Murray cod and modern fish screens: influence of water velocity and screen design on the entrainment and impingement of larval and young-of-year fish at water offtakes
Jerom R. Stocks

A
B
C Deceased.
Abstract
Entrainment and removal of fish from aquatic ecosystems can occur at water pump offtakes. Exclusion screens that reduce these impacts are recognised as an important conservation measure.
Evaluate the effectiveness of the Australian screen design guidelines in protecting larvae and young-of-year age class of a native fish species, Murray cod Maccullochella peelii.
Entrainment and impingement of postflexion larvae and young-of-year were assessed in a controlled laboratory environment. Tests were conducted under a range of approach velocities (AV) and impingement durations for two screen materials.
Fish screens reduced larval entrainment by ≤84%. Screens had no significant effect on reducing larval entrainment at AV ≥0.125 m s−1. Impingement of young-of-year was positively associated with AV and mortality increased with impingement duration, irrespective of screen type.
To protect early life-stage Murray cod, it is recommended that water pump offtakes be fitted with 2-mm vertical wedge-wire stainless steel screens and AV be limited to ≤0.1 m s−1.
This study represents the first assessment of the effectiveness of the Australian screen design guidelines in protecting larvae, providing knowledge to further refine specifications for screen design and support the recovery of native fish populations.
Keywords: approach velocity, fish losses, irrigation diversions, Murray–Darling Basin, native fish recovery, pump offtake, pump screen, water diversion.
Introduction
Meeting the global demand for freshwater poses a substantial environmental challenge (Smakhtin 2004; Baumgartner et al. 2019). Irrigation accounts for approximately 70% of total water extractions globally (Grafton 2019), and is critical for food production and economic prosperity (Galbraith et al. 2005; Australian Bureau of Statistics 2008). However, where levels of extraction and diversion of water from rivers exceed sustainable levels, impacts can be imposed on aquatic ecosystem health and ecological processes (Kingsford 2000; Grafton et al. 2013). One important ecological impact is the permanent removal of fish from natural waterways by pumps and gravity-fed water diversions.
Australia’s Murray–Darling Basin (MDB) covers an area of over 1 × 106 km2. The MDB contains 20% of national agricultural land (Australian Bureau of Statistics 2008), supported by diversion of surface water (11,836 GL year−1 sustainable diversion limit in 2022–23; Murray–Darling Basin Authority 2023). Here, the loss of native fish to entrainment at diversions has been identified as a concern for freshwater fisheries management, with historical and contemporary data suggesting that tens of millions of native fish are lost annually (Boys et al. 2021).
Modern fish-protection screens can reduce fish losses at pump offtakes (McMichael et al. 2004; Boys 2021). In the MDB, significant public investment has been dedicated to incentivisation programs to stimulate uptake of modern screening technology (Boys 2021). Notably, the Australian Commonwealth Government has invested a total of A$26 × 106 under the Northern Basin Toolkit in the states of New South Wales (NSW) and Queensland, and the NSW Government has invested $13.5 × 106 in the Macquarie River valley (Rayner et al. 2023). Other, smaller programs are also available in NSW and other jurisdictions. All these programs aim to support water users in accessing technology that is suitable for their water diversion, to provide a combination of private and public benefits.
Testing the effectiveness of screens is an essential component of developing ‘fish-friendly’ designs and operating specifications (Boys 2021). Screens are of limited utility if they fail to prevent fish entrainment or cause injuries and mortality by impinging fish on the screen surface (Stocks et al. 2019). Screen design specifications, tailored to species and river conditions, are available for North America and Europe (Department of Fisheries and Oceans 1995; Electric Power Research Institute 2000; McMichael et al. 2004; Turnpenny and O’Keeffe 2005). Australia also has relatively new design specifications (Boys 2021). However, these are based largely on experiments using juvenile native species, namely golden perch (Macquaria ambigua) and silver perch (Bidyanus bidyanus) (Boys et al. 2013a, 2013b; Stocks et al. 2019). A significant knowledge gap remains in relation to larvae for all native Australian fish species (Stocks et al. 2019), and for the larval and juvenile life-history stages of Murray cod (Maccullochella peelii). Given a number of native Australian fish species of the MDB have obligate or facultative downstream drifting phases that are likely vulnerable to entrainment (Humphries et al. 1999, 2002; Humphries and Lake 2000, Lintermans and Phillips 2004; King et al. 2005; Koehn and Harrington 2005), further studies are required to examine what screen specifications can protect larval and early life-stage native fish.
Two critical specifications that influence performance of screens are: (1) the size of openings on the screen (referred to as the ‘mesh size’); and (2) the velocity of water approaching the front of the screen (referred to as ‘approach velocity’, AV), (Danley et al. 2002; Peake 2004; Swanson et al. 2004; Stocks et al. 2019). This study aimed to define mesh size and AV for screens to protect early life-history stages of Murray cod (up to the age of ~28 days). This species is: an ecological important apex predator that is widely distributed throughout the MDB; is important as a cultural and recreational fishing species; and has life-history traits of larval drift and pelagic juveniles that increase susceptibility to entrainment and impingement at water diversions (Gehrke 1990; Neira et al. 1998; Gilligan and Schiller 2003; Lintermans 2007; Humphries 2023). The specific objectives of this study were to examine: (1) entrainment of larval Murray cod; and (2) impingement and entrainment of young-of-year (YOY) Murray cod, at varied AV, in an experimental flume fitted with two types of modern fish screens; and (3) examine whether YOY Murray cod mortality was a function of impingement duration. Ultimately, the goal was to determine if current Australian specifications for mesh size and AV are suitable to protect early life-history stages of Murray cod.
Materials and methods
Experimental design
Three experiments were conducted in a laboratory flume to examine the entrainment (propensity to be sucked through a screen or into the pump inlet) and impingement (propensity to be held against the screen) of larval and YOY Murray cod at a simulated offtake structure.
Experiment 1 examined entrainment at varying AV of hatchery-bred postflexion larval Murray cod, of age 15–16 days, that were small enough to physically pass through the two treatment screens (larval Murray cod mean ± s.e. standard length, SL = 10.6 ± 0.1 mm, minimum SL = 10 mm, maximum SL = 11.4 mm, mean head width = 2.00 mm). A ‘no screen’ treatment was also run at each AV.
Experiment 2 examined impingement at varying AV of hatchery bred YOY Murray cod, of age ~28 days, that were too large to be able to pass through the two treatment screens (YOY Murray cod mean ± s.e. SL = 26.6 ± 0.1 mm, minimum SL = 23.6 mm, maximum SL = 28.9 mm, mean head width = 4.93 mm). A ‘no screen’ treatment was also run at each AV to examine YOY entrainment.
Experiment 3 examined YOY Murray cod mortality as a function of impingement duration for the same size class of fish used in Experiment 2.
Each replicate in all experiments were run at 340 lx, measured using an ExTech Easy View 30 light meter. The water temperature for Experiment 1 was 17.9°C and 0 NTU turbidity. For Experiments 2 and 3 the water temperature was 21.3°C and turbidity was 0.3 NTU.
Fish collection and holding
Larvae and YOY Murray cod were obtained from the Narrandera Fisheries Centre Hatchery (NSW, Australia). Egg trays were removed from spawning boxes situated within earthen ponds and transferred to holding tanks within the hatchery. Larvae emerged after 7–10 days and were kept in aerated trays (50 cm long, 50 cm wide and 15 cm deep) before experimentation. Up to 17 days post-hatching, Murray cod larvae were sustained on their own yolk reserves, after which time this was supplemented with daily feeds of newly hatched nauplii. For YOY juvenile Murray cod used in the experiments, larvae were stocked into a large earthen pond (3600 m2 and ~3 ML) at 3 days post-hatching, where they fed naturally on the available plankton. At ~28 days post-hatching, 24 h before Experiment 2 commenced, Murray cod YOY were collected from the pond with a hand net.
Larvae and YOY were kept alive in 900-L troughs with flow-through river water at ambient temperatures for the duration of the experiments. Within the troughs, replicate groups of 10 fish were acclimated in 500-mL perforated plastic holding containers (AquaOne Midi Float Guppy tank) for 24 h prior to the experiment. Feeding was ceased 24 h prior to experimentation. Water quality parameters including temperature, dissolved oxygen, conductivity, and turbidity were monitored throughout the experiment using a water quality meter (Horiba Multi Water Quality Checker U-50 Series) to ensure conditions remained within an acceptable range (Barker et al. 2009).
The experiment was conducted under animal research authority number 14/02 granted by the Primary Industries (Fisheries) Animal Care and Ethics Committee and scientific collection permit P01/0059(A).
Experimental flume apparatus
The swimming flume apparatus used in this experiment was similar to the apparatus described in Stocks et al. (2019) and Boys et al. (2013a). The apparatus was comprised of an open top, partially submerged rectangular swimming flume constructed from a perforated aluminium plate (3 mm holes with 30% porosity; Fig. 1b). A removable, cylindrical fish-holding cradle constructed from the 3-mm perforated aluminium plate wrapped in woven fibreglass lattice mesh (0.28-mm diameter wire and 1.22-mm aperture) was used to allow easy removal of fish from the flume after each replicate treatment (Fig. 1). The fish-holding cradle was butted against the removable fish screen. The flume was positioned within the centre of a 2.5-m diameter circular flow-through tank filled to a depth of 0.70 m (containing ~1800 L). Water was drawn from the surrounding tank through the perforated mesh flume by a pump capable of delivering 1950 L min−1. Water drawn from the tank through the flume was recirculated into the holding tank through a network of 100-mm high-pressure plastic polymer piping. Water flow was controlled by two ball valves fitted within the pipe network allowing for manipulation of AV values by 0.01 m s−1. The velocity profile perpendicular to the screen was measured at eight increasing distances from the screen, up to a maximum distance of 0.50 m using a hand-held propeller-driven flow meter.
Schematics of experimental flume apparatus: (a) closeup of experimental flume; (b) experiment flume apparatus.
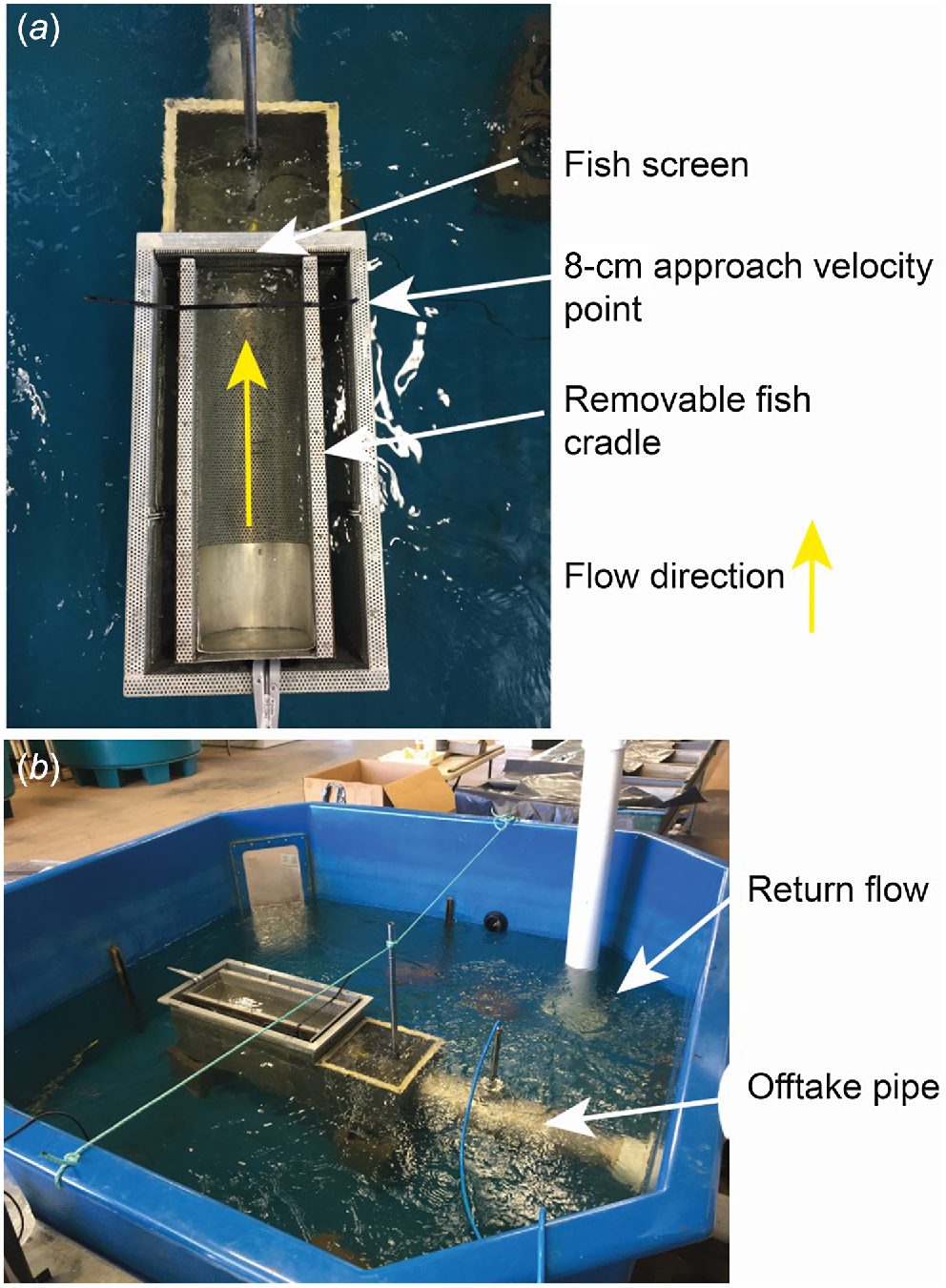
The flume was designed to allow for interchangeable fish screens. The two screen materials used in this study were a 2-mm-wide vertical wedge-wire stainless steel screen (hereafter referred to as ‘2-mm screen’) and a perforated aluminium plate with 3-mm diameter holes and 30% porosity (hereafter referred to as ‘3-mm screen’; Fig. 2). Both screen types were recommended for the protection of native fish in the current Australian screen design guidelines (Boys 2021). The velocity 8 cm in front of the screen was denoted as the AV for standardised comparison to earlier studies by Boys et al. (2013a) and Stocks et al. (2019) (Fig. 1 and 3) and was varied as outlined in the following experiments.
The two screen types assessed in this study: (a) 2-mm-wide vertical wedge-wire stainless steel screen; (b) perforated aluminium plate with 3-mm diameter holes and 30% porosity.
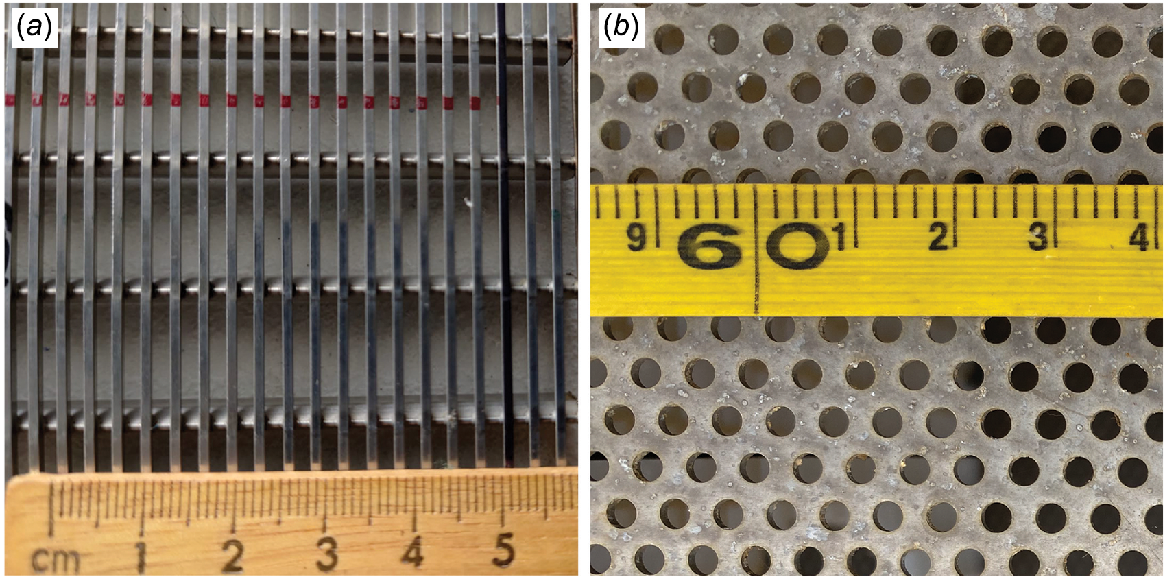
Velocity profile measured within the flume at eight increasing distances along the centreline from the fish screen (0.5, 5, 8, 10, 20, 30, 40 and 50 cm). Each datapoint shows the average of three velocity measurements with standard error. The treatment approach velocity of 8 cm in front of the screen is shown with the dashed line.
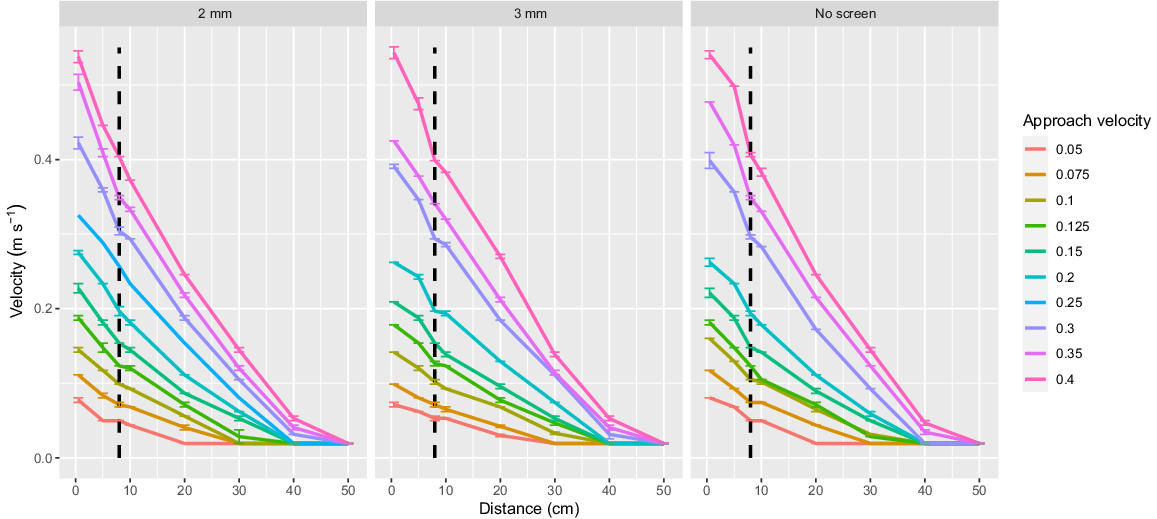
Experiment 1 – larval entrainment
The larval entrainment experiment was conducted from 24 to 27 October 2016. Entrainment was compared at seven AV treatments (0, 0.05, 0.075, 0.1, 0.125, 0.15 and 0.2 m s−1) in the presence of the 2-mm screen, 3-mm screen and absence of a screen, hereafter referred to as ‘no screen’. Five replicates were tested for each treatment, with each replicate consisting of a group of 10 fish in the flume. The order of replicates was assigned using a randomised block design. Additionally, five replicate 0.0 m s−1 AV treatment controls were included.
To begin a treatment, a replicate group of fish was placed in the flume cradle and held at the end farthest from the screen using a 2-mm perforated plate divider covered in woven fibreglass lattice mesh with 0.28-mm diameter wire and 1.22-mm aperture, that allowed fish to acclimate after the transfer (velocity 0.0–0.01 m s−1 at holding area). After 1 min of acclimatisation, the divider was removed and the fish were slowly guided with the divider, preventing erratic fleeing behaviour, to a distance of 8 cm from the exclusion screen, being the distance where fish were judged to interact with the a priori defined AV. Fish were guided to the a priori defined AV at 8 cm in front of the screen to ensure all fish were exposed to the treatment AV, providing a balanced experimental design, and ensuring no fish could occupy potential eddies or vortices in the corners of the fish cradle. Once all the fish were within 8 cm of the screen, the divider was removed, and the pump operation continued for another 1 min. After that time, the pump was turned off and all fish that were not entrained were counted to provide a proportional measure of the dependent variable ‘entrainment’ through the screen.
Experiment 2 – YOY impingement or entrainment
The YOY impingement or entrainment experiment was conducted from 21 to 24 November 2016. Impingement was compared at six AV treatments (0, 0.1, 0.2, 0.3, 0.35, and 0.4 m s−1) in the presence of the 2- and 3-mm screen, and entrainment was examined in the absence of a screen. Five replicates were tested for each treatment, with each replicate consisting of a group of 10 fish in the flume. The order of replicates was assigned using a randomised block design. Five replicate 0.0 m s−1 AV treatment controls were included. Each replicate treatment was performed as described in Experiment 1 above. After 1 min at the treatment AV, the number of fish impinged against the screen was counted and the pump was turned off. Screen impingement was a binary measure (impinged or free swimming) and was assessed at 1 min of exposure at the treatment velocity (i.e. instantaneous measure of the count of fish stuck to the screen at 1 min of pumping), providing a proportional measure of the dependent variable ‘impingement’. For the ‘no screen’ treatments, the pump was turned off after 1 min of pumping and the fish that were not entrained were counted to provide a proportional measure of the dependent variable ‘entrainment’.
Experiment 3 – YOY impingement duration
The YOY impingement duration experiment was conducted from 21 to 24 November 2016. A 0.3 m s−1 AV was identified as the minimum velocity required for 100% impingement to occur within 1 min. Five replicates, each containing 10 Murray cod, were run for four durations (0, 1, 10 and 20 min) for the 2- and 3-mm screen at 0.3 m s−1 AV. Additionally, five replicate holding controls were kept in the holding troughs but not transferred to the experimental flume to ensure any mortality was due to the impingement duration treatments rather than animal husbandry. The 0 m s−1 treatment, when the pump was not operating, acted as a handling control. For each treatment replicate, 10 fish were transferred into the end of the flume cradle farthest from the screen and held there to acclimate for 1 min as detailed above. After this time, the pump velocity was increased to create an AV of 0.3 m s−1 and the divider was removed and then used to slowly guide the fish to 8 cm from the exclusion screen. In all but the 0 m s−1 treatment, 100% impingement occurred within the first minute. At the end of each replicate, the flume was turned off and the number of impinged fish suffering acute mortality was counted. Fish were considered dead if no opercular movement was observed. All fish were transferred back into their holding containers within the troughs and kept for 24 h before they were inspected for further deaths, providing a proportional measure of the dependent variable ‘total mortality’ (acute mortality + 24 h mortality).
Statistical analysis
For Experiment 1 (entrainment), a two-factor ANOVA was used to compare the proportion of larvae entrained at each AV (0, 0.05, 0.075, 0.1, 0.125, 0.15 and 0.2 m s−1) for the two screen types (2- and 3-mm screen) and no screen.
For Experiment 2 (impingement) a two-factor ANOVA was used to compare the proportion of YOY impinged after 1 min of pump operation at each AV (0, 0.1, 0.2, 0.3, 0.35 and 0.4 m s−1) for the two screen types (2- and 3-mm screen).
For Experiment 3 (impingement duration) a two-factor ANOVA was used to compare ‘total mortality’ at each impingement duration (0, 1, 10 and 20 min) for the two screen types (2 mm screen and 3 mm screen) when the AV was 0.3 m s−1.
All data were assessed for normality and homogeneity of variances prior to analyses. Data that did not meet these assumptions were log10 transformed and reassessed. Post hoc pair-wise comparisons were performed using Tukey’s HSD with a P-value of <0.05 considered significant. All analyses were performed in the ‘R’ package (ver. 4.3.1, R Foundation for Statistical Computing, Vienna, Austria, see https://www.r-project.org/) and figures were produced using ggplot2 (ver. 3.4.3, see https://CRAN.R-project.org/package=ggplot2; Wickham 2009).
Results
Experiment 1 – larval entrainment
A significant interaction between the effects of screen type and AV was observed for larval entrainment (F12,86 = 3.02; P < 0.01). Both the 2- and 3-mm screen reduced entrainment of larval Murray cod at 0, 0.05, and 0.075 m s−1 AV compared to no screen (P < 0.05). No significant differences in entrainment were observed between the two screens at the 0, 0.05, and 0.075 m s−1 AV (Tukey’s HSD P > 0.05). At 0.1 m s−1 AV, only the 2-mm screen reduced entrainment in comparison to no screen. The 2- and 3-mm screen had no significant effect on reducing entrainment for AV ≥0.125 m s−1 (0.125, 0.15, and 0.2 m s−1 AV; Fig. 4). At an AV of 0.075 m s−1, the 2-mm screen reduced larval entrainment by 84%, compared to no screen (screened = 10% entrainment, unscreened = 62% entrainment; Fig. 4).
Mean percentage (±s.e.) of larval Murray cod (mean SL = 10.6 mm, minimum SL = 10 mm, maximum SL = 11.4 mm) entrainment when exposed to the six treatment approach velocities for a duration of 1 min using a 2-mm vertical wedge-wire fish screen (red line) and a perforated aluminium plate fish screen with 3-mm diameter holes and 30% porosity (green line). Mean percentages of fish entrainment when no fish screen was implemented are displayed for each treatment velocity (blue line). n = 5 replicates of 10 fish for each treatment. Approach velocity treatment controls are displayed as 0 m s−1 approach velocity.
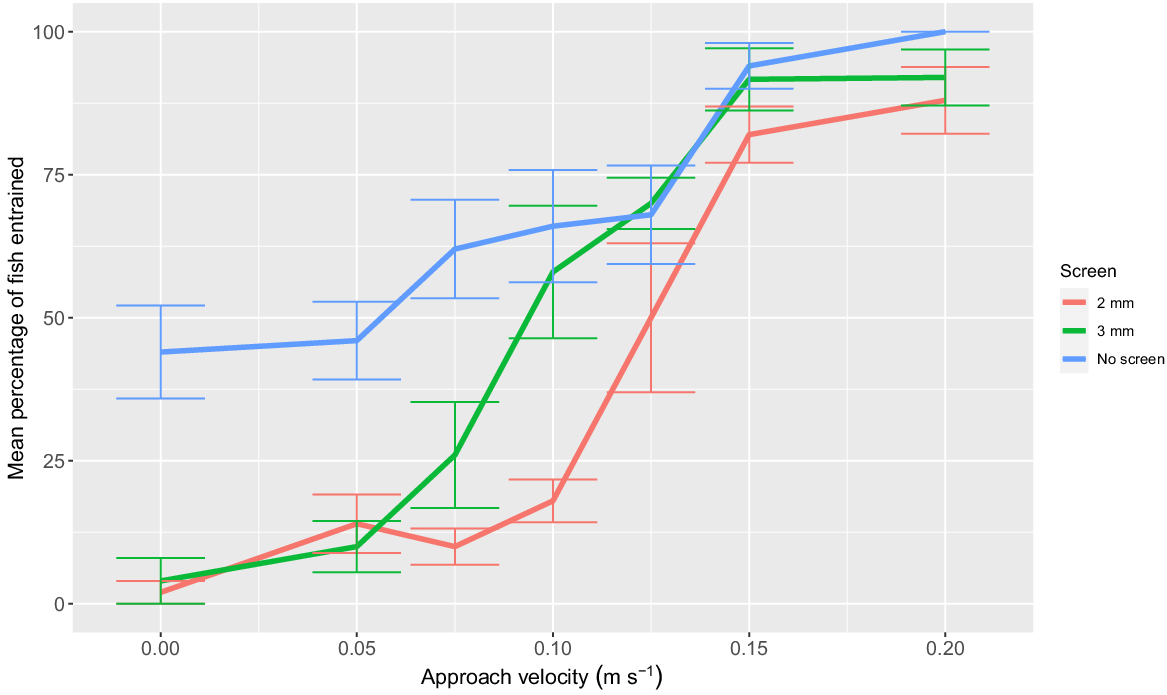
Experiment 2 – YOY impingement or entrainment
Approach velocity (F5,68 = 83.81; P < 0.001) had a significant effect on YOY impingement rates. Increasing AV led to a higher proportion of impinged fish after 1 min of pumping for the 2- and 3-mm screens (Fig. 5). At each AV, impingement was not significantly different between the 2- and 3-mm screens (F1,68 = 4.22; P > 0.05). The mean proportion of YOY entrained during the ‘no screen’ treatment ranged from 96 to 100% for AV of 0.10–0.40 m s−1 (Fig. 5). Both screens prevented the entrainment of all YOY.
Mean percentage (±s.e.) of young-of-year Murray cod (mean SL = 26.6 mm, minimum SL = 23.6 mm, maximum SL = 28.9 mm) impingement when exposed to the five treatment approach velocities for a duration of 1 min using a 2-mm vertical wedge-wire fish screen (red line) and a perforated aluminium plate fish screen with 3-mm diameter holes and 30% porosity (green line). Mean percentages of fish entrainment when no fish screen was implemented are displayed for each treatment velocity (blue line). n = 5 replicates of 10 fish for each treatment. Approach velocity treatment controls are displayed as 0 m s−1 approach velocity.
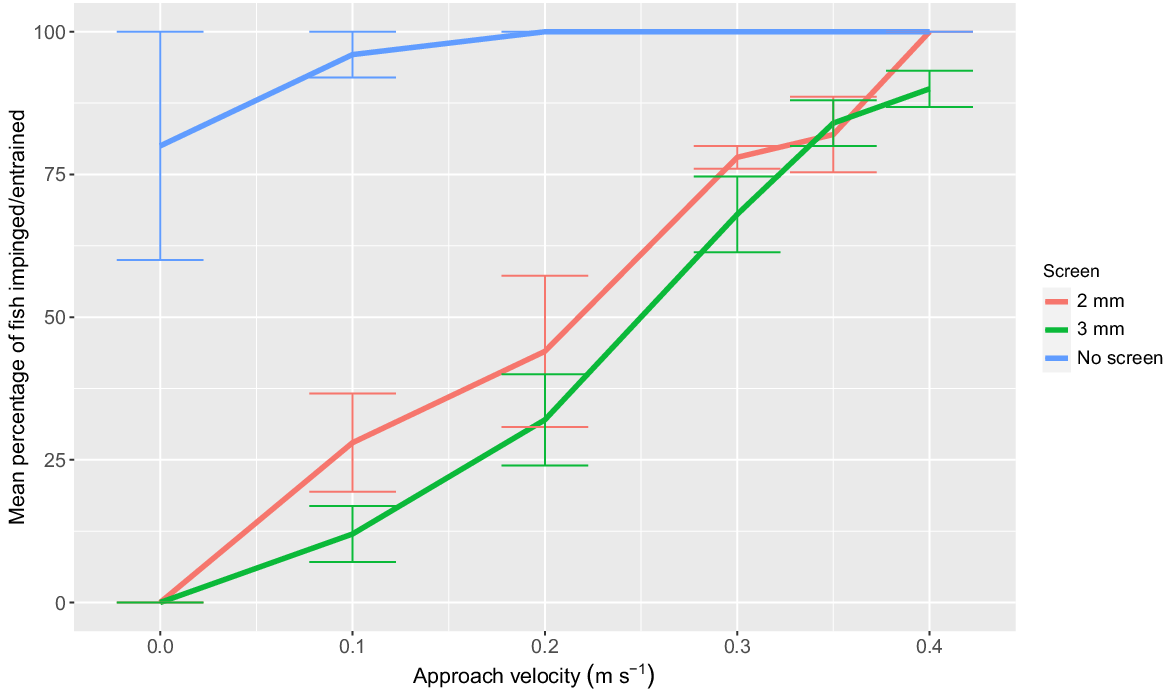
Experiment 3 – YOY impingement duration
Total mortality was positively related to impingement duration (F3,32 = 19.24; P < 0.0001). For both the 2- and 3-mm screen, total mortality for impingement durations greater than 1 min were significantly greater than the holding controls (Fig. 4). Total mortality was not significantly different between the 2- and 3-mm screen for each impingement duration (P > 0.05, Tukey’s HSD pairwise compassions), (Fig. 6).
Mean percentage (±s.e.) of young-of-year Murray cod (mean SL = 26.6 mm, minimum SL = 23.6 mm, maximum SL = 28.9 mm) that suffered mortality at the four impingement durations when exposed to a 0.3 m s−1 approach velocity at a 2-mm wedge-wire fish screen (red histogram) and a perforated aluminium plate fish screen with 3-mm diameter holes and 30% porosity (green histogram). n = 5 replicates of 10 fish for each treatment. Handling controls are displayed as 0-min impingement duration.
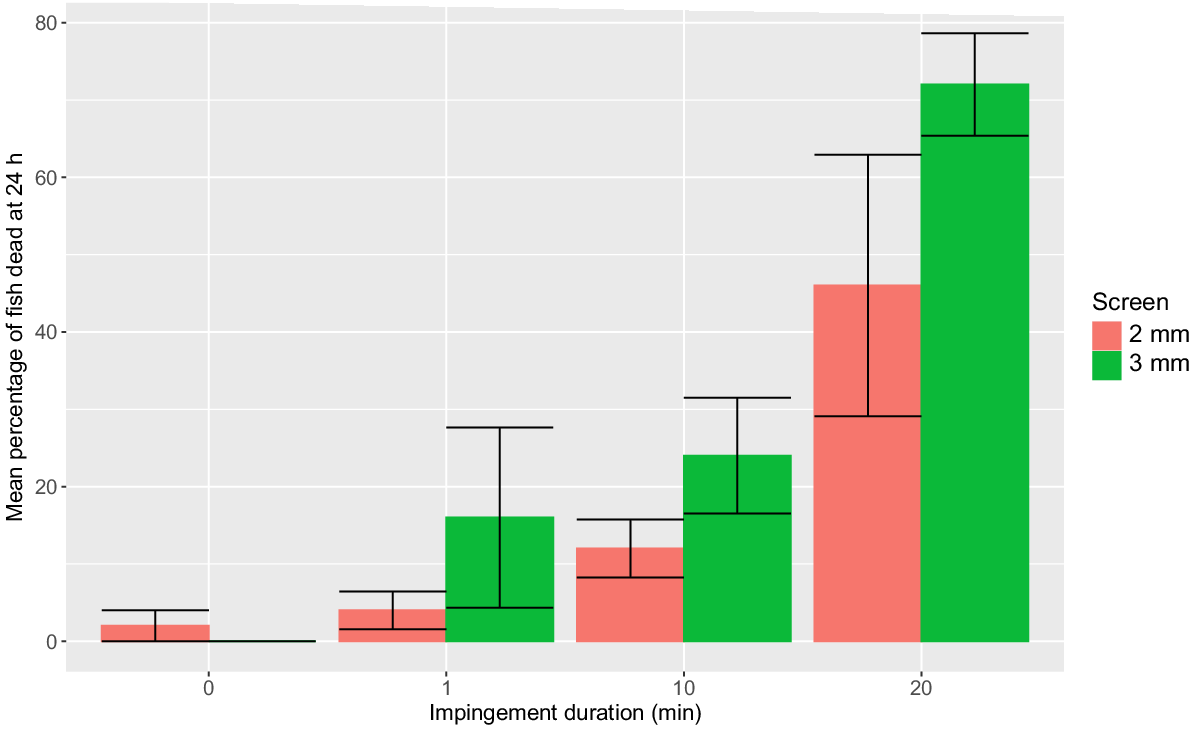
Discussion
This study represents the first published assessment of Australian fish screening design guidelines for a larval age class of a native species. Additionally, it is the first study to assess entrainment and impingement risk for larval and YOY Murray cod. Our results indicate that the prescribed Australian screening guidelines provide protection for postflexion larvae and YOY Murray cod. However, at 0.1 m s−1 AV, prescribed as the upper limit by Boys (2021), only the 2-mm screen provided significant protection, by reducing entrainment of larvae from 66% (no screen) to 18%. At ≥0.125 m s−1 AV, the 2- and 3-mm screens had no significant effect on reducing entrainment of larvae. Both screen types physically eliminated the entrainment of YOY Murray cod. However, impingement of YOY Murray cod was positively associated with AV between 0.05 and 0.4 m s−1, and mortality increased with impingement duration, irrespective of screen type.
Larval entrainment
The present study identified reduced entrainment of larval Murray cod at <0.125 m s−1 AV compared to treatments with no screen. This was likely a behavioural response to the presence of the screen, rather than the screen physically preventing entrainment (given screen aperture was greater than larval head width). This demonstrates that fish larvae are not purely passively drifting organisms and even very young Murray cod can avoid screens if the hydraulic conditions are suitable. Previous research by Boys et al. (2013a) demonstrated that juvenile silver perch and golden perch utilised visual cues to navigate the screen face, thereby enhancing their ability to avoid contact with the screen. However, as the AV increases towards the swimming capacity of larval fish, the physiological limitations of their swimming capacity likely overpower their avoidance behaviour, resulting in entrainment. Similarly, Carter et al. (2023) concluded that while physical exclusion is an important consideration when screening intakes, other biological or hydraulic processes may also influence entrainment.
Results indicated that the 2-mm screen outperformed the 3-mm screen. However, at ≥0.125 m s−1 AV, the fish screens trialled in this study did not reduce entrainment of larval Murray cod. This demonstrates that if the AV is not low enough, the mesh type used at the screen has little relevance to larval fish entrainment. It is important to note that susceptibility to entrainment varies between species (Kelso and Leslie 1979; McLaren and Tuttle 2000). This variability is influenced by factors such as swimming performance and the behavioural response of a species to the presence of a screen (Carter et al. 2023). Swimming performance of fish can be influenced by a range of biological and physical factors (Webb 1975), including morphology (Hammer 1995; Plaut 2001), muscle function (Kieffer 2000), swimming mode (Muller et al. 2001), body condition (Muller et al. 2001), and water temperature (Ojanguren and Brañta 2000). Moreover, ontogenetic changes in swimming performance during larval development, and interspecies variations attributed to precocial life-history strategies, may also influence entrainment susceptibility (Kopf et al. 2014).
To further explore the swimming capabilities of larval fish species, Kopf et al. (2014) tested the swimming performance of the larvae of six Australian freshwater fish species. The results revealed considerable interspecific and ontogenetic variation in swimming performance. For instance, metalarvae of trout cod (Maccullochella macquariensis) exhibited the highest swimming speed, with a maximum critical swimming speed of 0.464 m s−1 (defined as prolonged swimming speed for ≤60 min by Beamish 1978). By contrast, preflexion larvae of silver perch (Bidyanus bidyanus) displayed the slowest swimming speed, with a minimum critical swimming speed of 0.001 m s−1.
Kopf et al. (2014) reported the critical swimming speed of postflexion larval Murray cod ranged ~0.1–0.25 m s−1. Consistent with that research, the present study observed larval entrainment percentages exceeding 90% at AV ≥0.15 m s−1, which aligns with the lower end of the reported critical swimming speed for this species. Hutchison et al. (2020) suggest that burst and sprint speed, defined as maximum swimming speed obtained by anaerobic metabolism in <20 s according to Beamish (1978), may serve as a more explicit indicator of an individual fish’s ability to avoid entrainment. However, limited data on burst and sprint speed for larval species in the MDB are currently available (Watson et al. 2019a, 2019b; Hutchison et al. 2020). In the present study, the flume apparatus design aligns more so with a critical swimming speed assessment. In the wild, a fish may be able to employ a quick swimming burst until it is swept past the screen. To better test this, we recommend that future experiments use a flume design that allows for this behaviour. This may include letting fish volitionally approach the screen and allowing them to avoid impingement by using short bursts of swimming until they have passed by the screen.
In the Pacific Northwest of America, when developing juvenile fish screen criteria, fisheries agencies established criteria that would protect the weakest swimming species during their most vulnerable life-stage under adverse environmental conditions (Nordlund 1996). Kopf et al. (2014) demonstrated that preflexion silver perch larvae displayed the slowest critical swimming speed of Australian native species. Therefore, it is recommended that further fish screening trials be conducted using preflexion silver perch larvae given they are likely a highly vulnerable species and life-stage.
In addition to biophysical studies of swimming performance and screen interactions, studies on egg and larval drift dynamics within natural river systems may further refine design specifications for fish screens to protect native fish larvae. Drifting larval fish have an active component to their movement and are capable of altering their position in the water column (Braaten et al. 2008; Lechner et al. 2014); similarly, drifting fish eggs have shown spatial variability in drift abundances throughout the water column (Faulkner and Copp 2001; Tonkin et al. 2007). Understanding the depths at which native larval fish drift could guide the appropriate placement of offtakes within the water column to minimise exposure to entrainment.
Young-of-year impingement
Modern fish-protection screens have shown effectiveness in reducing entrainment. However, they still pose risks for injury and mortality due to impingement on the screen face (Peake 2004; Stocks et al. 2019). It is critical to design screens that do not exceed the AV at which fish can actively avoid contact with the screen (White et al. 2007). The present study observed impingement of YOY Murray cod at ≥0.1 m s−1 AV, with impingement rates increasing at higher AV. Previous literature supports the positive correlation between impingement and AV (Danley et al. 2002; Swanson et al. 2005; White et al. 2007; Young et al. 2010; Boys et al. 2013a; Stocks et al. 2019; Carter et al. 2023). Consistent with findings by Boys et al. (2013a), there was no statistically significant difference between the two screen types in the proportion of impinged fish, indicating that AV has a greater effect on impingement than screen type.
Fish injury and mortality are positively corelated with impingement duration (Peake 2004; Stocks et al. 2019). In our study, mortality of YOY Murray cod increased significantly with impingement duration for the 2- and 3-mm screens at 0.3 m s−1 AV. Similar findings were reported by Stocks et al. (2019) regarding YOY golden perch, suggesting that reducing impingement time through pump cycling (turning pump on or off to allow impinged fish to escape) could mitigate mortality while maintaining AV. However, implementing pump cycling from an engineering and maintenance perspective may be impractical. Therefore, the most effective approach to minimise impingement-induced mortality is to limit AV and avoid impingement.
Fish screening criteria
Our results indicate that the prescribed Australian fish screening guidelines provide protection for postflexion larvae and YOY Murray cod. Boys (2021) recommended limiting AV to ≤0.1 m s−1 and that aperture size of wedge-wire and perforated plate screens should not exceed 2 mm and 3 mm, respectively. However, it was recognised that further investigation was required to determine the specifications necessary for protecting larval and earlier life-stage fish (Stocks et al. 2019; Boys 2021). Results from the present study show that a 0.1 m s−1 AV did reduce the impingement of YOY Murray cod within an experimental flume. However, at 0.1 m s−1 AV, only the 2-mm screen reduced entrainment of postflexion larval Murray cod. The 3-mm screen at 0.1 m s−1 AV did not reduce entrainment of larval Murray cod when compared to the unscreened treatment. At ≥0.125 m s−1 AV, the fish screens trialled in this study did not reduce entrainment of larval Murray cod. This demonstrates that if the AV is not low enough, the mesh type used at the screen has little relevance to larval fish entrainment.
The present study also demonstrated that when using a 2-mm screen there was limited benefit in further reductions to the maximum prescribed AV of 0.1 m s−1. For the 2-mm screen at 0–0.1 m s−1 AV, there was no significant difference in larval entrainment. Only when AV was greater than 0.1 m s−1 were larval entrainment values significantly different from 0 m s−1. Consequently, we recommend that pump offtakes preferentially be fitted with 2 mm vertical wedge-wire screen over the 3-mm perforate plate screen given their superior performance in larval exclusion.
The positioning of offtake pumps within rivers also has the capability of further protecting fish (Hutchison et al. 2020). Positioning offtakes in rivers with flows acting parallel to the screen, creating sweep velocities across to the screen face, have been shown to reduce fish impingement and entrainment (Danley et al. 2002; Swanson et al. 2004; White et al. 2007; Young et al. 2010). As previously alluded to, the present study did not incorporate sweep velocities. Consequently, impingement and entrainment at the treatment AV are likely overestimated compared to scenarios in flowing water bodies.
Conclusion
Here, we present the first flume-based quantitative evidence of the influence of fish screen design on larval native fish entrainment under different hydraulic flow conditions. This study aimed to define mesh size and AV for screens to protect early life-history stages of Murray cod. The present study refines the current recommended design specifications for modern fish-protection screens in Australia. Specifically, we recommend that pump offtakes be fitted with 2 mm wide vertical wedge-wire stainless steel screens and AV be limited to ≤0.1 m s−1 to reduce larval Murray cod entrainment and YOY Murray cod impingement.
Additional studies investigating screens with smaller aperture sizes, capable of physically preventing larval entrainment, as well as assessing other larval species, drifting eggs, and their interactions with abiotic variables such as sweeping velocities, turbidities and light levels are recommended. Refinements to our experimental and flume design would further improve the applicability of flume studies to revising specifications for screens in the wild, further enhancing the protection of larval native fish and facilitating native fish recovery within agricultural landscapes.
Data availability
The data that support this study will be shared upon reasonable request to the corresponding author.
Declaration of funding
This study was funded by the Orange City Council in association with the Orange Pipeline monitoring program.
Acknowledgements
This article is dedicated to the memory of Dr Chris Walsh for his contribution to the field of fisheries research. We thank New South Wales Fisheries personnel who provided technical advice and assistance, particularly Michael Rodgers, Matthew McLellan, Lachie Jess and Peter Graham. We also thank the Narrandera Fisheries Hatchery for providing fish for the experiment.
References
Australian Bureau of Statistics (2008) 4610.0.55.007 – Water and the Murray–Darling basin – a statistical profile 2000–01 to 2005–06. ABS. Available at https://www.abs.gov.au/ausstats/abs@.nsf/0/DC0DC8AAE4ECD727CA2574A5001F803A
Baumgartner LJ, Deng ZD, Ning N, Conallin J, Lynch AJ (2019) Irrigation, fisheries and sustainable development goals: the importance of working collaboratively to end world hunger and malnutrition. Marine and Freshwater Research 70(9), i-iii.
| Crossref | Google Scholar |
Boys CA (2021) Design specifications for fish-protection screens in Australia. Edition 1. (New South Wales Department of Primary Industries: Taylors Beach, NSW, Australia) Available at https://www.dpi.nsw.gov.au/__data/assets/pdf_file/0006/1373577/Design-specifications-for-fish-protection-screens_FINAL_WPA.pdf
Boys CA, Baumgartner LJ, Lowry M (2013a) Entrainment and impingement of juvenile silver perch, Bidyanus bidyanus, and golden perch, Macquaria ambigua, at a fish screen: effect of velocity and light. Fisheries Management and Ecology 20, 362-373.
| Crossref | Google Scholar |
Boys CA, Robinson W, Baumgartner LJ, Rampano B, Lowry M (2013b) Influence of approach velocity and mesh size on the entrainment and contact of a lowland river fish assemblage at a screened irrigation pump. PLoS ONE 8, e67026.
| Crossref | Google Scholar | PubMed |
Boys CA, Rayner TS, Baumgartner LJ, Doyle KE (2021) Native fish losses due to water extraction in Australian rivers: evidence, impacts and a solution in modern fish- and farm-friendly screens. Ecological Management & Restoration 22(2), 134-144.
| Crossref | Google Scholar |
Braaten PJ, Fuller DB, Holte LD, Lott RD, Viste W, Brandt TF, Legare RG (2008) Drift dynamics of larval pallid sturgeon and shovelnose sturgeon in a natural side channel of the upper Missouri River, Montana. North American Journal of Fisheries Management 28, 808-826.
| Crossref | Google Scholar |
Carter LJ, Collier SJ, Thomas RE, Norman J, Wright RM, Bolland JD (2023) The influence of passive wedge-wire screen aperture and flow velocity on juvenile European eel exclusion, impingement and passage. Ecological Engineering 192, 106972.
| Crossref | Google Scholar |
Danley ML, Mayr SD, Young PS, Cech JJ, Jr (2002) Swimming performance and physiological stress responses of splittail exposed to a fish screen. North American Journal of Fisheries Management 22, 1241-1249.
| Crossref | Google Scholar |
Faulkner H, Copp GH (2001) A model for accurate drift estimation in streams. Freshwater Biology 46, 723-733.
| Crossref | Google Scholar |
Gehrke PC (1990) Clinotactic responses of larval silver perch (Bidyanus bidyanus) and golden perch (Macquaria ambigua) to simulated environmental gradients. Marine and Freshwater Research 41, 523-528.
| Crossref | Google Scholar |
Grafton RQ (2019) Policy review of water reform in the Murray–Darling Basin, Australia: the “do’s” and “do’nots”. Australian Journal of Agricultural and Resource Economics 63, 116-141.
| Crossref | Google Scholar |
Grafton RQ, Pittock J, Davis R, Williams J, Fu G, Warburton M, Udall B, Mckenzie R, Yu X, Che N, Connell D, Jiang Q, Kompas T, Lynch A, Norris R, Possingham H, Quiggin J (2013) Global insights into water resources, climate change and governance. Nature Climate Change 3, 315-321.
| Crossref | Google Scholar |
Hammer C (1995) Fatigue and exercise tests with fish. Comparative Biochemistry and Physiology – A. Physiology 112, 1-20.
| Crossref | Google Scholar |
Humphries P, Lake PS (2000) Fish larvae and the management of regulated rivers. Regulated Rivers: Research & Management 16, 421-432.
| Crossref | Google Scholar |
Humphries P, King AJ, Koehn JD (1999) Fish, flows and flood plains: links between freshwater fishes and their environment in the Murray–Darling River system, Australia. Environmental Biology of Fishes 56, 129-151.
| Crossref | Google Scholar |
Humphries P, Serafini LG, King AJ (2002) River regulation and fish larvae: variation through space and time. Freshwater Biology 47, 1307-1331.
| Crossref | Google Scholar |
Kelso JRM, Leslie JK (1979) Entrainment of larval fish by the Douglas Point Generating Station, Lake Huron, in relation to seasonal succession and distribution. Journal of the Fisheries Research Board of Canada 36, 37-41.
| Crossref | Google Scholar |
Kieffer JD (2000) Limits to exhaustive exercise in fish. Comparative Biochemistry and Physiology – A. Molecular & Integrative Physiology 126, 161-179.
| Crossref | Google Scholar |
King AJ, Crook DA, Koster WM, Mahoney J, Tonkin Z (2005) Comparison of larval fish drift in the Lower Goulburn and mid-Murray Rivers. Ecological Management & Restoration 6, 136-139.
| Crossref | Google Scholar |
Kingsford RT (2000) Ecological impacts of dams, water diversions and river management on floodplain wetlands in Australia. Austral Ecology 25, 109-127.
| Crossref | Google Scholar |
Koehn JD, Harrington DJ (2005) Collection and distribution of the early life stages of the Murray cod (Maccullochella peelii peelii) in a regulated river. Australian Journal of Zoology 53, 137-144.
| Crossref | Google Scholar |
Kopf SM, Humphries P, Watts RJ (2014) Ontogeny of critical and prolonged swimming performance for the larvae of six Australian freshwater fish species. Journal of Fish Biology 84, 1820-1841.
| Crossref | Google Scholar | PubMed |
Lechner A, Keckeis H, Schludermann E, Humphries P, McCasker N, Tritthart M (2014) Hydraulic forces impact larval fish drift in the free flowing section of a large European river. Ecohydrology 7, 648-658.
| Crossref | Google Scholar |
McLaren JB, Tuttle LR, Jr (2000) Fish survival on fine mesh traveling screens. Environmental Science & Policy 3, 369-376.
| Crossref | Google Scholar |
McMichael GA, Vucelick JA, Abernethy CS, Neitzel DA (2004) Comparing fish screen performance to physical design criteria. Fisheries 29, 10-16.
| Crossref | Google Scholar |
Muller UK, Smit J, Stamhuis EJ, Videler JJ (2001) How the body contributes to the wake in undulatory fish swimming: flow fields of a swimming eel (Anguilla anguilla). Journal of Experimental Biology 204, 2751-2762.
| Google Scholar | PubMed |
Murray–Darling Basin Authority (2023) Murray–Darling Basin Sustainable Diversion Limits for 2022–23 water year. (Murray–Darling Basin Authority: Canberra, ACT, Australia) Available at https://www.mdba.gov.au/sites/default/files/publications/sustainable-diversion-limit-sdls-2022-2023-water-year-surface-water-calculated-9-october-2023.pdf
Ojanguren AF, Brañta F (2000) Thermal dependence of swimming endurance in juvenile brown trout. Journal of Fish Biology 56, 1342-1347.
| Crossref | Google Scholar |
Peake S (2004) Effect of approach velocity on impingement of juvenile northern pike at water intake screens. North American Journal of Fisheries Management 24, 390-396.
| Crossref | Google Scholar |
Plaut I (2001) Critical swimming speed: its ecological relevance. Comparative Biochemistry and Physiology – A. Molecular & Integrative Physiology 131, 41-50.
| Crossref | Google Scholar |
Rayner TS, Conallin J, Boys CA, Price R (2023) Protecting fish and farms: incentivising adoption of modern fish-protection screens for water pumps and gravity-fed diversions in Australia. PLoS Water 2(8), e0000107.
| Crossref | Google Scholar |
Stocks JR, Walsh CT, Rodgers MP, Boys CA (2019) Approach velocity and impingement duration influences the mortality of juvenile Golden Perch (Macquaria ambigua) at a fish exclusion screen. Ecological Management & Restoration 20, 136-141.
| Crossref | Google Scholar |
Swanson C, Young PS, Cech JJ, Jr (2004) Swimming in two-vector flows: performance and behavior of juvenile Chinook salmon near a simulated screened water diversion. Transactions of the American Fisheries Society 133, 265-278.
| Crossref | Google Scholar |
Swanson C, Young PS, Cech JJ, Jr (2005) Close encounters with a fish screen: integrating physiological and behavioral results to protect endangered species in exploited ecosystems. Transactions of the American Fisheries Society 134, 1111-1123.
| Crossref | Google Scholar |
Tonkin Z, King A, Mahoney J, Morrongiello J (2007) Diel and spatial drifting patterns of silver perch Bidyanus bidyanus eggs in an Australian lowland river. Journal of Fish Biology 70, 313-317.
| Crossref | Google Scholar |
Watson JR, Goodrich HR, Cramp RL, Gordos MA, Franklin CE (2019a) Assessment of the effects of microPIT tags on the swimming performance of small-bodied and juvenile fish. Fisheries Research 218, 22-28.
| Crossref | Google Scholar |
Watson JR, Goodrich HR, Cramp RL, Gordos MA, Yan Y, Ward PJ, Franklin CE (2019b) Swimming performance traits of twenty-one Australian fish species: a fish passage management tool for use in modified freshwater systems. bioRxiv 861898 [Preprint, published 2 December 2019].
| Crossref | Google Scholar |
Webb PW (1975) Hydrodynamics and energetics of fish propulsion. Bulletin – Fisheries Research Board of Canada 190, 1-159.
| Google Scholar |
White DK, Swanson C, Young PS, Cech JJ, Jr, Chen ZQ, Kavvas ML (2007) Close encounters with a fish screen II: delta smelt behavior before and during screen contact. Transactions of the American Fisheries Society 136, 528-538.
| Crossref | Google Scholar |
Young PS, Swanson C, Cech JJ, Jr (2010) Close encounters with a fish screen III: behavior, performance, physiological stress responses, and recovery of adult delta smelt exposed to two-vector flows near a fish screen. Transactions of the American Fisheries Society 139, 713-726.
| Crossref | Google Scholar |