Accuracy and precision of sea-finding orientation as a function of dune proximity in hatchlings of two species of sea turtles
Shigetomo Hirama
A Fish and Wildlife Research Institute, Florida Fish and Wildlife Conservation Commission, 1105 SW Williston Road, Gainesville, FL 32601, USA.
B Department of Wildlife Ecology and Conservation, University of Florida, 110 Newins–Ziegler Hall, PO Box 110430, Gainesville, FL 32611, USA.
C Inwater Research Group Inc., 4160 NE Hyline Drive, Jensen Beach, FL 34957, USA.
D Loggerhead Marinelife Center, 14200 US Highway 1, Juno Beach, FL 33408, USA.
E US Geological Survey, Florida Cooperative Fish and Wildlife Research Unit, PO Box 110485, Gainesville, FL 32611, USA.
Marine and Freshwater Research 74(11) 994-1001 https://doi.org/10.1071/MF23052
Submitted: 23 March 2023 Accepted: 3 June 2023 Published: 26 June 2023
© 2023 The Author(s) (or their employer(s)). Published by CSIRO Publishing. This is an open access article distributed under the Creative Commons Attribution-NonCommercial-NoDerivatives 4.0 International License (CC BY-NC-ND)
Abstract
Context: Sea turtle hatchlings generally emerge at night from nests on sand beaches and immediately orient using visual cues, which are believed to entail the difference in brightness between the light seen in the seaward direction and that seen in the duneward direction.
Aim: The aim of this study was to understand how dune proximity affected hatchling orientations in two sea turtle species that share a nesting beach 15 km long and 25.3 ± 9.4 m (N = 215) from dune to waterline, with low to moderate artificial light nearby.
Methods: For hatchling loggerhead and green turtles, we measured accuracy and precision of orientation, tested differences in distance from nest to dune, and investigated the effect of dune proximity on hatchling orientation.
Key results: We found a significant decrease in hatchling orientation accuracy and precision in both species as the distance increased from nests to dune. Loggerhead and green turtles showed similar orientation ability when in the same proximity to the dune.
Conclusions: We conclude that dune features provide important cues for hatchling orientation on sea turtle nesting beaches.
Implications: Restoring and maintaining natural beach profiles, especially dune systems, is likely to increase the accuracy and precision of sea finding in hatchling sea turtles.
Keywords: artificial light, coastal pioneer plants, dune proximity, green turtle, hatchling orientation, hatchling track, light pollution, loggerhead.
Introduction
Wildlife, especially migratory animals, orient or navigate to certain locations for feeding, sheltering and nesting by sensing a variety of cues. Whereas specific cues and methods of perception differ, animals generally rely on cues that are constant and steady with little to no variability. For example, a supratidal amphipod (Talorchestia longicornis) uses sun compass orientation to move to its home beach (Forward et al. 2009). The red honey ant (Melophorus bagoti) uses the contrast between the sky and ultraviolet light reflected on terrestrial objects for homing and finding food (Schultheiss et al. 2016). Whereas some animals use a single cue to navigate or orient, others use combinations of signals. Orientation cues in the sandhoppers (Talitrus saltator) were affected by season, global radiation, solar direction, distance to the river mouth and severity of shoreline erosion (Ugolini et al. 1991; Scapini et al. 2005). Merlin et al. (2012) found that migratory insects use sun compass as a main cue, with the night sky or Earth’s magnetic field as a supportive cue, whereas the earwig (Labidura riparia) uses both celestial and solar light cues to orient toward its home shore (Ugolini and Chiussi 1996). A range of animals (migratory, non-migratory, terrestrial, aquatic) uses the earth’s magnetic field to orient and navigate (Lohmann 2010). For instance, brown planthoppers (Nilaparvata lugens), spiny lobsters (Panulirus argus), pink salmon (Oncorhynchus gorbuscha) and yellow stingrays (Urobatis jamaicensis) all migrate using the earth’s magnetic field (Boles and Lohmann 2003; Ernst and Lohmann 2016; Newton and Kajiura 2017; Putman et al. 2020; Zhang and Pan 2021). Sea turtles also have magnetoreception, which they use for navigation (Lohmann et al. 2012).
Sea turtles’ abilities to orient and navigate are crucial. Immediately after entering the sea, hatchlings will swim away from the shore using wave action which involves sensing the wave’s orbital movement, diving into the waves, acquiring offshore-ward movement and swimming perpendicular to the line of a swell (Wyneken et al. 1990; Lohmann et al. 1995). After a turtle passes the wave refraction zone, the navigational cue shifts to the earth’s magnetic field. Hatchlings are believed to be able sense different magnetic inclination angles, which enables them to detect parameters correlated with latitude (Lohmann et al. 2012). Turtles use the earth’s magnetic field to navigate, including offshore movements during the post-hatching frenzy period, finding neritic developmental habitats as juveniles and subadults, and, in adult females, moving between nesting beaches and foraging grounds (Lohmann et al. 2008a, 2008b). Turtles use the magnetic field in almost all life stages, apart from emerging hatchlings crawling from nest to sea and post-nesting females’ return to the sea. Nesting turtles may use similar visual orientation cues as hatchlings during this time, given that in light-polluted locations, some disorient at the beach, unable to reach the sea (Witherington 1992; Florida Fish and Wildlife Conservation Commission 2023). After emerging from a nest, hatchlings find the sea using a visual cue (Daniel and Smith 1947; Mrosovsky and Shettleworth 1968).
Among multiple possible cues that sea turtle hatchlings might use to find the sea after emerging from the nest, visual cues have the greatest support from experimental evidence (Mrosovsky and Shettleworth 1968; Tuxbury and Salmon 2005). Sea turtle hatchlings typically emerge from nests at night (Mrosovsky 1968) after an incubation period of 45–80 days (Miller 1997). Immediately after emerging, hatchlings should crawl vigorously to the sea, the ideal path being a straight line from the point of emergence to the closest point on the shoreline. But on a light-polluted beach, hatchlings can crawl aimlessly around the beach or not reach the sea (McFarlane 1963; Peters and Verhoeven 1994). Crawling along an indirect path wastes limited energy and risks exhaustion and dehydration (Lorne and Salmon 2007), as well as predation (Tomillo et al. 2010). Hatchling orientation accuracy has also been shown to increase as the distance from nests to dune decreases, whereas the slope of the beach showed no correlation with orientation (Hirama et al. 2021). Loggerhead hatchlings crawled away from artificially created dark silhouettes in both laboratory and in situ experiments (Parker 1922; Salmon et al. 1992; Tuxbury and Salmon 2005). These findings indicate that tactile senses, activated by gravity, as when a hatchling descends a slope, serve little importance to navigation, whereas that the dark silhouette from the dune is important for seaward orientation (Limpus and Kamrowski 2013; Hirama et al. 2021).
Since the first publication of sea turtle hatchling orientation studies in 1908 (Hooker 1908), no study has compared orientation on loggerhead and green turtles in one location. Understanding the orientation performance of hatchlings by species is important in assessing the need for and effects of management practices for nesting beaches, such as sand nourishment, which changes a beach profile, and often turtle nesting behaviours as well, leading to, for example, nesting farther away from the dune (Brock et al. 2009). The purpose of our study was to assess the effects of dune proximity on hatchling orientation in two species of sea turtles, loggerheads (Caretta caretta) and green turtles (Chelonia mydas), on the same nesting beach. We hypothesised that turtles nesting closer to the dunes, which appear as a dark silhouette, would exhibit greater orientation accuracy and that nesting locations on the beach would differ between the two species, the premise being that, because the two species perceive light similarly, differences in their ability to orient are more likely related to species-specific nesting spot preferences (Witherington 1986; Wetterer et al. 2007).
Materials and methods
Study sites and hatchling orientation parameters
Our study site was an ~15-km stretch of the sea turtle nesting beach at Juno Beach (hereafter, Juno; 26.839°N, 80.041°W to 26.970°N, 80.080°W), Palm Beach County, FL, USA. Juno is one of few beaches in the world on which three sea turtle species (loggerhead, green and leatherback turtles, Dermochelys coriacea) nest in significant numbers. Juno is in northern Palm Beach County, where light pollution (from artificial light and sky glow) is milder than in the central to southern areas closer to Broward and Miami–Dade counties, which are more densely developed and have more severe light pollution (see https://www.lightpollutionmap.info, accessed 3 November 2022).
However, on the stretch at Juno mentioned above, no obvious area was visually appraised as brighter than others, and the two species of turtle nested evenly across the study area without complete intermixing, but also without noticeable clustering (Supplementary Fig. S1). We collected data equally across all areas for both species, resulting in a spatially random sample. In 2016–2018, we collected orientation data on hatchling tracks, following earlier studies (Salmon and Witherington 1995; Berry et al. 2013; Kamrowski et al. 2014; Dimitriadis et al. 2018, and especially Hirama et al. 2021). Hatchling orientation data were collected from 180 loggerhead, 56 green and 15 leatherback turtle nests. Because so few leatherback nests were found at the site, we omitted the species from further analysis but included the descriptive statistics of the orientation data (Supplementary Table S1). We measured two parameters representing hatchling orientation: (1) angular range, which depicts the spread of hatchling tracks and thus indicates precision of orientation, and (2) modal divergence, which shows the difference between the predominant direction in which hatchlings crawled (hereafter, mode) and the direction as tangent line to the sea, used to quantify orientation accuracy. From a hatchling emergence point, we marked a 10-m-radius circle (hereafter, testing circle) and measured the bearing of three hatchling tracks, one for a mode direction and two for calculating an angular range, where they intercepted the testing circle (Hirama et al. 2021). Kamrowski et al. (2014) considered the midpoint of bearing within an angular range as a mode direction. We determined mode direction by visually evaluating the tracks (Hirama et al. 2021). Although being somewhat subjective, this provides important information about the tendency in hatchling orientation and is better than using a bisected point of an angular range, given that a density distribution of tracks exiting the circle is often uneven. The modal divergence would be 0° (the most accurate) when the mode direction coincided with the ocean direction and 180° (the least accurate) when a mode direction was opposite to the ocean direction. Modal divergence is calculated as the absolute value of the difference between ocean and mode directions, but it does not indicate in which of the two possible directions the mode lies. For example, if an ocean direction was toward the east cardinal direction (90°) and modal divergence value was 90°, the mode direction can be toward the north (0°) or the south (180°) cardinal directions. Plotting the mode direction clarifies its directional skewness (Fig. 1). For all nests, no rocks or other obstacles were present that might influence the routes of hatchlings toward the sea; however, for some nests, vegetation was present inside the testing circle.
Circular plots of hatchling modal distributions for loggerheads (a; n = 178) and green turtles (b; n = 50). The dots outside the circle show the bearings of modal directions of nest emergences. The black arrow shows the average of modal directions, represented by the black dots. The grey rose diagram inside the circle indicates the ocean direction relative to nest locations. The grey arrow shows the average ocean direction. A dot located farther from the grey arrow depicts a proportionally lower orientation accuracy.
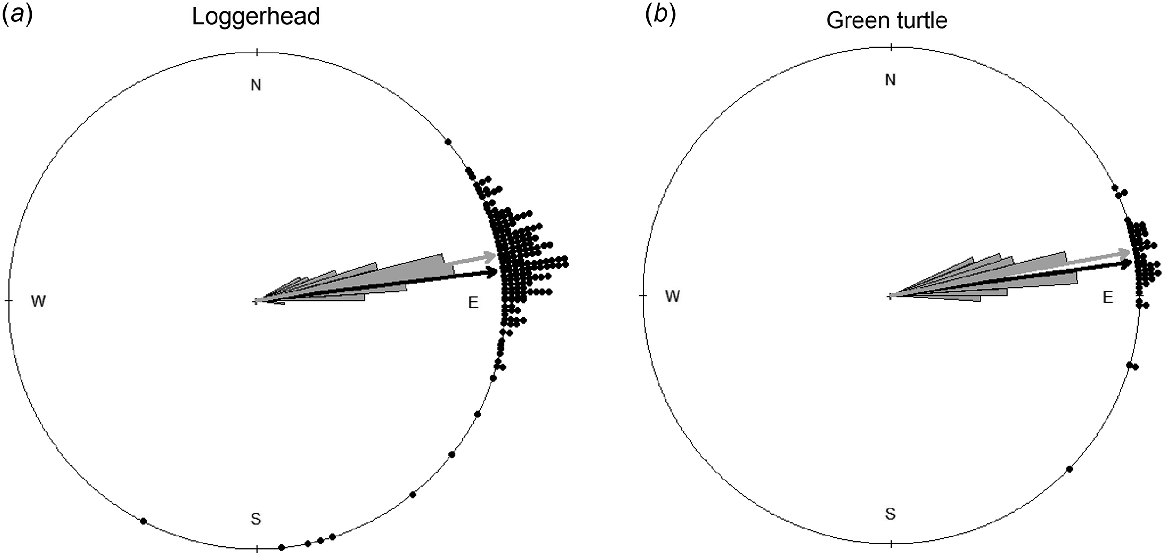
Hatchling track orientation was measured at a subsample of nests marked for nest-productivity assessment (Brost et al. 2015). In Juno, productivity was assessed for every 20th nest location for loggerheads and for all green turtle and leatherback nests. At these nests, surveyors searched the beach for hatchling tracks and used a compass in a phone app (accuracy confirmed against a traditional sighting compass) to measure angular range and modal divergence, using the nest (i.e. the track origin) as the central point. We collected data from a subsample of the marked nests because some nests were unsuccessful, with no emerging hatchlings. We ignored nests with fewer than five hatchling tracks (Pendoley 2005; Berry et al. 2013; Kamrowski et al. 2014; Hirama et al. 2021) or for which tracks had been erased by wind or trampling. The distance from a nest to the vegetation line that shows the toe of the dune (hereafter, distance from nest to dune) was measured at the time of hatchling emergence by using a laser range finder (Laser Technology: TruPulse 200). On the southern and central eastern coasts of Florida, the borders of dune vegetation lines are rather obvious and relate well with dune toe locations and have been associated with hatchling orientation (Hirama et al. 2021). Although not completely intermixed, the nests of each species were located evenly throughout the study site, and the data were collected throughout the hatchling emergence season; therefore, abiotic factors, such as sand colour, cloud cover percentage, dune height, dune vegetation density, slope steepness, ocean tide and the density of human development, were not considered in our analyses. We truncated the explanatory variable, distance from nest to dune, at −2 m. Truncating excluded nests that were at or behind the dune (n = 8 nests removed, two loggerhead and six green turtle). To account for moon illumination during the study period, we categorised the observation dates by eight moon phases by using the ‘lunar’ package (ver. 0.2-01, E. Lazaridis, see https://cran.r-project.org/web/packages/lunar/) in R (ver. 4.0.3, R Foundation for Statistical Computing, Vienna, Austria, see https://www.R-project.org/) to include in our statistical models.
Statistical analyses
To test whether nest locations between the two species differed with respect to distance from nest to dune, we used a two-sample Mann–Whitney U test. A non-parametric test was chosen because of the non-normality of the data and unequal variances between the two species. Next, to assess the influence of distance from nest to dune on modal divergence and angular range for each species, we fit generalised linear mixed-effects models with angular ranges and modal divergences as response variables and distance from nest to dune as the explanatory variable, using R (ver. 4.0.3). Given the highly skewed and discrete (integer) nature of the data (Table 1), we assumed a negative-binomial distribution. We included a random effect, lunar phase to allow for any variation in hatchling orientation due to the compounding of moon and artificial light on beaches (Mrosovsky and Carr 1967; Salmon and Witherington 1995). Models were fitted separately for loggerhead and green turtles, because their nesting locations relative to the distance to the dune did not overlap across the range (Fig. 2) and predictions outside of these ranges would be inappropriate. We confirmed uniformity in the modal hatchling directional distribution for each species with Rayleigh circular tests, using the ‘circular’ R package (ver. 0.4-93, U. Lund, C. Agostinelli, H. Arai, A. Gagliardi, E. García-Portugués, D. Giunchi, J.-O. Irisson, M. Pocernich and F. Rotolo, see https://cran.r-project.org/web/packages/circular/). Finally, to directly assess differences in modal divergence and angular range between the two species, we fit negative-binomial regression models. We retained the random effect of lunar phase for the modal divergence model, but did not include a random effect in the angular range model because there was not enough lunar phase-level variation to warrant a random effect. To compare orientation between species, we truncated the original data set to include only overlapping distances from nest to dune for loggerhead (n = 46) and green (n = 43) turtle nests. We retained overlapping distances from nest to dune for nests ranging from −2 to 6.0 m (Fig. 3). We considered 95% confidence intervals of parameter estimates that did not overlap zero to be significant for all regression models and an alpha = 0.05 for all other statistical tests.
Angular range (°) | Modal divergence (°) | Distance from nest to dune (m) | ||||||||
---|---|---|---|---|---|---|---|---|---|---|
Species | Sample size | Mean ± s.e. | Median | Min. to max. | Mean ± s.e. | Median | Min. to max. | Mean ± s.e. | Median | Min. to max. |
Loggerhead | 178 | 70 ± 0.3 | 49 | 6–360 | 14 ± 0.1 | 7 | 0–126 | 12.4 ± 0.7 | 10.1 | −1.2 to 55.8 |
Green turtle | 50 | 42 ± 0.4 | 40 | 13–113 | 7 ± 0.3 | 4 | 0–106 | 3.5 ± 0.8 | 1.7 | −1.8 to 34.1 |
Both species | 228 | 64 ± 0.2 | 46 | 6–360 | 13 ± 0.1 | 6 | 0–126 | 10.4 ± 0.6 | 8.7 | −1.8 to 55.8 |
The modelled relationship between hatchling orientation precision, expressed as angular range and distance from nest to dune for loggerhead (n = 178; a) and green (n = 50; c) turtles. The modelled relationship between accuracy, expressed as modal divergence, and the distance from nest to dune for loggerhead (b) and green (d) turtles. The black lines represent marginal mean predictions from the regression models, and the grey shading depicts their 95% confidence intervals, conditioned on the fixed-effects terms. All models were run individually, whereas each panel represents separete model results. Figures are presented together for ease in comparison.
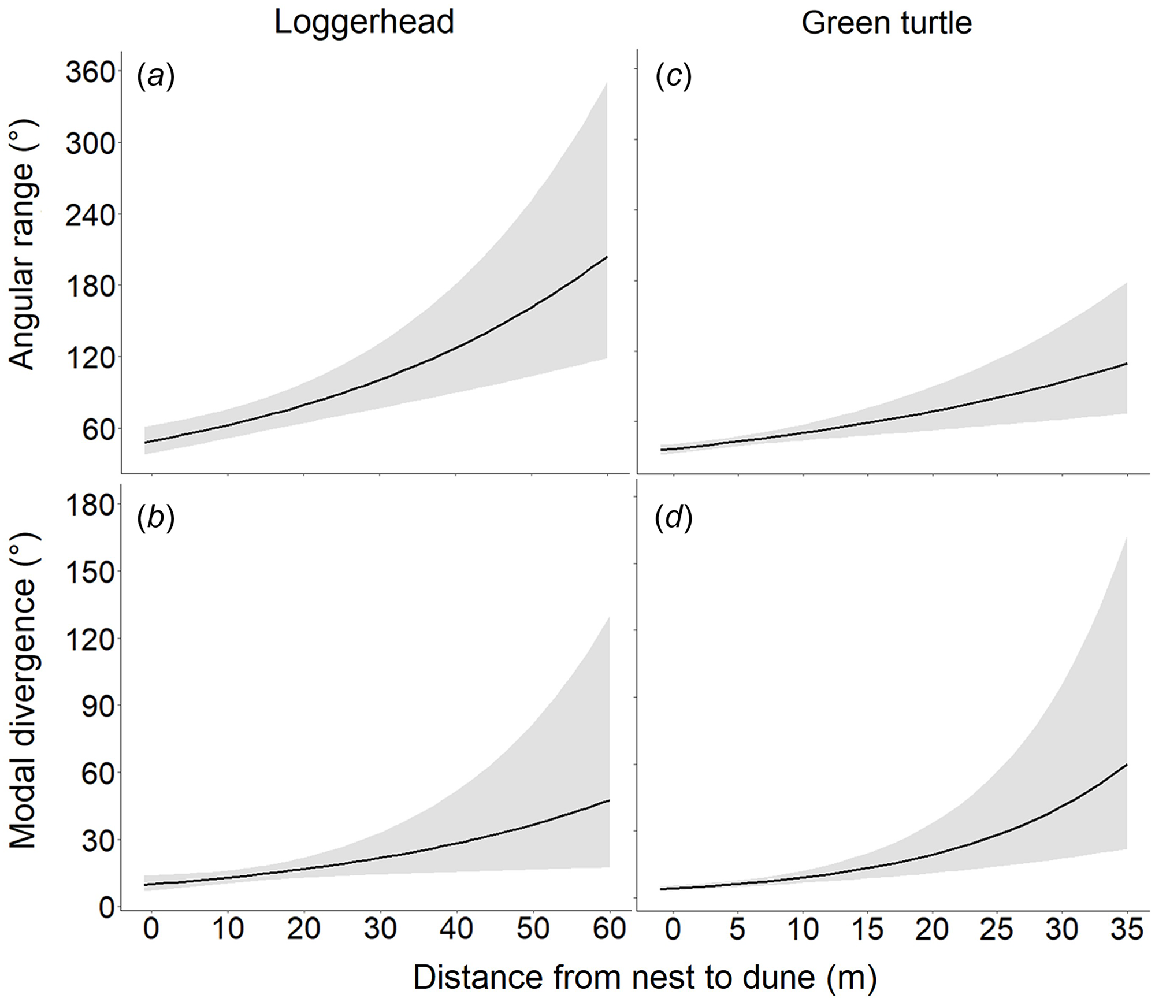
Density of sea turtle nest locations at Juno Beach, FL, USA, in relation to distance from nest to dune (value 0 on the x-axis shows the vegetation line, approximately equivalent to the toe of the dune), by species: loggerhead, n = 178, mean = 12.4 m, median = 9.0 m, range = −1.2–55.8 m, s.d. = 9.0 m; green turtle, n = 50, mean = 3.6 m, median = 1.7 m, range = −1.8–34.1 m, s.d. = 5.6 m. The two red vertical lines show the range (−1.8–6 m) of truncated data, depicting the overlap between green turtle and loggerhead nest locations with respect to the dune vegetation line.
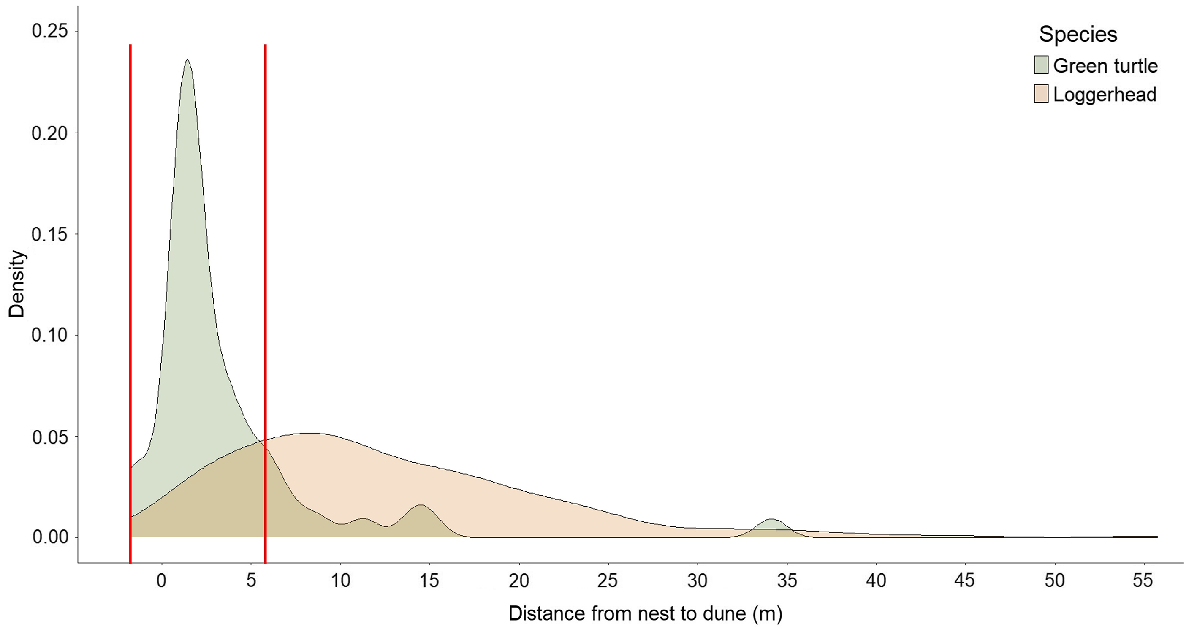
Ethical approval
The study does not contain any experiment using animals by any of the authors. This study was performed under the authorisation of Florida Fish and Wildlife Conservation Commission Marine Turtle Permit MTP-154. Any use of trade, firm or product names is for descriptive purposes only and does not imply endorsement by the US government.
Results
We collected the two orientation parameters from hatchlings emerging from 178 loggerhead and 50 green turtle nests (Table 1). Distributional plots of nest locations for the two species in relation to the dune exhibited apparent differences (Fig. 2). The mean distance from nest to dune for loggerhead nests was 12.37 m ± 0.68 s.e. and ranged from −1.20 to 55.78 m. For green turtles, mean distance from nest to dune was 3.55 m ± 0.79 s.e. and ranged from −1.83 to 34.14 m. The mean distance from nest to dune for the two species differed significantly (W = 7652.50, P < 0.001; Fig. 4), and uniformity tests indicated that the mode direction of each species showed non-randomness (loggerheads: Z85 = 0.95, P < 0.001, Fig. 1a; green turtles: Z85 = 0.98, P < 0.001, Fig. 1b).
Box plots of loggerhead (n = 178) and green (n = 50) turtle nest locations in relation to distance from nest to dune at Juno Beach, FL, USA. The line inside each box represents the 50th percentile; the top and bottom sides of the box represent respectively, the 75th and 25th percentiles, which define the interquartile range, and the whiskers depict the interquartile range multiplied by 1.5. Differing letters denote significant differences between the species’ nesting locations.
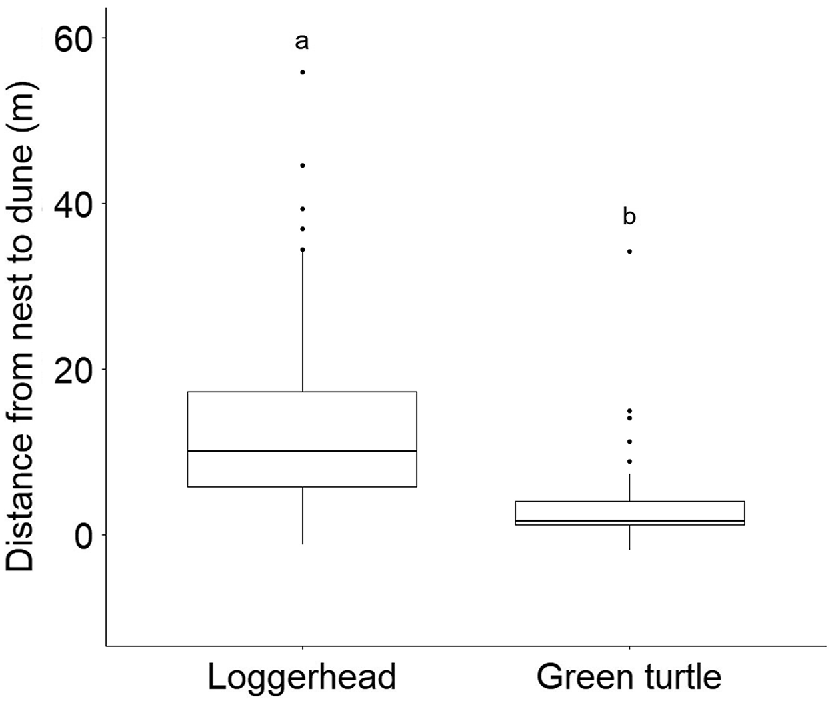
The mean angular range and modal divergence for loggerheads were respectively 69.56° (±0.32° s.e.) and 14.34° (±0.12° s.e.). The mean angular range for green turtles was 41.96° (±0.35° s.e.) and the mean modal divergence was 7.18° (±0.30° s.e.). Angular range and modal divergence were significantly associated with distance from nest to dune, and orientation accuracy and precision decreased with an increasing distance from nest to dune for both species (Supplementary Table S2). At overlapping dune distances, loggerhead and green turtles did not differ statistically in angular range (Supplementary Table S3). However, modal divergence was significantly lower for green turtles, −33.18% (95% confidence interval −53.37 to −4.26%; Table S3) than for loggerheads at overlapping distances.
Discussion
Given that the orientation accuracy and precision of sea turtle hatchlings increased with nest proximity to the dune, and given the sensitivity of hatchlings to horizon brightness, we presume that a dark dune silhouette is equally important to the seaward orientation for the two species. The importance of dunes in loggerhead hatchling orientation has been confirmed (Parker 1922; Salmon et al. 1992; Tuxbury and Salmon 2005; Limpus and Kamrowski 2013; Hirama et al. 2021), but evidence is lacking for green turtles. Although variation in orientation parameters was high, especially with increasing dune distance, and there were differences between species in nest position relative to the dune, our results indicated that the two species have comparable orientation abilities at similar distances from the dune.
Overall, green turtles showed more accurate and precise hatchling orientation. Because green turtles nest closer to the dune than do loggerheads, it is possible that green turtle hatchlings are more likely to receive stronger light cues of the dark dune silhouette, which contrasts with the brighter, open, seaward horizon. For both species, orientation was better for hatchlings whose nests were closer to the dune. These results do not resolve the hierarchy of possible orientation cues from silhouette patterns and brightness (Limpus 1971; Van Rhijn 1979; Limpus and Kamrowski 2013) but do support the hypothesis that that the dune’s form or brightness characteristics have an important influence on hatchling orientation.
Mechanisms by which sea turtle hatchlings measure light fields during seaward orientation have been hypothesised for light fields that vary over the same horizontal plane where orientation takes place (Mrosovsky and Shettleworth 1968; Mrosovsky and Kingsmill 1985) but have not been hypothesised for light fields with varied light elevations or with complex shapes (Limpus and Kamrowski 2013). Dunes are likely to dominate light fields on beaches with these latter complications. We were able to measure the visual effects of dunes on hatchling orientation only by observing effects on orientation relative to dune distance. Although we did not measure orientation direction farther than 10 m from the nest, we do not reason that a larger radius would have significantly changed accuracy and precision measures, but it would have created challenges as the radius intersected with the wavewash. The hatchling tracks inside our 10-m testing-circle were all straight, except for a few hatchlings that briefly crawled along the wrack line. We expect that the 10-m radius that defines our orientation measurements captured several light-field assessments by hatchlings. These assessments are thought to occur during periodic stops when hatchlings show an inspection stance (Carr and Ogren 1960). At these brief pauses, depending on the perceived light field, hatchlings change or remain on their courses. One condition within the 10-m radius that had the potential to affect orientation was the presence of dune vegetation. Some nests were located landward of the dune toe, and hatchlings there had to traverse vegetation that might have altered their crawl direction. However, our results indicated that these plants did not disrupt orientation cues or hinder crawling direction, although the crawling path required to reach the sea was slightly longer than for a clear path.
We conclude that the dune, which on sea-turtle nesting beaches provides a dark silhouette that contrasts with the seaward horizon, is an important environmental cue for hatchling orientation, confirming assessments by other authors (Parker 1922; Salmon et al. 1992; Limpus and Kamrowski 2013). Because dune height at Juno was uniform, we consider that spatial variation on the dune had a negligible influence on our findings. The applicability of our results to hatchling orientation on other beaches may depend on important variables such as the amount of artificial light and how artificial light sources are blocked by dunes. We hypothesise that, on beaches with minimal artificial light, dune proximity would have similar effects on orientation as those seen at Juno. Hirama et al. (2021) found that on a naturally lighted beach, the distance from nest to dune was the most influential of several environmental factors considered. Other studies showed that moon-illumination percentage (i.e. moon phase) significantly affected hatchlings’ seaward orientation (Salmon and Witherington 1995; Berry et al. 2013). In the present study, we accounted for these differences by including moon phase as a random effect in the model, if statistically supported.
The findings of the present study suggest that beach-management strategies, namely those protecting coastal pioneer plants such as sea oats (Uniola paniculata), sea grape (Coccoloba uvifera) and saw palmetto (Serenoa repens) that promote dune formation and heighten the silhouette of the dune, blocking more ambient light, might be implemented in all cases because of the importance of the dune as a guiding feature for hatchling loggerhead and green turtles, and likely for other sea turtle species that were not included in the present study.
Data availability
The data that support this study will be shared upon reasonable request to the corresponding author.
Declaration of funding
Funding for this project was provided by the Archie Carr Center for Sea Turtle Research (ACCSTR) at the University of Florida through support from the Disney Conservation Fund (DCF) to reduce threats to Florida’s sea turtles. Neither ACCSTR nor DCF are in possession of the data.
Acknowledgements
We thank D. Aoki, K. Ariotti, S. Bolds, W. Brown, B. Canitz, G. Carvajal, S. Colios, A. Ferrera, K. Fraser, A. Frye, R. Grimley, S. Hoover, R. Johnson, K. Kantra, S. Kedzuf, E. Kelly, E. Kluga, A. McCracken, M. O’Briant, J. Reilly, K. Rigano, H. Seaman, J. Stuczynski, L. Wenk and M. Wilgus for the field assistance. We thank B. Bankovich, K. Bjorndal, S. Johnson and E. Lada for providing technical guidance. We thank S. A. Ceriani, B. Crowder, A. M. Foley and S. Johnson for reviewing the manuscript.
References
Berry M, Booth DT, Limpus CJ (2013) Artificial lighting and disrupted sea-finding behaviour in hatchling loggerhead turtles (Caretta caretta) on the Woongarra coast, south-east Queensland, Australia. Australian Journal of Zoology 61, 137-145.
| Crossref | Google Scholar |
Boles LC, Lohmann KJ (2003) True navigation and magnetic maps in spiny lobsters. Nature 421, 60-63.
| Crossref | Google Scholar |
Brock KA, Reece JS, Ehrhart LM (2009) The effects of artificial beach nourishment on marine turtles: differences between loggerhead and green turtles. Restoration Ecology 17, 297-307.
| Crossref | Google Scholar |
Brost B, Witherington B, Meylan A, Leone E, Ehrhart L, Bagley D (2015) Sea turtle hatchling production from Florida (USA) beaches, 2002–2012, with recommendations for analyzing hatching success. Endangered Species Research 27, 53-68.
| Crossref | Google Scholar |
Carr A, Ogren L (1960) The ecology and migration of sea turtles 4: the green turtle in the Caribbean Sea. Bulletin of the American Museum of Natural History 121, 1 Available at http://hdl.handle.net/2246/1602.
| Google Scholar |
Daniel RS, Smith KU (1947) The sea-approach behavior of the neonate loggerhead turtle, Caretta caretta. Journal of Comparative and Physiological Psychology 40, 413-420.
| Crossref | Google Scholar |
Dimitriadis C, Fournari-Konstantinidou I, Sourbes L, Koutsoubas D, Mazaris AD (2018) Reduction of sea turtle population recruitment caused by nightlight: evidence from the Mediterranean region. Ocean & Coastal Management 153, 108-115.
| Crossref | Google Scholar |
Ernst DA, Lohmann KJ (2016) Effect of magnetic pulses on Caribbean spiny lobsters: implications for magnetoreception. Journal of Experimental Biology 219, 1827-1832.
| Crossref | Google Scholar |
Florida Fish and Wildlife Conservation Commission (2023) Sea turtle disorientations. (FWC: Tallahassee, FL, USA) Available at https://myfwc.com/wildlifehabitats/wildlife/sea-turtle/lighting/disorientations/
Forward RB, Jr, Bourla MH, Lessios NN, Cohen JH (2009) Orientation to shorelines by the supratidal amphipod Talorchestia longicornis: wavelength specific behavior during sun compass orientation. Journal of Experimental Marine Biology and Ecology 376, 102-109.
| Crossref | Google Scholar |
Hirama S, Witherington B, Kneifl K, Sylvia A, Wideroff M, Carthy R (2021) Environmental factors predicting the orientation of sea turtle hatchlings on a naturally lighted beach: a baseline for light-management goals. Journal of Experimental Marine Biology and Ecology 541, 151568.
| Crossref | Google Scholar |
Hooker D (1908) The breeding habits of the loggerhead turtle and some early instincts of the young. Science 27, 490-491.
| Google Scholar |
Kamrowski RL, Limpus C, Pendoley K, Hamann M (2014) Influence of industrial light pollution on the sea-finding behaviour of flatback turtle hatchlings. Wildlife Research 41, 421-434.
| Crossref | Google Scholar |
Limpus CJ (1971) Sea turtle ocean finding behaviour. Search 2, 385-387.
| Google Scholar |
Limpus C, Kamrowski RL (2013) Ocean-finding in marine turtles: the importance of low horizon elevation as an orientation cue. Behaviour 150, 863-893.
| Crossref | Google Scholar |
Lohmann KJ (2010) Q&A: animal behaviour: magnetic-field perception. Nature 464, 1140-1142.
| Crossref | Google Scholar |
Lohmann KJ, Swartz AW, Lohmann CMF (1995) Perception of ocean wave direction by sea turtles. Journal of Experimental Biology 198, 1079-1085.
| Crossref | Google Scholar |
Lohmann KJ, Luschi P, Hays GC (2008a) Goal navigation and island-finding in sea turtles. Journal of Experimental Marine Biology and Ecology 356, 83-95.
| Crossref | Google Scholar |
Lohmann KJ, Putman NF, Lohmann CMF (2008b) Geomagnetic imprinting: a unifying hypothesis of long-distance natal homing in salmon and sea turtles. Proceedings of the National Academy of Sciences 105, 19096-19101.
| Crossref | Google Scholar |
Lohmann KJ, Putman NF, Lohmann CMF (2012) The magnetic map of hatchling loggerhead sea turtles. Current Opinion in Neurobiology 22, 336-342.
| Crossref | Google Scholar |
Lorne JK, Salmon M (2007) Effects of exposure to artificial lighting on orientation of hatchling sea turtles on the beach and in the ocean. Endangered Species Research 3, 23-30.
| Crossref | Google Scholar |
McFarlane RW (1963) Disorientation of loggerhead hatchlings by artificial road lighting. Copeia 1963, 153.
| Crossref | Google Scholar |
Merlin C, Heinze S, Reppert SM (2012) Unraveling navigational strategies in migratory insects. Current Opinion in Neurobiology 22, 353-361.
| Crossref | Google Scholar |
Mrosovsky N (1968) Nocturnal emergence of hatchling sea turtles: control by thermal inhibition of activity. Nature 220, 1338-1339.
| Crossref | Google Scholar |
Mrosovsky N, Carr A (1967) Preference for light of short wavelengths in hatchling green sea turtles, Chelonia mydas tested on their natural nesting beaches. Behaviour 28, 217-231.
| Crossref | Google Scholar |
Mrosovsky N, Kingsmill SF (1985) How turtles find the sea. Zeitschrift für Tierpsychologie 67, 237-256.
| Crossref | Google Scholar |
Mrosovsky N, Shettleworth SJ (1968) Wavelength preferences and brightness cues in the water finding behaviour of sea turtles. Behaviour 32, 211-257.
| Crossref | Google Scholar |
Newton KC, Kajiura SM (2017) Magnetic field discrimination, learning, and memory in the yellow stingray (Urobatis jamaicensis). Animal Cognition 20, 603-614.
| Crossref | Google Scholar |
Parker GH (1922) The crawling of young loggerhead turtles toward the sea. Journal of Experimental Zoology 36, 322-331.
| Crossref | Google Scholar |
Peters A, Verhoeven KJF (1994) Impact of artificial lighting on the seaward orientation of hatchling loggerhead turtles. Journal of Herpetology 28, 112-114.
| Crossref | Google Scholar |
Putman NF, Williams CR, Gallagher EP, Dittman AH (2020) A sense of place: pink salmon use a magnetic map for orientation. Journal of Experimental Biology 223, jeb218735.
| Crossref | Google Scholar |
Salmon M, Witherington BE (1995) Artificial lighting and seafinding by loggerhead hatchlings: evidence for lunar modulation. Copeia 1995, 931-938.
| Crossref | Google Scholar |
Salmon M, Wyneken J, Fritz E, Lucas M (1992) Seafinding by hatchling sea turtles: role of brightness, silhouette and beach slope as orientation cues. Behaviour 122, 56-77.
| Crossref | Google Scholar |
Scapini F, Chelazzi L, Colombini I, Fallaci M, Fanini L (2005) Orientation of sandhoppers at different points along a dynamic shoreline in southern Tuscany. Marine Biology 147, 919-926.
| Crossref | Google Scholar |
Schultheiss P, Wystrach A, Schwarz S, Tack A, Delor J, Nooten SS, Bibost A-L, Freas CA, Cheng K (2016) Crucial role of ultraviolet light for desert ants in determining direction from the terrestrial panorama. Animal Behaviour 115, 19-28.
| Crossref | Google Scholar |
Tomillo PS, Paladino FV, Suss JS, Spotila JR (2010) Predation of leatherback turtle hatchlings during the crawl to the water. Chelonian Conservation and Biology 9, 18-25.
| Crossref | Google Scholar |
Tuxbury SM, Salmon M (2005) Competitive interactions between artificial lighting and natural cues during seafinding by hatchling marine turtles. Biological Conservation 121, 311-316.
| Crossref | Google Scholar |
Ugolini A, Chiussi R (1996) Astronomical orientation and learning in the earwig Labidura riparia. Behavioural Processes 36, 151-161.
| Crossref | Google Scholar |
Ugolini A, Felicioni S, Macchi T (1991) Orientation in the water and learning in Talitrus saltator Montagu. Journal of Experimental Marine Biology and Ecology 151, 113-119.
| Crossref | Google Scholar |
Van Rhijn FA (1979) Optic orientation in hatchlings of the sea turtle, Chelonia mydas. I. Brightness: not the only optic cue in sea-finding orientation. Marine Behaviour and Physiology 6, 105-121.
| Crossref | Google Scholar |
Wetterer JK, Wood LD, Johnson C, Krahe H, Fitchett S (2007) Predaceous ants, beach replenishment, and nest placement by sea turtles. Environmental Entomology 36, 1084-1091.
| Crossref | Google Scholar |
Witherington BE (1992) Behavioral responses of nesting sea turtles to artificial lighting. Herpetologica 48, 31-39.
| Google Scholar |
Wyneken J, Salmon M, Lohmann KJ (1990) Orientation by hatchling loggerhead sea turtles Caretta caretta L. in a wave tank. Journal of Experimental Marine Biology and Ecology 139, 43-50.
| Crossref | Google Scholar |
Zhang Y, Pan W (2021) Removal or component reversal of local geomagnetic field affects foraging orientation preference in migratory insect brown planthopper Nilaparvata lugens. PeerJ 9, e12351.
| Crossref | Google Scholar |