Impact of severe drought on movement and survival of freshwater turtles in the Gwydir Wetlands, Australia
Annette R. Deppe
A
B
Abstract
The increasing number and length of droughts is a threat for many freshwater turtle populations.
Our study investigated the movement and survival of Emydura macquarii, Chelodina expansa and Chelodina longicollis in drought and flood conditions.
Turtles were captured in the Gwydir Wetland, New South Wales, Australia. We assessed the species composition of live captures and carcasses over the drought to assess relative survival among species. Using radiotelemetry, we compared the movement of E. macquarii and C. longicollis over a severe drought followed by a flood.
For tracked turtles with known fates, 28.6% of E. macquarii and 7.1% of C. longicollis, died during the drought. The proportional composition of captured turtles compared with those of desiccated shells suggested that C. expansa had the highest mortality. E. macquarii and C. longicollis both appeared to navigate terrestrially and moved further in the flood water than in the drought, but C. longicollis moved further generally.
Our findings suggest that turtles in isolated wetlands of the northern Murray–Darling Basin are at high risk of mortality during severe drought.
Environmental water delivery during periods of sustained drought will be critical to ensure persistence of populations of long-lived species such as turtles.
Keywords: Chelodina expansa, Chelodina longicollis, drought, Emydura macquarii, flood, movement, radio transmitter, refuge, survival, tracking, turtle, wetland.
Introduction
Increased wetland drying resulting from climate change and extraction of water negatively affects freshwater biodiversity (Kingsford 2000; Dudgeon et al. 2006; Aldous et al. 2011). Over-allocation of water and prolonged drought reduce the quality of floodplain environments by decreasing the extent and the resilience of wetland vegetation (Kingsford 2000; Bunn and Arthington 2002). Furthermore, wetland loss has caused declines in populations of wetland fauna such as frogs (Wassens et al. 2013), birds (Campos-Cerqueira and Aide 2021) and fishes (Chessman 2013). Many Australian freshwater turtle species also use floodplains and wetlands to sustain populations (Chessman 1988; Fordham et al. 2008; Bouma et al. 2020).
Turtles are declining globally (Dudgeon et al. 2006; Kingsford et al. 2011) and are likely to be negatively influenced by reduced periods of inundation (Gibbons et al. 1983; Chessman 2011; Stanford et al. 2020), which influences both survival and movement of freshwater turtles, as a lack of water can cause dehydration (Chessman 2018), reduce growth rate (Ferronato et al. 2017), lower reproduction (Kennett and Georges 1990), increase risk of predation (Chessman 2011) and decrease survival (Purcell et al. 2017; Beard and Powell 2023). Aquatic freshwater turtles can respond to drought by congregating in refuge pools where there is high competition for food (Meathrel et al. 2002), leaving the pool in search of another more permanent refuge (Roe and Georges 2008) or estivating terrestrially (Ligon and Stone 2003). If the refuge pool dries completely, some highly aquatic turtles can emigrate terrestrially (Cann and Sadlier 2017), exposing them to desiccation and terrestrial predators (Chessman 2011), or bury themselves in the mud of the dried waterbody (Gibbons et al. 1983).
Despite extensive extraction of water and increased drought severity in the Murray–Darling Basin, we still lack an understanding of the impact of floodplain drying on freshwater turtles (Aldous et al. 2011; Francis et al. 2022). Many knowledge gaps still exist for freshwater turtles, such as movement cues, cognitive abilities and baseline ecological information (Bower et al. 2023). Different species have different habitat requirements, including lakes, rivers and ephemeral water bodies (Chessman 1988), and this suggests that they have differing responses to hydrological change. During drought conditions, the decrease or loss of refuge pools can have a major effect on freshwater turtles, especially for highly aquatic species that are not adapted for terrestrial survival (Gibbons et al. 1983; Chessman 2011). The threat of prolonged drought in areas where alternative refuge pools are not available makes the survival of isolated populations tenuous (Purcell et al. 2017). Understanding the needs of freshwater turtles will further our ability to manage wetlands and increase the survival prospects of Emydura macquarii, Chelodina expansa and Chelodina longicollis.
Here, we examined the behaviour of turtles during a severe drought in the northern Murray–Darling basin, with the aims of (1) comparing survival of three species of turtle during severe drought and (2) comparing the movement of a highly aquatic species (E. macquarii) and an aquatic species that is known to frequently migrate and estivate terrestrially (C. longicollis). This study illustrated the resourcefulness of freshwater turtles in movement and survival during a severe drought, while also highlighting the need for continued conservation effort.
Materials and methods
Study species
Emydura macquarii, C. longicollis and C. expansa are freshwater turtle species broadly distributed on the eastern coast of Australia (Chessman 2018). Emydura macquarii and C. expansa are highly aquatic turtles that generally inhabit rivers and wetlands or tributaries that are close to a main channel (Chessman 1988; Van Dyke et al. 2019). Emydura macquarii prefers water more than 2 m deep (Chessman 1988) and C. expansa is capable of moving extensively in a river system (Bower et al. 2012). Chelodina longicollis also requires water, but they generally inhabit shallow or ephemeral bodies of water (such as networks of farm dams) and frequently migrate or estivate terrestrially (Chessman 1988, 2022; Graham et al. 1996). Chelodina longicollis is also active through larger changes in temperature and has a lower level of evaporative weight loss than do E. macquarii and C. expansa (Chessman 1984). These three freshwater species are sympatric in the waterbodies of the Gwydir Wetland State Conservation Area.
Study area
The Gwydir Wetland State Conservation Area is located in the lower Gingham Watercourse north-west of Moree, in New South Wales, Australia. It receives an average annual rainfall of 585 mm, and summer temperatures often exceed 35°C (Bureau of Meteorology 2022). Our study encompassed the period from November 2019 through October 2020, during a portion of the driest 4 years on record for this area (Commonwealth Environmental Water Office 2021). Conditions were generally dry and hot, with the temperature on 22 December 2019 reaching a record 45.9°C and only 125.4 mm of rainfall in 2019 (Bureau of Meteorology 2019). This area was in severe drought offering a unique opportunity to study the behaviour of the three species of freshwater turtle that inhabited the drying wetland.
We trapped turtles at two locations within the Gingham Watercourse in the Gwydir Wetland State Conservation Area (Fig. 1). Sites were chosen for their accessibility and availability of water. The first site, Gingham Waterhole, is a natural pool surrounded by cumbungi (Typha orientalis), marsh club-rush (Bolboschoenus fluviatilis), lignum (Muehlenbeckia florulenta), and river cooba (Acacia stenophylla) shrubland, coolabah (Eucalyptus coolabah) and river red gum (Eucalyptus camaldulensis) woodland. At the start of our study, 8 November 2019, the Gingham Waterhole had retracted from a full-capacity area of 57,873 m2 and a depth of ~2 m to a small pool with an area of 1116 m2 and a depth of 0.19 m. Under this thin layer of turbid water was a thick layer of mud that ranged in depth from 0.25 to 0.92 m. Three temperatures were taken at Gingham Waterhole (on 10, 14 and 19 November 2019), contrasting the temperature of the water, which was 35, 30 and 37°C, with that of the mud at 22, 24 and 22°C (based on measurements from a Holman Weather Whiz Wireless Weather Reader, Holman Industries, Perth, WA, Australia, and a Dreambaby Room and Bath Thermometer, Tee-Zed Products Pty Ltd, Sydney, NSW, Australia). On 13 December 2019, the pool surface dried completely, and the area remained without water until 17 February 2020 (66 days), when the Gingham Waterhole began filling to overflowing from an inflow.
Study sites at Gingham Waterhole and Moolaboola Dam within the Gwydir Wetlands State Conservation Area, in the Lower Gwydir Wetlands of New South Wales, Australia. Adapted from the Gwydir Selected Area 2021–2022 Annual Summary Report – Appendix A. (Department of Climate Change, Energy, the Environment and Water 2023).
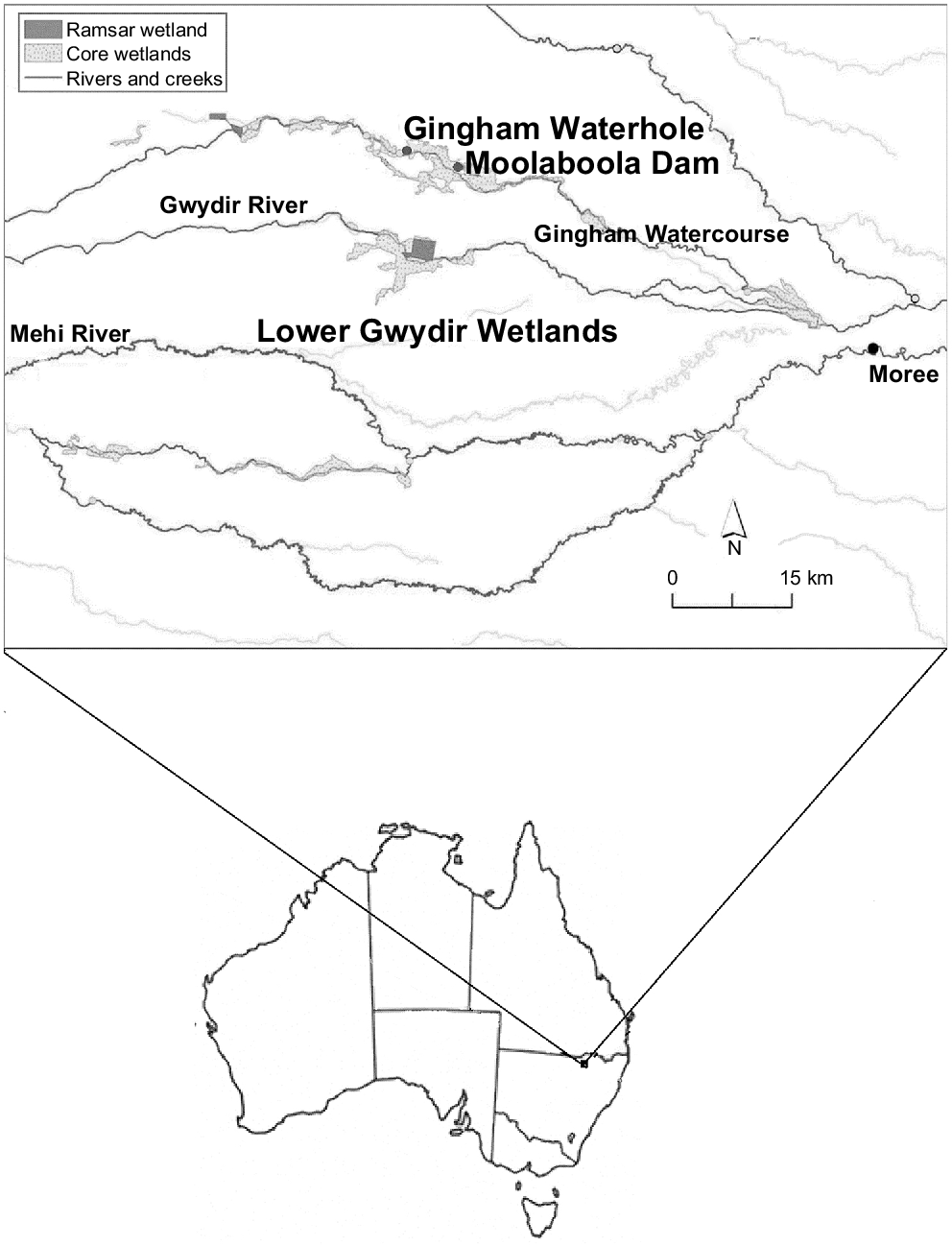
The second site, Moolaboola Dam, was an artificial dam 7 km east of the Gingham Waterhole, on the same watercourse. It was surrounded by cumbungi (T. orientalis), marsh club-rush (B. fluviatilis) and swamp wallaby grass (Amphibromus nervosus). Approximately 200 m to the north was a coolabah (E. coolabah) woodland area. Moolaboola Dam did not dry completely during our study period, but evaporated to an area of 2300 m2. On 29 January 2020, an inflow of water through the channels and across the floodplain in the Gingham Wetlands refilled the dam past its capacity area of 4200 m2. The water came from two sources, including water released from the upstream Copeton Dam and runoff from higher-rainfall areas further upstream.
Study methods
Turtles were captured from 8 to 20 November 2019 at Gingham Waterhole and from 9 December 2019 to 5 January 2020 at Moolaboola Dam. The low water level in Gingham Waterhole made it difficult to place traps successfully; so, all but three individual turtles (C. longicollis) were captured by hand (muddling hours = 13) (Limpus 2008). On 31 January 2020, we observed exposed, desiccated turtle carapaces or plastrons on the surface of the cracked mud within a radius of 45 m of the last-refuge pool (search hours = 3). In Moolaboola Dam, water levels were sufficient to deploy three cathedral traps (modified square 3-cm mesh crab traps, two openings 0.5 × 0.25 m each) and two fyke nets (1-m diameter, 3-cm mesh, double throat, two 15-m wings) baited with beef liver (trap hours = 1034).
A total of 16 E. macquarii (15 from Gingham Waterhole and 1 from Moolaboola Dam) and 15 C. longicollis (9 from Gingham Waterhole and 6 from Moolaboola Dam) individuals were fitted with radio transmitters (Advanced Telemetry Systems Series R1600, Isanti, MN, USA) and tracked from 8 November 2019 to 5 January 2020. Transmitters were attached to the carapace by using marine epoxy resin, and they were covered with black silicone for camouflage. The resin and silicone were allowed to dry for 30 min before releasing the turtles.
Turtles were tracked using a hand-held scanning receiver (Advanced Telemetry Systems, ATS, Model R410, Isanti) and a folding three-element Yagi receiving antenna (Model F150-3FB, ATS). The turtles were tracked as regularly as possible; however, because of unavoidable circumstances, such as the evacuation of the reserve because of fire warnings or aerial pig shootings, tracking frequency varied between once a day and once every 2 weeks from November 2019 to May 2020. A final tracking period was completed from 4 to 7 October 2020. In drought, turtles could be detected from up to 1 km away, but during flooding (i.e. once underwater) they could not be detected if they were more than ~500 m away. A turtle’s carapace could be sighted at terrestrial locations, or a bump on the ground where they had dug under the soil and leaflitter could show their location. Terrestrial fixes were recorded to an accuracy of 3.65 m by using a hand-held GPS (Garmin GPSMAP 64sx, Olathe, KS, USA), but when turtles were in water, estimates of their locations were less accurate, because they could not be sighted.
Statistical analyses
We examined both the effects of the hydrological state (drought or flood) and species (E. macquarii, C. longicollis) on the daily displacement (i.e. the minimum daily movement based on a straight line between points) of turtles. To determine the effect of waterhole drying during the drought, we subset our data to 23 turtles from Gingham Waterhole (24 turtles were tracked, but 1 turtle was excluded because it did not move from the mud even after water flooded the waterhole, so was assumed to have died). R statistical software was used (ver. 4.2.2, R Foundation for Statistical Computing, Vienna, Austria, see https://www.r-project.org/) with the following packages: ggplot2 (ver. 3.4.0, see https://CRAN.R-project.org/package=ggplot2; Wickham 2016), performance (ver. 0.10.2, see https://cran.r-project.org/package=performance; Lüdecke et al. 2021), car (ver. 3.1-1, see https://CRAN.R-project.org/package=car; Fox and Weisberg 2019) and lme4 (ver. 1.1-32, see https://CRAN.R-project.org/package=lme4; Bates et al. 2015). Our data were not normally distributed, so we used a mixed-effects negative binomial model with metres moved in a straight line from the previous fix as the response variable. The fixed effects were turtle species, drought conditions (before or after the arrival of water) and the interaction between these factors. Because the number of days between fixes varied, we limited the data to points with a maximum interval of 30 days between fixes, and included the natural log of the number of days from the previous fix, as an offset. The model was assessed with a Type II ANOVA and a significance level of 0.05.
To determine whether the tracked turtles moved randomly, the bearings of their tracks were analysed by testing the uniformity of the circular spread of fixes around the individual wetlands by using the Rayleigh Test of Uniformity (ver. 0.4-95, C. Agostinelli and U. Lund, see https://rdrr.io/cran/circular/man/rayleigh.test.html), with the mean bearing of each individual (from its original location in Gingham Waterhole or Moolaboola Dam) as the response. The following packages were used: dplyr (ver. 1.1.2, H. Wickham, R. Francois, L. Henry, K. Muller and D. Vaughan, see https://CRAN.R-project.org/package=dplyr), circular (ver. 0.4-95, U. Lund, C. Agostinelli, H. Arai, A. Gagliardi, E. Garcia-Portugues, D. Giunchi, J. Irisson, M. Pocernich and F. Rotolo, see https://CRAN.R-project.org/package=circular), sp (ver. 2.0-0, see https://cran.r-project.org/package=sp; Pebesma and Bivand 2005) and geosphere (ver. 1.5-18, R. J. Hijmans, C. Karney, E. Williams and C. Vennes, see https://cran.r-project.org/package=geosphere). We conducted a separate test for each species at each waterhole in each hydrological state (Gingham Waterhole or Moolaboola Dam in drought or flood).
To test for interspecific differences in the ability to survive a drying waterhole, we used a Pearson’s Chi-Square test to compare the number of each species captured at Gingham Waterhole in November 2019 (when it had water) to the number of corresponding desiccated shells found in January 2020 (when it was dry). The survivorship of the tracked turtles at Gingham Waterhole was also tested for the period from transmitter attachment to 7 October 2020, by using a log rank Kaplan–Meier estimate. The packages used were: readr (ver. 2.1.5, H. Wickham, J. Hester and J. Bryan, see https://cran.r-project.org/package=readr), survival (ver. 3.5.0, T. M. Therneau, see https://cran.r-project.org/package=survival; Therneau and Grambsch 2000) and survminer (ver. 0.4.9, A. Kassambara, M. Kosinski and P. Biecek, see https://cran.r-project.org/package=survminer). Moolaboola Dam was not included in this test because it held water for the entire study period.
Animal research ethics
Research was conducted under the University of New England’s Ethics Permit AEC19-081, a scientific licence from the New South Wales Government Department of Planning, Industry and Environment Licence Number SL1022308, and the New South Wales Government Department of Primary Industries Scientific Collection Permit Number P19/0047-1.0. Every effort has been made to acknowledge any owners of copyright material.
Results
Tracked-turtle movement
Emydura macquarii and C. longicollis responded differently to the drying of the Gingham Waterhole. During the drought, the E. macquarii at Gingham Waterhole travelled significantly less per day, on average, than did the C. longicollis (F = 4.87, P = 0.027; E. macquarii mean 3.19, median 0.00 and s.d. 27.03 m day−1; C. longicollis mean 16.81, median 0.00 and s.d. 60.64 m day−1; Fig. 2). Both species increased their movements once flood waters arrived (F = 6.81, P = 0.009; E. macquarii mean 38.65, median 5.45 and s.d. 116.85 m day−1; C. longicollis mean 37.37, median 60.06 and s.d. 67.80 m day−1), and there was no significant interaction between species and hydrological state (F = 0.67, P = 0.403).
Box plot of tracked Emydura macquarii and Chelodina longicollis daily displacement (i.e. the minimum daily movement based on a straight line between points) at Gingham Waterhole and Moolaboola Dam.
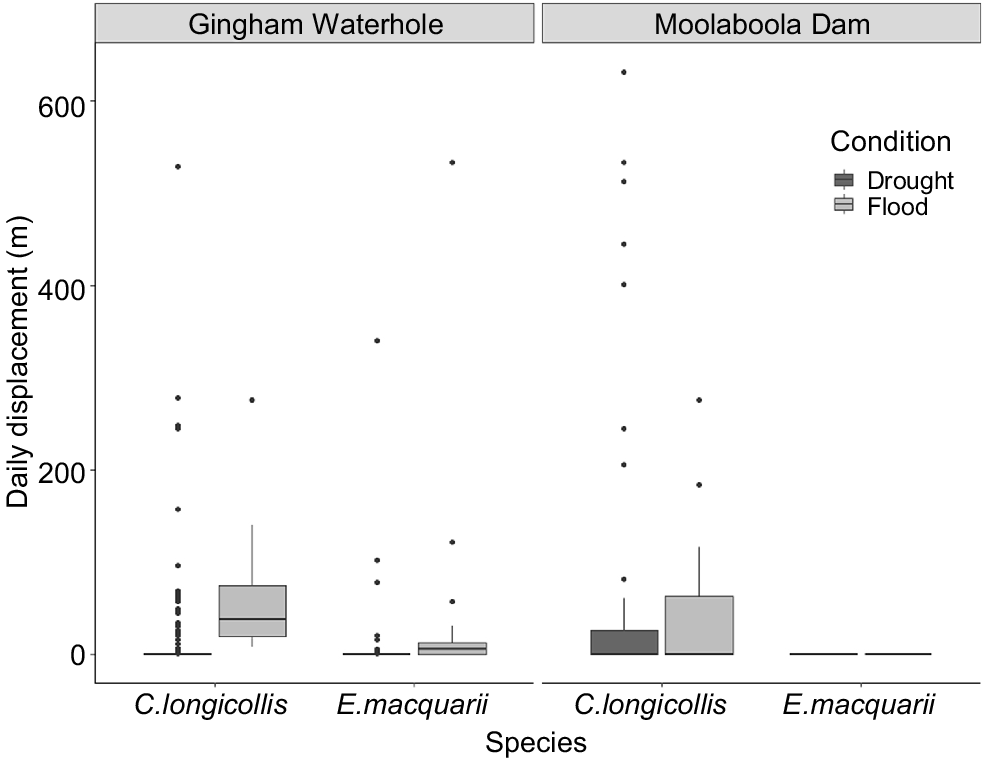
Of the 15 E. macquarii individuals tracked at Gingham Waterhole, all remained in the mud of the waterhole at least until all surface water dried completely (13 December 2019). In total, 5 E. macquarii individuals never left the waterhole and remained in the mud until water returned after 102 days (66 of these days had no surface water and 1 of the E. macquarii died in the mud). The remaining 10 E. macquarii individuals left the Gingham Waterhole after it dried. Three individuals died on their first move out of the mud and were found desiccated at a short distance (13, 74 and 122 m) from their previous location in the mud. Predators, such as sea eagles (Haliaeetus leucogaster) and foxes (Vulpes vulpes), were observed in the area, and scavengers such as goannas (Varanus varius), pigs (Sus scrofa) and crows (Corvus spp.) were also frequently seen. Two of the E. macquarii individuals still had their desiccated heads, one with four limbs and the other with only one. The third turtle was found consumed, and the carapace and plastron had been separated.
Of the seven E. Macquarii individuals that remained alive after climbing out of the mud, one walked terrestrially up the Gingham Watercourse for 1.7 km to an artificial dam that retained water throughout the drought. Four E. macquarii individuals went out of range of the transmitter during the drought, but two returned when Gingham Waterhole filled with water. One E. macquarii sheltered in the large cracks created by the drying mud under a clump of hairy carpet weed (Glinus lotoides) in the bed of Gingham Waterhole, ~80 m from the last refuge pool. This turtle made two small movements in the weeds (2 and 4 m), but stayed in that general location for a total of 28 days. Another E. macquarii individual moved out of the mud to hide under cumbungi straw (T. orientalis) on the edge of Gingham Waterhole, ~85 m from where the last pool of surface water had been. This individual E. macquarii changed location under the straw after 8 days, remaining in this new location under the straw for 19 days until water returned.
The E. macquarii at Gingham Waterhole had directional movement during the drought, often moving in a south-easterly direction at an average bearing of 127° from the waterhole (test statistic = 0.88, P > 0.001), but it did not have a consistent directional pattern in movement during the flood (test statistic = 0.18, P = 0.800). The one E. macquarii individual tracked in Moolaboola Dam stayed in the dam until water returned, then moved out onto the flooded plain.
Chelodina longicollis turtles were more mobile than were E. macquarii. All the tracked C. longicollis individuals at the Gingham Waterhole left the drying waterhole before the water receded to 0.07 m in depth, and they frequently changed terrestrial locations in the vicinity of the waterhole throughout the drought. Chelodina longicollis were generally found in particular microhabitats, such as buried in soil and leaflitter or under or near a log or logs, usually in the shade of covering tree canopy. They rested terrestrially in one location for an average of 33 days before relocating; however, one individual estivated for 84 days in one place and another in one location for 67 days. Chelodina longicollis turtles at Gingham Waterhole had a pattern in their terrestrial movement around the dried waterhole, often moving north-east at an average bearing of 37° from the waterhole during the drought (test statistic = 0.61, P = 0.0301). They continued with a consistent pattern of movement during the flood, generally moving at an average bearing of 76° while frequenting the floodplain and its channels (test statistic = 0.84, P = 0.003).
At Moolaboola Dam, one C. longicollis individual stayed in the water of the dam throughout the drought and two moved onto land until floodwater arrived. The remaining three C. longicollis individuals repeatedly moved between the water in the dam and terrestrial locations, then moved into the floodwater when it arrived. Chelodina longicollis at Moolaboola Dam showed a directional pattern, commonly moving in an easterly direction at an average bearing of 103° from the dam during the drought (test statistic = 0.998, P < 0.001), and during the flood the tracked C. longicollis moved at an average bearing of 96° (test statistic = 0.99, P = 0.001).
Survivorship of turtle species
A total of 70 individual turtles were captured at Gingham Waterhole in November 2019 (before it completely dried), and 36 desiccated turtle shells were observed within the dried Gingham Waterhole in January 2020. Of the 36 desiccated turtle shells, 29 were identifiable to species and included 16 C. expansa individuals (55%), 11 E. macquarii individuals (38%) and 2 C. longicollis individuals (7%). By contrast, the distribution of species captured in traps at the waterhole was 20 C. expansa individuals (28%), 39 E. macquarii individuals (56%) and 11 C. longicollis individuals (16%). The difference in species assemblages was significant (χ2 = 6.49, P = 0.039), with C. expansa being disproportionately more represented in the desiccated shells (Fig. 3).
Species composition of live turtles captured in Gingham Waterhole before drying and desiccated shells observed in the dried waterhole.
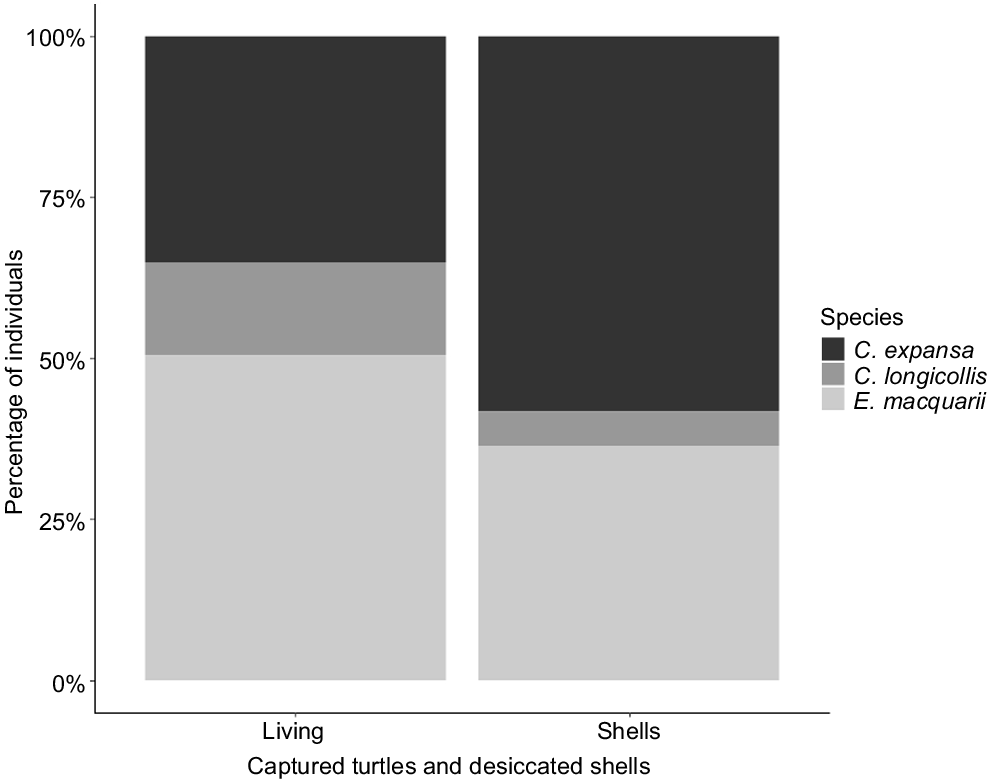
Of the 15 C. longicollis individuals tracked, 13 were confirmed alive at the end of our study, 1 died and 1 either moved out of range or the transmitter failed. Of the 16 E. macquarii individuals tracked, 10 were confirmed alive at the end of the study, 4 died and 2 were out of range or the transmitters failed. The difference in survivorship between C. longicollis and E. macquarii was not statistically significant (χ2 = 1.63, P = 0.22); however, sample sizes were small (Table 1, Fig. 4).
Item | Stayed in water | Sought refuge on land | Stayed buried in mud | Total | |
---|---|---|---|---|---|
Gingham Waterhole | |||||
E. macquarii | 0 | 10 (3 died) | 5 (1 died) | 15 | |
C. longicollis | 0 | 9 (1 died) | 0 | 9 | |
Moolaboola Dam | |||||
E. macquarii | 1 | 0 | 0 | 1 | |
C. longicollis | 1 | 5 | 0 | 6 |
Discussion
Movement strategies
The tracked E. macquarii and C. longicollis turtles showed different behavioural strategies for surviving a severe drought, as has also been found between other species, reflective of their different ecologies (Gibbons et al. 1983; Chessman 2018). Terrestrial estivation of E. macquarii has not been previously recorded, and they generally travel on land only to nest (Chessman 1984) or once a waterbody has dried (Cann and Sadlier 2017). Here, we demonstrated that during the drying out of the Gingham Waterhole, one-third of the tracked E. macquarii individuals stayed in the mud at the bottom of the waterhole. This is a strategy that has been observed in terrestrially migratory and estivating Australian turtles such as Chelodina rugosa (Fordham et al. 2008) and aquatic cryptodirans such as Sternotherus odoratus in South Carolina (Gibbons et al. 1983). Four of the five E. macquarii individuals that stayed in the mud at Gingham Waterhole survived with no surface water for 66 days until water returned (the fifth died), suggesting that this strategy is moderately successful in the short term. Other sites, such as Moolaboola Dam, did not have a thick layer of mud, so the success of this strategy may be limited to waterholes with specific qualities.
During the drought, the majority of tracked E. macquarii individuals left the drying waterhole, a behaviour that has been previously observed anecdotally in Emydura macquarii emmotti (Cann and Sadlier 2017). Turtles that attempted to relocate by moving terrestrially across the floodplain had higher mortality rates than did those that stayed in the waterbody, and the success of this strategy is likely to be dependant on the distance to the nearest waterbody and the condition of the individual turtle (Roe et al. 2008). Some individuals moved only a short distance before dying. There is the possibility they may have already been in low body condition or dehydrated, or they may have left at dangerously hot times of day, reiterating the risks of leaving a waterhole during drought (Chessman 1984; Roe et al. 2008). Emydura macquarii travelled further once water was available, moving throughout the flooded area, as has previously been found in closely related E. m. emmotti (McKnight et al. 2023). This movement in flooded areas demonstrates the importance of intermittent flows and aquatic connections for the dispersal of aquatic turtles.
Unlike E. macquarii, C. longicollis in the Gingham Waterhole moved onto land when the water became shallow. In the Gingham Waterhole, C. longicollis left the waterhole before it dropped to 0.7 m, as in a previous study where the number of C. longicollis in a wetland decreased as the water level dropped from 1.5 to 0.8 m (Kennett and Georges 1990). In the Gwydir Wetlands, most C. longicollis individuals did not stay in one estivation site, but moved frequently throughout the drought. Their choice of resting locations demonstrated a preference for particular microhabitats akin to other studies that have found that turtles such as C. longicollis, Clemmys guttata and Deirochelys reticularia miaria are selective in choosing estivation sites (Milam and Melvin 2001; Rees et al. 2009; McKnight and Ligon 2020). This suggests that maintenance of floodplain vegetation that offers cover during times of drought is important to freshwater turtles and echoes previous work on the need for maintaining large buffer zones around wetlands protecting them from activities such as land clearing (Semlitsch and Bodie 2003; McKnight and Ligon 2020).
Behaviour of C. longicollis in the Gingham Waterhole is consistent with previous evidence that the species is purposeful in its movement when travelling terrestrially (Stott 1987; Dalem 1998; Roe et al. 2008). Both C. longicollis and E. macquarii moved in a directional pattern, demonstrating an ability to navigate. The terrestrial movement was not constrained, as the topography is very flat (average gradient ~0.001) and the E. macquarii tended to follow the water channels, whereas the C. longicollis used the more thickly wooded area suitable for estivation. Chelodina longicollis turtles can orient their travel over distances of more than 2 km as they move among wetlands (Graham et al. 1996). In our study, only one C. longicollis individual travelled more than 2 km during the drought and none of the C. longicollis individuals relocated to another waterhole. This suggests that the separation of waterbodies by expanses of arid land reduces the propensity of attempted overland movement to other wetlands. This lack of a network of waterholes will make these turtles more vulnerable to extended drought than are turtles in areas with more aquatic refugia. However, during the flood, C. longicollis was able to travel extensively in the water by using the shallow flooded areas, and these times of flood are important times for feeding and migration (Kennett and Georges 1990).
Chelodina longicollis individuals tracked at Moolaboola Dam, where water provided a consistent aquatic refuge, were not forced to remain on land for the duration of the drought as they were at Gingham Waterhole. Chelodina longicollis is less likely to cohabit permanent waterbodies with E. macquarii and C. expansa when other wetlands are available, possibly because of food competition (Chessman 1988; Beard and Powell 2023), and when animal numbers exceed the carrying capacity of a waterbody, C. longicollis turtles have low bodyweight (Kennett and Georges 1990). Chelodina longicollis turtles feed only aquatically and cannot replenish their energy reserves when estivating, which limits the length of time they can travel or estivate (Chessman 2011). Turtles with good body condition may estivate more than those that have lower fat reserves (Dalem 1998). Individual turtle behaviour is altered by the perceived cost of movement in an isolated unpredictable environment and the likelihood that an individual will estivate, and the time it spends estivating, increases as distance to permanent water increases (Roe and Georges 2008). Individual choices to stay in a drying wetland or move out to estivate and migrate become more unpredictable as isolation of the wetland increases (Roe and Georges 2008). In this case, all strategies used by C. longicollis at Moolaboola Dam were successful in that none of the tracked turtles died.
Survival in drought
Chelodina longicollis turtles have adaptations, such as having low evaporative water loss and the ability to reabsorb water from their bladder, that allows them to estivate terrestrially (Chessman 1984). They can survive on land longer than can E. macquarii and C. expansa (Chessman 1984), and this ability to estivate when water is not available may have resulted in lower mortality for C. longicollis than for E. macquarii at Gingham Waterhole. However, the difference was not statistically significant and the higher ability of C. longicollis to move about freely on land may have reduced our ability to detect carcasses near the last refuge pool. Mark–recapture studies over long periods would be useful to document the effects of disappearing refugia and better grasp the population-level impacts of drought.
The relatively over-represented carcasses of C. expansa suggest that they may be less tolerant of extended drought than are other species. Chelodina expansa turtles are a highly aquatic animal that can travel long distances aquatically (Bower et al. 2012), but is rarely observed on land outside of the nesting season (Chessman 1988), although their evaporative water loss is intermediate between that of C. longicollis and that of E. macquarii (Chessman 1984). Chelodina expansa is one of the largest Australian species of freshwater turtle and has a lower abundance than do C. longicollis and E. macquarii (Chessman 2011; Bower et al. 2012; Van Dyke et al. 2019). Chelodina expansa appears well placed to withstand a drought by remaining in a refuge pool, but in our study, the refuge pool dried, and C. expansa shells were predominant in the carcasses observed around the waterhole, suggesting they may attempt to leave once water has evaporated. As there were predators and scavengers at Gingham Waterhole, it is also possible that their size made it difficult to carry away or they were a larger target and easier to catch than were other species, making them the predominant carcass observed around the area. Long-term studies are needed to determine the effect increased drought duration and drying of waterholes may have on C. expansa.
Our data demonstrated that C. longicollis and E. macquarii in the Gwydir Wetland State Conservation Area have different behavioural responses to drought, and the majority of individuals of both species survived until waters returned. Nevertheless, increases in the frequency or severity of these droughts as a result of climate change present a significant problem, because turtles have generally evolved a life-history strategy that relies on low adult mortality (Spencer 2018), and many dead individuals were observed during the drought at Gingham Waterhole. Further, 28.6% of our tracked E. macquarii adults (with a known fate) died, and adult mortality rate is likely to be unsustainable (Spencer and Thompson 2005), especially if droughts become more frequent. Additionally, if the drought had continued longer, the mortality rate of turtles would have likely increased. Environmental flows are a critical tool to deliver water during periods of waterbody drying and allocating water to deliver to terminal wetlands will be paramount to enabling the survival of long-lived species, such as turtles, during future droughts.
Declaration of funding
The University of New England, Faculty of Science, Agriculture, Business and Law supplied funding for equipment and travel and the Commonwealth Environmental Water Holder provided funding for radio-telemetry equipment. The research was conducted with the support of the Australian Government Research Training Program Scholarship to A. R. Deppe.
Acknowledgements
We thank Sarah Mika, Mark Southwell, Darryn Ryder and Paul Frazier for assistance with the project. We also thank Johann Brueggemann, Michael Spinaze and David Preston from the New South Wales National Parks and Wildlife Service and Jane Humphries with the New South Wales Office of Environment and Heritage for their assistance with the project. We acknowledge the Gamilaroi nation as the traditional custodians of the land where the research was completed.
References
Aldous A, Fitzsimons J, Richter B, Bach L (2011) Droughts, floods and freshwater ecosystems: evaluating climate change impacts and developing adaptation strategies. Marine and Freshwater Research 62(3), 223-231.
| Crossref | Google Scholar |
Bates D, Mächler M, Bolker B, Walker S (2015) Fitting linear mixed-effects models using lme4. Journal of Statistical Software 67(1), 1-48.
| Crossref | Google Scholar |
Beard AN, Powell LA (2023) Drought influences annual survival of painted turtles in Western Nebraska. Diversity 15, 597.
| Crossref | Google Scholar |
Bouma A, Kuchling G, Zhai SY, Mitchell N (2020) Assisted colonisation trials for the western swamp turtle show that juveniles can grow in cooler and wetter climates. Endangered Species Research 43, 75-88.
| Crossref | Google Scholar |
Bower DS, Hutchinson M, Georges A, Hayssen V (2012) Movement and habitat use of Australia’s largest snake-necked turtle: implications for water management. Journal of Zoology 287(1), 76-80.
| Crossref | Google Scholar |
Bower DS, McKnight DT, Sullivan K, Macdonald SL, Georges A, Clulow S, Mathwin R, Connell MJ, Nelson HV, Santoro A, Nordstrom B, Van Dyke JU, Kidman RA, Streeting LM, Dillon ML, Spencer R-J, Thompson MB, Nordberg EJ (2023) Opportunities for research and conservation of freshwater turtles in Australia. Austral Ecology 48, 1483-1491.
| Crossref | Google Scholar |
Bunn SE, Arthington AH (2002) Basic principles and ecological consequences of altered flow regimes for aquatic biodiversity. Environmental Management 30(4), 492-507.
| Crossref | Google Scholar | PubMed |
Bureau of Meteorology (2019) New South Wales in 2019: record warm and record dry. Thursday, 9 January 2020 – Annual Climate Summary for New South Wales – Product code IDCKGC55R0. (The Bureau) Available at http://www.bom.gov.au/climate/current/annual/nsw/archive/2019.summary.shtml [Verified 9 June 2023]
Bureau of Meteorology (2022) Climate of Moree. (The Bureau) Available at http://www.bom.gov.au/nsw/moree/climate.shtml [Verified 9 June 2023]
Campos-Cerqueira M, Aide TM (2021) Impacts of a drought and hurricane on tropical bird and frog distributions. Ecosphere 12(1), e03352.
| Crossref | Google Scholar |
Chessman BC (1984) Evaporative water loss from three south-eastern Australian species of water turtle. Australian Journal of Zoology 32(5), 649-655.
| Crossref | Google Scholar |
Chessman BC (1988) Habitat preferences of fresh-water turtles in the Murray Valley, Victoria and New South Wales. Australian Wildlife Research 15(5), 485-491.
| Crossref | Google Scholar |
Chessman BC (2011) Declines of freshwater turtles associated with climatic drying in Australia’s Murray–Darling basin. Wildlife Research 38(8), 664-671.
| Crossref | Google Scholar |
Chessman BC (2013) Identifying species at risk from climate changes: traits predict the drought vulnerability of freshwater fishes. Biological Conservation 160, 40-49.
| Crossref | Google Scholar |
Chessman BC (2018) Effects of temperature and exercise on metabolism of three species of Australian freshwater turtles: implications for responses to climate change. Australian Journal of Zoology 66, 317-325.
| Crossref | Google Scholar |
Chessman BC (2022) The value of artificial farm ponds to Australian eastern long-necked turtles. Hydrobiologia 849, 113-120.
| Crossref | Google Scholar |
Commonwealth Environmental Water Office (2021) Gwydir booms again in 2021. (Department of Climate Change, Energy, the Environment and Water) Available at https://www.dcceew.gov.au/water/cewo/publications/gwydir-booms-again-2021 [Verified 9 June 2023]
Department of Climate Change, Energy, the Environment and Water (2023) Gwydir selected area 2021–22 annual summary report – Appendix A: Gwydir River hydrology. (DCCEEW) Available at https://www.dcceew.gov.au/sites/default/files/documents/gwydir-river-selected-area-2021-22-annual-summary-report-appendix-a.pdf [Verified 27 April 2023]
Dudgeon D, Arthington AH, Gessner MO, Kawabata Z-I, Knowler DJ, Lévêque C, Naiman RJ, Prieur-Richard A-H, Soto D, Stiassny MLJ, Sullivan CA (2006) Freshwater biodiversity: importance, threats, status and conservation challenges. Biological Reviews 81(2), 163-182.
| Crossref | Google Scholar | PubMed |
Ferronato BO, Roe JH, Georges A (2017) Responses of an Australian freshwater turtle to drought-flood cycles along a natural to urban gradient. Austral Ecology 42, 442-455.
| Crossref | Google Scholar |
Fordham DA, Georges A, Brook BW (2008) Indigenous harvest, exotic pig predation and local persistence of a long-lived vertebrate: managing a tropical freshwater turtle for sustainability and conservation. Journal of Applied Ecology 45, 52-62.
| Crossref | Google Scholar |
Francis RJ, Brandis KJ, Chessman BC, Slavich E, Kingsford RT (2022) Abundance and nutritional status of freshwater turtles to guide delivery of environmental flows in the Murray–Darling Basin, south-eastern Australia. Aquatic Conservation: Marine and Freshwater Ecosystems 32(2), 282-293.
| Crossref | Google Scholar |
Gibbons JW, Greene JL, Congdon JD (1983) Drought-related responses of aquatic turtle populations. Journal of Herpetology 17(3), 242-246.
| Crossref | Google Scholar |
Graham T, Georges A, McElhinney N (1996) Terrestrial orientation by the eastern long-necked turtle, Chelodina longicollis. from Australia. Journal of Herpetology 30(4), 467-477.
| Crossref | Google Scholar |
Kennett RM, Georges A (1990) Habitat utilization and its relationship to growth and reproduction of the eastern long-necked turtle, Chelodina longicollis (Testudinata: Chelidae) from Australia. Herpetologica 46(1), 22-33.
| Google Scholar |
Kingsford RT (2000) Ecological impacts of dams, water diversions and river management on floodplain wetlands in Australia. Austral Ecology 25, 109-127.
| Crossref | Google Scholar |
Kingsford RT, Walker KF, Lester RE, Young WJ, Fairweather PG, Sammut J, Geddes MC (2011) A Ramsar wetland in crisis – the Coorong, Lower Lakes and Murray Mouth, Australia. Marine and Freshwater Research 62, 255-265.
| Crossref | Google Scholar |
Ligon DB, Stone PA (2003) Radiotelemetry reveals terrestrial estivation in sonoran mud turtles (Kinosternon sonoriense). Journal of Herpetology 37(4), 750-754.
| Crossref | Google Scholar |
Limpus C (2008) Freshwater turtles in the Mary River: review of biological data for turtles in the Mary River, with emphasis on Elusor macrurus and Elseya albagula. (Queensland Government) Available at https://www.qld.gov.au/__data/assets/pdf_file/0018/314550/mary-river-turtles.pdf [Verified 17 January 2024]
Lüdecke D, Ben-Shachar MS, Patil I, Waggoner P, Makowski D (2021) Performance: an R package for assessment, comparison and testing of statistical models. Journal of Open Source Software 6(60), 3139.
| Crossref | Google Scholar |
McKnight DT, Ligon DB (2020) Estivation site selection of western chicken turtles (Deirochelys reticularia miaria). The Southwestern Naturalist 64(3-4), 187-194.
| Crossref | Google Scholar |
McKnight DT, Georges A, Guarino F, Bower DS (2023) The ecology and morphology of Australia’s desert turtle (Emydura macquarii emmotti). Austral Ecology 48, 1657-1680.
| Crossref | Google Scholar |
Meathrel CE, Suter PJ, Radford NM (2002) Niche segregation between three species of freshwater turtle in a large billabong during flood. The Victorian Naturalist 119(4), 160-173.
| Google Scholar |
Milam JC, Melvin SM (2001) Density, habitat use, movements, and conservation of spotted turtles (Clemmys guttata) in Massachusetts. Journal of Herpetology 35(3), 418-427.
| Crossref | Google Scholar |
Pebesma E, Bivand R (2005) Classes and methods for spatial data in R. R News 5(2), 9-13.
| Google Scholar |
Purcell KL, McGregor EL, Calderala K (2017) Effects of drought on western pond turtle survival and movement patterns. Journal of Fish and Wildlife Management 8(1), 15-27.
| Crossref | Google Scholar |
Rees M, Roe JH, Georges A (2009) Life in the suburbs: behavior and survival of a freshwater turtle in response to drought and urbanization. Biological Conservation 142(12), 3172-3181.
| Crossref | Google Scholar |
Roe JH, Georges A (2008) Maintenance of variable responses for coping with wetland drying in freshwater turtles. Ecology 89(2), 485-494.
| Crossref | Google Scholar | PubMed |
Roe JH, Georges A, Green B (2008) Energy and water flux during terrestrial estivation and overland movement in a freshwater turtle. Physiological and Biochemical Zoology 81(5), 570-583.
| Crossref | Google Scholar | PubMed |
Semlitsch RD, Bodie JR (2003) Biological criteria for buffer zones around wetlands and riparian habitats for amphibians and reptiles. Conservation Biology 17(5), 1219-1228.
| Crossref | Google Scholar |
Spencer R-J (2018) How much long-term data are required to effectively manage a wide-spread freshwater turtle? Australian Zoologist 39, 568-575.
| Crossref | Google Scholar |
Spencer R-J, Thompson MB (2005) Experimental analysis of the impact of foxes on freshwater turtle populations. Conservation Biology 19(3), 845-854.
| Crossref | Google Scholar |
Stanford CB, Iverson JB, Rhodin AGJ, Paul van Dijk P, Mittermeier RA, Kuchling G, Berry KH, Bertolero A, Bjorndal KA, Blanck TEG, Buhlmann KA, Burke RL, Congdon JD, Diagne T, Edwards T, Eisemberg CC, Ennen JR, Forero-Medina G, Frankel M, Fritz U, Gallego-García N, Georges A, Gibbons JW, Gong S, Goode EV, Shi HT, Hoang H, Hofmeyr MD, Horne BD, Hudson R, Juvik JO, Kiester RA, Koval P, Le M, Lindeman PV, Lovich JE, Luiselli L, McCormack TEM, Meyer GA, Páez VP, Platt K, Platt SG, Pritchard PCH, Quinn HR, Roosenburg WM, Seminoff JA, Shaffer HB, Spencer R, Van Dyke JU, Vogt RC, Walde AD (2020) Turtles and tortoises are in trouble. Current Biology 30(12), R721-R735.
| Crossref | Google Scholar | PubMed |
Stott P (1987) Terrestrial movements of the fresh-water tortoise Chelodina-longicollis Shaw as monitored with a spool tracking device. Australian Wildlife Research 14, 559-567.
| Crossref | Google Scholar |
Van Dyke JU, Spencer R-J, Thompson MB, Chessman B, Howard K, Georges A (2019) Conservation implications of turtle declines in Australia’s Murray River system. Scientific Reports 9(1), 1998.
| Crossref | Google Scholar |
Wassens S, Walcott A, Wilson A, Freire R (2013) Frog breeding in rain-fed wetlands after a period of severe drought: implications for predicting the impacts of climate change. Hydrobiologia 708(1), 69-80.
| Crossref | Google Scholar |