Pump my wetland: potential benefits of using water pumps fitted with large-mesh screens to conserve anurans in regulated floodplain environments
Damian R. Michael
A
B
C
D
Abstract
Water pumps fitted with filtering screens are effective for delivering water to floodplain environments and excluding large-bodied exotic fish; yet, the benefits to wetland fish and anurans are unknown.
To quantify fish and tadpole responses to refilling wetlands with water pumps fitted with large-mesh screens following drawdown v. overland reconnections in the mid-Murrumbidgee region of New South Wales.
Frog and fish communities were compared between wetlands under managed inundation and overland reconnections by using PERMANOVA, and non-metric multidimensional scaling was used to evaluate community divergence between watering strategies. Classification and regression trees were used to identify thresholds in explanatory variables and predicted threshold responses in fish and tadpole abundance.
Fish and tadpole communities differed in relation to watering strategies. Managed inundation resulted in a higher abundance of tadpole species and one native fish, whereas overland reconnections resulted in a high abundance of exotic fish species and fewer tadpoles. Water depth was a driver of tadpole abundance in two species, including the threatened southern bell frog (Litoria raniformis).
Using filtered pumps with large-mesh screens to deliver water to wetlands of high conservation value may be an effective strategy for reducing large-bodied exotic fish and enhancing frog and fish populations in regulated floodplain systems.
Keywords: alien species, environmental water, fish screens, frog conservation, invasive fish management, larval anurans, management intervention, pumping, wetland restoration.
Introduction
River regulation is a significant threat to freshwater ecosystems worldwide (Nilsson and Dynesius 1994; Hawke et al. 2021). Changes to the frequency and magnitude of flow pulses have contributed to decreased frequency of natural connections between rivers and floodplains (Frazier et al. 2005; Page et al. 2005), permanent drying or inundation of ephemeral wetlands (Walker and Thoms 1993; Kingsford 2000; Rayner et al. 2015) and significant changes to the composition of wetland biodiversity (Kingsford 2000; Beesley et al. 2014; Littlefair et al. 2021). Small-bodied fish and frog communities can be particularly affected by long-term changes in wetland hydrology (Gilligan 2005; Wassens and Maher 2011), whereas the creation of permanent weir pools may favour exotic fish populations (Jellyman and Harding 2012). In Australia, fish biomass is often dominated by invasive common carp (Cyprinus carpio) and gambusia (Gambusia holbrooki), two species responsible for causing broad-scale wetland degradation and influencing the structure of aquatic communities (Koehn 2004; Gilligan 2005; Kloskowski 2011). Both species pose a significant threat to amphibians and their larvae (Morgan and Buttemer 1996; Webb and Joss 1997; Gillespie and Hero 1999; Pyke and White 2000; Hunter et al. 2011; Kloskowski 2011; Atobe et al. 2014) and have been implicated in the decline of endangered frog and fish species (Hunter et al. 2011; Mac Nally et al. 2014; Marshall et al. 2019).
Rivers that have a high percentage of their annual flows extracted from the system often need water to be reinstated to maintain ecological processes (Linke and Deretic 2020). This can be achieved through environmental water allocations to restore overbank flows or re-water disconnected wetlands (Arthington et al. 2006). In the Murray–Darling Basin, environmental water allocations (often termed water for the environment) are used by State and Commonwealth organisations to restore natural hydrological regimes in rivers and floodplain wetlands (Arthington et al. 2006; Gawne et al. 2020). Although highly desirable in replicating natural connectivity between the river and floodplain wetlands, provision of larger flow pulses poses a significant challenge in multi-use river systems (Banks and Docker 2014) and may inadvertently benefit invasive fish species by delivering them to suitable spawning sites (Conallin et al. 2012), impeding the recovery of native wetland biodiversity (Rayner et al. 2015).
In an era of water scarcity (Arthington et al. 2018; Berbel and Esteban 2019), managed inundation of freshwater habitats using pumps may be an important strategy to improve native species diversity (Vilizzi et al. 2013; Hoffmann 2018). Large irrigation pumps can transfer significant volumes of water directly from the main river channel or through irrigation distributary networks to floodplain wetlands (King and O’Connor 2007; Boys et al. 2021a), and, when fitted with filtering screens, can reduce entrainment of native fish (Baumgartner and Boys 2012; Boys 2021; Boys et al. 2021a, 2021b). Pumping water from river channels can maintain water levels in refuge wetlands during non-flood years, and thus sustain diversity of wetland fauna such as small-bodied native fish (Vilizzi et al. 2013), freshwater turtles (Ocock et al. 2018), amphibians (Hoffmann 2018; Littlefair et al. 2021) and floodplain snakes (Michael et al. 2023). Delivering water by pumping infrastructure to wetlands can be further enhanced by using large-mesh filtering screens to exclude large-bodied exotic fish (Hillyard et al. 2010; Vilizzi et al. 2013), while delivering small-bodied native species to targeted wetlands of high conservation value. Significant efforts are underway to implement modern fish-protection screens on water infrastructure (Baumgartner and Boys 2012; Boys et al. 2021a, 2021b). Prioritisation of sites for modernisation requires an assessment of the ecological goals for restoration. Central to this assessment are the desired outcomes of waterways receiving allocations. Modern fish-protection screens feature a fine mesh (2–3-mm mesh size), with the goal of protecting native biota by avoiding entrainment into water delivered through water pumps. This technology is appropriate for sites where water is extracted for irrigated agriculture. However, at sites where water is delivered to habitats with ecological significance (such as floodplain wetlands), managers may seek to exclude native fish and amphibians from pumped water. At these sites, use of large-mesh screens (>30-mm mesh size) may be appropriate. However, the benefits of using large-mesh screens in floodplain anuran conservation is largely unknown. To address this knowledge gap, we compared responses of fish and larval frog (tadpole) over a 10-year period with two water-delivery methods, managed inundation using large-mesh screens v. overland reconnections, in four ecologically significant wetlands in south-eastern Australia. We hypothesised that managed inundation using large-mesh screens would reduce adult carp abundance (Vilizzi et al. 2013), thereby increasing tadpole numbers owing to predator release (Hunter et al. 2011; Atobe et al. 2014). We also postulated that small-bodied native fish abundance would be similar between methods as large-mesh screens do not protect native fish from entrainment (Boys et al. 2021a, 2021b). Threshold responses to water level and temperature were also examined because changes in these metrics are important for structuring fish and frog communities (Bino et al. 2018).
Methods
Study sites
This study was conducted in the mid-Murrumbidgee region of southern New South Wales (NSW) (Fig. 1). Wetlands in this region are part of a semi-arid floodplain ecosystem and support large stands of river red gum (Eucalyptus camaldulensis) forest as well as significant populations of native fish and the threatened southern bell frog (Litoria raniformis) (Wassens et al. 2021), listed as Endangered in NSW (Biodiversity Conservation Act 2016), nationally Vulnerable (Environment Protection and Biodiversity Conservation Act 1999) and globally Endangered (IUCN Red List). Four wetlands, classified as ox-bow lagoons (Yarradda, Sunshower, Gooragool and McKennas), form part of a broader long-term ecological monitoring program in the Murrumbidgee catchment (Wassens et al. 2021) and were chosen because they have experienced significant shifts in hydrology following river regulation and recent changes in water management. Yarradda and Gooragool lagoons are in the Murrumbidgee Valley National Park. Grazing is absent from Gooragool and ceased at Yarradda in 2018, whereas McKennas and Sunshower lagoons are located on private property and are intermittently grazed by cattle. Prior to the Millennium Drought (2001–2009), this wetland cluster experienced regular floods and was inundated, on average, in 9 or 10 years by natural river to wetland overland reconnection events (Hall et al. 2019). Between 2000 and 2009, all four wetlands remained continuously dry and filled in 2010–11 during a natural flood (Hall et al. 2019).
Water delivery and timeline
In response to changes in hydrology and declining wetland condition, the NSW Department of Planning, Industry and Environment (DPIE) and Commonwealth Environmental Water Office (CEWO) returned water to Yarradda and Sunshower lagoons by delivering environmental water from the Murrumbidgee River by pumping infrastructure (hereafter termed managed inundation) (Fig. 2). In the austral winter of 2014, Yarradda Lagoon dried up, after which, CEWO and DPIE refilled the wetland in summer 2015 by using filtering screens (~80-mm mesh) fitted to the inlet of an impeller-type pump to control excessive carp numbers. The irrigation pump delivered 40 mL day−1 and water level was maintained to at least 50% capacity by additional pumping actions between January 2014 and mid-2016 (Fig. 2). A large and natural overland reconnection (flood event) occurred between August and November 2016, complemented with a managed environmental water allocation used to facilitate a river to wetland reconnection in August 2017. The wetland was allowed to briefly dry (less than 5 days) in spring 2018, to remove carp that had entered the wetland and was subsequently refilled through a filtering pump in November 2018. At Sunshower Lagoon, three small pumps delivered a combined total of ~20 mL day−1 in 2020 after a 2-year dry phase. Each pump inlet was fitted with ~30-mm holes and, post pump, a 400-m poly pipeline was installed to deliver the environmental water to Sunshower Lagoon via a small tributary. Gooragool and McKennas lagoons connected to the main channel during unregulated flood events (hereafter termed overland reconnections) during 2011–12 and 2015–18 (Fig. 2). Natural flood events and managed reconnections using environmental water allocations were both classified as overland reconnections in this study.
Sampling protocol
At each of the four wetlands, annual fish and tadpole surveys were conducted between 2011 and 2020 water years (July–June) by using a combination of two large (5-m double wing and 12-mm mesh) and two small (3-m double wing and 4-mm mesh) fyke nets set overnight for an average of 14 h. When water levels were low, two D-fyke nets (3-m double wing and 12-mm mesh) were deployed. Surveys were conducted during September, November, January and March each year. Fish and tadpole catch-per-unit-effort (CPUE, tadpole fish−1 h−1) was calculated for each wetland by dividing net soak time by the number of individuals collected. Small-bodied fish were defined as being <100 mm and adult carp were defined as being sexually mature at >150 mm. Water depth was measured using a water-level logger (Hobo U20L) at each wetland. Developed models of water depths as a function of days since last commence-to-fill were used to predict water depth between 2010 and 2012 and in Sunshower Lagoon following logger failure during the 2019–2020 surveys (Fig. S1 of the Supplementary material). Water temperature was measured using a hand-held water-quality meter (Horiba U50).
Data analysis
Survey-level data were pooled for each water year (June–July). Fish and tadpole CPUE was averaged for each water year and underwent fourth-root transformation. Comparisons of frog and fish communities between wetlands under managed inundation v. overland reconnections were undertaken using PERMANOVA on the basis of a Euclidian distance resemblance matrix, sums of squares Type III (partial) and unrestricted permutation of the transformed data, with site being included as a random factor. Non-metric multidimensional scaling (nMDS) was used to examine community divergence between watering strategies. Key species contribution to differences between managed inundation and overland reconnections were assessed using SIMPER (Primer, ver. 7, Primer-E Ltd, Lutton, UK, see https://www.primer-e.com/our-software/). A Wilcoxon test was used to compare mean CPUE between reconnection events and managed inundation actions, and a one-way Student’s t-test was used for species with low numbers under managed inundation events by using R (ver. 4.3.2, R Foundation for Statistical Computing, Vienna, Austria, see https://www.R-project.org/).
To identify thresholds in explanatory variables that best predicted threshold in response variables, a regression tree approach (Classification and Regression Trees, CART) was used to explore data for Yarradda Lagoon because this wetland experienced repeated managed inundation and overland reconnection events (Fig. 2). Responses in the CPUE of each of the five detected tadpole species, the total combined tadpole CPUE, common carp CPUE and gambusia CPUE were examined. Tadpoles of Limnodynastes tasmaniensis and Limnodynastes fletcheri are indistinguishable in the field and were combined for analysis. Ecological response thresholds against water depth, water temperature and water delivery method (managed inundation v. overland reconnections) were then evaluated. The Gini index in the ‘rpart’ package (ver. 4.1.21, T Therneau, B Atkinson and B Ripley, see https://cran.r-project.org/package=rpart) was used to progressively identify thresholds in explanatory variables. No assumptions were made regarding the distribution of response variables (Breiman et al. 1984). Analysis was implemented using the ‘rpart’ function in the ‘rpart’ package in R.
Results
Summary statistics
Between 2011 and 2020, we recorded 109 603 individuals from 14 fish species (five introduced, n = 54 747; and nine native species, n = 54 856) (Table 1). Carp gudgeon (Hypseleotris sp.) was the most abundant species (n = 49 063) and contributed 89% of the total native fish catch. Carp were the most abundant exotic species (n = 37 348, 62% of total exotic fish catch), followed by gambusia (n = 18 360, 31% of total exotic fish catch). During the same period, 1950 tadpoles of five frog species were recorded (Crinia parinsignifera, n = 4; Limnodynastes sp., n = 367; Litoria peronii, n = 1173; L. raniformis, n = 42; and L. interioris, n = 364). Limnodynastes sp. (L. tasmaniensis and L. fletcheri), Litoria interioris and L. peronii tadpoles were recorded across all wetlands. C. parinsignifera and Litoria raniformis tadpoles were recorded only at Yarradda Lagoon.
Site | Metric | 2011 | 2012 | 2013 | 2015 | 2016 | 2017 | 2018 | 2019 | 2020 | |
---|---|---|---|---|---|---|---|---|---|---|---|
Sunshower | Tadpole (n) | 0 ± 0 | 0.33 ± 0.47 | 0.12 ± 0.13 | 0 ± 0 | 0 ± 0 | 1.56 ± 2.71 | 4.72 ± 6.67 | 0 ± 0 | 2.94 ± 0.89 | |
Carp (n) | 0 ± 0 | 58.84 ± 114.19 | 0.92 ± 1.09 | 0 ± 0 | 0 ± 0 | 62.36 ± 92.71 | 22.69 ± 32.08 | 0 ± 0 | 0 ± 0 | ||
Water depth (m) | 0.86 ± 0.21 | 0.53 ± 0.32 | 0.35 ± 0.31 | 0 ± 0 | 0.14 ± 0.16 | 1.25 ± 1.1 | 0.59 ± 0.54 | 0 ± 0 | 0.34 ± 0.44 | ||
Water temperature (°C) | 27.34 ± 1.65 | 25.37 ± 4.98 | 28.35 ± 7.05 | 0 ± 0 | 0 ± 0 | 25.2 ± 10.08 | 21.16 ± 4.73 | 0 ± 0 | 22.52 ± 1.66 | ||
Gooragool | Tadpole (n) | 0 ± 0 | 0.41 ± 0.81 | 0 ± 0 | 0 ± 0 | 0.29 ± 0.25 | 0.08 ± 0.17 | 0.05 ± 0.04 | 0 ± 0 | 0.5 ± 0.79 | |
Carp (n) | 0 ± 0 | 11.19 ± 7.45 | 0 ± 0 | 0 ± 0 | 17.42 ± 25.05 | 4.83 ± 5.3 | 2.37 ± 2.37 | 0 ± 0 | 123.78 ± 146.37 | ||
Water depth (m) | 0.83 ± 0.26 | 0.76 ± 0.38 | 0.15 ± 0.09 | 0.08 ± 0.1 | 0.68 ± 0.36 | 1.1 ± 0.75 | 0.49 ± 0.41 | 0 ± 0 | 0.8 ± 0.57 | ||
Water temperature (°C) | 30.2 ± 1.7 | 27.13 ± 6.25 | 28.1 ± 4.05 | 27.2 ± 1.94 | 23.94 ± 6.52 | 23.99 ± 7.85 | 29.02 ± 8 | 0 ± 0 | 27.14 ± 2.77 | ||
McKennas | Tadpole (n) | 0 ± 0 | 0.79 ± 1.05 | 0.1 ± 0.11 | 0 ± 0 | 0 ± 0 | 2.95 ± 5.91 | 6.47 ± 9.15 | 0 ± 0 | 0 ± 0 | |
Carp (n) | 0 ± 0 | 197.83 ± 340.01 | 8.25 ± 8.05 | 0 ± 0 | 0 ± 0 | 62.73 ± 119.06 | 0.53 ± 0.66 | 0 ± 0 | 0 ± 0 | ||
Water depth (m) | 1.7 ± 0.27 | 1.17 ± 0.7 | 0.82 ± 0.72 | 0 ± 0 | 0 ± 0 | 1.71 ± 0.85 | 0.59 ± 0.46 | 0 ± 0 | 0 ± 0 | ||
Water temperature (°C) | 28.71 ± 3.11 | 26.74 ± 7.61 | 27.68 ± 5.77 | 0 ± 0 | 0 ± 0 | 26.66 ± 8.06 | 27.79 ± 12.02 | 0 ± 0 | 0 ± 0 | ||
Yarradda | Tadpole (n) | 0 ± 0 | 0.08 ± 0.12 | 0.07 ± 0.13 | 3.53 ± 3.58 | 4.45 ± 5.16 | 0.05 ± 0.1 | 0.28 ± 0.57 | 15.23 ± 26.21 | 1.71 ± 1.94 | |
Carp (n) | 0 ± 0 | 7.96 ± 13.49 | 0.46 ± 0.51 | 0 ± 0 | 7.58 ± 9.52 | 52.47 ± 48.56 | 5.77 ± 8.93 | 26.83 ± 31.72 | 2.97 ± 3.77 | ||
Water depth (m) | 2.57 ± 0.18 | 2.14 ± 0.4 | 1.86 ± 0.47 | 0.96 ± 1.11 | 2.07 ± 0.29 | 2.78 ± 0.92 | 2.14 ± 0.4 | 2.02 ± 0.69 | 2.03 ± 0.44 | ||
Water temperature (°C) | 26.76 ± 5.52 | 25.13 ± 5.67 | 25.68 ± 2.72 | 27.61 ± 5.06 | 22.51 ± 5.77 | 25.21 ± 7.17 | 26.07 ± 4.21 | 24.84 ± 5.2 | 24 ± 4.46 |
SUN, Sunshower lagoon; GOO, Gooragool lagoon; MCK, McKennas lagoon; YAR, Yarradda lagoon.
Managed inundation versus overland reconnections
Overall, fish and tadpole community composition differed significantly between managed inundation and overland reconnections (PERMANOVA pseudo-F 2.088, P = 0.009) (Fig. 3). Differences were driven by higher average abundance of C. parinsignifera (P < 0.001), L. peronii (P < 0.01), L. interioris (P < 0.01), L. raniformis (P < 0.01) tadpoles and bony herring (Nematalosa erebi) (P < 0.05) following pumping (Fig. 4). Fish communities associated with overland reconnections had higher abundance of carp gudgeon, Australian smelt (Retropinna semoni), carp, gambusia, unspecked hardyhead (Craterocephalus fulvus) and goldfish (Carassius auratus) (Fig. 4), although differences were not statistically significant. We found no statistical differences in average CPUE between managed inundation and overland reconnections for small-bodied native fish such as Murray–Darling rainbowfish (Melanotaenia fluviatilis), flat-headed gudgeon (Philypnodon grandiceps) or oriental weatherloach (Misgurnus anguillicaudatus). Large-bodied native fish such as golden perch (Macquaria ambigua) were absent following managed inundation events (Fig. 4).
Tadpole and fish threshold responses in Yarradda Lagoon
Regression tree analysis identified managed inundation as a key driver of total tadpole CPUE, as well as for CPUE of C. parinsignifera, Limnodynastes sp. and L. peronii (Fig. 5a, b, d), with a higher CPUE being associated with managed inundation compared with reconnections. L. raniformis tadpoles were associated with managed inundation, although water depth was also an important predictor. L. raniformis tadpoles were not detected when water depth was below 2.3 m, but CPUE increased when water levels exceeded 2.3 m (Fig. 5c). Limnodynastes interioris CPUE was driven by a combination of water depth and managed inundation, having high CPUE when water levels exceeded 2.6 m. When water levels dropped below 2.6 m then managed inundation was associated with high L. interioris CPUE (Fig. 5f). Water delivery method was not a significant predictor of carp CPUE, instead, a water depth threshold was identified as a key predictor, with a higher carp CPUE when depths exceeded 2.2 m (Fig. 5g). Water temperature was the key predictor of gambusia CPUE, with an initial threshold when temperatures were greater than 27°C and a second threshold reached when temperatures exceeded 29°C (Fig. 5h).
Regression tree thresholds for (a) total tadpole; (b) Crinia parinsignifera, (c) Litoria raniformis, (d) Limnodynates sp., (e) Litoria peronii, (f) Limnodynates interios, (g) common carp and (h) eastern gambusia catch-per-unit-effort (CPUE) in Yarradda Lagoon. DM, delivery mode; rec, river to wetland reconnection event; pum, pumping with environmental water allocations, dark shading represents higher values.
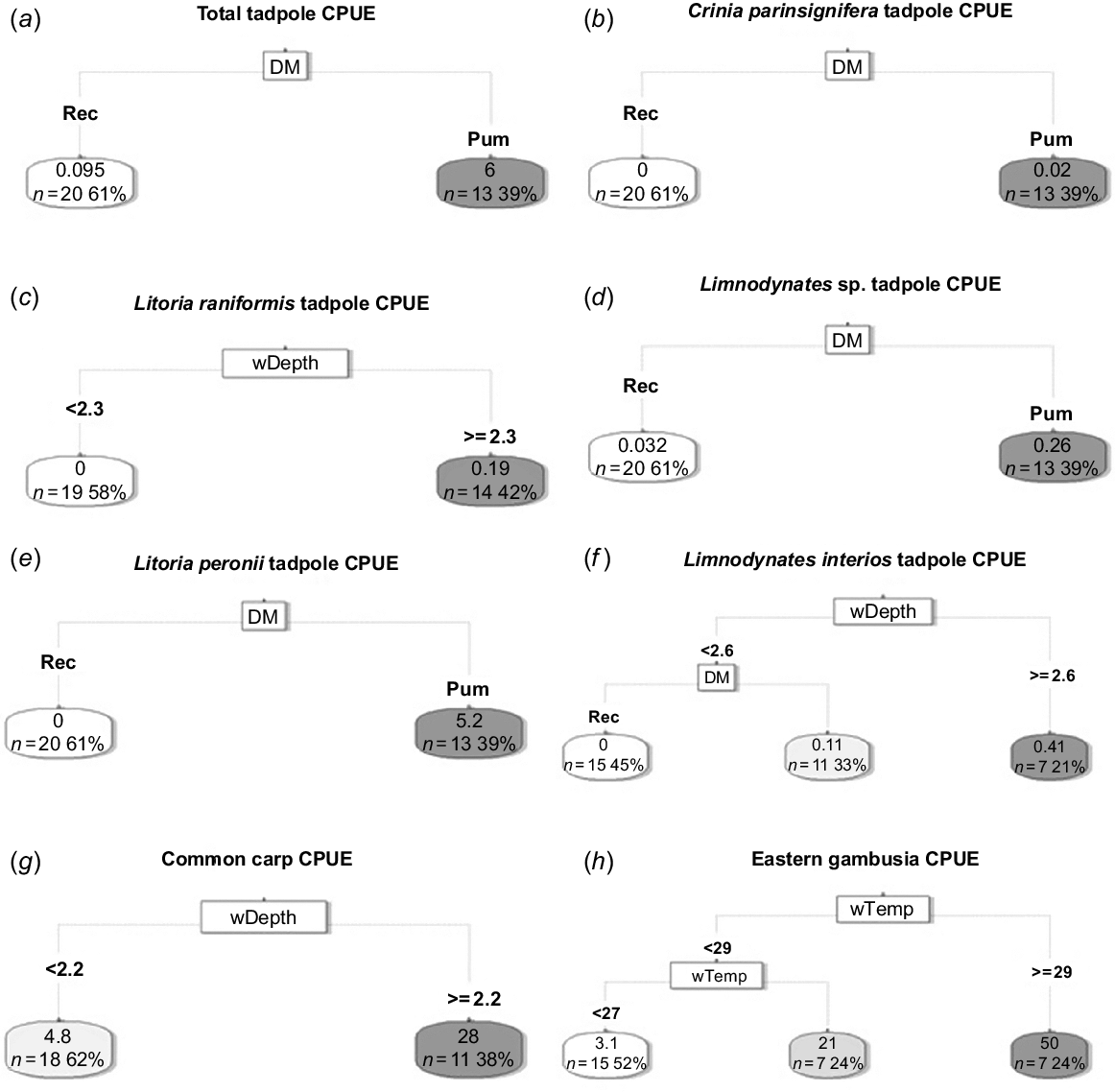
Discussion
In managed wetland ecosystems, the timing, frequency and volume of water delivered can have a profound effect on the structure of aquatic communities (Wassens and Maher 2011; Vilizzi et al. 2013; Beesley et al. 2014; Wedderburn et al. 2014). This study took advantage of a shift in wetland management (water delivery) and builds on the mesh screening literature by demonstrating the relationship between water delivery method (managed inundation through pumping v. overland reconnections), water depth, and water temperature and the structure of frog and fish communities in a semi-arid floodplain ecosystem. Managed inundation following dry phases was found to be associated with significant increases in tadpole abundance, especially of common and widespread species such as C. parinsignifera, L. interioris and L. peronii, but also of the threatened L. raniformis. Managed inundations were also found to reduce large-bodied exotic fish species from entering the system, a finding that is consistent with that of a similar study which investigated fish communities following managed inundation events (Vilizzi et al. 2013). Our findings are also congruent with studies that have demonstrated amphibian population recovery following eradication of non-native fish (Knapp et al. 2007; Pope 2008; Tiberti et al. 2019; Miró et al. 2020). Loss of common species can have profound ecological and economic consequences (Winfree et al. 2015; Gaston et al. 2018). Therefore, interventions that maintain or increase the abundance of widespread species are important for maintaining ecosystem stability. The use of pumps fitted with large-mesh screens, therefore, has broad implications for recovering wetland frog populations in regulated floodplain environments affected by reduced flows and water availability. However, we acknowledge that due to our small sample size, our capacity to draw general conclusions may be limited.
Environmental watering of wetlands by pumping may provide critical breeding opportunities and refugia for maintaining threatened floodplain frog species. The results of this study indicated that tadpoles of L. raniformis were higher in abundance following managed inundation than after overland reconnections, although regression tree analyses identified water depth as a strong variable explaining CPUE, highlighting the importance of restoring natural flood conditions to create suitable breeding conditions for threatened floodplain frog species. Ensuring that wetlands are inundated to capacity and for extended durations is likely to benefit L. raniformis because the species requires rising water levels to trigger breeding (Hamer et al. 2016) and sustained water levels to reach maturity. L. raniformis successfully bred in Yarradda Lagoon following managed inundation in 2014–15 when high tadpole counts where recorded. However, no L. raniformis tadpoles were recorded in the following years when water levels dropped to 50% capacity and exotic fish numbers increased. A second breeding event occurred following a natural reconnection event in 2017–2018, which raised water levels to 80% capacity. By contrast, we found no evidence that L. raniformis successfully bred in 2018–19 or 2019–20 during managed inundation, likely owing to sustained predation by exotic fish or our limited sample size or survey method. Larvae of this species are difficult to detect because of their cryptic foraging behaviour in shallow waters as well as their susceptibility to predation by gambusia (Pyke and White 2000), a species capable of passing through fish screens used in this study, possibly preventing L. raniformis from undergoing larval development and recruitment. Ongoing monitoring is required to determine whether managed inundation will benefit this species as well as other threatened floodplain frog species in the medium to long term.
Managed inundation using filtering screens fitted to pumping infrastructure is an effective tool for excluding large-bodied fish such as carp (Vilizzi et al. 2013). However, larval and juvenile carp can still enter the system and will require ongoing control; so, any potential benefits will be short-lived. In temperate environments, carp grow rapidly, reaching sexual maturity within 2 years and are highly fertile (Vilizzi and Walker 1999; Tessema et al. 2020). Thus, the amount of time that elapses following managed inundation after a dry phase before carp impose significant predation pressure on resident frog species is likely to be 2–3 years (Kloskowski 2011). River to wetland reconnections can also rapidly introduce high densities of carp to the floodplain (Conallin et al. 2012), undoing past management actions. The findings from Yarradda Lagoon highlighted this pattern, as over 200 adult carp (CPUE) and 300 gambusia (CPUE) were recorded in 2016–2017 following an overland reconnection. Allowing ephemeral wetlands to enter a dry phase is crucial for maintaining wetland health and will be important for reducing carp numbers. One promising pattern that emerged from this study was higher abundances of native bony herring following managed inundation, indicating that smaller individuals were able to safely pass through the large-mesh screens. Managed inundation also did not appear to affect small-bodied native fish with similar numbers of Murray–Darling rainbowfish and flat-headed gudgeon being recorded between water delivery methods, suggesting that timing managed inundation with native fish supply from the main channel is important. Therefore, using managed inundation through pumping during native fish breeding periods, coupled with strategically allowing wetlands to draw down annually or biannually during winter months, may be an effective management tool for restocking small-bodied native fish communities and reducing the incursion of large exotic carp between unregulated overland reconnections.
Associative relationships among watering methods, fish communities and tadpole numbers were identified in this study. However, the mechanism behind the patterns remains unclear because other factors influencing frog breeding success may be at play. Two potential mechanisms include released predation pressure on adult frogs and their eggs (Gillespie and Hero 1999; Komak and Crossland 2000; Hunter et al. 2011) and improved condition of aquatic vegetation or water quality, leading to greater tadpole survival (Bajer and Sorensen 2015). Thus, the interactive effects of predator release on other stages of amphibian life cycle and concurrent improvements in water quality or wetland vegetation could not be disentangled in this study. Other factors not measured in this study may have contributed to frog breeding success, including annual rainfall, inundation duration, temperature (Mac Nally et al. 2014) and grazing intensity (Jansen and Healey 2003). Future studies should include a broader set of wetlands stratified by different management interventions to decouple the interactive effects of different recovery pathways.
Conclusions and management implication
Our findings confirmed that the structure of fish and tadpole communities in floodplain wetlands differ depending on the method of water delivery. Following a dry phase, managed inundation using pumps fitted with large-mesh filtering screens can reduce incursions of large-bodied fish (including carp) and promote breeding in floodplain frog species, and some small-bodied native fish. By contrast, river to wetland reconnections contribute to a higher abundance of exotic fish species and reduced tadpole numbers, which must be considered in the long-term management of wetlands that support threatened frogs. Attempts to improve frog populations worldwide are often hampered by non-native fish. Thus, improved water delivery methods using screening technology provide novel opportunities for manipulating fish communities and improving breeding outcomes for anurans in regulated floodplain environments.
Data availability
The data that support the findings of this study are available from the corresponding author upon reasonable request.
Declaration of funding
This research is funded by the Commonwealth Environmental Water Office, approved by Charles Sturt Animal Care and Ethics committee (Protocol Numbers: A16054, A19028), under the scientific licences (OEH: SL100441) and DPI (P11/0043-3.0).
Author contributions
Damian Michael led the conceptualisation and writing of the original draft. Gilad Bino supported the conceptualisation and led the formal analysis and visualisation. James Maguire, Anthony Conallin and Skye Wassens provided equal contribution to the review and editing of the written material. Skye Wassens also the conceptualisation and funding acquisition.
Acknowledgements
The authors respectfully acknowledge the traditional owners, their Elders past, present and future, and their Nations on which this publication is based. We thank New South Wales National Parks and Wildlife Service, Vince Bucello and Robert Colbert for permitting site access. Gaye Bourke assisted with data collection.
References
Arthington AH, Bunn SE, Poff NL, Naiman RJ (2006) The challenge of providing environmental flow rules to sustain river ecosystems. Ecological Applications 16, 1311-1318.
| Crossref | Google Scholar | PubMed |
Arthington AH, Kennen JG, Stein ED, Webb JA (2018) Recent advances in environmental flows science and water management – innovation in the Anthropocene. Freshwater Biology 63, 1022-1034.
| Crossref | Google Scholar |
Atobe T, Osada Y, Takeda H, Kuroe M, Miyashita T (2014) Habitat connectivity and resident shared predators determine the impact of invasive bullfrogs on native frogs in farm ponds. Proceedings of the Royal Society of London – B. Biological Sciences 281, 20132621.
| Crossref | Google Scholar |
Bajer PG, Sorensen PW (2015) Effects of common carp on phosphorus concentrations, water clarity, and vegetation density: a whole system experiment in a thermally stratified lake. Hydrobiologia 746, 303-311.
| Crossref | Google Scholar |
Banks SA, Docker BB (2014) Delivering environmental flows in the Murray–Darling Basin (Australia) – legal and governance aspects. Hydrological Sciences Journal 59, 688-699.
| Crossref | Google Scholar |
Baumgartner LJ, Boys C (2012) Reducing the perversion of diversion: applying world-standard fish screening practices to the Murray–Darling Basin. Ecological Management & Restoration 13, 135-143.
| Crossref | Google Scholar |
Beesley L, King AJ, Gawne B, Koehn JD, Price A, Nielsen D, et al. (2014) Optimising environmental watering of floodplain wetlands for fish. Freshwater Biology 59, 2024-2037.
| Crossref | Google Scholar |
Berbel J, Esteban E (2019) Droughts as a catalyst for water policy change. Analysis of Spain, Australia (MDB), and California. Global Environmental Change 58, 101969.
| Crossref | Google Scholar |
Bino G, Wassens S, Kingsford RT, Thomas RF, Spencer J (2018) Floodplain ecosystem dynamics under extreme dry and wet phases in semi-arid Australia. Freshwater Biology 63, 224-241.
| Crossref | Google Scholar |
Boys CA, Rayner TS, Baumgartner LJ, Doyle K E (2021a) Native fish losses due to water extraction in Australian rivers: evidence, impacts and a solution in modern fish- and farm-friendly screens. Ecological Management & Restoration 22, 134-144.
| Crossref | Google Scholar |
Conallin AJ, Smith BB, Thwaites LA, Walker KF, Gillanders BM (2012) Environmental water allocations in regulated lowland rivers may encourage offstream movements and spawning by common carp, Cyprinus carpio: implications for wetland rehabilitation. Marine and Freshwater Research 63, 865-877.
| Crossref | Google Scholar |
Frazier P, Page K, Read A (2005) Effects of flow regulation in flow regime on the Murrumbidgee River, south eastern Australia: an assessment using a daily estimation hydrological model. Australian Geographer 36, 301-314.
| Crossref | Google Scholar |
Gaston KJ, Cox DTC, Canavelli SB, García D, Hughes B, Maas B, Martinez D, Ogada D, Inger R (2018) Population abundance and ecosystem service provision: the case of birds. BioScience 68, 264-272.
| Crossref | Google Scholar | PubMed |
Gawne B, Hale J, Stewardson MJ, Webb JA, Ryder DS, Brooks SS, Campbell CJ, Capon SJ, Everingham P, Grace MR, Guarino F, Stoffels RJ (2020) Monitoring of environmental flow outcomes in a large river basin: the commonwealth environmental water holder’s long-term intervention in the Murray–Darling Basin, Australia. River Research and Applications 36, 630-644.
| Crossref | Google Scholar |
Hall A, Thomas RF, Wassens S (2019) Mapping the maximum inundation extent of lowland intermittent riverine wetland depressions using LiDAR. Remote Sensing of Environment 233, 111376.
| Crossref | Google Scholar |
Hamer AJ, Heard GW, Urlus J, Ricciardello J, Schmidt B, Quin D, Steele WK (2016) Manipulating wetland hydroperiod to improve occupancy rates by an endangered amphibian: modelling management scenarios. Journal of Applied Ecology 53, 1842-1851.
| Crossref | Google Scholar |
Hawke T, Bino G, Kingsford RT (2021) Damming insights: variable impacts and implications of river regulation on platypus populations. Aquatic Conservation: Marine and Freshwater Ecosystems 31, 504-519.
| Crossref | Google Scholar |
Hillyard KA, Smith BB, Conallin AJ, Gillanders BM (2010) Optimising exclusion screens to control exotic carp in an Australian lowland river. Marine and Freshwater Research 61, 418-429.
| Crossref | Google Scholar |
Hoffmann EP (2018) Environmental watering triggers rapid frog breeding in temporary wetlands within a regulated river system. Wetlands Ecology and Management 26, 1073-1087.
| Crossref | Google Scholar |
Hunter DA, Smith MJ, Scroggie MP, Gilligan D (2011) Experimental examination of the potential for three introduced fish species to prey on tadpoles of the endangered Booroolong frog, Litoria booroolongensis. Journal of Herpetology 45, 181-185.
| Crossref | Google Scholar |
Jansen A, Healey M (2003) Frog communities and wetland condition: relationships with grazing by domestic livestock along an Australian floodplain river. Biological Conservation 109, 207-219.
| Crossref | Google Scholar |
Jellyman PG, Harding JS (2012) The role of dams in altering freshwater fish communities in New Zealand. New Zealand Journal of Marine and Freshwater Research 46, 475-489.
| Crossref | Google Scholar |
King AJ, O’Connor JP (2007) Native fish entrapment in irrigation systems: a step towards understanding the significance of the problem. Ecological Management & Restoration 8, 32-37.
| Crossref | Google Scholar |
Kingsford RT (2000) Ecological impacts of dams, water diversions and river management on floodplain wetlands in Australia. Austral Ecology 25, 109-127.
| Crossref | Google Scholar |
Kloskowski J (2011) Impact of common carp Cyprinus carpio on aquatic communities: direct trophic effects versus habitat deterioration. Fundamental and Applied Limnology 178, 245-255.
| Crossref | Google Scholar |
Knapp RA, Boiano DM, Vredenburg VT (2007) Removal of nonnative fish results in population expansion of a declining amphibian (mountain yellow-legged frog, Rana muscosa). Biological Conservation 135, 11-20.
| Crossref | Google Scholar | PubMed |
Koehn JD (2004) Carp (Cyprinus carpio) as a powerful invader in Australian waterways. Freshwater Biology 49, 882-894.
| Crossref | Google Scholar |
Komak S, Crossland MR (2000) An assessment of the introduced mosquitofish (Gambusia affinis holbrooki) as a predator of eggs, hatchlings and tadpoles of native and non-native anurans. Wildlife Research 27, 185-189.
| Crossref | Google Scholar |
Linke S, Deretic J-A (2020) Ecoacoustics can detect ecosystem responses to environmental water allocations. Freshwater Biology 65, 133-141.
| Crossref | Google Scholar |
Littlefair ME, Nimmo DG, Ocock JF, Michael DR, Wassens S (2021) Amphibian occurrence and abundance patterns across a modified floodplain ecosystem. Austral Ecology 46, 1343-1355.
| Crossref | Google Scholar |
Mac Nally R, Nerenberg S, Thomson JR, Lada H, Clarke RH (2014) Do frogs bounce, and if so, by how much? Responses to the ‘Big Wet’ following the ‘Big Dry’ in south-eastern Australia. Global Ecology and Biogeography 23, 223-234.
| Crossref | Google Scholar |
Marshall JC, Blessing JJ, Clifford SE, Hodges KM, Negus PM, Steward AL (2019) Ecological impacts of invasive carp in Australian dryland rivers. Aquatic Conservation: Marine and Freshwater Ecosystems 29, 1870-1889.
| Crossref | Google Scholar |
Michael DR, Nimmo DG, Stevens E, Schlen T, Wassens S (2023) Finding Ngabi (Hemiaspis damelii): factors affecting the use of modified floodplain wetlands by an endangered snake. Wildlife Research [Published online early 10 March 2023].
| Crossref | Google Scholar |
Miró A, O’Brien D, Tomàs J, Buchaca T, Sabás I, Osorio V, Lucati F, Pou-Rovira Q, Ventura M (2020) Rapid amphibian community recovery following removal of non-native fish from high mountain lakes. Biological Conservation 251, 108783.
| Crossref | Google Scholar |
Morgan LA, Buttemer WA (1996) Predation by the non-native fish Gambusia holbrooki on small Litoria aurea and L. dentata tadpoles. Australian Zoologist 30, 143-149.
| Crossref | Google Scholar |
Nilsson C, Dynesius M (1994) Ecological effects of river regulation on mammals and birds: a review. Regulated Rivers: Research & Management 9, 45-53.
| Crossref | Google Scholar |
Ocock JF, Bino G, Wassens S, Spencer J, Thomas RF, Kingsford RT (2018) Identifying critical habitat for Australian freshwater turtles in a large regulated floodplain: implications for environmental water management. Environmental Management 61, 375-389.
| Crossref | Google Scholar | PubMed |
Page K, Read A, Frazier P, Mount N (2005) The effect of altered flow regime on the frequency and duration of bankfull discharge: Murrumbidgee River, Australia. River Research and Applications 21, 567-578.
| Crossref | Google Scholar |
Pope KL (2008) Assessing changes in amphibian population dynamics following experimental manipulations of introduced fish. Conservation Biology 22, 1572-1581.
| Crossref | Google Scholar | PubMed |
Pyke G, White A (2000) Factors influencing predation on eggs and tadpoles of the endangered green and golden bell frog Litoria aurea by the introduced plague minnow Gambusia holbrooki. Australian Zoologist 31, 496-505.
| Crossref | Google Scholar |
Rayner TS, Kingsford RT, Suthers IM, Cruz DO (2015) Regulated recruitment: native and alien fish responses to widespread floodplain inundation in the Macquarie Marshes, arid Australia. Ecohydrology 8, 148-159.
| Crossref | Google Scholar |
Tessema A, Getahun A, Mengistou S, Fetahi T, Dejen E (2020) Reproductive biology of common carp (Cyprinus carpio Linnaeus, 1758) in Lake Hayq, Ethiopia. Fisheries and Aquatic Sciences 23, 16.
| Crossref | Google Scholar |
Tiberti R, Bogliani G, Brighenti S, Iacobuzio R, Liautaud K, Rolla M, von Hardenberg A, Bassano B (2019) Recovery of high mountain Alpine lakes after the eradication of introduced brook trout Salvelinus fontinalis using non-chemical methods. Biological Invasions 21, 875-894.
| Crossref | Google Scholar |
Vilizzi L, Walker KF (1999) Age and growth of the common carp, Cyprinus carpio, in the River Murray, Australia: validation, consistency of age interpretation, and growth models. Environmental Biology of Fishes 54, 77-106.
| Crossref | Google Scholar |
Vilizzi L, McCarthy BJ, Scholz O, Sharpe CP, Wood DB (2013) Managed and natural inundation: benefits for conservation of native fish in a semi-arid wetland system. Aquatic Conservation: Marine and Freshwater Ecosystems 23, 37-50.
| Crossref | Google Scholar |
Walker KF, Thoms MC (1993) Environmental effects of flow regulation on the lower River Murray, Australia. Regulated Rivers: Research & Management 8, 103-119.
| Crossref | Google Scholar |
Wassens S, Maher M (2011) River regulation influences the composition and distribution of inland frog communities. River Research and Applications 27, 238-246.
| Crossref | Google Scholar |
Wassens S, Michael D, Spencer J, Thiem J, Thomas R, Kobayashi T, Bourke G, Bino G, Brandis K, Turner A, Wright D, Heath J, Kuo W, Amos C, Hall A (2021) Commonwealth Environmental Water Office Monitoring, Evaluation and Research Program: Murrumbidgee River System Technical Report 2014-20. Commonwealth Environmental Water Office, Canberra, ACT, Australia.
Webb C, Joss J (1997) Does predation by the fish Gambusia holbrooki (Atheriniformes: Poeciliidae) contribute to declining frog populations? Australian Zoologist 30, 316-324.
| Crossref | Google Scholar |
Wedderburn SD, Barnes TC, Hillyard KA (2014) Shifts in fish assemblages indicate failed recovery of threatened species following prolonged drought in terminating lakes of the Murray–Darling Basin, Australia. Hydrobiologia 730, 179-190.
| Crossref | Google Scholar |
Winfree R, Fox JW, Williams NM, Reilly JR, Cariveau DP (2015) Abundance of common species, not species richness, drives delivery of a real-world ecosystem service. Ecology Letters 18, 626-635.
| Crossref | Google Scholar | PubMed |