Movement patterns of Murray cod (Maccullochella peelii) and golden perch (Macquaria ambigua) in a northern Murray–Darling Basin dryland river
D. J. Harding


A
B
C
Abstract
Dryland rivers are unique ecosystems, where drought and flood play an important role in shaping the ecosystem. River regulation has altered the natural flow regime in many of these systems, affecting migration cues and connectivity for many species.
To quantify the discharge-related movements of Murray cod and golden perch within the Condamine–Balonne River subject to differing levels of river regulation.
We quantified flow regime variability, river regulation and fish movement to develop generalised additive mixed models to predict movement probability for Murray cod and golden perch.
Both species showed strong positive relationships between discharge and movement. Murray cod did not show any association with river regulation; however, medium-sized individuals were significantly more likely to move than were smaller or larger fish. Golden perch movements varied among levels of regulation, were more likely to move as body weight increased and showed seasonality of movement, moving less during winter.
This study presents the largely unobserved movement behaviours of fish across a gradient of river regulation and environmental conditions in the northern Murray–Darling Basin.
This information is valuable for informing policy and management decisions that may affect species’ life-history requirements in analogous river systems.
Keywords: acoustic telemetry, dryland river, fish migration, flow regime variability, generalist species, movement ecology, river regulation, spawning cues, water management.
Introduction
Globally, anthropogenic changes to freshwater systems, such as the construction of dams and weirs and water abstraction, are having major impacts on the sustainability of riverine ecosystems (Dias et al. 2017). Seeing as human activities pose an increasing threat to freshwater ecosystems, free-flowing rivers are becoming increasingly scarce (Grill et al. 2019). Dryland rivers constitute unique ecosystems where the cyclic patterns of drought and flood play a pivotal role in shaping the environment and the species they support (Larkin et al. 2020). Water resource development in these hydrologically variable environments can negatively affect biota by altering the natural flow regime (Pennock et al. 2022). Freshwater fishes are among the most imperilled taxa, with river regulation, fragmentation, and flow regime change contributing to a decline in many populations (Burkhead 2012).
The Murray–Darling Basin (MDB) is an extensively regulated system with more than 5000 artificial barriers, (Lintermans 2023) and a large volume of water is removed for irrigation (Murray–Darling Basin Authority 2022). Numerous studies, primarily in the southern MDB, have quantified the flow-related ecology of key fish species present within the system and developed environmental flow recommendations to build resilience in these species (King et al. 2009; Koehn et al. 2014, 2019). However, there have been few studies evaluating multi-species, flow-related movements across a gradient of river regulation in the northern MDB. A greater understanding of fish flow ecology will improve the information available to support management of these systems.
The impact of river regulation is particularly noticeable on large-bodied native fish species (>250 mm at maturity) with critical links to components of the natural flow regime (Vertessy et al. 2019; Thiem et al. 2022). Key life-history processes such as dispersal (e.g. egg and larval drift and adult migrations), spawning, and recruitment are stimulated or facilitated by the timing, magnitude, frequency and duration of high- and low-flow events in systems where the majority of the natural flow regime remains intact (Poff et al. 1997). Conversely, highly regulated rivers are characterised by substantial changes to natural flow characteristics and loss of critical flow-related cues and conditions for spawning, recruitment and migrations (Harding et al. 2017, 2019; Tickner et al. 2020; Espinoza et al. 2021). Given the competing interests between water resource development and the management of fish populations in dryland river systems, with high flow variability, understanding the flow-related movement requirements of key native species is critical to sustainable development in the northern MDB (Koehn et al. 2019).
Murray cod (Maccullochella peelii) and golden perch (Macquaria ambigua) are two iconic large-bodied, long-lived fish species found throughout the MDB. Murray cod are Australia’s largest freshwater fish and a top predator and can reach a maximum length of 1800 mm and 113.6 kg, with the oldest known individual aged at 48 years (Koehn et al. 2020a). Golden perch can reach a maximum size of 760 mm and 23 kg, and a maximum age of 26 years (Mallen-Cooper and Stuart 2003; Koehn et al. 2020a). Murray cod has a restricted home range and high site fidelity, although small movements (e.g. 5–10 km) may occur out of refuge waterholes during elevated flows (Butler et al. 2022; Thiem et al. 2022; Carpenter-Bundhoo et al. 2023). Long-distance movements of adult Murray cod, up to 130 km, have been associated with spawning in late winter–spring, with return homing migrations being common (Koehn et al. 2009). Golden perch in comparison is highly mobile, commonly making long-distance upstream and downstream movements associated with spawning (Koehn and Nicol 2016; Koster et al. 2017). There are examples of 500–1000-km movements associated with high flows (Reynolds 1983). However, movement of tens to hundreds of kilometres followed by return homing migrations occur regularly (O’Connor et al. 2005; Marshall et al. 2016).
The current study aimed to quantify the discharge-related movements and migrations of Murray cod and golden perch within an ~700-km reach of the Condamine–Balonne River system, northernmost sections of the MDB. We used a broad-scale acoustic telemetry array to track the longitudinal movements of subadult and adult Murray cod and golden perch over 5 years. Specifically, we sought to understand the discharge drivers (magnitude and timing) of large-scale fish migrations (movements related to spawning or other critical life-history events) and general movements (movement among habitats within a fish’s lifetime) between sections of the river with varying degrees of flow regulation. We hypothesised that discharge events would cue a low number of tagged Murray cod to move and a large number of golden perch to move and that movements related to discharge would be greatest for both species in unregulated river reaches. Knowledge of discharge-related ecology and movement patterns of fish is essential to understand the impacts of river regulation and inform water management assessments in the northern MDB.
Materials and methods
Study area
This study was undertaken in the Condamine–Balonne River system located in the Queensland section of the Murray–Darling Basin (MDB), Australia (Fig. 1). The Condamine–Balonne catchment comprises ~13% of the MDB (Murray–Darling Basin Authority 2023) and begins in the Border Ranges in the east and flows south-west over 1195 km, to the New South Wales (NSW)–Queensland (Qld) border (Fig. 1). The catchment is typically arid to semiarid, with rainfall ranging from 400 to 1200 mm year−1 (Department of Agriculture, Water and the Environment 2023). The average annual flow in the mainstream is 1305 GL (St George) (Murray–Darling Basin Authority 2023), contributing ~8.5% to the total runoff for the MDB (Department of Agriculture, Water and the Environment 2023). The Condamine–Balonne River has been heavily developed for irrigated agriculture, particularly downstream of St George and upstream of Chinchilla (Fig. 1). However, between St George and Chinchilla, irrigated agriculture is not as prolific and gives way to grazing land. There is a large weir at Chinchilla (full supply volume 9780 ML) and a large weir and dam at St George (full supply volume 10,270 ML, 81,700 ML respectively) (Fig. 1). The study reach extended from upstream of Dalby in the north-east and continued ~700 km downstream of St George in the south (Fig. 1).
Hydrology and other environmental variables
The study reach was categorised into three levels of river regulation (high, moderate and low; Fig. 1). This assessment was based on an analysis of differences in key ecologically relevant flow metrics for representative locations, by using a simulated daily discharge time series (1900–2022) calculated for pre-development and full-development scenarios (Supplementary Table S1). Reporting nodes for water management were used as the representative locations across the study area, which corresponded to Gauging stations GS 422015, 422336A and 422213A (Department of Regional Development, Manufacturing and Water 2023). The full-development scenario was based on the sum of maximum extracted water volumes for all authorisations and dams, whereas the pre-development scenario assumed that no water extraction, dams or other infrastructure were present in the catchment (Department of Environment and Science 2018, 2019). The high-regulation reach was the furthest downstream in the study reach, spanning from St George downstream to the Brenda gauge station (422015) (190-km river length). Flow alteration in this reach was characterised by a major decrease in mean daily flow (−57%), major increase in daily flow variability (+72%) and major decreases in the magnitude, frequency and duration of high-flow (10th percentile flow) events (−78, −60 and −31% respectively) (Table S1). The rate of rise and fall in flow events also decreased markedly (>36%). The moderate-regulation reach was situated furthest upstream in the study reach, from Loudouns Weir in Dalby to Chinchilla Weir (153 km), and was characterised by smaller changes in average and high flows compared with the high-regulation reach, and increases in frequency and duration of low-flow periods were also more pronounced. The low-regulation reach was situated in the central part of the study reach, between Chinchilla Weir and St George (387 km), and generally exhibited the smallest differences in flow metrics between pre- and full-development scenarios (Table S1).
Water level (m) and discharge (ML h−1) data were obtained from the existing gauging station network and attributed to acoustic-detection data based on detection location (Qld gauges: GS 422333A, GS 422336A, GS 422308C, GS 422344A, GS 422325A, GS 422220A, GS 422213A, GS 422201F) (Department of Regional Development, Manufacturing and Water 2023). The assumption was that the gauges represented the water level and discharge that tagged fish experienced, even though they may not have been in close proximity to a gauge. Mean air temperature from Chinchilla was used as a surrogate because of discontinuous water-temperature data being available for the study period (The Bureau of Meteorology, see http://www.bom.gov.au/climate/data/index.shtml). The most upstream gauge in each regulation reach was used to determine the number of flows received during the study period.
Fish collection, tagging and telemetry
Fish were captured for acoustic tagging by using electrofishing. Electrofishing was conducted in deep permanent and semi-permanent riverine waterholes and weir pools by using a boat-mounted unit (2.5 GPP; Smith-Root, Inc., Vancouver, WA, USA). Electrofisher settings were adjusted per site depending on water physiochemistry, to ensure minimal negative effects on the aquatic biota.
Between 2018 and 2023 we tagged and tracked 263 individuals of golden perch and 40 of Murray cod, representing subadult and adult fish, along the Condamine–Balonne River (Table 1). Fish were collected, tagged and released at 14 different locations from 2018 to 2021. The surgical methods for transmitter implantation were adapted from Butler et al. (2009). After capture, fish were transferred to a 200 L, river water-filled, aerated holding tank. Fish were weighed (±1 g) (SKI0KWP Class II, A&D Weighing, Sydney, NSW, Australia), measured (standard and total length ±1 mm) and then placed ventral side up in a V-shaped surgical cradle. Fish sex was not investigated so as to reduce total surgery time. Fish were sedated by electronarcosis (Balazik et al. 2013) applied by a portable DC power source providing a constant direct current (0.1 A) and low voltage (25–30 V), within the operating bath. During surgery, fresh aerated river water was constantly irrigated over the fish gills by a circulatory pump system. Once sedation (narcosis) was observed, noted by lowering of gill respiration and lack of fish movement, transmitters were inserted into the peritoneal cavity through a 15–20-mm incision positioned on the ventral midline between the pelvic fins and the anus. Non-absorbable sutures were used to close the incision, then each fish was injected with a dose of 75 mg kg−1 of antibiotic (oxytetracycline). All fish were then allowed to recover in 200-L tubs filled with oxygen enriched river water until normal swimming behaviour was observed, before release at the capture location.
Species | Total number of fish tagged | Mean total length (mm) (range) | Mean weight (g) (range) | Transmitter model (number used) | |||
---|---|---|---|---|---|---|---|
High | Mod | Low | |||||
Murray cod | 13 | 6 | 21 | 692 (262–995) | 6396 (199–14,840) | V9 (4), V13 (36) | |
Golden perch | 72 | 44 | 147 | 367 (220–538) | 753 (138–2068) | V9 (73), V13 (190) |
Innovasea V13–1X and V9–2X transmitters (Innovasea Amirix Systems Inc., Bedford, NS, Canada) were used and set to a roving ping rate of 80–140 s−1 (nominal 120 s−1) on a low-frequency pulse. The transmitters had an estimated life of 806–1460 days. We used an array of 62 acoustic receivers installed along ~700 km of the Condamine–Balonne River (Fig. 1). Receiver stations were deployed at regular intervals along the river where possible (average 12 km) in deep riverine and weir pools. Range testing confirmed a receiver detection radius greater than the maximum river width. Receiver stations were deployed instream, tethered to a bank-side tree, concrete block and float by using 4-mm stainless steel cable. There was no overlap of detection range for any receiver. Receiver stations were downloaded, and batteries replaced annually.
This research was conducted under animal ethics permit numbers SA2023-06-889 and SA2020-06-746 and general Fisheries permit number 258650.
Data analysis
The distance of each acoustic receiver location from the NSW–Qld border was calculated by digitising satellite images of the study reach into a spatial object in QGIS (ver. 3.20, see www.qgis.org). A distance matrix was then generated using the spatial object in the V-Track package (ver. 2.2, see https://github.com/RossDwyer/VTrack; Campbell et al. 2012) in R (ver. 4.2.2, R Foundation for Statistical Computing, Vienna, Austria, see www.r-project.org) and the distance between individual fish detections was calculated. A daily sum of total distance moved was then calculated for each individual fish. Detections occurring within the first 24 h for each fish were removed from the data analysis to discount any abnormal behaviour resulting from the tagging process (Carpenter-Bundhoo et al. 2020). Total linear range was calculated as the river distance between the furthest upstream and furthest downstream detections for each fish for the entire study period, and cumulative distance moved was calculated as the sum of all upstream and downstream movements for each fish for the entire study period.
This daily movement database was then aligned with daily total river discharge and sum of discharge for the previous 30 days, generated from the flow gauge closest to each fish’s current position in the system. Mean daily air temperature, and fish size (total length and weight) were also attributed to the daily-movement database.
Generalised additive mixed models (GAMMs) fitted with binomial distributions and a logit link were used to analyse probability of each Murray cod and golden perch movement. All models were built using the mgcv package (ver. 1.9-0, see https://cran.r-project.org/package=mgcv/; Wood 2003) in R. The binary response was calculated as a detection by an individual on a minimum of two receivers within a given 24-h period for movement (1) or, otherwise, no movement (0). Daily river discharge, antecedent flow (sum of flows in previous 30 days), river regulation level, month of year, daily temperature, and fish length and weight were included as candidate predictor variables. The moderate-regulation reach was omitted for Murray cod models because the number of detections was too low. As discharge and antecedent flow were highly skewed, they were transformed with a natural logarithm. A random intercept was included for each transmitter (ID) in each model because the data comprised repeated observations of each tagged fish. Year of study (Year) was included as another random intercept to account for yearly variation in hydrological and other environmental parameters not captured by the fixed effects.
Continuous predictors were fitted with cubic splines, month was fitted with a cyclic cubic spline, and river regulation was included as a parametric term. An autocorrelation structure (AR1) was incorporated in each GAMM to account for temporal autocorrelation. All possible models and interactions were examined, and the best model was selected using Chi-Square tests of restricted maximum likelihood (fREML) scores. Following the protocol of Zuur et al. (2010), data were checked for outliers, and collinearity among predictor variables was assessed using variance inflation factors (VIF).
Results
Hydrology
The 5-year study period encompassed a sequence of dry years (2018–2020) followed by a distinctly wetter period from late 2021 to early 2023 (Fig. 2). This was reflected in river flows, with high-flow events (exceeding the 10th percentile flow) being relatively infrequent between 2018 and 2020 (averaging 1.3 events per year). However, from late 2021 to 2022, between 13 and 17 distinct high-flow events occurred across the study reaches (averaging 6.5–8.5 events per year; Fig. 2). Peak flow varied across gauging stations, with the highest flows occurring in the furthest downstream (high-regulation) reach. The moderate-regulation reach received a higher number of high-flow events over the study period (total of 24 events) compared with the low- and high-regulation reaches (17 and 16 events respectively) (Fig. 2).
Plot of daily discharge (ML day−1) from three gauging stations that represent the (a) high regulation, St George gauge (422201F), (b) moderate regulation, Loudouns Bridge gauge (422333A), and (c) low regulation, Bedarra gauge (422344A), river sections in the Condamine–Balonne catchment. Also shown are the high-flow (10th percentile) threshold values for each gauge (indicated with horizontal dashed lines).
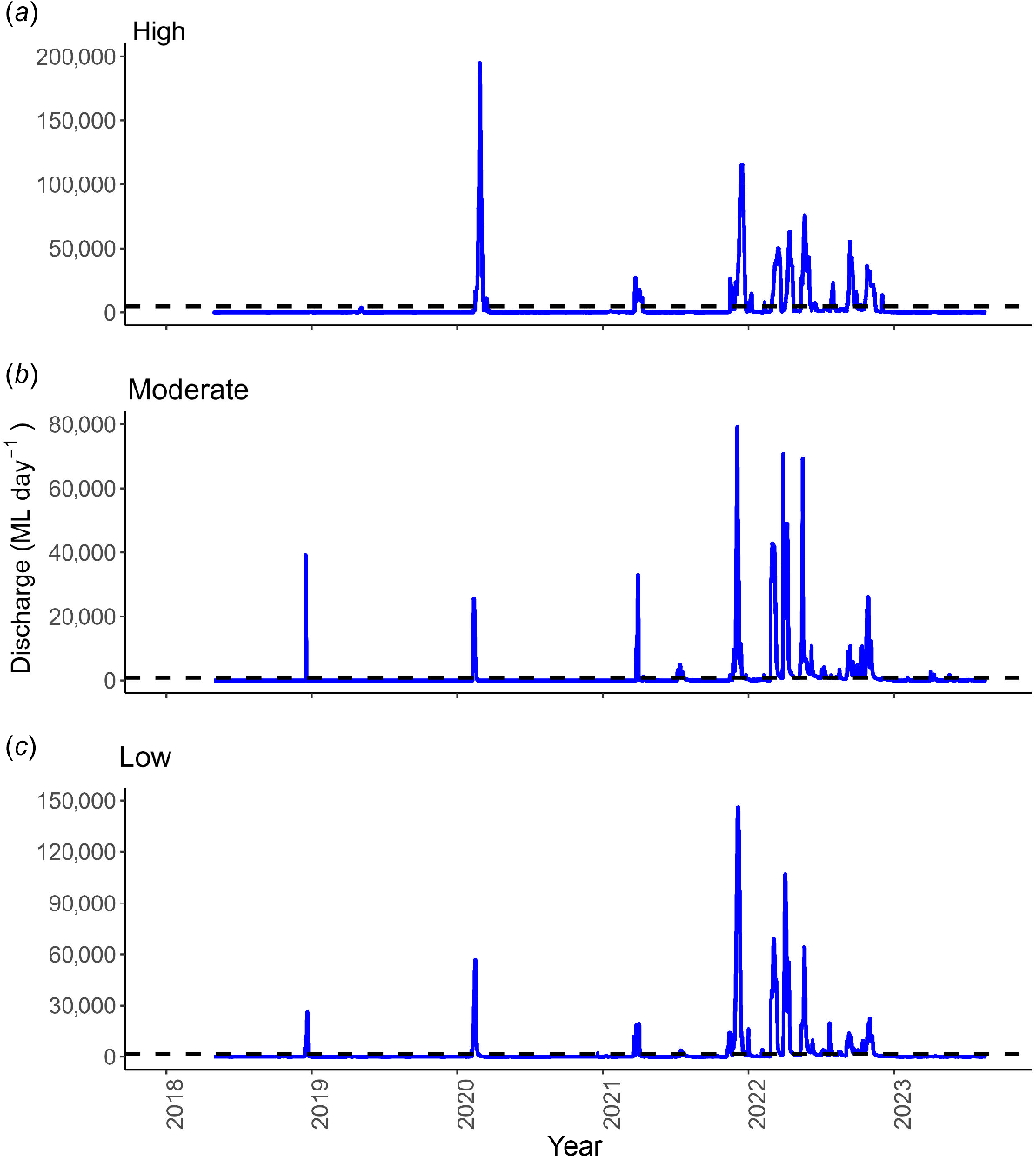
Movement summary
In all, 246 of 263 (93%) tagged golden perch and 31 of 40 (78%) tagged Murray cod were detected within the array during the study period. The percentage of golden perch that were mobile was relatively low (37%), with the majority of fish remaining in the tagging waterhole (63%) (Table 2). A greater percentage of Murray cod (58%) moved from the tagging waterhole. For both golden perch (62%) and Murray cod (78%), upstream movements were more likely. Philopatry (returning to the same waterhole) was uncommon for golden perch, with only 36% of migrating fish returning, whereas Murray cod showed a greater (78%) homing tendency.
Item | Murray cod | Golden perch | |||||||
---|---|---|---|---|---|---|---|---|---|
Flow regulation | Total | Flow regulation | Total | ||||||
High | Moderate | Low | High | Moderate | Low | ||||
Number of tagged fish | |||||||||
Supplied data | 12 | 3 | 16 | 31 | 60 | 43 | 143 | 246 | |
No data | 9 | 17 | |||||||
Movement types per tagged fish | |||||||||
Moved | 8 | 2 | 8 | 58% | 23 | 19 | 49 | 37% | |
Sedentary | 4 | 1 | 8 | 42% | 37 | 24 | 94 | 63% | |
Initial move US | 6 | 2 | 6 | 78% | 5 | 18 | 33 | 62% | |
Initial move DS | 2 | 0 | 2 | 22% | 18 | 1 | 16 | 38% | |
Philopatric (on 1 flow) | 6 | 0 | 4 | 56% | 8 | 5 | 11 | 26% | |
Philopatric (study duration) | 0 | 1 | 3 | 22% | 2 | 2 | 5 | 10% | |
Movement distances (km) | |||||||||
Maximum cumulative distance moved | 804 | 121 | 744 | 163 | 260 | 696 | |||
Average cumulative movement ± s.e. | 204 ± 69 | 87 ± 23 | 145 ± 57 | 29 ± 7 | 32 ± 9 | 53 ± 11 | |||
Maximum distance moved on 1 flow | 54 | 15 | 107 | 54 | 242 | 260 | |||
Average maximum distance moved on 1 flow ± s.e. | 25 ± 5 | 10 ± 5 | 24 ± 9 | 13 ± 2 | 18 ± 7 | 25 ± 4 | |||
Maximum linear range | 86 | 15 | 281 | 59 | 217 | 425 |
Note: some golden perch individuals moved between the regulation reaches; therefore, these movements were related to the regulation reach associated with the tagging location.
Longitudinal movements
For Murray cod, the maximum cumulative distance moved was 804 km (ID 62216) in the high-regulation reach. Maximum distance moved on one flow was 107 km (ID 62198) in the low-regulation reach, and maximum linear range (detected on the furthest-upstream and furthest-downstream receiver in the array) was 281 km (ID 24963) in the low-regulation reach (Fig. 3, Table 2). Across the entire study reach, cumulative movements of >10 km were observed for 86% of Murray cod individuals, and cumulative movements of >100 km were observed for 46% of Murray cod individuals. For any reach, on a single flow, 64% of Murray cod individuals moved >10 km and 11% moved >50 km. No Murray cod moved between the regulation reaches, across weirs (without fishways), in either a downstream or upstream direction.
Representative examples of movement patterns for Murray cod (Maccullochella peelii) and golden perch (Macquaria ambigua) within the (a) high-, (b) moderate- and (c) low-regulation reaches. Solid circles show detections on acoustic receiver stations. Dashed lines represent weirs.
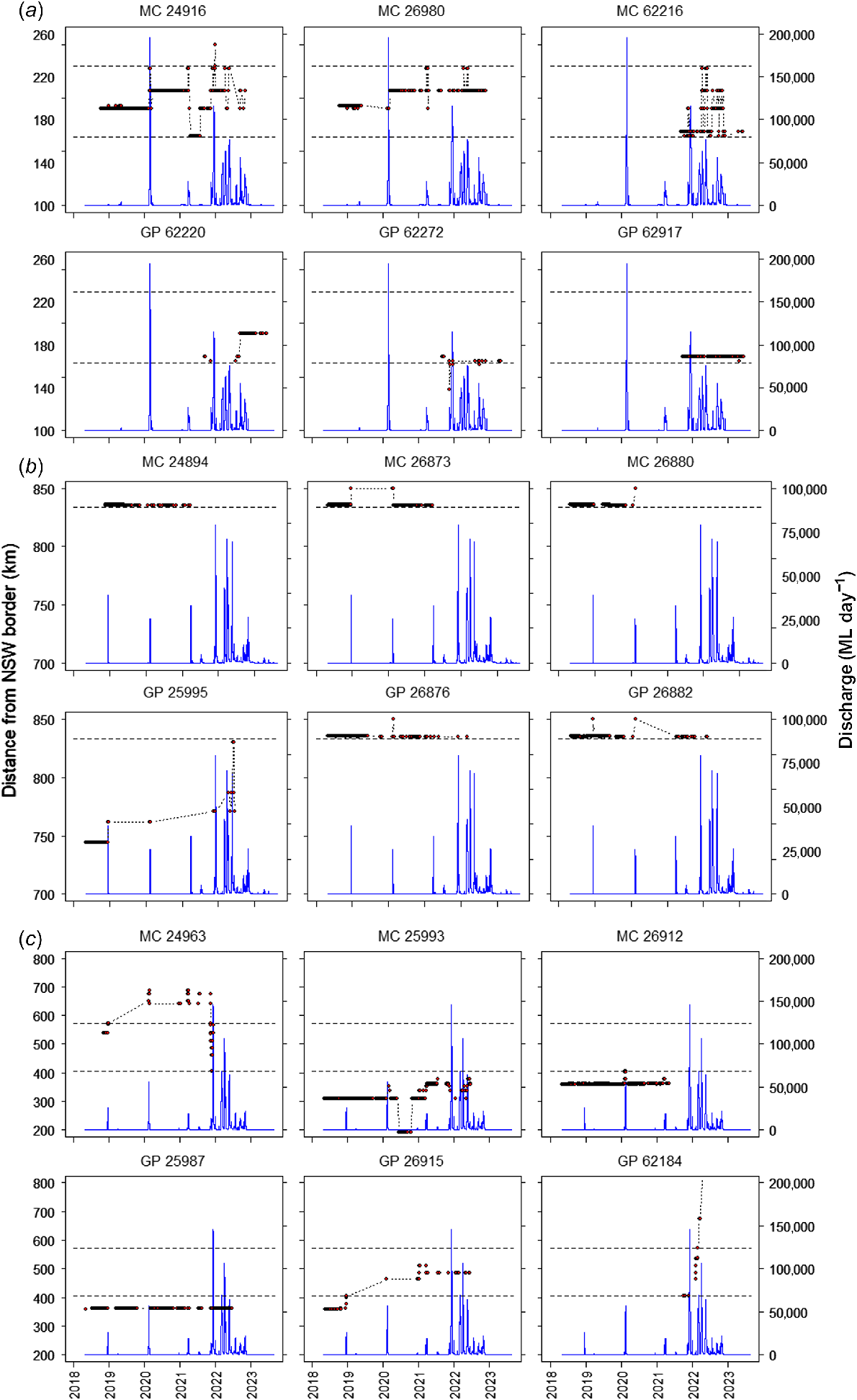
For golden perch, the maximum cumulative distance moved was 696 km, maximum distance moved on one flow was 260 km, and maximum linear range was 425 km, which all occurred in the low-regulation reach (ID 62184) (Fig. 3, Table 2). For all reaches, cumulative movements of >10 km were observed for 52% of golden perch individuals and >100 km for 13% of golden perch individuals. For all reaches, on a single flow, 45% of golden perch individuals made >10-km movements and 12% made >50-km movements. Two golden perch individuals moved upstream, and one moved downstream across weirs (without fishways) between the low- and moderate-regulation reaches during high flows.
Movement responses to flow
The overall probability of Murray cod movement was not significantly different among levels of regulation (estimated coefficient value, Est. ± s.e. = −0.467 ± 0.478, P = 0.326) (Table 3); however, probability of movement as function of daily discharge and antecedent discharge varied significantly among levels of regulation (Fig. 4a, b, Table 3). Murray cod were significantly (P < 0.001) more likely to move as discharge increased in both high- and low-regulation reaches, but probability of movement increased slightly more rapidly in the low-regulation reach (Fig. 4b). The GAMM predictions indicated that fish movements were triggered on moderately sized flow events, irrespective of location. For example, probability of fish movement of >50% occurred at discharge magnitudes of 1186 and 736 ML day−1 for the high- and low-regulation reaches respectively (Fig. 4a, b), both being equivalent to 15th percentile flows. Probability of movement did not increase appreciably after 6th percentile flows were reached (17,342 ML day−1 and 5360 ML day−1 for high- and low-regulation reaches respectively; Fig 4a, b). Murray cod was also more likely to move following higher antecedent discharge in both high and low regulation (Fig. 4c, d, Table 3). Weight of Murray cod significantly affected the probability of movement, with the probability of movement being highest for medium-sized fish (mean 6396 g), and smaller (presumed subadults) and larger fish moving less (P = 0.006; Fig. 4e).
Response | Adjusted R2 | Type | Basis | Variable | Estimate | s.e. | z-stat | d.f.e | d.f.ref | χ2 | P-value | |
---|---|---|---|---|---|---|---|---|---|---|---|---|
Murray cod | ||||||||||||
Movement (1/0) | 0.272 | Fixed | Low regulation | −0.47 | 0.478 | −0.983 | 0.326 | |||||
Spline | Cubic regression | log(discharge): high | 1.884 | 2.345 | 70.19 | <0.001 | ||||||
Cubic regression | log(discharge): low | 2.641 | 3.312 | 41.22 | <0.001 | |||||||
Cubic regression | log(antecedent): high | 2.226 | 2.72 | 11.13 | 0.009 | |||||||
Cubic regression | log(antecedent): low | 1 | 1 | 9.95 | 0.002 | |||||||
Cubic regression | Weight | 2.796 | 3.079 | 13.39 | 0.006 | |||||||
Random effect | Fish ID | 14.034 | 28 | 82.35 | <0.001 | |||||||
Random effect | Year | 3.741 | 5 | 32.47 | <0.001 | |||||||
Golden perch | ||||||||||||
Movement (1/0) | 0.254 | Fixed | Low regulation | −0.927 | 0.3441 | −2.695 | 0.007 | |||||
Moderate regulation | −0.237 | 0.4896 | −0.485 | 0.628 | ||||||||
Spline | Cubic regression | log(discharge): low | 3.64 | 4.51 | 190.432 | <0.001 | ||||||
Cubic regression | log(discharge): moderate | 4.186 | 5.125 | 83.279 | <0.001 | |||||||
Cubic regression | log(discharge): high | 1 | 1 | 84.942 | <0.001 | |||||||
Cyclic cubic regression | Month | 5.769 | 8 | 71.995 | <0.001 | |||||||
Cubic regression | Weight | 1 | 1 | 4.953 | 0.026 | |||||||
Random effect | Fish ID | 105.753 | 238 | 343.263 | <0.001 | |||||||
Random effect | Year | 2.716 | 5 | 18.736 | 0.007 |
Spline statistics are presented with estimated degrees of freedom (d.f.e), reference degrees of freedom (d.f.ref) and P-values. Significant values are indicated in bold.
Predicted probabilities (±95% CI) of Murray cod (Maccullochella peelii) movement as a function of daily discharge in (a) high- and (b) low-regulation, antecedent discharge in (c) high and (d) low regulation, and (e) fish weight. Discharge and antecedent discharge were exponentiated from log-transformed values used in the model.
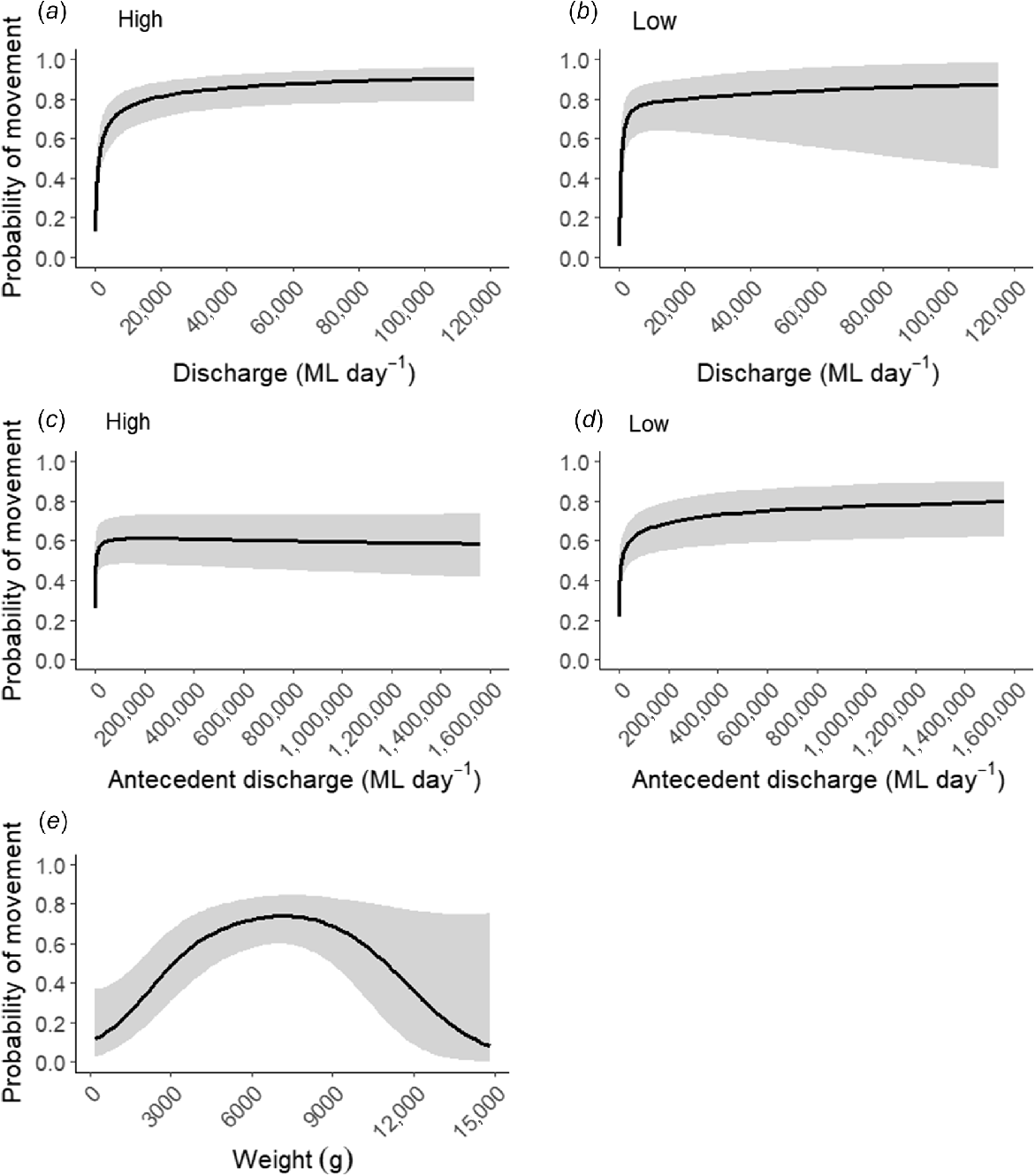
Probability of golden perch movement varied significantly among levels of regulation, with fish in low regulation having a significantly lower probability of movement than those in high regulation (Est. ± s.e. = −0.927 ± 0.344, P = 0.007) (Table 3). The moderate- and high-regulation reaches were not significantly different overall (Est. ± s.e. = −0.237 ± 0.490, P = 0.628) (Table 3). Probability of golden perch movement as a function of daily discharge varied significantly (P < 0.001) among all levels of regulation. Similar to Murray cod, golden perch movements were triggered on moderately sized flow events, irrespective of location (Fig. 5a–c). For example, probability of fish movement of >50% occurred at discharge magnitudes of 2108, 332 and 738 ML day−1 for the high-, moderate- and low-regulation reaches respectively (equivalent to 13–15th percentile flows). The probability of movement increased progressively with an increasing discharge in the high-regulation reach (Fig. 5a). By contrast, probability of movement in the moderate- and low-regulation reaches peaked once flows reached ~16,642 ML day−1 (equivalent to a 2nd-percentile flow) and 14,305 ML day−1 (equivalent to a 3rd-percentile flow) respectively, then gradually decreased at higher flows (Fig. 5b, c). Golden perch was significantly more likely to move as it increased in weight (smaller fish were presumed to be subadult) (Fig. 5d). The highest probability of movement for golden perch was predicted for the January–February period, and the lowest for the June–August period (Fig. 5e).
Discussion
This study expands on the current knowledge of environmental cues and conditions that facilitate movement for two dryland fish species in a highly variable and regulated river system. We found that the movement responses of both species varied notably under differing river-regulation levels, as either a main effect or through interactions with discharge. Movement patterns for both species were associated with a range of separate and interacting environmental and biological factors, including discharge, river regulation, seasonality and body size. When considered with other studies, our findings demonstrated that the movements of golden perch differ in response to the variability of flow regimes across the MDB (Marshall et al. 2016; Zampatti et al. 2018; Koster et al. 2020), whereas flow-related movements in Murray cod showed less variability across its range (Koehn et al. 2020a). This specific movement information could inform a review of the current management arrangements for these key dryland fish species, with a view towards managing population dynamics, including recruitment and movement based on river regulation and flow regimes specific to the northern MDB.
General Murray cod movements
Our study corroborated contemporary findings that Murray cod is an active mover outside of the breeding season (Koehn et al. 2009), or when triggered by high flow (Thiem et al. 2018, 2020; Carpenter-Bundhoo et al. 2023). We found Murray cod to be mobile, with 58% of individuals moving from the tagging waterhole and some fish being active throughout the year. Heightened movement activity in response to higher river discharges might suggest an expanded opportunity to find more suitable habitats or nesting sites, facilitated by improved connectivity (Carpenter-Bundhoo et al. 2023). Most initial movements by Murray cod were upstream, a finding that is consistent with Thiem et al. (2020), who reported that the species behaved similarly during elevated flows in the Murray River. Murray cod in our study also had significant homing behaviour, with 78% of individuals returning to their tagging waterhole. Homing behaviour in Murray cod was common in the upper and lower Murray River (Koehn et al. 2009; Leigh and Zampatti 2013). However, Carpenter-Bundhoo et al. (2023), did not report large-scale philopatric behaviour for Murray cod in the Gwydir and Mehi rivers, although their study assessed movement responses to relatively small-magnitude environmental-flow releases.
Murray cod was observed moving long distances, with one individual achieving a maximum linear range of 281 km, and a second individual accumulating 804 km of total movements throughout the study period. Although there are examples of Murray cod making large-scale movements in the southern MDB (Koehn et al. 2009; Leigh and Zampatti 2013), movements are more typically in the order of 5–29 km, with few fish observed moving distances greater than 200 km (Koehn et al. 2009; Thiem et al. 2020). These findings indicated that spatial variability in Murray cod movement ecology is common and potentially linked to location-specific flow regimes, water quality and habitat availability (Koster et al. 2020; Butler et al. 2022; Carpenter-Bundhoo et al. 2023). To support sustainable Murray cod populations, future research programs could focus on the efficacy of environmental flows or protection of existing flow regimes that support Murray cod life-history processes, such as spawning, movement timing and spatial extent, which are specific to local flow and environmental conditions. This study highlighted that long-distance, upstream, philopatric movements occurred during elevated flows. Water managers should consider options to support these obligate, flow-dependent life-history processes when protecting and restoring flow regimes across the northern MDB. Moreover, although our study was focussed on adult movement patterns across a gradient of river regulation, we recognise the need to understand dispersal in all life-stages (e.g. eggs, larvae, fingerlings) when facilitating Murray cod movements and highlight this as an area for future research in the northern MDB.
General golden perch movements
We predicted a high percentage of golden perch (similar to Murray cod) moving in this study because of the large number of high-flow events recorded. We found that golden perch was typically sedentary, with only 37% of individuals initiating any movement from their tagging location. Previous studies in the MDB have found that flooding flows promote large-scale upstream migrations by large proportions of tagged individuals (Koster et al. 2017; Zampatti et al. 2018), whereas other golden perch studies in the MDB have reported periods of sedentary behaviour interspersed with movement during high flows (Marshall et al. 2016; Koster et al. 2020). We also found lower rates of homing behaviour in golden perch (36% philopatry) than did Marshall et al. (2016; 64.7% in the Moonie River) and O’Connor et al. (2005; >50% in the Murray River). Some golden perch individuals undertook long-distance longitudinal movements; however, unlike Murray cod, this pattern was less apparent in the highly regulated reaches. Golden perch displayed a variety of movement patterns within the Condamine–Balonne River, such as residency, local movements (>10 km) and large-distance movements (>100 km). These findings are generally consistent with other studies throughout the MDB, with varying levels of water-resource development and contrasting flow regimes being the primary determinates (Marshall et al. 2016; Zampatti et al. 2018; Thiem et al. 2020).
Making large-scale unidirectional or philopatric movements can expose fish populations to increased mortality risk in dryland rivers (Jaeger et al. 2014; Marshall et al. 2021). Changes to flow-recession rates and increases in the frequency and duration of low-flow events owing to water-resource development can strand individuals in less favourable locations (Bond et al. 2015). Alternatively, individuals in areas with lower-quality food resources, limited breeding habitat and higher competition may benefit from movement to new river reaches (Crook 2004). In the Condamine–Balonne River, return movements to home-range waterholes may not be obligate because of the presence of other refugial waterholes throughout the system (Fawcet and Prior 2018).
Movement patterns in response to flow and river regulation
We did not find a difference in the probability of Murray cod moving among the levels of river regulation alone. Instead, daily discharge magnitude was found to be the primary driver of Murray cod movement. The flows recorded in the high-regulation reach appear to have facilitated or cued Murray cod movements over our study period. Multiple studies that observed similar movements in the MDB have proposed that greater opportunities for emigration, exploration and ability to find suitable mating and nesting sites are associated with larger flow events (Leigh and Zampatti 2013; Thiem et al. 2018). Further, movement before and after the spawning season (austral spring) has been attributed to increased reproductive-related activity in the MDB (Koehn et al. 2009; Carpenter-Bundhoo et al. 2020; Koster et al. 2020). We did not find time of year to be associated with any increase in movement probability for Murray cod; however, a high number of flow events was recorded over the study period. This would potentially provide many opportunities for movement in lieu of fewer critically timed flow events, a conclusion that is supported by our analysis, which showed that movement probability increased with antecedent flow. In the Condamine–Balonne River, ‘wet’ years that enable fish to increase body condition and increase investment in reproduction may be a more important trigger of Murray cod spawning migrations than is flow timing or river regulation (Balcombe et al. 2012; Koster et al. 2017; Tonkin et al. 2017).
It was found that movements by medium-sized Murray cod occurred more often than did those by their smaller or larger conspecifics. Reduced movement of both smaller and larger fish is possibly due to small fish being sexually immature and therefore not seeking spawning territories (Jones and Stuart 2007), and larger fish occupying successfully defined existing home ranges (Butler et al. 2022). Reproductive maturity for Murray cod in the Border Rivers (northern MDB) is ~440 mm and 4 years old (G. L. Butler, pers. comm.), suggesting that increased movement of medium-sized fish could be attributed to seeking unoccupied, high-quality reproductive habitat and other resources under less competitive pressure (Koehn et al. 2009).
Flow magnitude was also found to be an important predictor of golden perch movement; however, unlike Murray cod, the level of river regulation was also found to affect the probability of movement. This suggests that other aspects of the flow regime, such as periodicity, may also be important drivers for movement. Altered flow regimes have previously been highlighted as a threat to golden perch, with a reduction in small and medium in-channel flow pulses, spring–summer flood flows and a reduction in flow peaks all affecting this species (Stuart and Sharpe 2020). In the low- and moderate- regulation reaches of the Condamine–Balonne River, the probability of golden perch movement decreased once flows exceeded second or third percentile values, 16,642 ML day−1 and 14,305 ML day−1 respectively. This result was similar to that found on the Goulburn River in the southern MDB, where a reduction in movement at higher flows was observed (Koster et al. 2017). Additionally, recruitment of golden perch has previously been found to be poor on high flows and stronger on spring regulated within-channel flows in lowland river systems (Mallen-Cooper and Stuart 2003). Smaller within-channel flows that cue migrations related to spawning may therefore provide sufficient movement opportunities within the northern MDB. This would illustrate that golden perch has flexible spawning and movement strategies adapted for unpredictable local hydrology and environmental conditions synonymous with dryland river systems (Thiem et al. 2023).
Juvenile and subadult golden perch individuals are known to undertake upstream migrations in response to flow, with large numbers of juveniles and subadults (1+ and 2+) having been observed aggregating below weirs (Mallen-Cooper and Stuart 2003). Although we tagged small-sized golden perch in this study (representative of subadult, 2+ fish), our analysis showed that larger golden perch individuals were much more likely to migrate than were smaller golden perch individuals. Adult golden perch are known to be mobile, with migrations undertaken on elevated discharges linked to spawning (Stuart et al. 2008; Koster et al. 2017; Thiem et al. 2020). These larger specimens have been observed with higher body fat content, which could be more readily converted to reproductive energy prior to spawning and therefore facilitating a high probability of migrations in adult size classes (Sternberg et al. 2008).
Similar to other studies (Koehn and Nicol 2016; Marshall et al. 2016; Koster et al. 2017), the probability of golden perch movements increased across the spring, late summer and autumn months and was restricted during the winter months. However, we found a much higher probability of movement within the autumn months (peak probability in May of ~60%) than in southern MDB populations. In the northern MDB, water temperatures are warmer for longer compared to the southern MDB and during dry years, higher river discharge in the Condamine–Balonne River often occurs in late summer and autumn (Department of Regional Development, Manufacturing and Water 2023). These factors potentially combine to extend the golden perch spawning window through to the end of autumn in the northern MDB, as shown by the increased probability of golden perch movement during these months.
Synthesis and future directions
Our study suggests that widely distributed species such as Murray cod and golden perch respond to local environmental and hydrological cues across a range of river regulation and antecedent conditions. Addressing priority knowledge gaps linked to these species key life-history characteristics and their interaction with the flow regime, critical habitats, environmental-flow applicability, impact from artificial barriers and movement connectivity are a priority for water-resource managers (Koehn et al. 2020b). Given that philopatry of golden perch appears to be limited in Condamine–Balonne River populations, further research could assess the configuration of waterholes (e.g. location and size) across the landscape, their drought-refuge quality and the frequency of connection events that relate to fish movement and their specific life-history requirements. In the highly regulated section of the river (downstream of St George), there is provision for an environmental, stock and domestic (ESD) low flow. This flow-management strategy is likely to benefit golden perch and Murray cod to some extent where spatial variability in movement ecology is indicative of generalist species inhabiting highly variable dryland river ecosystems. Augmenting flows via environmental releases is a common strategy to help maintain species critical life-history requirements and mitigate impacts associated with river regulation (King et al. 2009). Therefore, further research of the ESD flow will provide a greater understanding of the environmental benefits to help inform future management. Our study observed no fish migrating between the high- and low-regulation river sections and very few fish (golden perch only) moved between the low- and moderate-regulation sections across large barriers. Because flow alone is unlikely to be the only determinant of Murray cod and golden perch longitudinal connectivity, an analysis of the impact of barrier configuration and connectivity opportunities in the Condamine–Balonne River, would highlight instream barriers for fish-passage remediation. Although it is acknowledged the natural flow regime has been affected by water-resource development, protecting flow pulses throughout the system that connects tributaries across the northern MDB, will help support life-history requirements in these key species. To maintain the long-term viability of Murray cod and golden perch populations within dryland rivers of the northern MDB, the spatial and temporal variability in behaviour and movement requires management approaches at river scale and level of river regulation tailored to the life-histories of these migratory fish.
Data availability
All data associated with this study will be made available upon request to the corresponding author.
Declaration of funding
The authors received funding from the Queensland Department of Regional Development, Manufacturing and Water for the Environmental Flows Assessment Program, which gave rise to this paper.
Acknowledgements
The authors thank Dr Karen Caldwell and Dr Jackie Lee for conducting fish surgery and staff from Department of Regional Development, Manufacturing and Water and Department of Environment, Science and Innovation who provided assistance in the field, repairs to equipment, flow data and mapping, in particular, Jess MacKenzie, Col Farrawell, Lucas de Castro, Ryan Woods, Cameron Schulz, Matthew Tuffield, Josh Heberlein, Leanne Hughes, Craig Johanson, Ken Craw, Caitlin Gersekowski, Susan Harch, Peter Brownhalls and Suzi Johnson. We also thank the property owners for their continued support of this project.
References
Balazik MT, Langford BC, Garman GC, Fine ML, Stewart JK, Latour RJ, McIninch SP (2013) Comparison of MS-222 and electronarcosis as anesthetics on cortisol levels in juvenile Atlantic Sturgeon. Transactions of the American Fisheries Society 142(6), 1640-1643.
| Crossref | Google Scholar |
Balcombe SR, Lobegeiger JS, Marshall SM, Marshall JC, Ly D, Jones DN (2012) Fish body condition and recruitment success reflect antecedent flows in an Australian dryland river. Fisheries Science 78, 841-847.
| Crossref | Google Scholar |
Bond NR, Balcombe SR, Crook DA, Marshall JC, Menke N, Lobegeiger JS (2015) Fish population persistence in hydrologically variable landscapes. Ecological Applications 25(4), 901-913.
| Crossref | Google Scholar | PubMed |
Burkhead NM (2012) Extinction rates in North American freshwater fishes, 1900–2010. BioScience 62(9), 798-808.
| Crossref | Google Scholar |
Butler GL, Mackay B, Rowland SJ, Pease BC (2009) Retention of intra-peritoneal transmitters and post-operative recovery of four Australian native fish species. Marine and Freshwater Research 60(4), 361-370.
| Crossref | Google Scholar |
Butler GL, Davis TR, Brooks SG, Bowen C, Cameron LM, Rowland SJ, Smith D, St Vincent Welch J, Carpenter-Bundhoo L (2022) Combining bio-telemetry and underwater imagery to elucidate the reproductive behaviour of a large, long-lived Australian freshwater teleost. Journal of Environmental Management 317, 115298.
| Crossref | Google Scholar | PubMed |
Campbell HA, Watts ME, Dwyer RG, Franklin CE (2012) V-Track: software for analysing and visualising animal movement from acoustic telemetry detections. Marine and Freshwater Research 63, 815-820.
| Crossref | Google Scholar |
Carpenter-Bundhoo L, Butler GL, Espinoza T, Bond NR, Bunn SE, Kennard MJ (2020) Reservoir to river: quantifying fine-scale fish movements after translocation. Ecology of Freshwater Fish 29, 89-102.
| Crossref | Google Scholar |
Carpenter-Bundhoo L, Butler GL, Bond NR, Thiem JD, Bunn SE, Kennard MJ (2023) Fish movements in response to environmental flow releases in intermittent rivers. Freshwater Biology 68(2), 260-273.
| Crossref | Google Scholar |
Crook DA (2004) Is the home range concept compatible with the movements of two species of lowland river fish? Journal of Animal Ecology 73(2), 353-366.
| Crossref | Google Scholar |
Department of Agriculture, Water and the Environment (2023) Surface water flow. (Bioregional Assessments, Australian Government) Available at www.bioregionalassessments.gov.au/assessments/11-context-statement-maranoa-balonne-condamine-subregion/1153-surface-water-flow
Department of Regional Development, Manufacturing and Water (2023) Water Monitoring Information Portal. (Queensland Government) Available at https://water-monitoring.information.qld.gov.au/
Dias MS, Tedesco PA, Hugueny B, Jézéquel C, Beauchard O, Brosse S, Oberdorff T (2017) Anthropogenic stressors and riverine fish extinctions. Ecological Indicators 79, 37-46.
| Crossref | Google Scholar |
Espinoza T, Burke CL, Carpenter-Bundhoo L, Marshall SM, McDougall AJ, Roberts DT, Campbell HA, Kennard MJ (2021) Quantifying movement of multiple threatened species to inform adaptive management of environmental flows. Journal of Environmental Management 295, 113067.
| Crossref | Google Scholar | PubMed |
Grill G, Lehner B, Thieme M, Geenen B, Tickner D, Antonelli F, Babu S, Borrelli P, Cheng L, Crochetiere H, Ehalt Macedo H, Filgueiras R, Goichot M, Higgins J, Hogan Z, Lip B, McClain ME, Meng J, Mulligan M, Nilsson C, Olden JD, Opperman JJ, Petry P, Reidy Liermann C, Sáenz L, Salinas-Rodríguez S, Schelle P, Schmitt RJP, Snider J, Tan F, Tockner K, Valdujo PH, van Soesbergen A, Zarfl C (2019) Mapping the world’s free-flowing rivers. Nature 569(7755), 215-221.
| Crossref | Google Scholar | PubMed |
Harding DJ, Dwyer RG, Mullins TM, Kennard MJ, Pillans RD, Roberts DT (2017) Migration patterns and estuarine aggregations of a catadromous fish, Australian bass (Percalates novemaculeata) in a regulated river system. Marine and Freshwater Research 68(8), 1544-1553.
| Crossref | Google Scholar |
Harding DJ, Roberts DT, Sternberg D, Mullins TM, Kennard MJ, Dwyer RG (2019) Flow-related migration, juvenile dispersal and gonad development in two co-occurring mullet species, Mugil cephalus and Trachystoma petardi, in a regulated river system. Marine and Freshwater Research 70(8), 1105-1115.
| Crossref | Google Scholar |
Jaeger KL, Olden JD, Pelland NA (2014) Climate change poised to threaten hydrologic connectivity and endemic fishes in dryland streams. Proceedings of the National Academy of Sciences 111(38), 13894-13899.
| Crossref | Google Scholar |
Jones MJ, Stuart IG (2007) Movements and habitat use of common carp (Cyprinus carpio) and Murray cod (Maccullochella peelii peelii) juveniles in a large lowland Australian river. Ecology of Freshwater Fish 16(2), 210-220.
| Crossref | Google Scholar |
King AJ, Tonkin Z, Mahoney J (2009) Environmental flow enhances native fish spawning and recruitment in the Murray River, Australia. River Research and Applications 25(10), 1205-1218.
| Crossref | Google Scholar |
Koehn JD, Nicol SJ (2016) Comparative movements of four large fish species in a lowland river. Journal of Fish Biology 88(4), 1350-1368.
| Crossref | Google Scholar | PubMed |
Koehn JD, McKenzie JA, O’Mahony DJ, Nicol SJ, O’Connor JP, O’Connor WG (2009) Movements of Murray cod (Maccullochella peelii peelii) in a large Australian lowland river. Ecology of Freshwater Fish 18(4), 594-602.
| Crossref | Google Scholar |
Koehn JD, King AJ, Beesley L, Copeland C, Zampatti BP, Mallen-Cooper M (2014) Flows for native fish in the Murray–Darling Basin: lessons and considerations for future management. Ecological Management & Restoration 15, 40-50.
| Crossref | Google Scholar |
Koehn JD, Balcombe SR, Zampatti BP (2019) Fish and flow management in the Murray–Darling Basin: directions for research. Ecological Management & Restoration 20(2), 142-150.
| Crossref | Google Scholar |
Koehn JD, Raymond SM, Stuart I, Todd CR, Balcombe SR, Zampatti BP, Bamford H, Ingram BA, Bice CM, Burndred K, Butler G, Baumgartner L, Clunie P, Ellis I, Forbes JP, Hutchison M, Koster WM, Lintermans M, Lyon JP, Mallen-Cooper M, McLellan M, Pearce L, Ryall J, Sharpe C, Stoessel DJ, Thiem JD, Tonkin Z, Townsend A, Ye Q (2020a) A compendium of ecological knowledge for restoration of freshwater fishes in Australia’s Murray–Darling Basin. Marine and Freshwater Research 71(11), 1391-1463.
| Crossref | Google Scholar |
Koehn JD, Balcombe SR, Baumgartner LJ, Bice CM, Burndred K, Ellis I, Koster WM, Lintermans M, Pearce L, Sharpe C, Stuart I, Todd CR (2020b) What is needed to restore native fishes in Australia’s Murray–Darling Basin? Marine and Freshwater Research 71(11), 1464-1468.
| Crossref | Google Scholar |
Koster WM, Dawson DR, Liu C, Moloney PD, Crook DA, Thomson JR (2017) Influence of streamflow on spawning-related movements of golden perch Macquaria ambigua in south-eastern Australia. Journal of Fish Biology 90(1), 93-108.
| Crossref | Google Scholar | PubMed |
Koster WM, Dawson DR, Kitchingman A, Moloney PD, Hale R (2020) Habitat use, movement and activity of two large-bodied native riverine fishes in a regulated lowland weir pool. Journal of Fish Biology 96(3), 782-794.
| Crossref | Google Scholar | PubMed |
Larkin ZT, Ralph TJ, Tooth S, Fryirs KA, Carthey AJR (2020) Identifying threshold responses of Australian dryland rivers to future hydroclimatic change. Scientific Reports 10, 6653.
| Crossref | Google Scholar |
Leigh SJ, Zampatti BP (2013) Movement and mortality of Murray cod, Maccullochella peelii, during overbank flows in the lower River Murray, Australia. Australian Journal of Zoology 61(2), 160-169.
| Crossref | Google Scholar |
Mallen-Cooper M, Stuart IG (2003) Age, growth and non-flood recruitment of two potamodromous fishes in a large semi-arid/temperate river system. River Research and Applications 19(7), 697-719.
| Crossref | Google Scholar |
Marshall JC, Menke N, Crook DA, Lobegeiger JS, Balcombe SR, Huey JA, Fawcett JH, Bond NR, Starkey AH, Sternberg D, Linke S, Arthington AH (2016) Go with the flow: the movement behaviour of fish from isolated waterhole refugia during connecting flow events in an intermittent dryland river. Freshwater Biology 61(8), 1242-1258.
| Crossref | Google Scholar |
Marshall JC, Lobegeiger JS, Starkey A (2021) Risks to fish populations in dryland rivers from the combined threats of drought and instream barriers. Frontiers in Environmental Science 9, 671556.
| Crossref | Google Scholar |
Murray–Darling Basin Authority (2023) Condamine–Balonne catchment. (MDBA: Canberra, ACT, Australia) Available at www.mdba.gov.au/basin/catchments/northern-basin-catchments/condamine-balonne-catchment
O’Connor JP, O’Mahony DJ, O’Mahony JM (2005) Movements of Macquaria ambigua, in the Murray River, south-eastern Australia. Journal of Fish Biology 66(2), 392-403.
| Crossref | Google Scholar |
Pennock CA, Budy P, Macfarlane WW, Breen MJ, Jimenez J, Schmidt JC (2022) Native fish need a natural flow regime. Fisheries 47(3), 118-123.
| Crossref | Google Scholar |
Poff NLR, Allan JD, Bain MB, Karr JR, Prestegaard KL, Richter BD, Sparks RE, Stromberg JC (1997) The natural flow regime. BioScience 47(11), 769-784.
| Crossref | Google Scholar |
Reynolds LF (1983) Migration patterns of five fish species in the Murray–Darling River system. Marine and Freshwater Research 34(6), 857-871.
| Crossref | Google Scholar |
Sternberg D, Balcombe S, Marshall J, Lobegeiger J (2008) Food resource variability in an Australian dryland river: evidence from the diet of two generalist native fish species. Marine and Freshwater Research 59(2), 137-144.
| Crossref | Google Scholar |
Stuart IG, Sharpe CP (2020) Riverine spawning, long distance larval drift, and floodplain recruitment of a pelagophilic fish: a case study of golden perch (Macquaria ambigua) in the arid Darling River, Australia. Aquatic Conservation: Marine and Freshwater Ecosystems 30(4), 675-690.
| Crossref | Google Scholar |
Stuart IG, Zampatti BP, Baumgartner LJ (2008) Can a low-gradient vertical-slot fishway provide passage for a lowland river fish community? Marine and Freshwater Research 59(4), 332-346.
| Crossref | Google Scholar |
Thiem JD, Wooden IJ, Baumgartner LJ, Butler GL, Forbes J, Taylor MD, Watts RJ (2018) Abiotic drivers of activity in a large, free-ranging, freshwater teleost, Murray cod (Maccullochella peelii). PLoS ONE 13(6), e0198972.
| Crossref | Google Scholar | PubMed |
Thiem JD, Wooden IJ, Baumgartner LJ, Butler GL, Taylor MD, Watts RJ (2020) Hypoxic conditions interrupt flood-response movements of three lowland river fish species: implications for flow restoration in modified landscapes. Ecohydrology 13(3), e2197.
| Crossref | Google Scholar |
Thiem JD, Baumgartner LJ, Fanson B, Sadekov A, Tonkin Z, Zampatti BP (2022) Contrasting natal origin and movement history informs recovery pathways for three lowland river species following a mass fish kill. Marine and Freshwater Research 73(2), 237-246.
| Crossref | Google Scholar |
Thiem JD, Michie LE, Butler GL, Ebner BC, Sharpe CP, Stuart I, Townsend A (2023) A protected flow breaks the drought for golden perch (Macquaria ambigua) spawning along an extensive semi-arid river system. Ecohydrology 16(7), e2576.
| Crossref | Google Scholar |
Tickner D, Opperman JJ, Abell R, Acreman M, Arthington AH, Bunn SE, Cooke SJ, Dalton J, Darwall W, Edwards G, Harrison I, Hughes K, Jones T, Leclère D, Lynch AJ, Leonard P, McClain ME, Muruven D, Olden JD, Ormerod SJ, Robinson J, Tharme RE, Thieme M, Tockner K, Wright M, Young L (2020) Bending the curve of global freshwater biodiversity loss: an emergency recovery plan. BioScience 70(4), 330-342.
| Crossref | Google Scholar | PubMed |
Tonkin Z, Kitchingman A, Lyon J, Kearns J, Hackett G, O’Mahony J, Moloney PD, Krusic-Golub K, Bird T (2017) Flow magnitude and variability influence growth of two freshwater fish species in a large regulated floodplain river. Hydrobiologia 797, 289-301.
| Crossref | Google Scholar |
Vertessy R, Barma D, Baumgartner L, Mitrovic S, Sheldon F, Bond N (2019) Independent assessment of the 2018–19 fish deaths in the Lower Darling. Final report. (La Trobe University) Available at https://opal.latrobe.edu.au/articles/report/Final_report_of_the_Independent_Assessment_of_the_2018-19_fish_deaths_in_the_lower_Darling/16869591
Wood SN (2003) Thin plate regression splines. Journal of the Royal Statistical Society – B. Statistical Methodology 65, 95-114.
| Crossref | Google Scholar |
Zampatti BP, Leigh SJ, Bice CM, Rogers PJ (2018) Multiscale movements of golden perch (Percichthyidae: Macquaria ambigua) in the River Murray, Australia. Austral Ecology 43(7), 763-774.
| Crossref | Google Scholar |
Zuur AF, Ieno EN, Elphick CS (2010) A protocol for data exploration to avoid common statistical problems. Methods in Ecology and Evolution 1, 3-14.
| Crossref | Google Scholar |