uptake was similar in starved and fed pāua and declined similarly during hypoxia.
Pāua may survive extended periods of starvation by metabolising body tissues and haemocyanin, with only minor changes in their mass, condition and aerobic respiration.
These primitive gastropods have mechanisms to survive stressors associated with low food availability. Maintenance of body volume in this osmoconformer supports gill perfusion and the functioning of the hydraulic skeleton in locomotion.
Keywords: abalone starvation, haemocyanin, haemolymph protein, Haliotis iris, hypoxia, New Zealand abalone, oxygen uptake, pāua.
Introduction
The black-foot pāua (Haliotis iris) is endemic to New Zealand. It is the largest of three New Zealand species of Haliotidae, and is the main commercial abalone species. Adults of this long-lived species are found in shallow pools in intertidal rocky reef areas and, subtidally, down to 10 m below low-water springs (Poore 1972a, 1972b, 1973). In these habitats, they can be exposed to disturbances caused by anthropogenic activities, long- and short-term seasonal changes and natural events such as changes in shore levels following seismic events (Travers et al. 2008; Gerrity et al. 2020). Aerial exposure, temperature extremes, lack of oxygen, salinity change and food availability all potentially affect the ability of pāua to survive and withstand these stressors (Ragg et al. 2000; Ragg and Watts 2015; Chapperon et al. 2019; Nguyen 2023; Nguyen et al. 2023; Ragg 2023).
Unlike fish, which can move to avoid detrimental environments, haliotids exhibit relatively restricted mobility and, therefore, must adjust their physiology and biochemistry to maintain basic biological activities (Calow and Sibly 1990; Carefoot et al. 1998). A lack of available energy potentially reduces growth and reproduction (Diaz and Rosenberg 1995; Morash and Alter 2016) and could compromise haliotid health by decreasing their immune functional capacity (Dang et al. 2012; Hooper et al. 2014).
Pāua and other haliotids readily feed on large macroalgae both in aquaculture and in the wild (Allen et al. 2006; Cook 2010). Growth rate in gastropod molluscs is related to food availability and quality (Lachambre et al. 2017) and when food is unavailable or limited, natural populations may be depleted. Starvation effects in molluscs have been recorded within 2 weeks in both freshwater and marine snails (Calow 1974; El-Eman and Madsen 1982; Nelson et al. 2016), and differ among species. Starvation effects include behavioural responses, including reduced mobility and physiological responses of the whole animal, tissues and haemolymph (Zhao et al. 2011).
The effects of limited food supply on health and survival of several adult haliotid species have been investigated by Carefoot et al. (1993) and Braid et al. (2005), and several studies have examined effects of diet on juvenile abalone (Durazo-Beltrán et al. 2004; Cho et al. 2011). Surprisingly few studies have examined growth responses in pāua. Stress effects of this species have been recorded following exposure to high wave action, handling and aerial exposure (Wells and Baldwin 1995; Ragg et al. 2000; Behrens et al. 2002; Donovan and Taylor 2008; Ragg and Watts 2015). There is little detailed information on the effects of long-term starvation on abalone. Carefoot et al. (1993) investigated the effects of starvation on Haliotis kamtschatkana over a period of 27 days; Rogers-Bennett et al. (2010) undertook a laboratory starvation experiment for 13 months in the red abalone Haliotis rufescens; and Braid et al. (2005) starved individuals of this species for over 250 days. These studies suggested that, despite their primitive form, abalone might be more tolerant of starvation than are some other gastropods, allowing survival in habitats where food supply is variable.
The present study was undertaken to examine the resilience to long-term starvation of pāua from a Banks Peninsula population at the mouth of Lyttelton Harbour. This population had been exposed to the Christchurch earthquake sequences in 2010–2012 and the morphology of the shell suggests that they are a stunted population. The objective was to establish whether these individuals, which may normally live in a stressful habitat, are able to survive an extended period of starvation exceeding 100 days. We investigated the effects of starvation on body mass, condition and gonad indices, respiration rate, and changes in haemocyanin and total protein concentrations in the haemolymph as potential indicators of pāua health. Such indicators may be useful in assessing the stress status of individuals in aquaculture facilities or to evaluate the recovery of populations such as those following the Kaikoura Earthquake series of 2014.
Materials and methods
Animals and treatments
Haliotis iris individuals of 105–265-g body mass (shell length 88–119 mm) were collected on 8 August 2017 from Little Port Cooper, Canterbury, New Zealand. The experimental design was limited to six individuals per experimental treatment, following guidance from the Animal Ethics Committee to minimise the number of individuals used in experiments on iconic New Zealand species. The distance between the collecting site and the laboratory was 29 km. After capture, pāua were transported in large chilly bins (i.e. insulated portable coolers) in seawater from the collection site. They were in the aquarium within 3 h of collection. They were acclimated for 2 weeks in a recirculating seawater system at 15°C. During this time, they were fed ad libitum with seaweed (giant kelp, Macrocystis pyrifera). This was selected because this kelp is common in the field where the animals were collected and we wanted to avoid a change in the usual diet. The shell was cleaned of encrusting organisms, and a small numbered tag was glued to the shell. At the start of the experiment, seven individuals (Group F0) were randomly selected to measure the initial rate of oxygen uptake, initial dry-weight condition index (CI) and initial gonad index (GI). Four treatment groups, each with five pāua of approximately similar size range, were then established in four separate 60 L tanks within the seawater system. There was one replicate tank for starved and one replicate tank for fed individuals. A few spare individuals were maintained in separate tanks in starved or fed conditions in case of initial mortalities. Individuals in Group ‘F4’ were continuously fed and, after 0, 35, 65 and 105 days, were weighed and haemolymph samples were taken. Group ‘S4’ was continuously starved and sampled at the same four times. Group ‘F2’ was continuously fed with samples taken only at the start and end of the 105-day experimental period to minimise possible effects of handling and haemolymph removal. Group ‘S2’ was continuously starved, with only initial and final samples. One pāua in the F4 group died shortly after the initial sampling and one animal in group S4 died just before the final (105-day) sample. The tanks were supplied with seawater from a recirculating system. They were drained every 4 days and the surface of the tanks was cleaned with a brush to minimise the establishment of a surface film. Oxygen uptake was measured on all individuals at the end of the experiment. Pāua were then were blotted dry and body cavities opened for measurements of weight and condition indices.
Oxygen uptake
Oxygen uptake was measured on individual pāua using closed-box measurements as described in Chandurvelan et al. (2015). Individuals were placed within specially designed circular respirometers filled with 1–3 L of fully aerated seawater, within a water bath at 15°C, and the taps were closed. Oxygen uptake was measured at 15-min intervals by removing a small sample of water and measuring oxygen concentrations by using aunicell containing a clark-type oxygen electrode (Strathkelvin 1302) with its own recirculating water system to maintain it at the required temperature. Values were read from an oxygen meter and recorded either on a chart record or manually. The respirometer remained closed for a period 3 h, or until the oxygen saturation levels had declined owing to removal of oxygen by respiration of the individual to ~20% of the starting value. Oxygen uptake was expressed as the shell-free dry weight-specific rate (mL g−1 h−1) calculated over time by using a straight-line relationship. For each individual, values were calculated at 100, 50 and 20% oxygen saturation.
Haemolymph sampling and weight measurements
The experiment commenced on 22 August 2017, at which time each animal was drained of external water (head down), total body mass (including the shell) was recorded, and a sample of haemolymph was taken for measurements of haemocyanin and protein concentrations. Groups F4 and S4 were again weighed and sampled after 35, 65 and 105 days, when the experiment was terminated. Groups F2 and S2 were left undisturbed after the initial measurements, until final measurements on Day 105.
Haemolymph samples of 1 mL were removed from the pedal sinus by using a syringe and 25-gauge needle inserted 0.5–1.0 cm into the foot in the midline ~1 cm behind the anterior V notch. The samples were centrifuged at 960g (20°C) for 15 min to remove blood cells. Haemocyanin and protein measurements were made on the supernatant within a few hours.
For measurement of the haemocyanin concentration in haemolymph samples, 200 μL of the supernatant was added, by using a positive displacement pipette, to 3 mL of buffer (10 mmol L−1 of EDTA, 50 mmol L−1 of glycine, pH 8.8, to dissociate haemocyanin into subunits) in a 1-cm2 polystyrene cuvette. After initial mixing by pumping the pipette, the contents were inverted several times by using a Parafilm cap, to ensure complete oxygenation of the haemocyanin. Absorbance at 346 nm was measured against a buffer blank by using a UV spectrophotometer (Unicam UV/Vis, Philips, Eindhoven, Netherlands) with a deuterium lamp, and haemocyanin concentrations (mmol L−1) in the undiluted samples were calculated using a practical extinction coefficient for haemocyanin functional units determined from copper analysis (EmM cm = 22.84; Behrens et al. 2002).
Samples with A346 of >0.5 were repeated at higher dilution (100 μL to 3 mL) to avoid non-linearity caused by light-scattering at 346 nm.
Protein concentrations were determined in haemolymph samples diluted in the same buffer (20 μL + 1 mL of buffer) in quartz micro-cuvettes from absorbance measurements at 280 nm. Concentrations were converted to milligrams per millilitre of bovine serum albumen (BSA) equivalent by using the extinction coefficient of E% cm = 6.67 (Pierce Biotechnology 2002).
Condition and gonad indices
At the end of the experimental period, the gonads, separated visually by colour differences from digestive gland, the other body tissues, and the shell were dried separately on aluminium foil at 60°C for 10 days. These measurements were used to calculate the dry-weight condition index (Eqn 3) and the gonad index (Eqn 4, %).
Statistical analysis
In Groups F4 and S4, values of body mass, and haemocyanin and protein concentrations at sequential sampling times were analysed using repeated measures ANOVA, with post hoc multiple comparison of means using Tukey’s HSD test (Statistica, ver. 8, Statsoft Inc., Tulsa, OK, USA, see http://www.statsoft.com). Differences between initial and final measurements in Groups F2 and S2 were examined using two-tailed paired Student’s t-tests (Excel 2019, ver. 2412 (Build 16.0.18324.20092) 32-bit, Microsoft Corp., Redmond, WA, USA). Animals that did not complete the full 105-day trial were excluded from the statistical analyses. Differences were considered significant at P < 0.05. Means ± standard deviation (s.d.) or ± standard error of the mean (s.e.m.) are cited, as indicated. Comparisons among and within groups for oxygen uptake, condition and gonad indices were made using one-way or two-way ANOVA.
Results
Survival
Of the 20 pāua individuals established initially, all except two survived the handling and sampling procedures, and were alive and mobile after 105 days. One animal in the S4 group died between the 65- and 105-day sample times.
Body mass
Pāua in all four groups maintained nearly constant mean total bodyweights (including their shells) throughout the 105-day experimental period (Fig. 1). The two fed groups (F4 and F2) gained weight slightly (but not significantly) by 3.1 and 2.2% respectively (F4, F3,12 = 1.429, P = 0.283, repeated-measures ANOVA; F2, P > 0.05, paired t-test); however, combining the two fed groups indicated a significant 2.5% weight gain between initial and final measurements (P < 0.05, t = −2.322, d.f. = 10, two-tailed paired t-test). Pāua in the starved groups (S4 and S2) lost weight (not significantly) by 4.5 and 0.9% respectively (S4, F3,9 = 0.872, P = 0.491, repeated-measures ANOVA; S2, P > 0.05, paired t-test). Any differences between treatments in this experiment may have been masked by low n values (Type 2 error).
Total wetmass (including shell, g ± s.e.m.) of pāua maintained in aquaria for 105 days. Treatment groups F4 and F2 were fed ad libitum with seaweed; S4 and S2 were unfed; 1-mL haemolymph samples were taken from F4 and S4 at 0, 35, 65 and 105 days; F2 and S2 were sampled only at 0 and 105 days. Same lower-case letters indicate lack of significant differences among means within a treatment group.
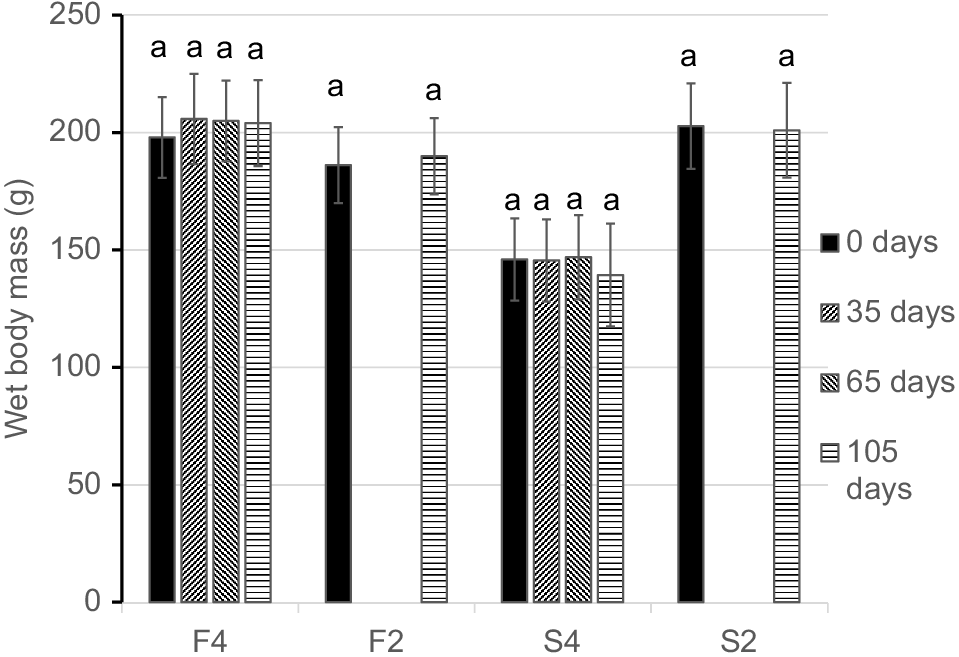
Condition index (CI)
At the start of the experiment, the mean CI of all pāua was 3.61 ± 0.36 (±s.e.m., n = 7) and this was similar to that of sampled and non-sampled fed groups at the end of the experimental period (F2,16 = 0.026, P = 0.97) (Fig. 2, top). Following starvation for 105 days, CI in sampled and non-sampled groups was similar (F1,19 = 0.178, P = 0.68) and significantly reduced compared with values for fed individuals at the end (F1,19 = 5.28, P = 0.0.03). There were no signs of atrophy in the tissues of starved individuals. The tissues of all individuals both starved and fed appeared healthy.
Gonad index (GI)
Mean GI was 13.29 ± 1.35 (±s.e.m., n = 7 at the start of the experiment, and was similar to final values for fed individuals sampled periodically (12.96 ± 17.45, n = 5), and those sampled only at the end (14.28 ± 12.32, n = 5) (Fig. 2, bottom). Corresponding values for starved individuals were (9.30 ± 2.44, n = 5 and 12.52 ± 13.00, n = 5). There were no significant differences in the GI due to feeding (F1,19 = 1.45, P = 0.24) or sampling (F1,19 = 1.03, P = 0.33). It is noted that the error bars were higher at the end of the experiment and potential differences may have been masked by low sample size, as described above.
Haemocyanin concentration
The haemocyanin concentration of the haemolymph of individual pāua was highly variable in all four treatment groups. The initial mean value for all groups was 0.317 ± 0.083 mmol L−1 (±s.d., n = 21), with a more than four-fold range (0.106–0.485 mmol L−1). Within individuals, in the fed and periodically sampled group (F4), haemocyanin concentration was nearly constant for 65 days, at a mean value of ~0.29 mmol L−1 (Fig. 3), and fell (non-significantly) by 27.2% after 105 days (repeated measures ANOVA, F3,12 = 2.607, P = 0.0998). In Group F2, a significant 17.1% decrease in haemocyanin concentration occurred over 105 days (P < 0.05, t = 4.449, d.f. = 3, two-tailed paired t-test).
Haemocyanin concentration (mmol L−1, ±s.e.m.) in haemolymph of pāua maintained in aquaria for 105 days. Treatment groups F4 and F2 were fed ad libitum with seaweed; S4 and S2 were unfed; 1-mL haemolymph samples were taken from F4 and S4 at 0, 35, 65 and 105 days; F2 and S2 were sampled only at 0 and 105 days. Different lower-case letters indicate significant differences between means within each treatment group (repeated-measures ANOVA and Tukey tests for F4 and S4; paired t-tests for F2 and S2).
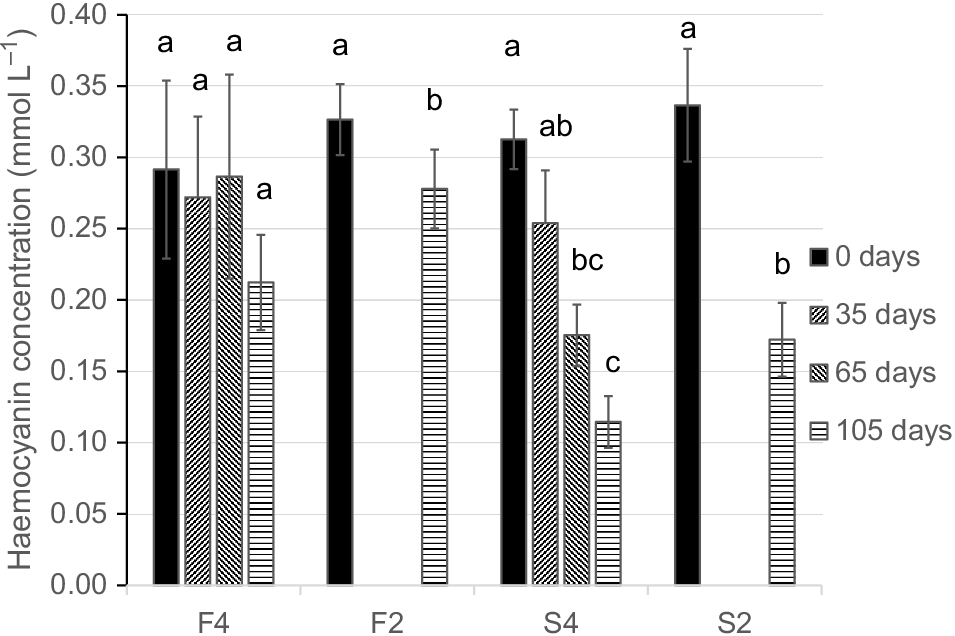
In the starved and repeatedly sampled group (S4), mean haemolymph haemocyanin concentration fell progressively by an overall 63% between the initial and 105-day samples in the four surviving pāua individuals, from 0.313 to 0.115 mmol L−1 (F3,9 = 17.532, P < 0.001, repeated-measures ANOVA); in the five pāua individuals sampled at 65 days, mean haemocyanin concentration fell from 0.312 to 0.183 (F4,8 = 15.125, P < 0.01). A large and significant 49% fall in haemolymph haemocyanin concentration, from 0.337 to 0.172 mmol L−1, was also observed in Group S2 (P < 0.001, t = 9.577, d.f. = 4, two-tailed paired t-test).
Protein concentration
Changes in the protein concentration of the haemolymph during the experiment closely mirrored changes in haemocyanin concentrations and were similarly variable among individual pāua. The initial mean value for all groups was 38.9 ± 10.1 mg mL−1 (±s.d., n = 21, range 13.5–56.7 mg mL−1) BSA equivalent. Individual protein concentrations measured in the haemolymph (Y, mg mL−1) were highly correlated with the measured haemocyanin concentrations (X, mmol L−1) (linear regression: Eqn 5). The low intercept indicates that proteins other than haemocyanin comprised a very minor fraction of the total (Fig. 4).
where R2 = 0.98, P = 1.71 × 10−52 and n = 61.
Relationship between haemocyanin (Hcy) and protein (BSA, bovine serum albumen) concentrations for all haemolymph samples taken from fed and starved pāua.
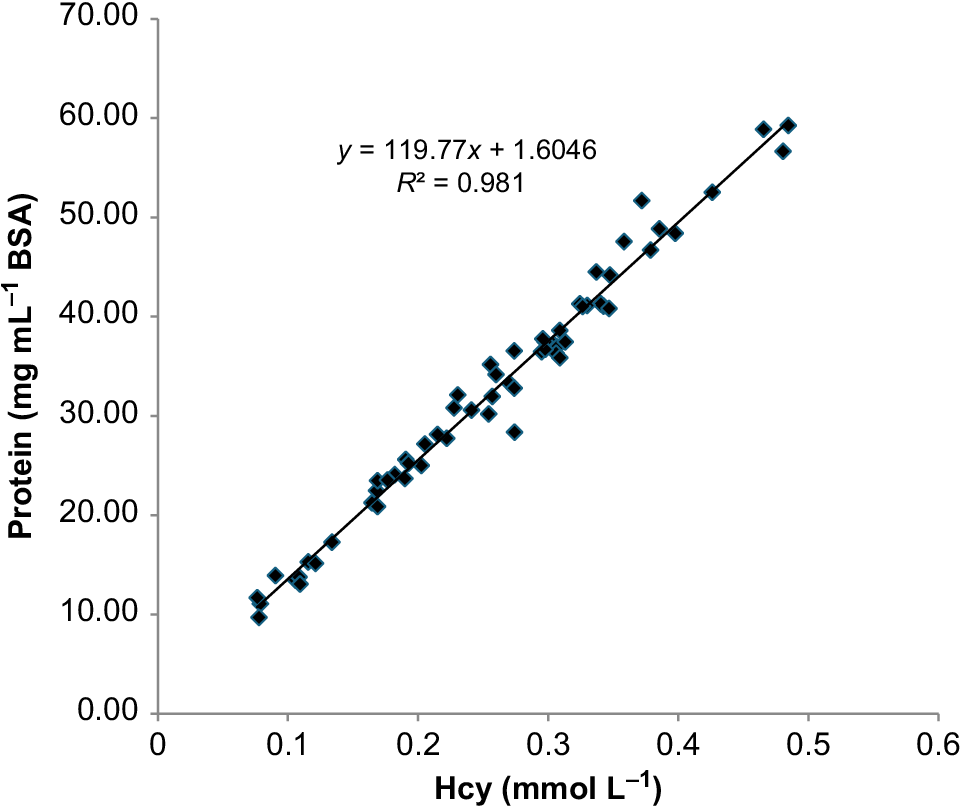
As for haemocyanin, individual haemolymph protein concentration in the fed group (F4) remained nearly constant for 65 days, at a mean value of ~36 g L−1, and fell (non-significantly) by ~27% after 105 days (repeated-measures ANOVA, F3,12 = 2.781, P = 0.0866). In the fed Group F2, a significant 11.5% decrease in protein concentration occurred over 105 days (P < 0.05, t = 3.314, d.f. = 3, two-tailed paired t-test).
In the starved and repeatedly sampled group (S4), mean haemolymph protein concentration fell progressively by an overall 61% between the initial and 105-day samples, from 38.3 to 14.9 mg mL−1 (F3,9 = 16.607, P < 0.001, repeated-measures ANOVA). A large and significant 45% fall in mean haemolymph protein concentration, from 41.0 to 22.6 mg mL−1, was also observed in Group S2 (P < 0.001, t = 9.670, d.f. = 4, two-tailed paired t-test).
Oxygen uptake
In the seven pāua individuals measured before the start of the experiment, normoxic oxygen uptake (mean ± s.e.) was a 100.1 ± 20.6 μL g−1 h−1 and significantly declined with a decreasing oxygen partial pressure in the respirometers to values of 21.3 ± 9.6 μL g−1 h−1 at 20% saturation (Fig. 5). There was no obvious plateau of respiratory independence at higher oxygen partial pressures. After 105 days in the seawater system, the rate of oxygen consumption had decreased to 84.8 ± 7.8 μL g−1 h−1 in fed and 81.6 ± 18.8 in starved groups. Values for pāua sampled periodically (F4, S4) were similar to those sampled only at the end of the experiment (F2, S2) and so these were combined to compare fed and starved animals at selected saturation levels. Oxygen uptake was similar for both groups at 100% saturation (F1,17 = 0.28, P = 0.86), 50% saturation (F1,17 = 2.40, P = 0.141) and 20% saturation (F1,17 = 0.26, P = 0.66).
Discussion
Pāua belong to an ancient and morphologically conservative molluscan group. They are adapted to cold, highly oxygenated waters, reaching the largest size and greatest abundance in the southern part of the South Island of New Zealand (Behrens et al. 2002; Naylor et al. 2006). Our study supports previous research suggesting that these gastropods are reasonably tolerant of environmental stressors (Ragg and Watts 2015; Ragg 2023), including restriction of their food supply.
Mass and condition
At 15°C, the pāua in our experiments maintained essentially constant mean total body mass for 105 days in a recirculating seawater system (Fig. 1). Although the two fed groups gained mass overall and the starved pāua lost mass, these changes were small and not significant. Maintenance of total body mass, despite haemolymph sampling and starvation, and the observed decreases in dry mass and haemocyanin concentration, discussed below, suggest that regulation of water balance and body volume was prioritised, which is presumably important for maintenance of gill perfusion (Ragg and Taylor 2006a, 2006b) and for the functioning of the hydraulic skeleton in locomotion (Trueman and Brown 1985; Thompson et al. 1998; Taylor and Ragg 2005). Regulation of body volume is a function of the excretory system (Taylor and Ragg 2005).
Adult pāua, like other haliotids, show seasonal patterns of tissue weight gain and loss in the field related to their breeding and growth cycles, and to temperature and food availability (Poore 1972a, 1972b, 1973; Uki and Kikuchi 1975; Shin et al. 2020). Our values of ~3.5 for the dry-weight condition index (CI) and ~13–14% for the gonad index (GI) of pāua collected from Banks Peninsula are generally below those found in other winter-collected individuals from the more northern Kaikoura coastline (Alestra et al. 2018).
After 105 days, the CI was essentially unchanged in both groups of fed pāua but was significantly lower in individuals both starved and serially haemolymph, suggesting that this extra handling was not an additional stress factor. This effect was further illustrated by a significantly lower GI by approximately one-third in the starved and sampled group. Over the observation period, fed pāua (F2, F4) and the unsampled starved pāua (S2) maintained relative gonad weight at levels similar to those at the start of the experiment. This suggests that, during starvation, tissue weights and gonad development were maintained, implying a selective allocation of resources from general body growth toward reproduction. Occurring at a relatively low seawater temperature of 15°C in our experiments is consistent with H. iris being a cold-adapted species with a lower temperature range than for some other haliotids, including H. midae (Barkai and Griffiths 1988) and H. rufescens (Dı́az et al. 2000). Rogers-Bennett et al. (2010) examined the effects of warm temperatures, starvation and disease on the reproduction of red abalone, H. rufescens. Males and females differed in their responses. They also concluded that starvation had a greater effect on egg production than did warm temperatures. This study has highlighted the potential interactive effects of natural stressors in the wild.
Respiration
Gastropods vary in their ability to maintain constant oxygen uptake under different environmental conditions. Most show adaptation to seasonal temperature change (Leung et al. 2021) and some maintain rates of oxygen uptake during short-term daily changes in temperature. Many gastropods survive starvation for several weeks at low environmental temperatures and this often results in a lowered rate of oxygen consumption (Nelson et al. 2016). In contrast, in the present study, prolonged starvation did not reduce the rate of oxygen consumption of pāua under normoxic or hypoxic conditions (Fig. 5).
Hypoxia is considered stressful for gastropods that live in highly oxygenated waters and this has been well documented for haliotids in aquaculture (Harris et al. 1999; Morash and Alter 2016). Slow-moving gastropods such as haliotids generally reduce rates of oxygen uptake when oxygen availability is lowered (Gaty and Wilson 1986), which is consistent with the present observations. Interestingly, although the oxygen uptake rates of pāua decreased with a declining oxygen partial pressure in the respirometers, there was no difference between the rates measured in starved and fed individuals. In contrast, some gastropods are able to maintain aerobic rates of oxygen uptake during hypoxia (Marsden et al. 2012). Nevertheless, pāua can withstand short periods of severe internal hypoxia by switching to anaerobic respiration while clamped down onto the substrate following disturbance. Despite their primitive structure and respiratory levels, pāua appear well adapted to survive short periods of hypoxia in the wild.
Effect of starvation on haemolymph haemocyanin and protein concentrations
Initial haemolymph concentrations of the oxygen-transporting protein haemocyanin in pāua varied more than four-fold, which is consistent with other reports of very high variability in field populations of haliotids, among different species and among individuals of the same species (Pilson 1965; Ainslie 1980). Haemocyanin concentrations present in pāua at the start of the experiment were similar to those reported in other studies (Behrens et al. 2002; Ragg and Taylor 2006b), including values for winter-acclimatised pāua (Nguyen et al. 2023), and also following 4 weeks of acclimation to 12°C (Nguyen 2023). The strong correlation between haemocyanin and protein concentrations, and the low value of the regression intercept indicated that proteins other than haemocyanin represent very minor constituents of pāua haemolymph.
Carefoot et al. (1993), when investigating the effects of starvation in Haliotis kamtschatkana, found depletion of glycogen reserves in the digestive gland and reduction of haemolymph glucose to half of the control value after 6 days, and glycogen depletion in the foot after 27 days. As in the present study, oxygen uptake was unaffected by starvation. After 105 days without food, approximately half of the haemocyanin was lost from the haemolymph (Fig. 3), accompanied by loss of tissue dry mass, as indicated by the change in CI (Fig. 2). Although the turnover time of haemocyanin is unknown, it is possible that resource-limited abalones were unable to maintain production. Haemocyanin is a valuable metalloprotein and it would be interesting to determine whether apohaemocyanin or copper were conserved for re-mobilisation when food became available again. However, the close relationship between haemocyanin and total protein concentrations in the haemolymph (Fig. 4) suggests that it was not stored in the haemolymph. Possibly, haemocyanin was simply catabolised for energy along with other tissues, when energy reserves were depleted. Because pāua possess a very large haemolymph volume (Haliotis iris, 56.6 mL per 100 g of soft tissues; Taylor and Ragg 2005), this could represent an important fraction of the dry-weight loss during starvation (~17% on the basis of CI change).
As noted above, the respiration data do not indicate a statistical difference in oxygen uptake between fed and starved individuals. This is consistent with the conclusions of other authors in that haemocyanin is not a major contributor to oxygen delivery to tissues, its main function being to serve as an oxygen reserve during periods of clamping to the substrate (Behrens et al. 2002; Ragg and Taylor 2006a, 2006b).
Stress effects
There is increased interest in understanding how organisms might cope with the stressors associated with climate change and associated weather patterns and some authors believe that haliotids, especially adults, are particularly vulnerable (Vilchis et al. 2005; Aalto et al. 2020). Clarke and Creese (1998), Young et al. (2006) and Morash and Alter (2016)suggested that these stressors also extend to haliotid aquaculture and pointed out that more research is needed on the cellular and biochemical responses. Such research has been undertaken for commercial pāua in New Zealand exposed to air for 4–120 h at 16°C. Ragg and Watts (2015) found that the ratio of haemolymph sodium and potassium showed a strong correlation with emersion time, suggesting that this was a good indicator of stress. More recent research by Alfaro et al. (2021) successfully used metabolomics to identify the multiple stressors associated with the live transport of pāua to other countries. We suggest that haemolymph haemocyanin or protein concentrations could be added to the list of potential indicators of stress for this mollusc. However, it is noteworthy that natural populations of haliotids exhibit haemocyanin concentrations that vary over orders of magnitude (Pilson 1965; Ainslie 1980, present study). Whether this reflects past periods of poor food availability or previous haemolymph loss caused by injury, exacerbated by a lack of haemolymph clotting ability (Taylor et al. 1994), is unknown.
The current research was undertaken using pāua from a population regarded as stunted where, traditionally, individuals reach a smaller maximal length than in nearby subtidal populations. The reasons for the reduced growth rate are unknown; however, it might be that these individuals may have been under stress at the start of the experiment, given that local scientists suggest that translocated pāua grow quickly in good conditions (Reyn Naylor, pers. comm.). In our experiments, pāua were acclimated to laboratory conditions with good water quality and abundant food supply for 2 weeks before experimentation. We consider the responses recorded in this research are most likely to be typical of pāua from other locations. Finally, the responses to starvation will depend on the physiological state of the individuals and individuals from a more vigorous population might respond differently.
Ecological and bioenergetic consequences
Many gastropods live in conditions of variable food availability; however, possible effects on survival and growth are unclear because of interactions with other environmental factors (Chapperon et al. 2019). However, there is evidence of starvation effects of red abalone H. rufescencs following the loss of kelp forests to sea urchin barrens (Rogers-Bennett et al. 2021), where survivors were of lower weight and had a reduced gonad index, resulting in poor reproductive potential.
Pāua are typically found in clear water, but silt and suspended material could influence normal behaviour and long-term exposure might lead to reduced growth. Silt is increasingly present in coastal situations following storms and natural hazards, including earthquake activity. It is likely that following the Kaikoura earthquake sequences, pāua populations were unable to feed for several months. Our data suggest that these individuals could have survived these conditions until suitable water quality and food became available. This would allow reproduction to continue as usual. The success of paua recruitment the year following earthquake disturbances supports this prediction (Schiel et al. 2024).
The current research contributes to dynamic energy budget (DEB) theory, which describes the way an organism acquires and uses its energy for growth and metabolism and how this is influenced by environmental variables, including food quality and quantity (Nisbet et al. 2012). The models, which have been developed mainly using fish examples, generally use routine or normal metabolic rates, which include energy used for ingestion and digestion. In the present study, pāua fasting for 100 days excludes these energy losses and represents the seldom measured standard metabolic rate. The results from the present study suggest that the routine metabolic rate measured in our experimental set up is similar to the standard rate and thus could be used in assessing the efficiency of growth of adult pāua in aquaculture systems.
Conclusions
This research has confirmed that unlike some gastropods, pāua are able to survive quite long periods of starvation at 15°C. Although there were changes in tissue dry weights, total bodyweight was maintained and oxygen uptake was reasonably unaffected by starvation. This appeared to be supported by changes in the major haemolymph protein, which may function as a nutritive reserve, in addition to its roles in the transport, storage and exchange of oxygen.
Although there were limitations due to sample size in the present research, it might be of interest to extend the starvation period further than 100 days and evaluate the associated physiological responses. However, it would be informative to examine the time course of haemocyanin concentration recovery after natural or experimental resupply of their food. Despite the absence of a haemolymph clotting mechanism, fed pāua survived serial haemolymph sampling and remained active. With care, serial blood sampling could be used to minimise the number of individuals needed for laboratory manipulations in some experiments.
Acknowledgements
We thank Renny Bishop for collecting the pāua and algal food used in these experiments. Jonathan Hill is thanked for supplying and maintaining the equipment used.
References
Aalto EA, Barry JP, Boch CA, Litvin SY, Micheli F, Woodson CB, De Leo GA (2020) Abalone populations are most sensitive to environmental stress effects on adult individuals. Marine Ecology Progress Series 643, 75-85.
| Crossref | Google Scholar |
Ainslie A (1980) Haemocyanin concentrations in field populations of three species of southern Australian abalone. Australian Journal of Marine and Freshwater Research 31, 627-633.
| Crossref | Google Scholar |
Alestra T, Gerrity S, Dunmore R, Marsden I, Pirker J, Schiel DR (2018) Rocky reef impacts of the Kaikoura earthquake: quantification and monitoring of nearshore habitats and communities. New Zealand Aquatic Environment and Biodiversity Report Number 253. (Fisheries New Zealand, Ministry for Primary Industries: Wellington, New Zealand) Available at https://www.mpi.govt.nz/dmsdocument/45130-Rocky-reef-impacts-of-the-2016-Kaikoura-earthquake-extended-monitoring-of-nearshore-habitats-and-communities-to-3.5-years
Alfaro AC, Nguyen TV, Venter L, Ericson JA, Sharma S, Ragg NLC, Mundy C (2021) The effects of live transport on metabolism and stress responses of abalone (Haliotis iris). Metabolites 11, 748.
| Crossref | Google Scholar |
Allen VJ, Marsden ID, Ragg NLC, Gieseg S (2006) The effects of tactile stimulants on feeding, growth, behaviour, and meat quality of cultured blackfoot abalone, Haliotis iris. Aquaculture 257, 294-308.
| Crossref | Google Scholar |
Barkai R, Griffiths CL (1988) An energy budget for the South African abalone Haliotis midae Linnaeus. Journal of Molluscan Studies 54(1), 43-51.
| Crossref | Google Scholar |
Behrens JW, Elias JP, Taylor HH, Weber RE (2002) The archaeogastropod mollusc Haliotis iris: tissue and blood metabolites and allosteric regulation of haemocyanin function. Journal of Experimental Biology 205, 253-263.
| Crossref | Google Scholar | PubMed |
Braid BA, Moore JD, Robbins TT, Hedrick RP, Tjeerdema RS, Friedman CS (2005) Health and survival of red abalone, Haliotis rufescens, under varying temperature, food supply, and exposure to the agent of withering syndrome. Journal of Invertebrate Pathology 89, 219-231.
| Crossref | Google Scholar | PubMed |
Calow P (1974) Some observations on locomotory strategies and their metabolic effects in two species of freshwater gastropods, Ancylus fluviatilis Müll. and Planorbis contortus Linn. Oecologia 16, 149-161.
| Crossref | Google Scholar | PubMed |
Calow P, Sibly RM (1990) A physiological basis of population processes: ecotoxicological implications. Functional Ecology 4, 283-288.
| Crossref | Google Scholar |
Carefoot TH, Qian P-Y, Taylor BE, West T, Osborne J (1993) Effect of starvation on energy reserves and metabolism in the northern abalone, Haliotis kamtschatkana. Aquaculture 118, 315-325.
| Crossref | Google Scholar |
Carefoot TH, Taylor BE, Donovan DA (1998) Seasonality in digestive-gland size and metabolism in relation to reproduction in Haliotis kamtschatkana. Journal of Shellfish Research 17, 713-716.
| Google Scholar |
Chandurvelan R, Marsden ID, Glover CN, Gaw S (2015) Assessment of a mussel as a metal bioindicator of coastal contamination: relationships between metal bioaccumulation and multiple biomarker responses. Science of The Total Environment 511, 663-675.
| Crossref | Google Scholar | PubMed |
Chapperon C, Clavier J, Dugué C, Amice E, Le Goff M, Roussel S (2019) Seasonal and diurnal variability in carbon respiration, calcification and excretion rates of the abalone Haliotis tuberculata L. Journal of the Marine Biological Association of the United Kingdom 99, 393-402.
| Crossref | Google Scholar |
Cho SH, Cho YJ, Choi CY (2011) Effect of feeding regime on compensatory growth of juvenile abalone, Haliotis discus hannai, fed on the dry sea tangle, Laminaria japonica. World Aquaculture 42, 122-126.
| Crossref | Google Scholar |
Clarke CB, Creese RG (1998) On-growing cultured abalone (Haliotis iris) in northern New Zealand. Journal of Shellfish Research 17, 607-613.
| Google Scholar |
Cook PA (2010) Developments and trends in worldwide abalone farming and implications for the European Industry. Aquaculture Europe 30, 5-8.
| Google Scholar |
Dang VT, Speck P, Benkendorff K (2012) Influence of elevated temperatures on the immune response of abalone, Haliotis rubra. Fish & Shellfish Immunology 32, 732-740.
| Crossref | Google Scholar | PubMed |
Diaz RJ, Rosenberg R (1995) Marine benthic hypoxia: a review of its ecological effects and the behavioural responses of benthic macrofauna. Oceanography and Marine Biology: an Annual Review 33, 245-303.
| Google Scholar |
Dı́az F, del Rı́o-Portı́lla MA, Sierra E, Aguilar M, Re-Araujo AD (2000) Preferred temperature and critical thermal maxima of red abalone Haliotis rufescens. Journal of Thermal Biology 25, 257-261.
| Crossref | Google Scholar |
Donovan DA, Taylor HH (2008) Metabolic consequences of living in a wave-swept environment: effects of simulated wave forces on oxygen consumption, heart rate, and activity of the shell adductor muscle of the abalone Haliotis iris. Journal of Experimental Marine Biology and Ecology 354, 231-240.
| Crossref | Google Scholar |
Durazo-Beltrán E, Viana MT, D’Abramo LR, Toro-Vasquez JF (2004) Effects of starvation and dietary lipid on the lipid and fatty acid composition of muscle tissue of juvenile green abalone (Haliotis fulgens). Aquaculture 238, 329-341.
| Crossref | Google Scholar |
El-Eman MA, Madsen H (1982) The effect of temperature, darkness, starvation and various food types on growth, survival and reproduction of Helisoma duryi, Biomphalaria alexandrina and Bulinus truncatus (Gastropoda: Planorbidae). Hydrobiologia 88, 265-275.
| Crossref | Google Scholar |
Gaty G, Wilson JH (1986) Effect of body size, starvation, temperature and oxygen tension on the oxygen consumption of hatchery-reared ormers Haliotis tuberculata L. Aquaculture 56, 229-237.
| Crossref | Google Scholar |
Gerrity S, Alestra T, Fischman HS, Schiel DR (2020) Earthquake effects on abalone habitats and populations in southern New Zealand. Marine Ecology Progress Series 656, 153-161.
| Crossref | Google Scholar |
Harris JO, Maguire GB, Edwards SJ, Johns DR (1999) Low dissolved oxygen reduces growth rate and oxygen consumption rate of juvenile greenlip abalone, Haliotis laevigata Donovan. Aquaculture 174, 265-278.
| Crossref | Google Scholar |
Hooper C, Day R, Slocombe R, Benkendorff K, Handlinger J, Goulias J (2014) Effects of severe heat stress on immune function, biochemistry and histopathology in farmed Australian abalone (hybrid Haliotis laevigata × Haliotis rubra). Aquaculture 432, 26-37.
| Crossref | Google Scholar |
Lachambre S, Huchette S, Day R, Boudry P, Rio-Cabello A, Fustec T, Roussel S (2017) Relationships between growth, survival, physiology and behaviour – a multi-criteria approach to Haliotis tuberculata phenotypic traits. Aquaculture 467, 190-197.
| Crossref | Google Scholar |
Leung JYS, Russell BD, Coleman MA, Kelaher BP, Connell SD (2021) Long-term thermal acclimation drives adaptive physiological adjustments of a marine gastropod to reduce sensitivity to climate change. Science of The Total Environment 771, 145208.
| Crossref | Google Scholar |
Marsden ID, Shumway SE, Padilla DK (2012) Does size matter? The effects of body size and declining oxygen tension on oxygen uptake in gastropods. Journal of the Marine Biological Association of the United Kingdom 92, 1603-1617.
| Crossref | Google Scholar |
Morash AJ, Alter K (2016) Effects of environmental and farm stress on abalone physiology: perspectives for abalone aquaculture in the face of global climate change. Reviews in Aquaculture 8, 342-368.
| Crossref | Google Scholar |
Naylor JR, Andrew NL, Kim SW (2006) Demographic variation in the New Zealand abalone Haliotis iris. Marine and Freshwater Research 57, 215-224.
| Crossref | Google Scholar |
Nelson MK, Cruz BC, Buena KL, Nguyen H, Sullivan JT (2016) Effects of abnormal temperature and starvation on the internal defense system of the schistosome-transmitting snail Biomphalaria glabrata. Journal of Invertebrate Pathology 138, 18-23.
| Crossref | Google Scholar | PubMed |
Nguyen TT, Marsden ID, Davison W, Pirker J (2023) Effects of acclimation temperature and exposure time on the scope for growth of the blackfoot pāua (Haliotis iris). Marine and Freshwater Research 74, 1465-1477.
| Crossref | Google Scholar |
Nisbet RM, Jusup M, Klanjscek T, Pecquerie L (2012) Integrating dynamic energy budget (DEB) theory with traditional bioenergetic models. Journal of Experimental Biology 215, 892-902.
| Crossref | Google Scholar | PubMed |
Pilson MEQ (1965) Variation of hemocyanin concentration in the blood of four species of Haliotis. The Biological Bulletin 128, 459-472.
| Crossref | Google Scholar |
Poore GCB (1972a) Ecology of New Zealand abalones, Haliotis species (Mollusca: Gastropoda). 1. Feeding. New Zealand Journal of Marine and Freshwater Research 6, 11-22.
| Crossref | Google Scholar |
Poore GCB (1972b) Ecology of New Zealand abalones, Haliotis species (Mollusca: Gastropoda). 3. Growth. New Zealand Journal of Marine and Freshwater Research 6, 534-559.
| Crossref | Google Scholar |
Poore GCB (1973) Ecology of New Zealand abalones, Haliotis species (Mollusca: Gastropoda). 4. Reproduction. New Zealand Journal of Marine and Freshwater Research 7, 67-84.
| Crossref | Google Scholar |
Ragg NLC, Taylor HH (2006a) Heterogeneous perfusion of the paired gills of the abalone Haliotis iris Martyn 1784: an unusual mechanism for respiratory control. Journal of Experimental Biology 209, 475-483.
| Crossref | Google Scholar | PubMed |
Ragg NLC, Taylor HH (2006b) Oxygen uptake, diffusion limitation, and diffusing capacity of the bipectinate gills of the abalone, Haliotis iris (Mollusca: Prosobranchia). Comparative Biochemistry and Physiology – A. Molecular and Integrative Physiology 143, 299-306.
| Crossref | Google Scholar | PubMed |
Ragg NLC, Watts E (2015) Physiological indicators of stress and morbidity in commercially handled abalone Haliotis iris. Journal of Shellfish Research 34, 455-467.
| Crossref | Google Scholar |
Ragg NLC, Taylor HH, Behrens J (2000) Stress and weight loss associated with handling in the blackfoot abalone, Haliotis iris. Journal of Shellfish Research 19, 528-529.
| Google Scholar |
Rogers-Bennett L, Dondanville RF, Moore JD, Vilchis LI (2010) Response of red abalone reproduction to warm water, starvation, and disease stressors: implications of ocean warming. Journal of Shellfish Research 29(3), 599-611.
| Crossref | Google Scholar |
Rogers-Bennett L, Klamt R, Catton CA (2021) Survivors of climate driven abalone mass mortality exhibit declines in health and reproduction following kelp forest collapse. Frontiers in Marine Science 8, 725134.
| Crossref | Google Scholar |
Shin SR, Kim HJ, Lee DH, Kim H, Sohn YC, Kim JW, Lee JS (2020) Gonadal maturation and main spawning period of Haliotis gigantia (Gastropoda: Haliotidae). Development & Reproduction 24, 79-88.
| Crossref | Google Scholar | PubMed |
Taylor HH, Ragg NLC (2005) Extracellular fluid volume, urine filtration rate and haemolymph mixing time in the abalone, Haliotis iris Martyn: a comparison of 51Cr-EDTA and 14C-inulin as extracellular markers. Marine and Freshwater Research 56, 1117-1126.
| Crossref | Google Scholar |
Taylor J, Schiel D, Taylor HH (1994) The first cut is the deepest: wounding, bleeding and healing in the black-footed paua (Haliotis iris). Seafood New Zealand 2(February), 47-48.
| Google Scholar |
Thompson JT, Lowe AD, Kier WM (1998) The columellar muscle of prosobranch gastropods: morphological zonation and its functional implications. Invertebrate Biology 117, 45-56.
| Crossref | Google Scholar |
Travers M-A, Mirella de Silva P, Le Goic N, Marie D, Donval A, HuChette S, Koken M, Paillard C (2008) Morphologic, cytometric and functional characterisation of abalone (Haliotis tuberculata) haemocytes. Fish & Shellfish Immunology 24, 400-411.
| Crossref | Google Scholar | PubMed |
Trueman ER, Brown AC (1985) The mechanism of shell elevation in Haliotis (Mollusca: Gastropoda) and a consideration of the evolution of the hydrostatic skeleton in Mollusca. Journal of Zoology 205, 585-594.
| Crossref | Google Scholar |
Uki N, Kikuchi S (1975) Oxygen consumption of the abalone, Haliotis discus hannai in relation to body size and temperature. Bulletin of Tohoku Regional Fisheries Research Laboratory 35, 73-84.
| Google Scholar |
Vilchis LI, Tegner MJ, Moore JD, Friedman CS, Riser KL, Robbins TT, Dayton PK (2005) Ocean warming effects on growth, reproduction, and survivorship of southern California abalone. Ecological Applications 15, 469-480.
| Crossref | Google Scholar |
Wells RMG, Baldwin J (1995) A comparison of metabolic stress during air exposure in two species of New Zealand abalone, Haliotis iris and Haliotis australis: implications for the handling and shipping of live animals. Aquaculture 134(3–4), 361-370.
| Crossref | Google Scholar |
Young JL, Bornik ZB, Marcotte ML, Charlie KN, Wagner GN, Hinch SG, Cooke SJ (2006) Integrating physiology and life history to improve fisheries management and conservation. Fish and Fisheries 7, 262-283.
| Crossref | Google Scholar |
Zhao Q, Cheung SG, Shin PKS, Chiu JMY (2011) Effects of starvation on the physiology and foraging behaviour of two subtidal nassariid scavengers. Journal of Experimental Marine Biology and Ecology 409, 53-61.
| Crossref | Google Scholar |