Lobster predation on barren-forming sea urchins is more prevalent in habitats where small urchins are common: a multi-method diet analysis
Jennifer E. Smith



A
B
Abstract
In Tasmania, Australia, the government’s response to range-extending, barren-forming longspined sea urchin (Centrostephanus rodgersii) includes rebuilding of southern rock lobster (Jasus edwardsii) stocks to increase predation. But lobster preference for native species and continued barren expansion challenges the control efficacy.
To determine the impact of lobster predation on C. rodgersii in different habitats.
Multi-method dietary analysis consisting of stomach contents, faecal DNA and stable isotopes was performed on 64 lobsters from four habitats varying in barren extent and density of urchins and lobsters.
C. rodgersii contributed to lobster diet in all barren habitats and was found in lobsters of every size class. Stable isotope and DNA analyses showed that C. rodgersii was more common in lobster diet than were targeted native species at incipient barren sites. Surprisingly, in extensive barrens C. rodgersii is less prominent in lobster diet.
Combined with site-specific urchin population data, our findings indicated that lobster predation may be less effective at sites where most C. rodgersii individuals have reached a size refuge than at sites dominated by small urchins.
Lobster predation may provide a useful control for smaller C. rodgersii, but top–down predatory control may be constrained at sites dominated by urchins that exceed the size suitable for lobster predation.
Keywords: biodiversity, climate change, crustaceans, diet, echinoderms, ecology, fisheries, genetics, invertebrates, marine, stable isotopes.
Introduction
Top–down trophic cascades in which predators benefit producers by suppressing herbivores are highly important in marine systems (Pace 2013). Furthermore, evidence from studies on birds, fishes and amphibians has shown that predation or competition by native species can yield significant advantages in reducing the survival of pest species (Santos et al. 2009; Cabrera-Guzmán et al. 2011; Diller et al. 2014; Mori et al. 2020). In this way, predatory control of overabundant species can play an important role in regulating long-term dynamics of novel pests (Symondson et al. 2002; Carlsson et al. 2009). Yet, predator preference towards native prey species compared with novel species may contribute to a time lag in the effectiveness of top–down predatory control (Carlsson et al. 2009; Santamaría et al. 2022).
Sea urchins provide a global example of marine herbivores that, when left uncontrolled by their predators, can escalate to pest status (Galloway et al. 2023). Their excessive grazing can lead to extensive macroalgal destruction, which in turn triggers complete phase shifts (Filbee-Dexter and Scheibling 2014). Fishing pressure on natural predators of sea urchins (e.g. sea otters, lobsters and predatory fish) has resulted in declining predator populations, a key factor driving the proliferation of sea urchins in many regions (Sheppard-Brennand et al. 2017).
In Tasmania, south-eastern Australia, the climate-induced range-extending longspined sea urchin, Centrostephanus rodgersii, is increasing in numbers within the reach of a native invertebrate predator, the southern rock lobster, Jasus edwardsii, Hutton, 1875 (hereafter ‘lobster’). Since its initial detection in Tasmania in 1978, this urchin has proliferated significantly in the north-eastern region of the state, overgrazing kelp-dominated reefs to form ‘extensive urchin barrens’ (Johnson et al. 2005). Extensive barrens here have been established for over two decades and encompass kilometres squared of bare rock with limited or no presence of kelp (usual dominant species: Ecklonia radiata, J.Agardh, 1848 and Phyllospora comosa, C.Agardh, 1839), and significantly lower biodiversity than on undisturbed rocky reef (Ling 2008). Further south, the longspined sea urchin incursion has been more recent. In this region, clusters of urchins graze around their home site, creating ‘incipient’ urchin barrens, i.e. areas of patchy bare-rock barren between healthy kelp-dominated reefs. These incipient barren habitats have a comparatively higher biodiversity than have extensive barrens, likely owing to the higher amount of kelp habitat in close proximity. Incipient barrens have been increasing in Tasmania in recent decades, alongside the southern range-extension of longspined sea urchins (Johnson et al. 2005; Flukes et al. 2012; Ling and Keane 2018, 2021). The Tasmanian longspined sea urchin problem has parallels with other urchin takeovers, with suggestion that overfishing of a primary predator in the system (the lobster) has exacerbated the barren formation (Ling et al. 2009). However, owing to the relatively recent climate-induced range-extension of the urchin, this scenario presents other challenges. Lobsters from C. rodgersii-free habitat in Tasmania were shown to be naive to C. rodgersii as prey and therefore may not provide high predatory pressure in the range recently colonised by C. rodgersii (Carlsson et al. 2009; Smith et al. 2022).
Large lobsters are known to eat the longspined sea urchin in Tasmania (Johnson et al. 2005; Ling et al. 2009; Redd et al. 2014), and increasing their biomass is part of management initiatives to reduce urchin numbers (Department of Primary Industries, Parks, Water and Environment 2018). Increased lobster density in areas protected from fishing for over 10 years has been successful in suppressing expansion of existing urchin barrens, but urchin density remains high at these sites (Ling and Keane 2021). J. edwardsii is a known generalist predator and experimental research has found that lobsters prefer native prey if the choice is available (Smith et al. 2022). It then follows that, in addition to the suppression of longspined sea urchins, significant reductions in the abundance of blacklip abalone (Haliotis rubra, W. E. Leach, 1814), and the native shortspined sea urchin (Heliocidaris erythrogramma, Valenciennes, 1846), were observed at sites with a higher lobster abundance (Ling and Keane 2021). In these areas showing stabilised urchin barrens, we hypothesise that lobster preference for native prey over longspined sea urchin is outweighed by prey availability, because lobsters are left with fewer options for other diet choices.
Habitat type and associated prey recognition (or naivety) is another important factor to consider in this predator–prey interaction and perceived lobster preference. In captive experiments, where prey size nor availability was a limiting factor, lobsters from a long-term, extensive urchin barren were more likely to predate on longspined sea urchins than was a naive lobster from a habitat where the urchin is absent (Smith et al. 2022). This behaviour is suggesting of a learnt response and prey recognition. Other spiny lobsters have been shown to switch to an invasive prey species rapidly once it becomes the only option (Alexander et al. 2022). Further, spiny lobsters, which prefer native prey, significantly increased consumption of novel prey when another predator was present (Skein et al. 2020). Spiny lobsters are therefore capable of a diet switch, but it is unknown where and when this switch takes place in southern rock lobsters, given the absence of a major competitor.
The effectiveness of top–down predatory control further relies on the prey being accessible to the predator. Despite large lobsters often being associated with the predatory pressure on longspined sea urchins in Tasmania, mature sea urchins are known to reach a size refuge, which effectively protects them from predation against even the largest of lobsters (Andrew and MacDiarmid 1991; Ling et al. 2009). Size refuges for sea urchins and other marine prey species have been documented previously (Connell 1972; Andrew and MacDiarmid 1991; Van Zyl et al. 1998; Ling and Johnson 2009) and allow prey species populations to persist even in areas with predators. This size-refuge for C. rodgersii is particularly apparent in its extended range in Tasmania because lobsters are the primary predator (Ling and Johnson 2012). This contrasts with the urchin’s native range, where there is higher diversity of predators, including eastern blue groper (Achoerodus viridis, Steindachner, 1866) and eastern rock lobster (Sagmarisus verreauxi, H. Milne-Edwards, 1851), which are able to attack larger urchins, so size is less important as a refuge (Byrne and Andrew 2020; Day et al. 2023). Further, small urchins within the size range of predation for smaller lobsters are considered to be crevice-dwelling and ‘cryptic’ and therefore inaccessible to predation until they reach the size of emergence (Ling et al. 2009). This upper size refuge and small urchin crevice-dwelling behaviour could potentially narrow the window of opportunity for lobsters to predate on the urchins. Despite this, multiple studies have found evidence of C. rodgersii within the diet of small lobsters (Redd et al. 2008, 2014; Guest et al. 2009; Smith et al. 2022). In some cases, ingestion of urchins by scavenging and sediment uptake is credited for this (Redd et al. 2008, 2014), but a recent study described active predation events by small lobsters (Smith et al. 2022), highlighting the need for further investigation into this interaction.
Lobster diet and predation on longspined sea urchin in Tasmania have previously been studied in different habitats and by using a variety of diet analysis methods. DNA detection of urchin presence in lobster faecal samples has confirmed consumption by lobster and indicated potential importance of predation in extensive and incipient barren areas within marine reserves with high lobster densities (Redd et al. 2014). Stable isotope analysis has confirmed a difference in lobster diet between protected and fished sites (Guest et al. 2009). Single methods often have limitations, such as short-term view of diet (from stomach and faecal DNA analysis) or prior knowledge of important diet components (for stable isotope analysis); so, by combining different methods at once, more accurate insights to diet preference can be obtained (Nielsen et al. 2018).
Using multiple diet analysis techniques, we aimed (1) to determine whether and how the diet of lobsters differs between sites that vary in habitat, including urchin barren extent, C. rodgersii density and protected v. fished status (i.e. lobster density), (2) to determine how lobster size influences the diet of lobsters among the habitats and whether small lobsters contribute to the predatory pressure, and (3) to asses whether J. edwardsii is a universal effective top–down predatory control of C. rodgersii.
Materials and methods
Predator and prey collection
Samples were collected from five sites on the eastern coast of Tasmania, three were no-take reserves with high J. edwardsii density and a varying extent of barren (extensive, incipient, no barren), whereas two were open to fishing, had low lobster density, and incipient barrens present (Fig. 1). In 2008, two no-take research areas were established in areas of high urchin impact. Elephant Rock Fishery Research Area (ER) is situated in a multidecadal, extensive urchin barren in north-eastern Tasmania. This no-take research reserve has a stable, high density of large lobsters (>140-mm carapace length) protected from harvesting and a high density of longspined sea urchins. North Bay Fishery Research Area (NB) is 180 km south and covers incipient urchin barren with a high density of protected large lobsters and a medium density of longspined sea urchins. Crayfish Point Fishery Research Area (CP) was established in 1972 and sits at the mouth of the Derwent Estuary, has no longspined sea urchins present, is barren free, and has a high density of protected large lobsters. The fishery research areas are closed to the take of lobster, and any species, by diving (abalone, urchins and periwinkles). To compare the influence of predator density among sites, lobsters were collected from nearby fished sites with incipient barren habitat. Both of these sites have a medium density of longspined sea urchins and a low density of lobsters. Because of low catch, lobsters from Fortescue Bay (FB) and Cape Paul Lamanon (CL) were grouped into an ‘incipient-fished’ sample (FB/CL) to compare lobster density effect on diet at incipient barren sites (between NB and FB/CL). Further, both incipient barren sites (Fortescue Bay and Cape Paul Lamanon) were closed to abalone fishing in 2020 owing to low stock (Mundy and McAllister 2022); therefore, careful interpretation into prey abundance is required.
Southern rock lobster (Jasus edwardsii) collection sites in eastern Tasmania, describing habitat type, urchin impact and lobster density. Initials represent site names: ER, Elephant Rock Fishery Research Area; NB, North Bay Fishery Research Area; FB, Fortescue Bay; CL, Cape Paul Lamanon; and CP, Crayfish Point Fishery Research Area. Sample sizes for each site are displayed. Silhouettes indicate habitat features at each site (not to scale): presence of range-extending urchins (Centrostephanus rodgersii, Hawkey 2008), presence of kelp (represented by Ecklonia radiata, Carruthers 2002) and presence of fishing pressure on lobsters (represented by a lobster trap).
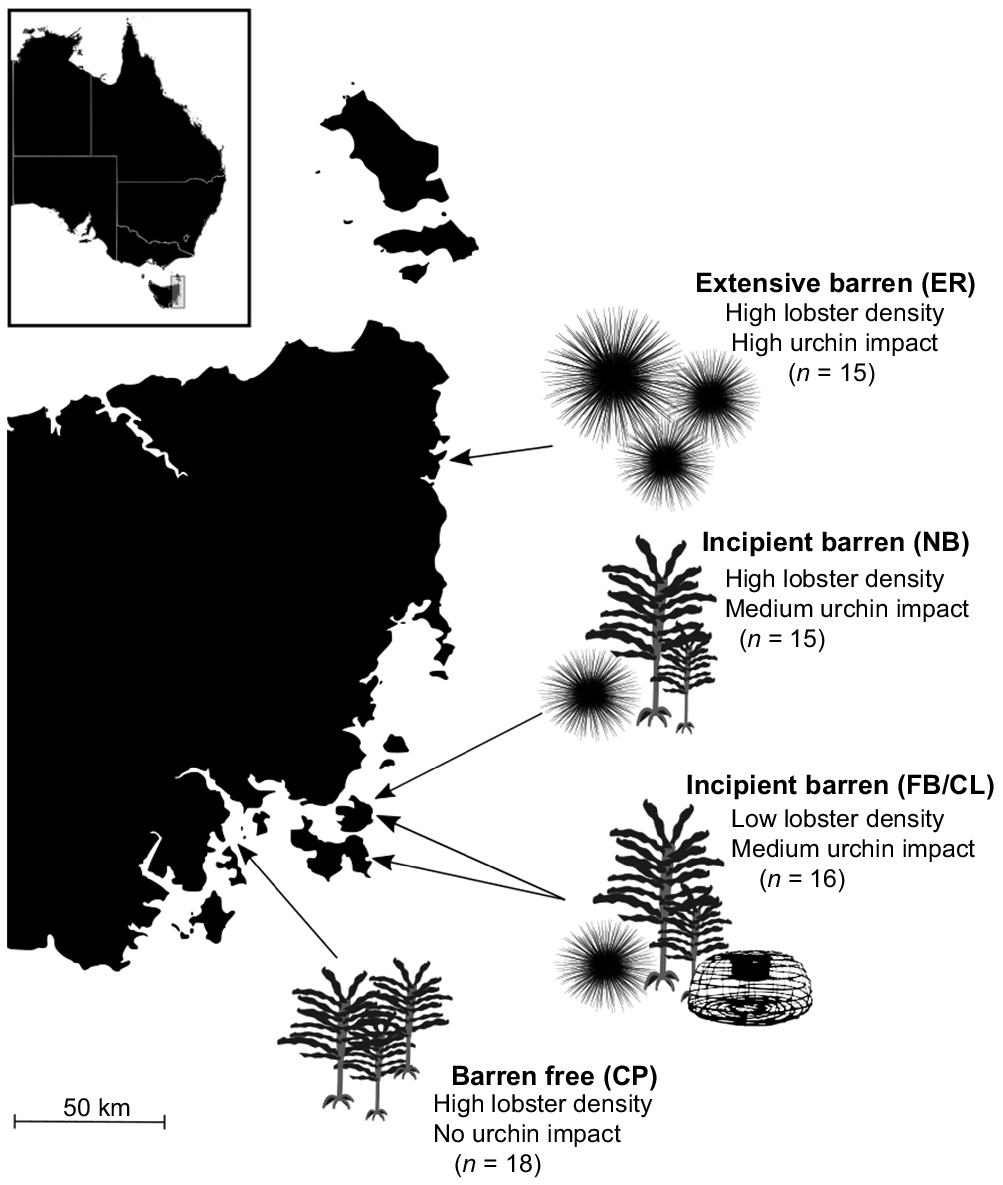
Sixty four J. edwardsii individuals were collected from the eastern coast of Tasmania during the summer season, between December 2019 and February 2020, by modified lobster pots with no escape for small lobsters. Lobsters were targeted between moult periods to ensure active eating behaviours. Lobsters collected were categorised into the following three size classes evenly within each habitat: small (65–109-mm carapace length, n = 21), medium (110–139-mm carapace length, n = 25) and large (140–202-mm carapace length, n = 18). In total, 27 of the lobsters were female and 37 were male. Number of lobsters varied slightly among sites, including Elephant Rock Fishery Research Area (n = 15), North Bay Fishery Research Area (n = 15), Crayfish Point Fishery Research Area (n = 18), Fortescue Bay (n = 6) and Cape Paul Lamanon (n = 10). We sampled six lobsters per size class at each site, except in three cases when only three lobsters within a size class within a habitat could be collected because of low lobster size-class availability. See Table S1 of the Supplementary material for the sampling matrix.
Following methods from Redd et al. (2008, 2014), a pipette was used to extract faecal pellet from the lobster’s anal pore. Faecal samples were immediately added to saline, held on ice, then frozen in −80°C at the soonest convenience (as recommended by King et al. 2008). After faecal collection, lobsters were placed immediately into ice slurry to halt digestion process and freeze the animal as soon as possible (Baker et al. 2014).
Animals were collected under Tasmanian Fisheries permits 19007 and 20090.
We targeted common prey species at each site for detection in lobster diet. These species are the same as those used in previous experiments and reflect important fishery species in Tasmania (Smith et al. 2022).
During the same collection period, five individuals of each target prey species of longspined sea urchin (C. rodgersii), native shortspined sea urchin (H. erythrogramma), blacklip abalone (H. rubra) and wavy periwinkle (Lunella undulata) were collected from each site by divers for stable isotope analysis. For the incipient-fished sites, we collected prey only at at Fortescue Bay. At Crayfish Point, no C. rodgersii was found to collect. Within the extensive barren, at Elephant Rock, native species were collected from within the barren where possible and, alternatively, from the barren edge. Five individuals of blue mussel (Mytilus galloprovincialis) were also collected at Elephant Rock because it was deemed to be in high enough abundance to be potential prey; insufficient numbers of M. galloprovincialis were found at other sites to sample for isotope analysis. We also took five samples of the teleost bait fish Scomber australasicus used in pots. Five C. rodgersii individuals were sampled 1 year later to improve sample size for the Elephant Rock source collection because of degradation of three of the original samples and not being able to separate roe from test. New samples were compared with original samples for similarity before using in analysis.
Stomach-content analysis
All 64 lobsters were dissected and their stomach was removed and preserved in 100% ethanol. Stomachs were cleaned with distilled water and fragments sorted using a dissecting microscope. Photographs were taken of all fragments and tissue found in stomachs and specialist help was sought for identification. Any identifiable fragments within stomachs were categorised down to the lowest possible taxonomic level and recorded as ‘present’. Categorisation resulted in 20 categories, which were reduced to 12 functional categories. Uncommon items were placed into the ‘miscellaneous’ category, which included plastic (n = 1) and parasitic worms (n = 4). Material that could not be assigned were placed into the ‘unidentified’ category. Frequency of occurrence (%F) was then calculated for the lobster population at each site (see Martínez-Coello et al. (2022), Buckland et al. (2017) for more on %F).
DNA analysis of faecal samples
Faecal samples were successfully obtained from 59 lobsters, and analysed for presence of prey DNA. Feacal matter was unavailable from five lobsters because of degradation of the sample, including three from ER and two from NB. Frozen faecal samples were defrosted and centrifuged and the saline liquid was removed using a pipette. Qiagen’s QIAamp PowerFecal Pro DNA Kit was used to extract DNA following the instruction manual (see the ‘QIAamp PowerFecalPro DNA Kit Instruction Manual’ section of the Supplementary material). DNA concentrations were analysed using a ThermoFisher Nanodrop 8000 spectrophotometer. All instruments and consumables were sterilised using a UV hood prior to extraction and all work was conducted on a sterile bench to reduce the likelihood of contamination.
Method optimisation, including positive template testing and gradient polymerase chain reactions (PCRs), were conducted prior to sample analysis. Although previous literature contains primers supposedly fit for this project, they were unable to differentiate among our target species (Redd et al. 2014). New, species-specific primers were designed using the R package ‘DECIPHER’ (ver. 2.20.0, see http://www2.decipher.codes/; Wright 2016, Table S2 of the Supplementary material). Primers were tested against positive and negative controls and cross-tested against all other species used.
PCR was conducted with Bioline MyTaq Red HS Mix in 20-μL reactions with 1 μL of DNA in 20 μL. Each primer required different annealing temperatures, but all were run through a 34× cycle. Specific primers and annealing temperatures are available in Table S2. Thermal cycling conditions for all four primers were the same, other than the specific annealing temperature, as follows: denaturation and DNA polymerase activation at 95°C for 3 min, followed by 34 repeat amplification cycles: 95°C for 30 s, followed by the primer-specific annealing temperature for 30 s, 72°C for 1 min. The final extension step was 72°C for 5 min and the reaction was held at 12°C until removed from the Bio-Rad T100 PCR cycler. PCR products were transferred to 2% agarose gel with Nippon Genetics Midori Green Advance for electrophoresis. All gels were loaded with 5 μL of PCR product and a 100-bp ladder used on every gel to confirm results. Gels were run at 80 V for 20 min and then a further 10 min to confirm band separation. PCR product bands were visualised using the BioRad GelDoc Go imaging system and all were repeated at least once, and up to twice to ensure clarity.
Positive and negative occurrence of target DNA in a faecal sample was treated as a categorical variable (occurrence: yes or no) and summed within each grouping factor in the dataset (target prey species, site, sex and lobster size class). A generalised linear model (formula below) was assessed using ANOVA to determine the influence of our grouping factors on the presence or absence of each prey species in lobster faecal samples.
Stable isotope analysis
During lobster dissections (n = 64), a 1-cm2 piece of dorsal muscle was taken from beneath the dorsal carapace on the left of the lobster. Soft tissue was removed from the five individuals of each target prey species at each site and the five samples of bait fish (teleost). Soft tissue included gonads for the two urchin species and muscle tissue for abalone, periwinkle, mussel and teleost, as previous work suggests this is what the lobsters consume (Smith et al. 2022). All tissue samples from lobsters, prey species and teleost were placed into glass vials and oven dried at 60°C for at least 48 h. Dried samples were weighed until dry weight stopped decreasing, indicating that all moisture had been removed. Dry samples were then ground into a homogeneous powder by using mortar and pestle. A subsample of 0.05 mg of sample powder was then weighed and folded into tin capsules for analysis at the Central Science Laboratory, University of Tasmania (Hobart, Tas., Australia).
Carbon and nitrogen stable isotopes were analysed using flash combustion isotope ratio mass spectrometry (varioPYRO cube coupled to Isoprime100 mass spectrometer, Elementar, Germany). The nitrogen produced during combustion is directly fed into the mass spectrometer and measured against a reference gas. Carbon dioxide is trapped in the meantime and released into the mass spectrometer after the nitrogen isotope measurement is completed and the system retuned accordingly.
Stable isotope abundances are reported in delta (δ) values as the deviations from conventional standards in parts per thousand (‰) from the following equation:
where X is 13C or 15N and R is the ratio 13C:12C or 15N:14N. δ13C and δ15N values are reported respective to PDB (Pee Dee Belemnite) and atmospheric air respectively. International reference standards with known isotopic composition (USGS 25, USGS 40, USGS 41, IAEA-N1 and IAEA-N2, NBS 21, USGS 24 and ANU-NAT-76H) were used to correct for instrumental drift and quality assurance purposes. The analytical performance of the instrumentation, drift correction and linearity performance were calculated from the repetitive analysis of these standards. Precision was 0.1% for both isotopes.
Using experimentally determined species-specific trophic discrimination factors (TDFs) for dorsal tissue, namely, 4.45 ± 1.04 for δ13C and 4.36 ± 0.6 for δ15N (Smith et al. 2023), R package MixSIAR (ver. 3.1.12, see https://cran.r-project.org/package=MixSIAR; Stock and Semmens 2022) was used in R (ver. 4.1.1, R Foundation for Statistical Computing, Vienna, Austria, see https://www.r-project.org/), in RStudio (ver. 2021.09.0, RStudio Posit Software, PBC, Boston, MA, USA, see https://posit.co/) to run four separate Bayesian mixing models for lobster populations from each of the four habitat types. Analytical precision values were incorporated into the model by combining with the source error (prey species) and TDF error (consumers). This ranged between 0.07 and 0.33‰ for δ15N and between 0.01 and 0.1‰ for δ13C. To meet minimum recommended stable isotope sampling requirements (n = 5, Mazumder et al. 2008) and because of there being no pattern in lobster size or sex and isotopic signature, lobsters were not grouped by size or sex in the final mixing models.
Isoplots created by MixSIAR allowed visual inspection of the source separation but for clarity; MixSIAR models on each site were run until convergence (checked using Geweke and Gelman diagnostics; Stock and Semmens 2022) and then the correlation between sources was assessed using the plots provided by the package. The correlation refers to the similarity of the isotopic signatures of the sources, if the correlation between two sources is high, the model may not be able to distinguish them and therefore the sources may need to be pooled into one. Despite some sources showing a high overlap in the correlation, we did not pool unless the absolute value of the correlation coefficient was over 0.5 (Giraldo et al. 2017) and the species in question could be pooled on an ecological basis. Source-pooling examples here include periwinkle and abalone because they are both gastropods and benthic grazers; however, the two urchin species differ because C. rodgersii is an omnivore but H. erythrogramma is an obligate herbivore. If this combination of factors were to occur, the two prey species would be combined and the model rerun (Giraldo et al. 2017). According to the model, abalone and periwinkle were correlated (correlation coefficient: 0.87) at Crayfish Point and so were pooled and the model was run again with one combined abalone–periwinkle source.
Results
Our primary results showed that lobsters are generalist predators, diet varies among our study populations, and depending on diet analysis method used, habitat and lobster density had a varied impact on lobster diet. Longspined sea urchin contributed to the diet of lobsters at all sites in which C. rodgersii was present. Several native benthic herbivores that we targeted as prey species of interest, including H. erythrogramma, H. rubra and L. undulata, were also present in lobster diet. Size of the lobster did not appear to influence the diet or the contribution of target species to the diet.
Stomach-content analysis
Stomach-content analysis provided details on the broad spectrum of lobster diet but displayed very low detection rate of our four target species (Fig. 2). Urchin remains were present in 3 of the 64 stomachs (5%), with identification to species level possible in two of these, namely, one longspined sea urchin in the extensive barren (Elephant Rock, large lobster), one H. erythrogramma in the incipient-fished (Fortescue Bay, small lobster) and one unidentified urchin in the incipient-reserve (North Bay, small lobster). In six stomachs (9%), abalone was identifiable by the unique holes in its shell. Unidentified gastropods were found in 22% of stomachs but were not possible to describe to more detailed taxonomic level because of small fragments. It was not possible to identify periwinkle in any of the stomachs. Aside from the unidentified matter and apparent vertebrate contribution to diet, the predominant dietary component found in lobster stomachs was bivalves (including blue mussel), occurring in 17 stomachs (27%) (Fig. 2). Across all sites, a large proportion of the contents could not be identified (63%). Vertebrate remains were also identified in 58% of the stomachs.
Percentage frequency (%F) of the Jasus edwardsii population sampled at each site with target and non-target diet items in their stomach. Target species are shown on the x-axis and non-target species were grouped into a stacked bar named ‘Other’. Each colour in the stacked bars is equal to the %F of the associated group; therefore, the stacked bar total can be over 100%. Other, non-target diet components were detected at a higher frequency than were target species. Periwinkle was not detected in any stomachs. (a–c) The three reserve sites (high lobster density). (d) The results from lobsters collected from fished, incipient barren sites (low lobster density). Silhouettes indicate habitat features at each site (presence of sea urchins, kelps, lobster traps). Prey items are represented as ‘Centro’ for longspined sea urchins and ‘Helio’ for shortspined sea urchin and abalone. Initials represent site names: ER, Elephant Rock Fishery Research Area; NB, North Bay Fishery Research Area; CP, Crayfish Point Fishery Research Area; and FB/CL, Fortescue Bay and Cape Paul Lamanon.
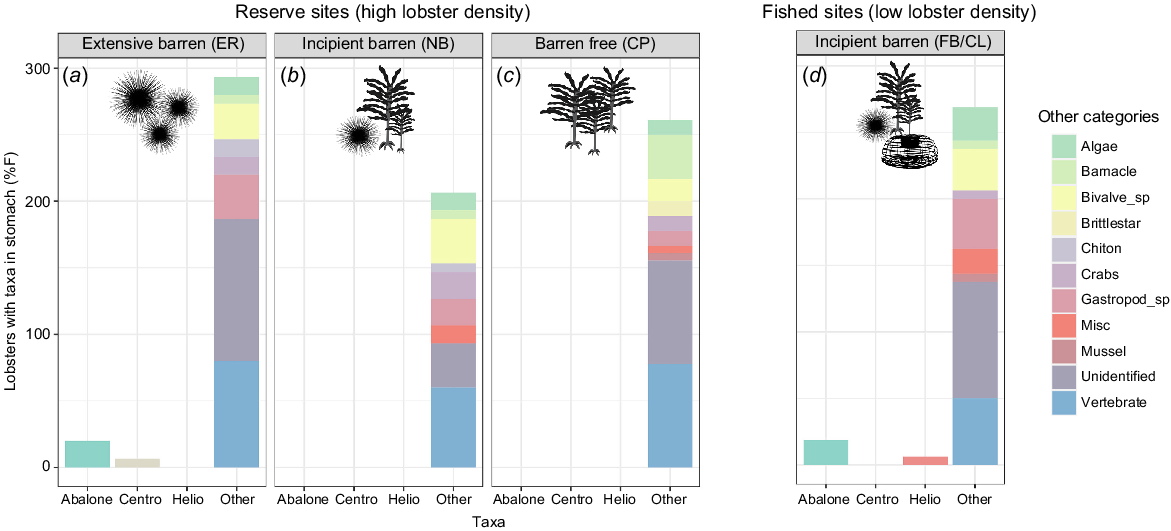
DNA analysis of faecal samples
DNA analysis provided insight into habitat-associated differences in target prey contribution to lobster diet. DNA from all four of the target species was found in lobster faecal samples; 45.8% of all samples had longspined sea urchin, 25.4% had abalone, 8.5% had periwinkle and 3.4% had shortspined sea urchin. The majority of faecal samples (71%) gave at least one positive prey-detection result.
The relative proportion of prey items in the diet confirmed by DNA methods was site dependent (Fig. 3). Longspined sea urchin was the most common prey species detected in all sites, except the barren free site; they were found in 100% of lobsters sampled from the incipient-fished population (FB/CL), 54% of lobsters from the incipient-reserve (NB) and 33% from the extensive barren (ER). In the barren-free habitat (CP), H. rubra was the most common prey species detected in lobsters (44%) and longspined sea urchins were not detected. H. rubra was also detected at both incipient sites (31% at FB and 15% at NB). L. undulata was detected only in samples from the incipient-fished site (19%) and the extensive barren (17%) habitat. H. erythrogramma was detected only in low numbers in lobsters from the barren-free (CP, 6%) and incipient-reserve site (NB, 8%).
Percentage frequency (%F) of positive DNA detections of targeted prey species in the faecal samples of Jasus edwardsii at each site. (a–c) The three reserve sites (high lobster density). (d) Results from lobsters collected from fished, incipient barren sites (low lobster density). Silhouettes indicate habitat features at each site (presence of sea urchins, kelps, lobster traps). Prey items are represented as ‘Centro’ for longspined sea urchins and ‘Helio’ for shortspined sea urchin and full names for abalone and periwinkle. Initials represent site names: ER, Elephant Rock Fishery Research Area; NB, North Bay Fishery Research Area; CP, Crayfish Point Fishery Research Area; and FB/CL, Fortescue Bay and Cape Paul Lamanon.
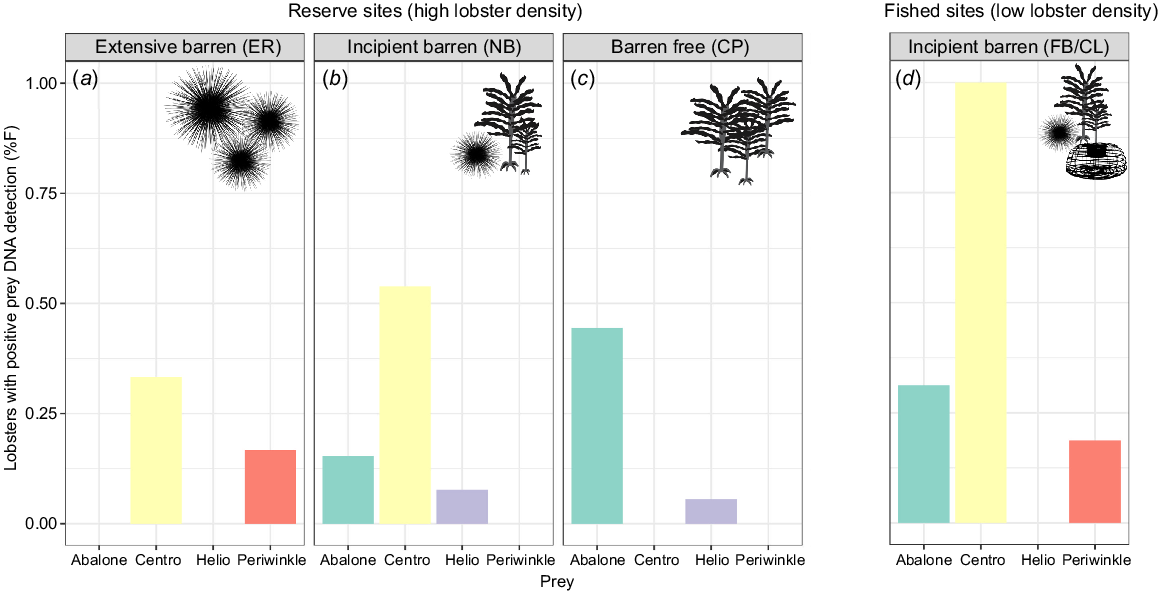
In our population of sampled lobsters across all sites, DNA of every prey species was detected in lobsters of all size classes, except H. erythrogramma, which was not detected in large lobsters at all. Within each site, not all size classes were found to consume all prey species. Longspined sea urchin was detected in small lobsters from both of the incipient barren populations, but not in small lobsters from the extensive barren, whereas at all three sites the longspined sea urchin was detected in large and medium lobsters (Fig. S1 of the Supplementary material). However, the generalised linear model showed that there was no significant effect of lobster size class, site, prey species or their interactions on the number of positive or negative detections of DNA in the whole population of lobster samples tested (ANOVAs, P > 0.05, Table S3 of the Supplementary material).
Stable isotope analysis
Stable isotope mixing models identified habitat-specific differences in prey contribution to long-term lobster diet in each population (Fig. 4). Habitat was an important determiner of diet; longspined sea urchin was more important to lobsters from incipient barrens than from other habitat types. This was consistent between the high lobster density populations (NB, mean: 0.73 and s.d.: ±0.09) and low lobster density populations (FB/CL, 0.54 ± 0.12) (Fig. 4b, d). There was also an increased importance of teleost in the incipient-fished population (FB/CL, 0.24 ± 0.07), compared with the incipient-reserve (NB, 0.12 ± 0.06). At the extensive barren site, periwinkles were identified as the most important prey item (ER, 0.48 ± 0.13), and in the barren-free habitat, the combined contribution of abalone and periwinkle (CP, 0.76 ± 0.09) outweighed other possible diet items.
MixSIAR predicted contributions of targeted prey species to Jasus edwardsii diet at each site. Boxes show the 25–75 percentiles with median diet in the centre, whiskers in black show the 5–95 percentiles. Note: some sites have different numbers of available prey, so a separate MixSIAR model was run for each site. (a–c) The three protected sites (high lobster density). (d) Results from lobsters collected from fished, incipient barren sites (low lobster density). Silhouettes indicate habitat features at each site (presence of sea urchins, kelps, lobster traps). Prey items are represented as ‘Centro’ for longspined sea urchins and ‘Helio’ for shortspined sea urchin and full names for abalone and periwinkle. Initials represent site names: ER, Elephant Rock Fishery Research Area; NB, North Bay Fishery Research Area; CP, Crayfish Point Fishery Research Area; FB/CL, Fortescue Bay and Cape Paul Lamanon.
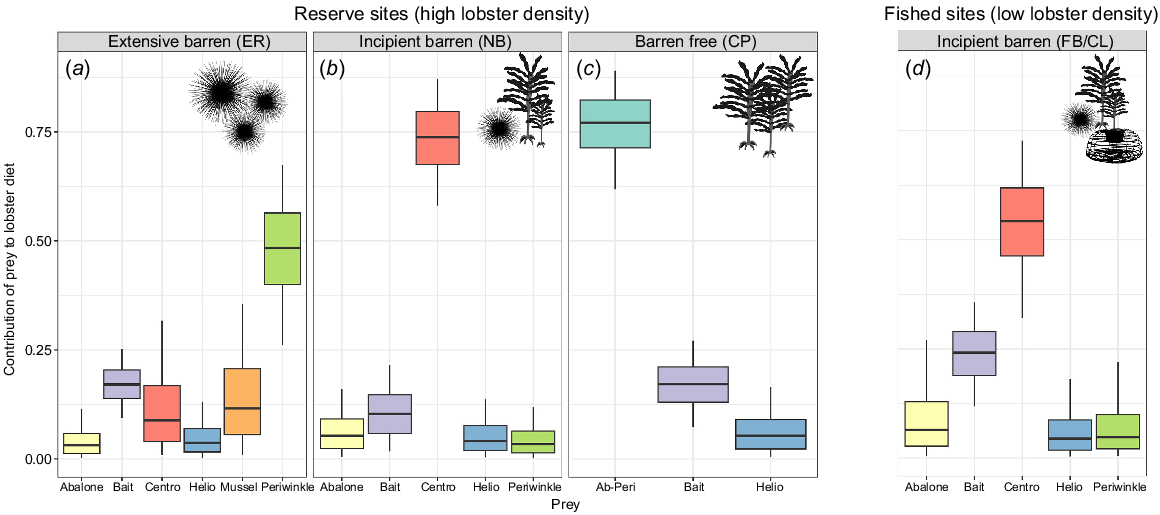
The mean δ13C and δ15N values for the population of lobsters sampled at the barren-free habitat (CP, −17.5 ± 0.43 and 13.6 ± 0.58 respectively, n = 18) differed significantly from those in all the other sites, being reduced in δ13C (ANOVA, F(3,60) = 27.5, P < 0.001) and enriched in δ15N (ANOVA, F(3,60) = 19.6, P < 0.001). No significant pair-wise differences were found among the other sites (ANOVA, Tukey comparisons, P > 0.05).
Stable isotope signatures across size classes were site dependent and inconsistent (Fig. S2 of the Supplementary material). ANOVA highlighted a significant interaction between site and size (F(6,52) = 3.2, P = 0.01). The only site at which size class appeared to influence δ13C was the incipient-fished population where medium lobsters were slightly enriched in δ13C compared with large lobsters (FB/CL, t-test, t = 2.3, P = 0.03), but ANOVA showed that size did not overly affect δ13C at the site (FB/CL, ANOVA, F(2,13) = 3.6, P = 0.06). Within each site, the effect of size was non-significant for δ15N (ANOVA, F(6,52) = 0.6, P = 0.71). No significant interaction of lobster sex and site was evident for δ13C at any of the sites (ANOVA, F(3,56) = 0.24, P = 0.87), nor was there a difference in δ15N between sexes at any site (ANOVA, F(3,56) = 0.53, P = 0.66). See Fig. S1 for visual comparisons. The influence of size within the incipient-fished population was tested in the stable isotope mixing models by including size as a fixed effect in the model. The model failed to converge when size was included (see Table S4 of the Supplementary material for more information).
Mean δ13C and δ15N values for C. rodgersii roe sampled from Elephant Rock (extensive barren) 12 months apart did not differ (δ13C, t-test, t = 0.76, d.f. = 2.41, P = 0.51; δ15N, t-test, t = −1.01, d.f. = 1.49, P = 0.45).
Graphical analysis of stable isotopes by isoplots confirmed that the population of lobsters sampled at each site fall within the isospace of the prey species (Fig. S3). Several samples from the incipient-fished population were distant from target sources, indicating consumption of items depleted in δ13C. At all the other sites, the source polygon covers the consumer points, but the spread of consumers is relatively wide.
Correlation of some sources were 0.5 or higher at the extensive barren (ER, periwinkle–mussel, 0.53; periwinkle–longspined, 0.50), the incipient-fished (FB/CL, abalone–longspined, 0.50), the incipient-reserve (NB, longspined–teleost, 0.72) and the barren-free (CP, abalone–periwinkle–teleost and abalone–periwinkle–shortspined, 0.76 and 0.78 respectively) sites, but these were not pooled because it would not be ecologically sensible because the species occupy different trophic niches. Graphical analysis by isoplots confirmed that there was also overlap across some diet sources (Fig. S3). This correlation and overlap leads to model uncertainty in distinguishing between sources and so explains the large overlapping error bars in Fig. 4. All other prey were not overly correlated at any of the sites according to the model output (absolute correlation coefficient less than 0.5).
Discussion
We hypothesised that lobsters would consume more longspined sea urchins in the extensive barren habitat than in incipient barrens, because of the reduced likelihood of lobster naivety, limited prey options and increased likelihood of encountering a longspined sea urchin; however, our results did not support this. At the extensive, multi-decadal urchin barren site (ER), we found a lower than expected contribution of longspined sea urchin to lobster diet and an elevated importance of periwinkle. In comparison, longspined sea urchin was detected in relatively higher rates in lobster diet from both incipient barren populations (NB with high, and FB/CL with low lobster density; Ling and Keane 2021). Further, we saw that all size classes of lobster appear to consume C. rodgersii in all sites at which the urchin exists. As expected, longspined sea urchin did not contribute to the diet of lobsters at the site where C. rodgersii was not present.
Divergent findings of several field and aquarium experiments hint at a greater complexity in the importance of longspined sea urchin in lobster diet and preferences among lobsters than previously thought. Previous work on lobsters in Tasmania has shown that lobsters from extensive barrens do prey on longspined sea urchins in the wild and in captivity (Redd et al. 2008, 2014; Ling et al. 2009; Smith et al. 2022). Yet, we observed here that lobsters predominantly consume native species at our extensive barren site, despite significantly reduced abundances of all prey species except longspined sea urchins. Differences in lobster diet between habitat types and with lobster density may be explained by the following: first, by preference for native prey over the longspined sea urchin, which when combined with high lobster densities, could reduce available prey options; and second by habitat-associated size-structure of longspined sea urchins, which may dictate prey availability to lobsters. Our findings may have implications for the management of predatory lobsters as a control tool for urchins in Tasmania, when combined with site-specific data on urchin population density and size structure.
Implications of lobster prey preference
DNA detection rates at the extensive barren (ER) and incipient-reserve (NB) sites directly align with those in a previous study conducted at these sites, which utilised DNA detection of lobster faecal samples to infer lobster predation on the two urchin species (Redd et al. 2014). Relative proportion of longspined sea urchin in lobster diet from our 2020 sampling approximately match those of 2008–2011 (within 5%), namely, 33% (2020) and 38% (2008–2011) at ER, and 27% (2020) and 25% (2008–2011) at NB. However, for the native shortspined sea urchin, we found decreased detection in 2020 at both sites, namely, 36% (2008–2011) to 0% (2020) at ER, and 32% (2008–2011) to 17% (2020) at NB). These declines in H. erythrogramma detections reflect a decreased presence of H. erythrogramma observed at the same sites during the same time period between 2008 and 2020 (Ling and Keane 2021). The decline in native preferred species at the sites with high lobster density suggests to us that predation is the likely cause of significant H. erythrogramma population decline. Further, despite known lobster preference for abalone over the longspined sea urchin (Smith et al. 2022), the lesser detection of abalone in the diet at the extensive and incipient barren sites is proportional to the depleted abalone abundance seen at these sites (Ling and Keane 2021; Mundy and McAllister 2022). Abalones are extremely rare within the extensive urchin barren, and this correlates with there being no DNA detections at this site. The decrease in native prey species in areas with high lobster densities indicates that lobsters preferentially consume native prey. Spiny lobsters are known to exhibit the ability to switch preference under certain circumstances, e.g. competition or scarcity of native prey options (Skein et al. 2020; Alexander et al. 2022). Therefore, in Tasmania, once native prey diminishes, lobsters may switch to secondary choices such as longspined sea urchin (Smith et al. 2022).
Longspined sea urchins consistently contributed proportionally less to the diets of lobster in the extensive barren sites than in both of the incipient barren sites; this was highlighted by both the stable isotope results and the DNA detections (Fig. 3, 4). Instead, at the extensive barren site, periwinkles were highlighted as an important contributor to lobster diet by both DNA and stable isotope approaches, a choice known be preferred by the lobster (Smith et al. 2022). It is possible that in our stable isotope analysis, the periwinkle ‘source’ represents a wider suite of gastropods important to lobster diet, as highlighted in our stomach-content results. An additional form of prey preference, and reason to why less urchins are consumed in the barren, may be habitat-associated urchin quality, because barren urchins are less attractive than are kelp urchins to other spiny lobsters, owing to degraded roe quality (Eurich et al. 2014). However, roe-quality testing between barren and kelp habitats in Tasmania has suggested that this influence may be minimal, because barren urchins in Tasmania are maintaining roe quality by consumption of drift algae (Campus 2021).
Importance of urchin size and evidence of a size refuge
Small lobsters have previously been considered to predate minimally on longspined sea urchins, with ingestion being attributed to means other than direct predation (e.g. scavenging or ingesting DNA through sediment; Redd et al. 2014). This is, first, due to the fact that small lobsters can physically handle only small urchins, because the lobsters’ front legs are too short to reach beyond the long spines of larger urchins (Tegner and Levin 1983; Ling et al. 2009), and second, due to the cryptic, non-emergent behaviour of smaller urchins, which is thought to make them inaccessible to lobster predation (Ling et al. 2009; Ling and Johnson 2012; Ling and Keane 2021). However, mounting evidence, including this study, suggests small lobsters actively predate on small, cryptic longspined sea urchins. We found that C. rodgersii was present in the diet of small, medium and large lobsters from various sites surveyed, and overall lobster size proved not to be an important factor in the consumption of either native prey or the longspined sea urchin. Similarly, Redd et al. (2014) found ‘higher than expected’ positive DNA detections of longspined sea urchin in small lobsters. Further, we now know that small longspined sea urchins are vulnerable to predation during nocturnal foraging events when both lobsters and urchins are active (Frusher et al. 2009; Flukes et al. 2012; Byrne and Andrew 2020; J. E. Smith, unpubl. data). For these reasons, we present an alternative hypothesis, namely, that small southern rock lobsters do predate on longspined sea urchins and this species is a key dietary component in the absence of native or preferred prey.
Lobsters have a relative maximum urchin size they can predate on, after which the urchins become too large for lobsters to handle (Tegner and Levin 1983; Ling et al. 2009). This size refuge means that the actual availability of urchins to lobsters can be low even at a site with a high density of urchins, because if the majority of urchins surpass the size lobsters can handle, they are essentially protected from predation. The size structure of urchin populations may therefore influence the effectiveness of predators in controlling urchin populations. For example, poor urchin recruitment will lead to populations skewed to larger individuals, whereas removal of large urchins (e.g. by commercial fishing) can result in higher recruitment of juveniles and more smaller urchins (Blount 2004, in Byrne and Andrew 2020). In these scenarios, it is likely that lobster prey preference could be driven by prey availability in the form of availability of suitably sized urchins. Results from DiFiore and Stier (2023)’s predation study on California spiny lobster Palunirus interruptus and local urchin species Strongylocentrotus purpuratus support our concept; that is, the more small urchins that are available, the more all sizes of lobster predate on urchins. As the availability of small urchins is reduced and the population is dominated with large urchins, the predatory interaction between lobsters and urchins is minimised (DiFiore and Stier 2023). Therefore, it is important to consider the size structure of urchin populations at different sites to understand whether availability of size-dependent prey may determine lobster predation rate.
In our results, lobster size class (between 65- and 202-mm carapace length) did not appear to influence diet; however, a larger sample size would be beneficial to learn more about sizes within sites. Despite lobster size appearing not to influence diet here, we hypothesise that urchin size may play an important role in the diet differences we observed among habitat types. Our incipient barren sites at which lobsters have a high proportion of urchins in the diet also are known to have a high proportion of suitably sized urchins, which are considered accessible to predation by lobsters (<95-mm test diameter): 43% at the incipient-reserve (NB) and 64% at the incipient-fished site (FB/CL) (Ling and Keane (2021), data also collected 2020). Conversely, the extensive, multidecadal barren site where we expected high urchin proportion in the diet, had a relatively low proportion of these suitably sized urchins (16%) compared to incipient sites and therefore a much higher proportion of inaccessible urchins within the size refuge, outside of the lobster’s physical handling range. Interestingly, our DNA detected evidence of longspined sea urchin in small lobsters from both incipient barrens (where small urchin abundance is relatively high), but not in small lobsters from the extensive barren (where most urchins are within the size refuge). In the context of the range expansion of the longspined sea urchin, and therefore relative age of the established urchins populations at our sites (i.e. the extensive barren being older establishment and the incipient sites as more recent establishment), the size-structure and subsequent suitable-size urchin hypothesis supports our lobster diet results.
The low proportion of suitably sized urchins in the extensive barren, and a lower proportion of longspined sea urchins in the diet, could result from the high number of lobsters that have diminished the suitable urchin size class over time. High lobster densities in the sites we studied are due to fishing restrictions (closed to fishing for >10 years), and therefore higher historical predation rate may have reduced the number of urchins currently available to predation. Alternatively, low numbers of smaller urchins may be due to the multidecadal barren site reaching a certain ‘carrying capacity’ and displaying reduced recruitment with high density of adults, as seen in experimental sites in New South Wales, eastern Australia (Blount 2004, in Byrne and Andrew 2020). Either way, this has implications for lobster predatory control in long-term extensive barrens, because if there is low abundance of urchins below the suitable-size threshold that lobsters can prey on, lobsters will not have a high predatory impact. Further, it implies lobsters, by predation, may be unable control the urchins after the population begins to reach the refuge size. This means that despite actions in Tasmania to increase lobsters for predatory control in urchin-affected areas, the predators may not have the required impact where long-established, extensive barrens reduce juvenile recruitment. Selective predation of small longspined sea urchins by lobsters, in combination with variable recruitment of the urchins, may shift the population size structure to a state in which urchins switch from being a common diet component to an inaccessible prey target. Importantly, over a long period (e.g. the longevity of the urchin, up to 50 years; Ling and Johnson 2009), consistent predation on smaller urchins could destabilise the population as the mature/larger urchins begin to naturally age and deteriorate. If the predator–prey relationship between J. edwardsii and C. rodgersii is dynamic rather than static, then predictive modelling of urchin control by lobsters must take into account the effect of prey availability, and the size-dependent nature of prey preference.
Implications for management
Here we found that longspined sea urchin is present in the diet of small-sized lobsters, matching captive-feeding experiments and implying their potential in predatory urchin control. Subsequently, on healthy kelp reefs, the reef resilience may be greater than previously assumed, owing to the previously unattributed predatory pressure that small and medium-sized lobsters contribute to the system. However, in well-established high urchin-density habitats dominated by large longspined sea urchins, such as the extensive barren habitat at Elephant Rock, longspined sea urchins played a diminished role in the diet of lobsters. Evidence suggesting that large urchins are physically or preferentially not predated on (Ling et al. 2009; Smith et al. 2022) means that, at such sites, lobsters are likely to have limited effect at controlling urchin density, and additional methods of control may be required to reduce urchin numbers. This further emphasises that establishment of large predator populations (e.g. by protected areas) after the establishment of a C. rodgersii barren (where urchin size refuge has been reached) is unlikely to restore kelp beds, at least within decadal time frames (Johnson et al. 2013).
This study has greatly increased our understanding of predator–prey dynamics of lobsters and longspined sea urchins, and has provided a basis for applying ecosystem-based fishery management approaches to addressing the longspined sea urchin problem in Tasmania and elsewhere. Our hypothesis that small lobsters, as much as larger lobsters, provide a predatory pressure on small urchins during nocturnal foraging events is important for evolving our expectations of ecosystem-based fisheries management in Tasmania. Our results emphasise the importance of basing the stock rebuilding of lobsters in Tasmania on the performance indicator of total biomass (rather than just large lobster biomass, Department of Primary Industries, Parks, Water and Environment 2018), because all size classes of lobster appear to contribute to urchin predation. In this way, our results support the rebuild strategy in Tasmania, to increase the total biomass of lobsters (of all sizes), so as to increase predation on the longspined sea urchin and increase reef resilience.
Diet analysis methods: strengths and limitations
A range of target and non-target species was found to contribute to lobster diet at all sites, by using a variety of diet analysis methods. Stomach-content analysis was less robust for detecting our target prey species, but provided insight on the broad spectrum of lobster diet and implied importance of other species, such as gastropods. The long-term reflection of diet obtained from stable isotopes is a valuable addition to the short-term data obtained by the DNA method. Both methods provide valuable and complementary insight to the importance of longspined sea urchin in lobster diet. Further combination of stable isotope results with stomach-content analysis confirmed that teleosts have a relatively low importance in lobster diet, and a high detection rate of vertebrate through stomach-content analysis was likely to be due to the baited capture method.
Applying multiple diet analysis techniques on the same lobsters enabled us to identify methodological strengths and limitations, and compensate for method-specific limitations in our assessment of lobster diet. For example, without prior knowledge including species-specific genetic primers, stable isotope reference samples and species-specific trophic discrimination factors, DNA and stable isotope methods will either not detect missing prey species (DNA) or group them with other taxa (stable isotopes, as mentioned with periwinkles above), potentially leading to overestimation of the importance of some diet components. Conversely, stomach-content analysis is valuable for visually detecting unexpected species, but relies on expert identification skills and prey fragments being in an identifiable condition. In this study, bivalves and gastropods were the highest contributors to the ‘other’ category in stomach contents but were not considered through other methods and so may be under-represented in our stable isotope and DNA results. Logistically, sampling every possible diet item of such a generalist predator for stable isotope analysis would be unrealistic, and our DNA results alongside previous feeding-preference trials (Smith et al. 2022) confirmed that the target prey species we included are generally present in the lobster diet.
Conclusions
This work has advanced the knowledge of southern rock lobster diet and will be important in future planning of efforts to control the range extension of longspined sea urchins in Tasmania. The suite of methods applied in this study provided further evidence that lobsters of all sizes prey on longspined sea urchins in Tasmania. We showed that the longspined sea urchin does make up a significant proportion of lobster diet in some habitats, despite the lobster’s known preference for native prey (Smith et al. 2022). Lobsters from incipient barren sites with a higher abundance of small- to medium-sized C. rodgersii showed a higher prevalence of the urchins in their diet than of native prey options, and a higher prevalence than did lobsters from the extensive barren. For lobsters from the multidecadal extensive barren we found a reduced proportion of longspined sea urchin in the diet, likely owing to the lack of small, accessible urchins available at the site. Overall, our results showed that the occurrence of longspined sea urchin in lobster diet is diminished as urchin populations reach a size refuge, further indicating that lobsters will be more effective in the prevention of longspined sea urchin barrens, than reversing barren habitats once formed.
Data availability
Data from this study are openly accessible at the PANGAEA data repository at: doi:10.1594/PANGAEA.962719. R scripts are available at the Zenodo data repository accessible at: doi:10.5281/zenodo.8157728.
Declaration of funding
This project and J. E. Smith were partly funded by the Holsworth Wildlife Research Endowment – Equity Trustees Charitable Foundation & the Ecological Society of Australia and by the Tasmanian Government under FRDC Project: 2021-020. M. Oellermann was supported by the German Research Foundation Fellowship 326692763. J. E. Smith was supported by an Australian Government Research Training Program (RTP) Scholarship.
Acknowledgements
We acknowledge the palawa and muwinina peoples, traditional custodians of lutruwita (Tasmania), in which this study took place. J. E. Smith thanks Adam Davey, Chris Dietz, Sharee McCammon, Adam Smolenski, Scott Ling, Lachie Tainsh, Aidan Dudgeon and Stuart Isles for technical support.
References
Alexander ME, Skein L, Robinson TB (2022) Rapid learning in a native predator shifts diet preferences towards invasive prey. Biology Letters 18(3), 20210655.
| Crossref | Google Scholar |
Andrew NL, MacDiarmid AB (1991) Interrelations between sea urchins and spiny lobsters in northeastern New Zealand. Marine Ecology Progress Series 70, 211-222.
| Crossref | Google Scholar |
Baker R, Buckland A, Sheaves M (2014) Fish gut content analysis: robust measures of diet composition. Fish and Fisheries 15(1), 170-177.
| Crossref | Google Scholar |
Buckland A, Baker R, Loneragan N, Sheaves M (2017) Standardising fish stomach content analysis: the importance of prey condition. Fisheries Research 196, 126-140.
| Crossref | Google Scholar |
Cabrera-Guzmán E, Crossland M, Shine R (2011) Can we use the tadpoles of Australian frogs to reduce recruitment of invasive cane toads? Journal of Applied Ecology 48(2), 462-470.
| Crossref | Google Scholar |
Carlsson NOL, Sarnelle O, Strayer DL (2009) Native predators and exotic prey – an acquired taste? Frontiers in Ecology and the Environment 7(10), 525-532.
| Crossref | Google Scholar |
Carruthers T (2002) Ecklonia radiata: illustration of Ecklonia radiata. (University of Maryland Center for Environmental Science) Available at https://ian.umces.edu/media-library/ecklonia-radiata/ [Verified 24 April 2023]
Connell JH (1972) Community interactions on Marine Rocky Intertidal Shores. Annual Review of Ecology and Systematics 3(1), 169-192.
| Crossref | Google Scholar |
Day J, Knott NA, Ayre D, Byrne M (2023) Effects of habitat on predation of ecologically important sea urchin species on east coast Australian temperate reefs in tethering experiments. Marine Ecology Progress Series 714, 71-86.
| Crossref | Google Scholar |
Department of Primary Industries, Parks, Water and Environment (2018) Tasmanian Rock Lobster Fishery: East Coast stock rebuilding strategy 2013–2023. Technical report. (Wild Fisheries Management Branch, DPIPWE, Tasmanian Government, Australia) Available at https://fishing.tas.gov.au/Documents/East_Coast_Stock_Rebuilding_Strategy_Sept18.pdf
DiFiore BP, Stier AC (2023) Variation in body size drives spatial and temporal variation in lobster–urchin interaction strength. Journal of Animal Ecology 92(5), 1075-1088.
| Crossref | Google Scholar |
Diller JL, Frazer TK, Jacoby CA (2014) Coping with the lionfish invasion: evidence that naïve, native predators can learn to help. Journal of Experimental Marine Biology and Ecology 455, 45-49.
| Crossref | Google Scholar |
Eurich JG, Selden RL, Warner RR (2014) California spiny lobster preference for urchins from kelp forests: implications for urchin barren persistence. Marine Ecology Progress Series 498, 217-225.
| Crossref | Google Scholar |
Filbee-Dexter K, Scheibling RE (2014) Sea urchin barrens as alternative stable states of collapsed kelp ecosystems. Marine Ecology Progress Series 495, 1-25.
| Crossref | Google Scholar |
Flukes EB, Johnson CR, Ling SD (2012) Forming sea urchin barrens from the inside out: an alternative pattern of overgrazing. Marine Ecology Progress Series 464, 179-194.
| Crossref | Google Scholar |
Frusher S, Buxton C, Barrett N, Tarbath D, Redd K, Semmens J, Pederson H, Valentine J, Guest M (2009) Towards integrated multi-species management of Australia’s SE reef fisheries: a Tasmanian example. Technical Report 2004/013 to the Fisheries Research and Development Corporation (Australia). (Tasmanian Aquacuture and Fisheries Institute, University of Tasmania) Available at https://www.imas.utas.edu.au/__data/assets/pdf_file/0007/743092/Towards-integrated-multi-species-management-of-Australias-SE-reef-fisheries-A-Tasmanian-example.pdf
Galloway AWE, Gravem SA, Kobelt JN, Heady WN, Okamoto DK, Sivitilli DM, Saccomanno VR, Hodin J, Whippo R (2023) Sunflower sea star predation on urchins can facilitate kelp forest recovery. Proceedings of the Royal Society of London – B. Biological Sciences 290(1993), 20221897.
| Crossref | Google Scholar |
Giraldo C, Ernande B, Cresson P, Kopp D, Cachera M, Travers-Trolet M, Lefebvre S (2017) Depth gradient in the resource use of a fish community from a semi-enclosed sea. Limnology and Oceanography 62(5), 2213-2226.
| Crossref | Google Scholar |
Guest MA, Frusher SD, Nichols PD, Johnson CR, Wheatley KE (2009) Trophic effects of fishing southern rock lobster Jasus edwardsii shown by combined fatty acid and stable isotope analyses. Marine Ecology Progress Series 388, 169-184.
| Crossref | Google Scholar |
Hawkey J (2008) Echinothrix diadema (long spined urchin). (University of Maryland Center for Environmental Science) Available at https://ian.umces.edu/media-library/echinothrix-diadema-long-spined-urchin/ [Verified 6 December 2021]
Johnson CR, Ling SD, Sanderson JC, Dominguez JGS, Frusher S, Gardner C, Hartmann K, Jarman S, Marzloff MP, Soulié C, Melbourne J (2013) Rebuilding ecosystem resilience: assessment of management options to minimise formation of ‘barrens’ habitat by the long-spined sea urchin (Centrostephanus rodgersii) in Tasmania. Technical report. Fisheries Research and Development Corporation, Australia.
King RA, Read DS, Traugott M, Symondson WOC (2008) Molecular analysis of predation: a review of best practice for DNA-based approaches. Molecular Ecology 17(4), 947-963.
| Crossref | Google Scholar |
Ling SD (2008) Range expansion of a habitat-modifying species leads to loss of taxonomic diversity: a new and impoverished reef state. Oecologia 156(4), 883-894.
| Crossref | Google Scholar |
Ling SD, Johnson CR (2009) Population dynamics of an ecologically important range-extender: kelp beds versus sea urchin barrens. Marine Ecology Progress Series 374, 113-125.
| Crossref | Google Scholar |
Ling SD, Johnson CR (2012) Marine reserves reduce risk of climate-driven phase shift by reinstating size- and habitat-specific trophic interactions. Ecological Applications 22(4), 1232-1245.
| Crossref | Google Scholar |
Ling SD, Keane JP (2018) Resurvey of the longspined sea urchin (Centrostephanus rodgersii) and associated barren reef in Tasmania. Technical report. (Institute for Marine and Antarctic Studies, University of Tasmania: Hobart, Tas., Australia) Available at https://www.imas.utas.edu.au/__data/assets/pdf_file/0005/1176026/129569-Resurvey-of-the-Longspined-Sea-Urchin-Centrostephanus-rodgersii-and-associated-barren-reef-in-Tasmania.pdf
Ling SD, Keane JP (2021) Decadal resurvey of long-term lobster experimental sites to inform Centrostephanus control. Final contracted report for the Abalone Industry Reinvestment Fund (AIRF Project 2019_08). (Institute for Marine and Antarctic Studies, University of Tasmania: Hobart, Tas., Australia) Available at https://www.imas.utas.edu.au/__data/assets/pdf_file/0008/1556522/Centro_lobster_exp_site_resurvey_final_report.pdf
Ling SD, Johnson CR, Frusher SD, Ridgway KR (2009) Overfishing reduces resilience of kelp beds to climate-driven catastrophic phase shift. Proceedings of the National Academy of Sciences 106(52), 22341-22345.
| Crossref | Google Scholar |
Martínez-Coello D, Lopeztegui-Castillo A, Puga-Millán R (2022) Spiny lobster Panulirus argus (Decapoda: Palinuridae) trophic spectrum update using diet descriptors and indices applied for first time in Cuba. Aquatic Ecology 56(4), 1019-1035.
| Crossref | Google Scholar |
Mazumder D, Williams RJ, Reid D, Saintilan N, Szymczak R (2008) Variability of stable isotope ratios of glassfish (Ambassis jacksoniensis) from mangrove/saltmarsh environments in southeast Australia and implications for choosing sample size. Environmental Bioindicators 3(2), 114-123.
| Crossref | Google Scholar |
Mori E, Malfatti L, Le Louarn M, Hernández–Brito D, Ten Cate B, Ricci M, Menchetti M (2020) ‘Some like it alien’: predation on invasive ring-necked parakeets by the long–eared owl in an urban area. Animal Biodiversity and Conservation 43, 151-158.
| Crossref | Google Scholar |
Mundy C, McAllister J (2022) Tasmanian abalone fishery assessment 2021. Technical report. (Institute for Marine and Antarctic Studies, University of Tasmania: Hobart, Tas., Australia) Available at https://www.imas.utas.edu.au/__data/assets/pdf_file/0004/1589017/Abalone-Fishery-Assessment-2021.pdf
Nielsen JM, Clare EL, Hayden B, Brett MT, Kratina P (2018) Diet tracing in ecology: method comparison and selection. Methods in Ecology and Evolution 9(2), 278-291.
| Crossref | Google Scholar |
Redd KS, Jarman SN, Frusher SD, Johnson CR (2008) A molecular approach to identify prey of the southern rock lobster. Bulletin of Entomological Research 98(3), 233-238.
| Crossref | Google Scholar |
Redd KS, Ling SD, Frusher SD, Jarman S, Johnson CR (2014) Using molecular prey detection to quantify rock lobster predation on barrens-forming sea urchins. Molecular Ecology 23(15), 3849-3869.
| Crossref | Google Scholar |
Santamaría J, Golo R, Verdura J, Tomas F, Ballesteros E, Alcoverro T, Arthur R, Cebrian E (2022) Learning takes time: biotic resistance by native herbivores increases through the invasion process. Ecology Letters 25(11), 2525-2539.
| Crossref | Google Scholar |
Santos AFGN, Santos LN, García-Berthou E, Hayashi C (2009) Could native predators help to control invasive fishes? Microcosm experiments with the Neotropical characid, Brycon orbignyanus. Ecology of Freshwater Fish 18(3), 491-499.
| Crossref | Google Scholar |
Sheppard-Brennand H, Dworjanyn SA, Poore AGB (2017) Global patterns in the effects of predator declines on sea urchins. Ecography 40(9), 1029-1039.
| Crossref | Google Scholar |
Skein L, Alexander ME, Robinson TB (2020) Co-occurring predators increase biotic resistance against an invasive prey. Marine Environmental Research 157, 104929.
| Crossref | Google Scholar |
Smith JE, Keane J, Mundy C, Gardner C, Oellermann M (2022) Spiny lobsters prefer native prey over range-extending invasive urchins. ICES Journal of Marine Science 79(4), 1353-1362.
| Crossref | Google Scholar |
Smith JE, Dietz C, Keane J, Oellermann M, Mundy C, Gardner C (2023) Trophic discrimination factors and stable isotope variability in a captive feeding trial of the southern rock lobster Jasus edwardsii (Hutton, 1875) (Decapoda: Palinuridae) in Tasmania, Australia. Journal of Crustacean Biology 43(3), ruad055.
| Crossref | Google Scholar |
Stock BC, Semmens BX (2022) MixSIAR GUI user manual. Version 3.1.12. Available at https://zenodo.org/records/47719 [Verified 30 November 2023]
Symondson WOC, Sunderland KD, Greenstone MH (2002) Can generalist predators be effective biocontrol agents? Annual Review of Entomology 47(1), 561-594.
| Crossref | Google Scholar |
Tegner MJ, Levin LA (1983) Spiny lobsters and sea urchins: analysis of a predator–prey interaction. Journal of Experimental Marine Biology and Ecology 73(2), 125-150.
| Crossref | Google Scholar |
Van Zyl RF, Mayfield S, Pulfrich A, Griffiths CL (1998) Predation by West Coast rock lobsters (Jasus lalandii) on two species of winkle (Oxystele sinensis and Turbo cidaris). South African Journal of Zoology 33(4), 203-209.
| Crossref | Google Scholar |
Wright ES (2016) Using DECIPHER v2.0 to analyze big biological sequence data in R. The R Journal 8(1), 352-359.
| Google Scholar |