Overexpression of HvVDE gene improved light protection in transgenic tobacco (Nicotiana tabacum)
Xiaojie Qu
A
B
C
Abstract
Hosta is commonly acknowledged as a popular and preferred plant for landscaping and gardening. The ‘sunburn’ caused by prolonged exposure to strong sunlight is reducing the ornamental values of Hosta plants. However, there is a scarcity of research focusing on the genetic components linked to light-induced harm in Hosta. Here, the violaxanthin de-epoxidase (VDE) homolog from Hosta ventricosa was isolated and functionally identified through conducting HvVDE-overexpression tobacco (Nicotiana tabacum) lines. The results showed that HvVDE encodes a putative protein comprising 481 amino acids with a molecular weight of 54.304 kDa. The phylogenetic analysis found that HvVDE exhibited close similarity to JcVDE. Besides, the expression patterns of HvVDE found that HvVDE was expressed differently across tissues, withexpression induced by high light intensities. And overexpression of HvVDE led to the restoration of non-photochemical quenching in tobacco, suggesting that HvVDE plays a role in dissipating excess light energy as thermal energy in H. ventricosa. These findings underscore the significance of HvVDE in mitigating photoinhibition and enhancing photoprotection mechanisms in H. ventricosa.
Keywords: ectopic expression, H. ventricosa, HvVDE, light stress, molecular breeding, non-photochemical quenching, photoprotection, xanthophyll cycle.
Introduction
Photosynthesis is a vital physiological process in plants that facilitates the transformation of light energy into chemical energy stored within the plant’s tissues. When faced with low light conditions, plants employ self-regulatory mechanisms to enhance the absorption and utilization of light energy (Bitterlich et al. 2019; Han et al. 2022). Nevertheless, an excessive amount of light can negatively impact plants (Hernández and Munné-Bosch 2015; Pinnola and Bassi 2018). For instance, prolonged exposure to high levels of light can lead to photoinhibition, a phenomenon that reduces the efficiency of photosynthesis and the maximum rate of photosynthetic activity. In severe cases, it can result in permanent photo-oxidative damage to the light absorption system, resulting in pigment fading or plant death. Plants have evolved various protective mechanisms over time to mitigate the potential damage caused by intense light exposure, thereby ensuring their optimal growth rates. These strategies encompass alterations in plant posture, activation of physiological and biochemical stress responses, and modulation of gene expression associated with light protection mechanisms, such as chloroplastic reactive oxygen species (ROS) scavenging, chloroplast and stomatal movement, and anthocyanin production, as well as thermal energy dissipation through non-photochemical quenching (NPQ), photorepair of Photosystem II (PSII), and transcriptional regulation of photosynthetic proteins (Nakamura and Izumi 2018, Levin et al. 2021; Shi et al. 2022). Through these adaptive capabilities, plants are able to successfully acclimate to a range of light intensities and fluctuations.
Among the adaptive strategies, plants have developed the xanthophyll cycle pathway as a primary mechanism to dissipate surplus light energy (García-Plazaola et al. 2003; Jahns and Holzwarth 2012; Lacour et al. 2020). The xanthophyll cycle is prevalent across various plant species, such as higher plants, ferns, and algae, predominantly taking place within the thylakoid membranes located in chloroplasts (Simkin et al. 2022). This cycle encompasses the compounds zeaxanthin, lutein, and violaxanthin, and entails the conversion of these substances in response to varying light intensities and pH levels (Lacour et al. 2020). In instances of heightened light stress, the reduction in pH within the thylakoid lumen stimulates the activity of violaxanthin de-epoxidase (VDE), facilitating the conversion of violaxanthin to antheraxanthin and zeaxanthin. Conversely, under low light or dark conditions, antheraxanthin and zeaxanthin are converted back to violaxanthin through the action of zeaxanthin epoxidase (ZEP), thereby bolstering the plant’s photosynthetic efficiency. Therefore, VDE is crucial in the xanthophyll cycle as it regulates how plants adjust their physiological processes under light.
Numerous research investigations have documented the significant involvement of the VDE gene in the regulation of plants’ response to photodamage. For example, increasing VDE expression in mutant eto1-1 and ctr1-3 could restore light-activated de-epoxidation and energy dependent non-photochemical quenching, and reduce superoxide production and photoinhibition in Arabidopsis (Chen and Gallie 2015; Guan et al. 2015). Guan et al. have cloned a VDE gene (LcVDE) from Lycium chinense, which may cooperate with the abscisic acid (ABA) signaling pathway to modulate the level of photosynthesis damage caused by drought stress (Guan et al. 2015).
The genus Hosta is widely recognized as a prominent and favored plant for landscaping and gardening in the northern temperate region. Belonging to the Asparagaceae family, Hosta are native to Northeast Asia, particularly Korea, Japan, China, and Russia, with Japan being identified as the primary hub of species diversity (Yoo et al. 2021). The genus Hosta has been extensively studied in horticulture, resulting in the development of approximately 6300 cultivars through rigorous hybridization and selection processes (Yang et al. 2021). In urban settings, Hosta are commonly utilized as ground cover and ornamental greenery in flower beds. However, during the cultivation of Hosta for urban greening in northern Chinese cities, issues such as sunburn may arise due to direct exposure to intense sunlight. This can lead to undesirable effects on the leaves, including thinning, scorching at the edges, curling, and a shift in color from green to yellowish white, consequently diminishing the aesthetic appeal of Hosta plants.
Previous studies on light-induced damage in Hosta have primarily focused on assessing physiological parameters such as soluble sugars, antioxidant enzymes, and photosynthetic traits. However, there is a limited amount of research investigating the genetic factors associated with light-induced damage to Hosta. Here, the isolation and characterization of the VDE homolog from H. ventricosa were conducted, and the HvVDE-overexpressed tobacco (Nicotiana tabacum) was obtained. By determining the photosynthesis of genetically modified lines, it was found that the VDE gene plays a role in stimulating the xanthophyll cycle, thereby improving the photoprotection. This investigation establishes a foundation for further exploration into the molecular pathways involved in light-induced damage in H. ventricosa and for the application of molecular breeding techniques.
Materials and methods
Plant materials and growth conditions
Plantlets of Hosta ventricosa Stearn were planted at Greenhouse A7 of Jilin Agricultural University Experimental Garden, in Jilin province, China. The cultivation conditions were an average temperature of 20.2°C, full sunlight, 60% humidity, and a photoperiod of 12/12 h, and the maximum light intensity of natural daylight was 3500 μmol m−2 s−1. When the H. ventricosa seedlings were 6 months old, we collected healthy root, stem, and leaf tissues, which were rapidly frozen in liquid nitrogen for subsequent experiments. Each tissue sample was sourced from at least three biological replicates. In additional, we conducted shading experiments using the aforementioned plants, which were randomly allocated into four groups, each consisting of 10 pots. Black nylon nets were employed to established shaded treatment, resulting in light transmission rates of 15% (ZQY), 30% (ZBG), and 50% (QGZ) in comparison to the non-shaded control group (CK), while maintaining consistent conditions for other growth parameters. The measured transmittance levels were determined using a ZDS-10 illuminance meter. Transmitance levels for the QGZ, ZBG, ZQY treatment groups were 55.38%, 34.12% and 16.41%. The maximum light intensities of shaded treatment groups were 1200 ± 10 μmol m−2 s−1 (QGZ), 600 ± 10 μmol m−2 s−1 (ZBG), and 100 ± 10 μmol m−2 s−1 (ZQY), respectively. Following a treatment duration of 7 days, leaf samples were collected from treatment groups and control groups, rapidly frozen using liquid nitrogen and stored at −80°C for further analysis.
The wild-type (WT) Nicotiana tabacum (L.) ‘NC89’ seedlings and transgenic tobacco lines were grown in a growth chambers maintained under controlled conditions. These conditions included a temperature of 25 ± 2°C, a humidity level of 70 ± 5%, and white light calibrated to mimic sunlight, with a light intensity of 100 ± 10 μmol m−2 s−1, and a photoperiod of 12 h of light and 12 h of darkness.
Cloning and bioinformation analysis of HvVDE
Total RNA was isolated utilizing the RNAprep Pure Extraction Kit (DP441, TIANGEN biochemical Technology, Beijing, China) and assessed for quality and integrity through 1.0% agarose gel electrophoresis. Subsequently, the initial complementary DNA (cDNA) strand was synthesized using the PrimeScript RT reagent Kit with gDNA (RR047, TaKaRa, Tokyo, Japan) following the manufacturer’s protocol. Specific primers were designed based on sequences derived from transcriptome data (Supplementary Table S1) using Permier Primer 6.0 software. The HvVDE gene was then amplified from the cDNA using TaKaRa Taq™ (R001A, TaKaRa, Tokyo, Japan) under the following cycling conditions: initial denaturation at 94°C for 3 min, followed by 33 cycles of denaturation at 94°C for 30 s, annealing at 58°C for 30 s, extension at 72°C for 30 s, and a final extension at 72°C for 10 min. Polymerase chain reaction (PCR) fragments were purified using a Gel Extraction Kit (CW2302 CWBIO, JiangSu, China), cloned into a pMD™ 18-T Vector Cloning Kit (6011 Takara, Tokyo, Japan), and sequenced by GENE Biotechnology company (Beijing, China). The conserved domain of the target gene was predicted using the NCBI Conserved Domain Database (https://www.ncbi.nlm.nih.gov/structure/cdd/wepsb.cgi). Alignment analysis of VDEs was conducted with DNAMAN, and a phylogenetic tree was constructed using MEGA 7.0 through the Neighbor-Joining method with 1000 bootstrap replicates.
Quantitative PCR (qPCR) analysis
The initial cDNA strand for qPCR was generated using the PrimeScript RT reagent Kit with gDNA Eraser (RR047, TaKaRa, Japan). The amplification process was conducted on a BioRad IQ5 instrument (Biorad, USA) utilizing the SYBR Prime Ex Tap II (RR420, TaKaRa, Japan) in accordance with the manufacturer’s instructions. The PCR protocol involved an initial denaturation step at 95°C for 30 s; followed by cycles of denaturation at 98°C for 5 s, annealing at 60°C for 34 s, for a total of 40 cycles; and a final extension step at 95°C for 15 s, annealing at 60°C for 60 s, and a final denaturation at 95°C for 15 s. The expression levels of HvVDE in various tissues and treatment groups of leaves were quantified using HvActin as the reference gene (Zhang et al. 2020).
The qPCR technique was utilized to identify the principal genes associated with photosynthesis and the xanthophyll cycle in tobacco. The experimental setup and procedures mirrored those described previously, with the primer sequences detailed in Table S1. The relative expression levels of the identified genes were assessed in comparison to a reference gene in the control group using the 2−ΔΔCT method (Luo et al. 2020).
Construction of overexpression vector and Agrobacterium-mediated transformation
The fragment of HvVDE gene was introduced the Kpn I and BamH I restriction enzyme sites and was inserted into a pCAMBIA1300 vector to create the recombined vector pCAMBIA1300- HvVDE. Subsequently, the recombinant vector was transformed into the Agrobacterium EHA105 strain (Hwang et al. 2015), and preformed PCR to confirm the successful transformants. Then, the transformation of tobacco was performed using the leaf-plate method as previously described (Okuzaki and Tabei 2012). Transgenic lines displaying the highest expression levels of the HvVDE gene were identified through qPCR analysis and selected for further investigations. The growth status was observed and compared with the WT tobacco plants.
The subcellular localization of HvVDE
The full-length cDNA of HvVDE without stop codon were amplified and inserted into the BamH I site of the vector 16318hGFP. The fusion 16318hGFP-HvVDE vector was constructed and transformed into Arabidopsis protoplast. The empty 16318hGFP vector was also transformed as a control. Following the protocol described by Abel and Theologis (1994), 20-day-old leaves from Arabidopsis thaliana seedlings were chosen for the protoplast transformation procedure. The supernatant was subjected to enzymatic digestion, followed by treatment with W5 and MMG solutions to yield a protoplast suspension. Subsequently, the recombinant plasmid 16318h-GFP-HvVDE was introduced and incubated in darkness for a period of 2 days. The visualization of fluorescence signals within the protoplasts was achieved through examination using a laser confocal scanning microscope (Nikon C2-ER, Japan).
Determination of fluorescence characteristic parameters of transgenic tobacco
In a previous study conducted by Li et al. (2015), a portable chlorophyll fluorometer (FMS-2, UK) was utilized to assess the fluorescence parameters of healthy leaves of transgenic tobacco T1 lines under controlled light conditions, with consistent leaf positioning. Prior to the measurements, the leaves underwent a dark treatment for 15 min, followed by exposure to a low light intensity of 1 μmol m−2 s−1 to determine the initial fluorescence (Fo). Subsequently, a saturated pulsed light intensity of 1200 μmol m−2 s−1 was employed to measure maximum fluorescence (Fm), variable fluorescence (Fv = Fm – Fo), and maximum photochemical efficiency of Photosystem II (PSII) (Fv/Fm). Three leaves were selected for each experimental treatment.
To further analyze the components of NPQ, we employed a typical quenching analysis followed by relaxation analysis, applied to the dark-adapted, young and fully developed leaves from WT and overexpression (OE) lines using actinic light of 1200 μmol m−2 s−1. The time-course of NPQ in 25 min dark-adapted leaves was determined. was defined as the maximal fluorescence during the illumination and was defined as the maximal fluorescence during dark recovery after previous illumination, .
Statistical analyses
Three distinct plants were utilized as individual replicates for each treatment. The data underwent a two-way analysis of variance (ANOVA), followed by the application of the Tukey test as a post hoc analysis. A significance level of P < 0.05 was established. Statistical analyses were conducted using GraphPad Prism 9.0 (GraphPad Software, Inc., San Diego, CA, USA).
Results
Isolation and characteristics of HvVDE
In order to investigate the gene function of HvVDE, gene-specific primers were designed based on contig sequences retrieved from a previous transcriptome database (Zhang et al. 2020). The open reading frame (ORF) sequence of HvVDE was successfully obtained through PCR amplification (Fig. 1a). A comparative analysis of the nucleotide sequence of the cloned gene with known homologous sequences of phytoene desaturase in different species was conducted using the NCBI database. The ORF with the length of 1446 bp, encoded a putative protein consisting of 481 amino acids with a molecular weight of 54.304 kDa and an isoelectric point (pI) of 5.19. The amino acid sequence of HvVDE exhibited the highest content of glutamate (Glu) and leucine (Leu) at 8.5%, followed by serine (Ser) at 7.7% and threonine (Thr) at 6.4%, but lacked pyrrolysine (Pyl) and selenocysteine (Sec). HvVDE was characterized as an unstable soluble protein with a hydrophobic index of −0.319 and a stability index of 47.25. Notably, HvVDE was found to lack a signal peptide, indicating its classification as a non-secretory protein.
Amino acid alignment and phylogenetic analysis of HvVDE and homologous proteins. (a) The PCR product of HvVDE. (b) The prediction of HvVDE protein structure. (c) Alignment of amino acid sequences of VDEs in different plants. Red lines indicate the conserved domain of the VDE protein. The pink and blue color means >75% and >50% homology among those sequences, respectively. (d) Phylogenetic tree of HvVDE constructed with MEGA 11.0, using the neighbor-joining (NJ) method and 1000 bootstrap replicates.
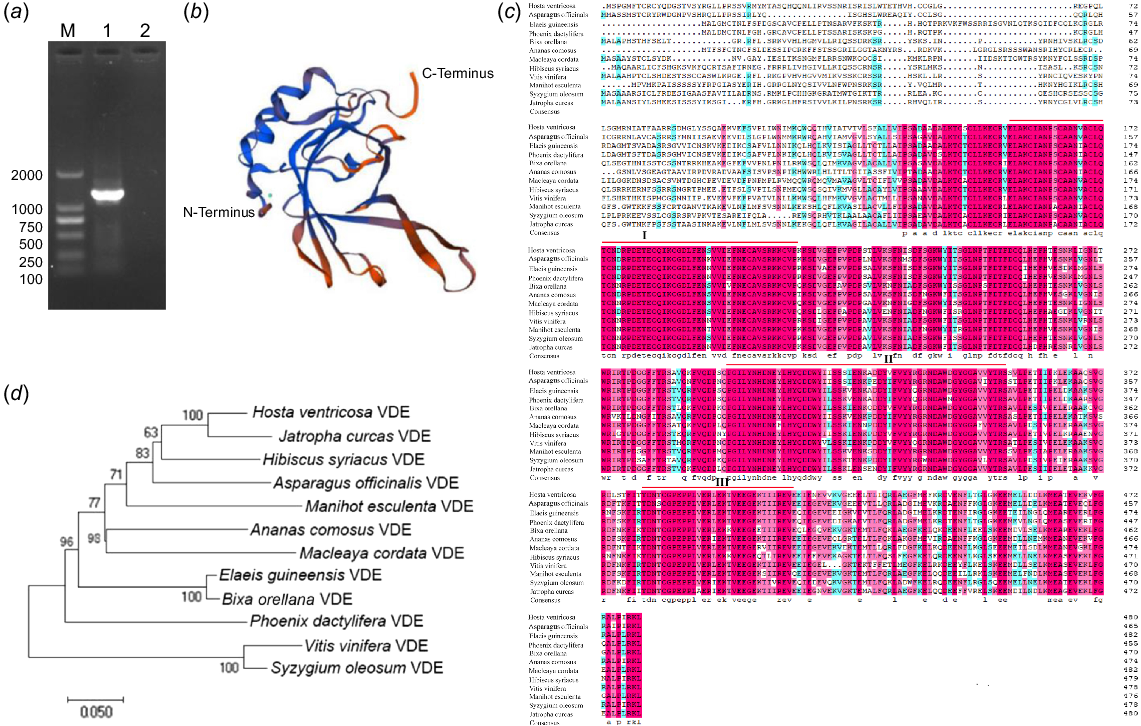
Moreover, the HvVDE protein was found to consist of 43.04% alpha helix, 16.63% extended strand, 6.65% beta-turn, and 33.68% random coils, and the protein structure of HvVDE is shown in Fig. 1b. Furthermore, examination of the conserved domain in the NCBI database revealed that HvVDE contains a PLN02372 conserved domain, which is categorized as a member of the violaxanthin de-epoxidase group within the lipid carrier protein superfamily.
Multi-alignment analysis and phylogenetic tree conduction
As shown in Fig.1c, multiple sequence alignment revealed that HvVDE predicted protein was highly similar to violaxanthin de-epoxidase proteins of other species (69.46–88.74%). Three regions with high homology for VDE were identified: a cysteine-rich region (I, E162-F201), a lipid transport protein signal structural region (II, P299-V360), and a high-charged glutamic acid-rich region (III, T380-V415).
Furthermore, a phylogenetic analysis was conducted to explore the evolutionary relationship of HvVDE with other VDEs found in H. ventricosa and 11 additional plant species. Through a comparison of amino acid sequences, it was observed that HvVDE forms a closely related clade with JcVDE (XP_012092715.1) (Fig. 1d). This conservation of homology within the plant family highlights the crucial functional role of VDE.
Subcellular localization prediction analysis
To investigate the intracellular localization of the VDE protein, an analysis of the signal peptide of the HvVDE protein was conducted using SignalP 5.0. The results revealed the absence of a signal peptide sequence within the coding region of the HvVDE protein. Subsequent examination utilizing target P 2.0 software suggested a potential localization of this protein within the chloroplast.
To further validate the cellular localization of VDE, we generated HvVDE fusion constructs controlled by the CaMV35S promoter which were then expressed in Arabidopsis protoplasts. The results demonstrated that in protoplasts transfected with the empty control vector, the fluorescence signal was observed in both the nucleus and chloroplasts. Conversely, in protoplasts transfected with the recombinant vector, the fluorescence signal was exclusively localized in the chloroplasts. These results indicate that the protein encoded by the HvVDE gene is indeed situated within the chloroplasts, aligning with the anticipated localization (Fig. 2).
Expression pattern of HvVDE
To study the expression profiles of HvVDE gene, qPCR analysis of HvVDE in tissues was performed. The HvVDE was modestly expressed in root and stem, but it expressed highly in leaf tissue (Fig. 3a). Furthermore, we detected the expression level of HvVDE under shading treatment groups. It is shown that the expression of the HvVDE gene was the highest in the QGZ group without shade, and the lowest in the ZQY group. This finding indicated that the expression of HvVDE gene is influenced by varying levels of light intensity.
The HvVDE transcript abundance in tissues and under the (a) light treatments and (b) light intensities determined by qPCR. Error bar, s.d. Lowecase letters represent significant differences between samples.
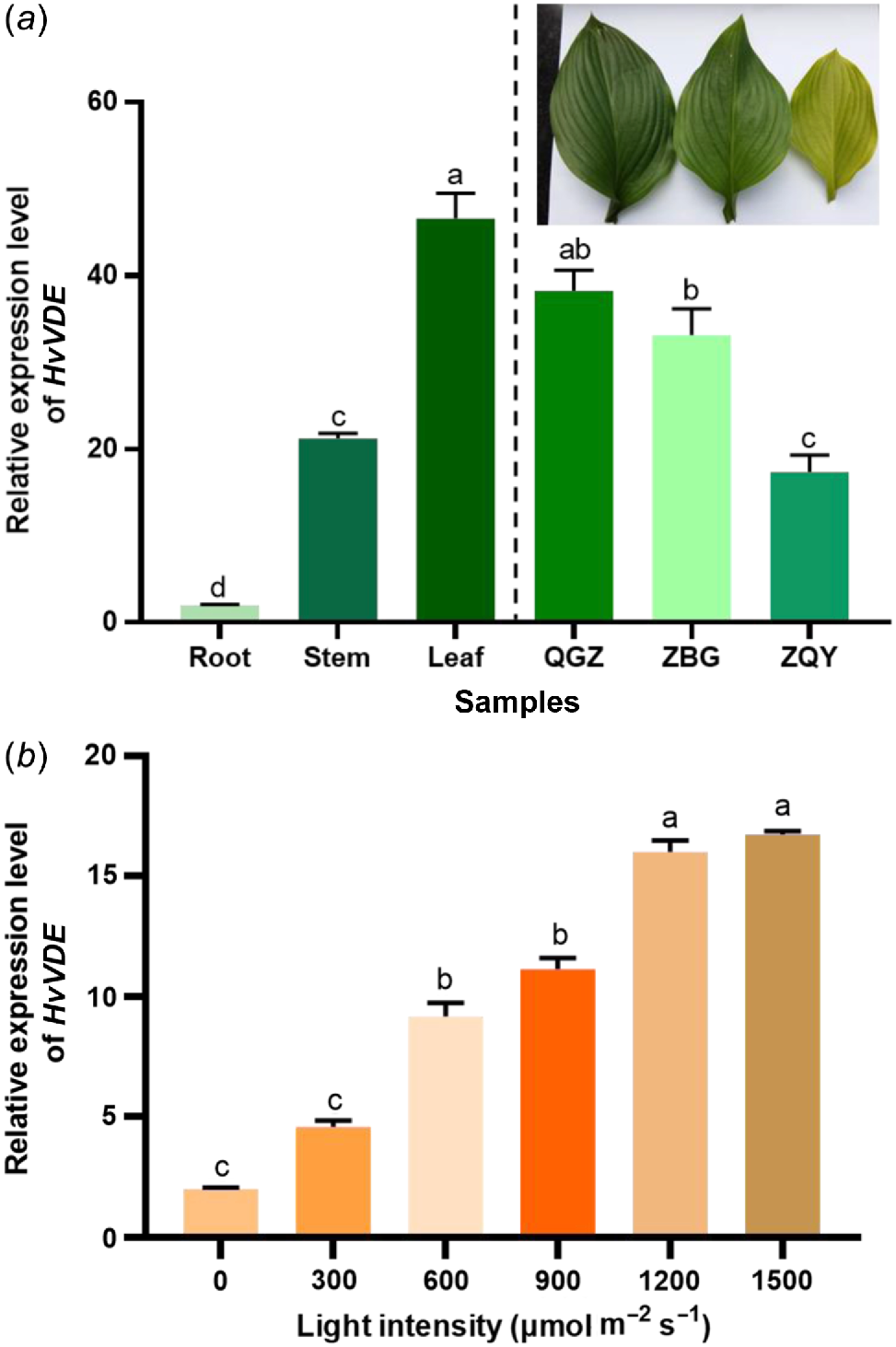
Besides, we further investigated the level of HvVDE transcription in H. ventricosa leaves exposed to different light intensities range from 300 to 1500 μmol m−2 s−1 for 2 h (Fig. 3b). The results showed that the level of HvVDE expression gradually increased with increasing light intensity, reaching the highest level at a light intensity of 1500 μmol m−2 s−1, which was 3.65 times higher than in complete darkness (0 μmol m−2 s−1).
Overexpression of HvVDE transgenic tobacco lines were generated by Agrobacterium-mediated transformation
Firstly, the Kpn I and BamH I were introduced to the open reading frame of HvVDE, and the recombined vector pCAMBIA1300-HvVDE-FLAG under the 35S promoter was conducted (Fig. 4a). Then, the freeze–thaw method was employed to transfer the recombined plasmid pCAMBIA1300-HvVDE to A. tumefaciens EHA105 and the positive transformants were confirmed through HvVDE specific PCR analysis (Fig. 4b). Through co-cultivation, the transgenic plantlets were regenerated, then the plantlets were screened on MS (Murashige and Skoog) medium plates supplemented with hygromycin (10 mg mL−1). Resistant shoots were developed from the explants and transgenic plants of the T0 generation were obtained (Fig. 4d). The presence of HvVDE gene was validated through genomic DNA (gDNA) PCR using FLAG-Chek-F/R primers on leaves of T0 generation, where a 1400 bp band indicated successful introduction of HvVDE in transgenic tobacco (Fig. 4c). Plants transformed with an empty vector pCAMBIA1300 were used as controls. A total of 19 positive lines after Agrobacterium tumefaciens-mediated genetic transformation. The T0 tobacco seeds were germinated and cultivated on MS medium contained hygromycin (20 mg mL−1) to obtain 15 genetically transgenic T1 tobacco lines harboring the HvVDE gene.
The construction of the overexpression vector and genetic transformation procedure. (a) The schematic of the HvVDE gene overexpression cassette. (b) Agarose gel showing the specific fragment for the HvVDE in recombinat plasmides; M, DL 2000 DNA marker; 1–4, the recombinat plasmide; 5, empty vector control. (c) The specific fragment for the HvVDE in transgenic tobacco line. (d) The process of Agrobacterium-mediated transformation in tobacco. (e) The relative expression level of HvVDE in the transgenic tobacco lines. Asterisks indicate that there is a significant difference between OE lines and WT; * P ≤ 0.05, ** P ≤ 0.01. Error bar, s.d.
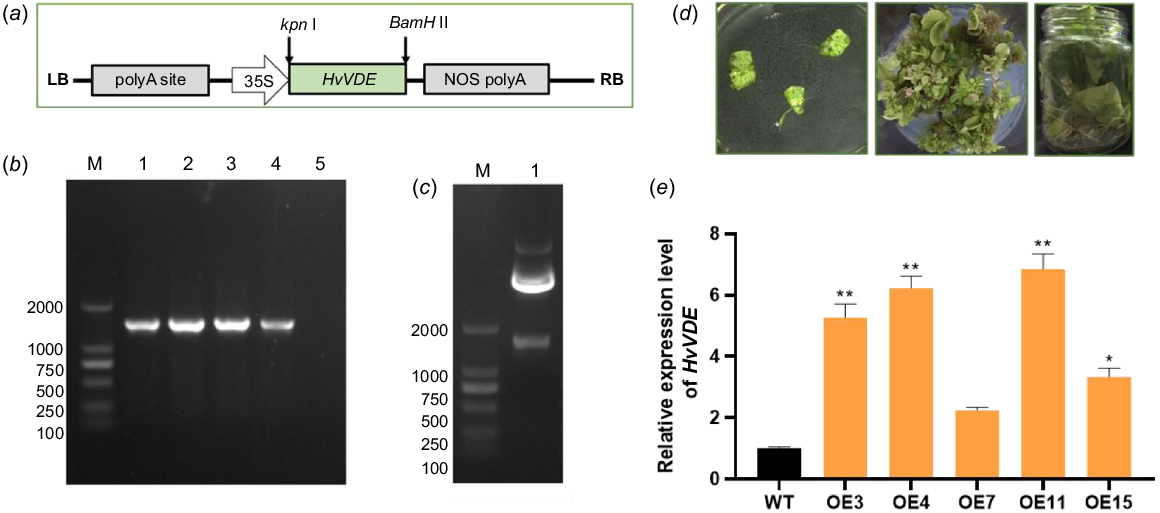
qPCR was utilized to detect the expression of the HvVDE gene. The results showed that OE3, OE4, OE7, OE11, and OE15 exhibited high levels of HvVDE gene expression (Fig. 4e). Among these, OE3, OE4, and OE11 were selected for additional analysis due to their high expression levels. After selection we obtained T1 generation seeds of transgenic tobacco lines. Then, the T1 seeds of OE3, OE4, OE11, and WT tobacco were sown and grown under identical conditions. The results indicated no statistically significant differences in seed germination rates or plant height of the seedlings (Table S2). Furthermore, there were no observable differences in plant growth morphology or leaf color.
Photosynthesis determined in overexpression of HvVDE in tobacco
One of the most rapid and effective methods of protection involves the conversion of surplus absorbed light energy into heat energy. This can be accomplished by monitoring NPQ of chlorophyll fluorescence. To assess the impact of HvVDE heterologous expression on tobacco NPQ, we examined alterations in NPQ levels in transgenic tobacco and wild-type lines exposed to a light intensity of 1200 μmol m−2 s−1 (Fig. 5a). The findings indicate a progressive rise in non-photochemical quenching (NPQ) levels in both genetically modified tobacco and wild-type tobacco, with a notable surge observed within a 2-h timeframe. Throughout the duration of the treatment, the NPQ levels in genetically modified tobacco consistently exceeded those in wild-type tobacco. The finding suggests that under conditions of heightened light stress, genetically modified tobacco plants demonstrate a heightened capacity for energy dissipation through the xanthophyll cycle compared to their wild-type counterparts. The parameter Fv/Fm denotes the peak photochemical efficiency of the Photosystem II (PSII) reaction center in plants under optimal conditions, serving as an indicator of photoinhibition in the plant. Therefore, we measured the Fv/Fm of these genotypes and it showed that there is a notable decline in the maximum photochemical efficiency in conditions of intense light stress. The transgenic tobacco plants exhibit a greater Fv/Fm ratio in comparison to their wild-type counterparts (Fig. 5b).
(a) Non-photochemical quenching (NPQ) and (b) Fv/Fm levels in transgenic tobacco and WT. Asterisks indicate that there is a significant difference between OE lines with WT at test point; **P ≤ 0.01. Error bar, s.d.
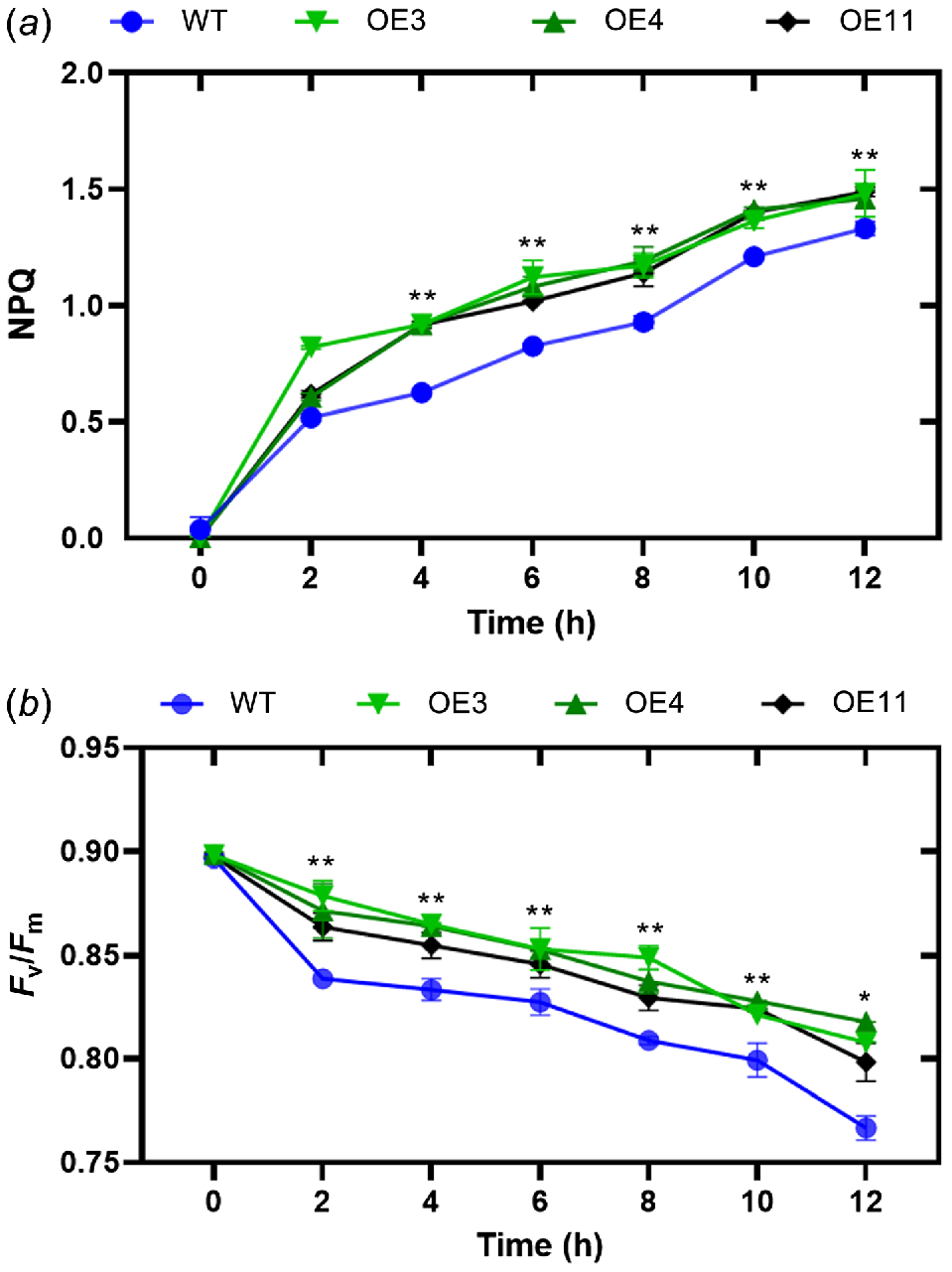
The identification of the various components of NPQ was facilitated by their distinct relaxation times during a dark period following exposure to high light intensity. Fig. 6a illustrates the time-course of NPQ at light density of 1200 μmol m−2 s−1. Fig. 6b, c present the dark relaxation kinetics derived from Fig. 6a, specifically highlighting the rapid (qE) and slow relaxing (qI) components of NPQ. A substantial portion of NPQ was attributed to qE. In comparison to WT, OE lines exhibited a markedly higher qE and a lower qI.
NPQ relaxation analysis and calculation of energy quenching (qE) and photoinhibitory quenching (qI). (a) Time-course of NPQ in 25 min dark-adapted leaf. Black bar represents dark, white bar represents actinic light of 1200 μmol m−2 s−1. (b) qE and (c) qI were calculated. Vertical bars represent s.d. (n = 6). Asterisks denote significant differences between light treatments in WT and OE transgenic lines (**P < 0.01).
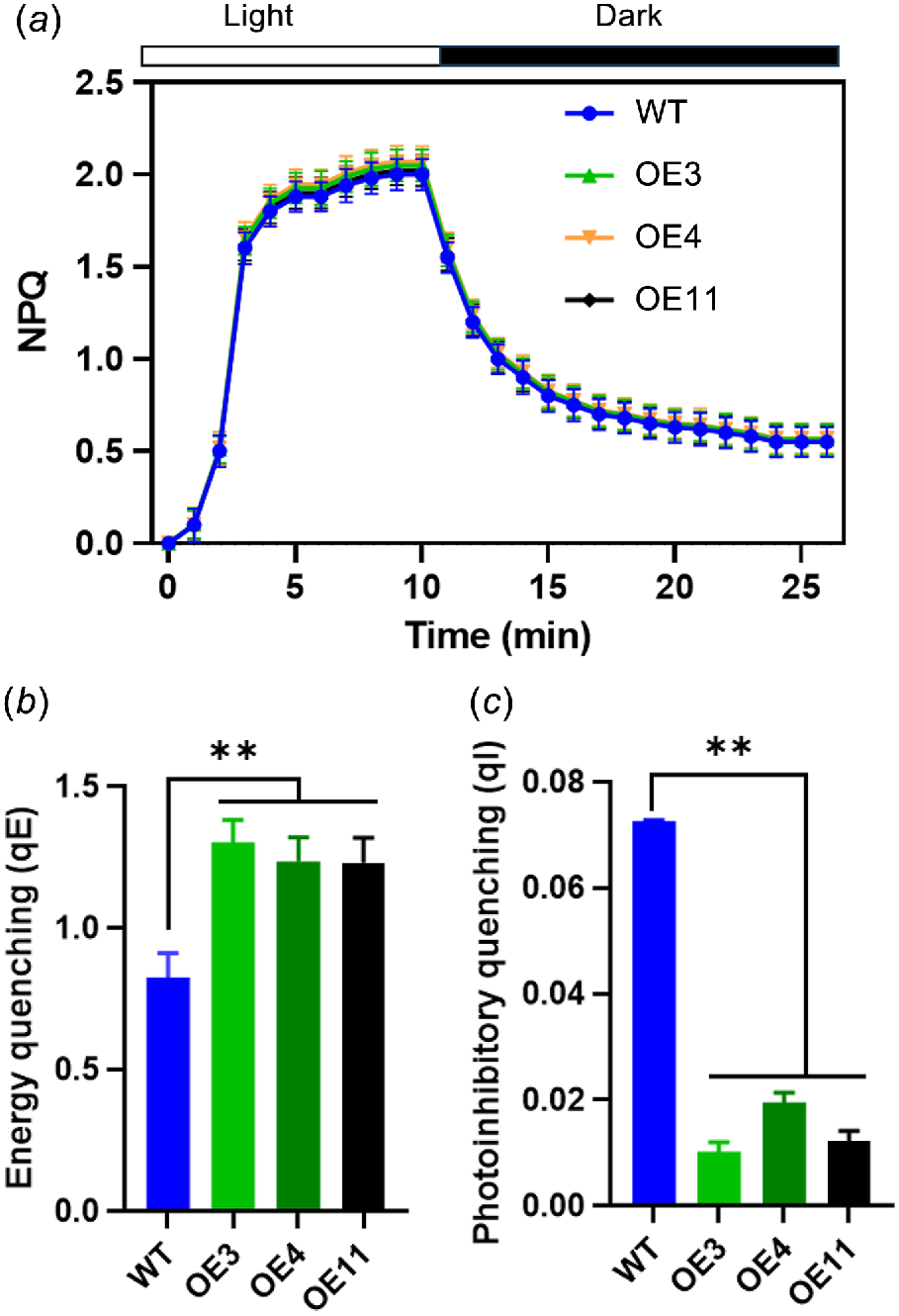
Discussion
Under conditions of intense illumination, the light energy that plant leaves can effectively utilize constitutes only a minor fraction of the total light energy absorbed by the photosynthetic structures. Failure to dissipate excess excitation energy promptly may result in photoinhibition and potential harm to the photosynthetic machinery (Badger et al. 2009). The detrimental effects of photodamage can be exacerbated in the presence of additional environmental stresses (Gerganova et al. 2019; Zia et al. 2024). Throughout the extensive course of evolution, plants have developed a comprehensive array of mechanisms to mitigate excess light energy, one of which is the xanthophyll cycle. This cycle plays a crucial role in safeguarding plants from damage induced by high light intensity, with the VDE gene being particularly significant in this protective process. H. ventricosa known for its preference for shaded environments, and is susceptible to photodamage under intense light conditions, although the underlying molecular mechanisms remain uncertain. This investigation involved the cloning of the VDE gene from H. ventricosa, with subsequent analysis of its conserved domains, evolutionary lineage, and expression profiles. Through the overexpression of VDE in tobacco plants, researchers observed a restoration of non-photochemical quenching (NPQ) and an augmented capacity for photoprotection. Our findings suggest that HvVDE is involved in the conversion of surplus light energy into heat within H. ventricosa. The multiple sequence alignment results show that the central and C-terminal regions of the HvVDE protein contain the VDE superfamily domain, a cysteine-rich domain, a lipocalin domain, and a glutamic acid-rich domain, indicating a high similarity with VDE proteins from other species (Emanuelsson et al. 2003; Arnoux et al. 2009), suggesting that these regions are highly conserved (Fig. 1). Notably, the lipocalin domain is thought to possess the capability to interact with zeaxanthin molecules in an all-trans configuration, thereby serving as a crucial functional component of the VDE protein. Furthermore, in order to investigate the genetic association between HvVDE and VDEs found in various species, a phylogenetic tree was constructed. Our data indicates that the HvVDE is closely related to the JrVDE, creating a distinct lineage, while having a distant genetic relationship with Vitis vinifera and Syzygium oleosum, aligning with findings from prior phylogenetic investigations. Consistent with prior findings on AtVDE, LeVDE, and GhVDE (Huang et al. 2007; Han et al. 2010; Zhao et al. 2012), the expression levels of these genes were notably higher in photosynthetic tissues, particularly in leaves (Fig. 3). The expression of VDE showed a significant increase in response to intense light exposure, aligning with previous research.
In the natural environment, exposure to high light intensity is a common stressor that can lead to photoinhibition of Photosystem II (PSII) and harm to the photosynthetic machinery due to excessive light. Plants have evolved diverse strategies to safeguard themselves against light-induced damage and photoinhibition. These protective mechanisms include leaf and chloroplast movement to avoid light, light screening, systems for removing reactive oxygen species (ROS), distribution of heat energy, cyclic electron flow around Photosystem I (PSI), and photorespiratory pathways. One of the mechanisms that has received significant attention in research is the dispersion of heat energy, which is frequently studied through the quantitative evaluation of non-photochemical quenching (NPQ) of chlorophyll fluorescence. Hence, in investigations of the xanthophyll cycle in photosynthesis, NPQ is frequently employed as a diagnostic marker to evaluate the efficacy of plant photoprotection following alterations in the expression of the violaxanthin de-epoxidase (VDE) gene. An illustration of this is the exploration of PeVDE’s role in bamboo within the Arabidopsis VDE mutant npq1, which demonstrated PeVDE’s capacity to reinstate the NPQ ability of the Arabidopsis mutant and augment its photoprotective functions (Lou et al. 2022). Besides, Sun (Sun et al. 2019) found that overexpressing VDE plants displayed improved root growth, elevated chlorophyll levels, and the highest capacity for xanthophyll cycle de-epoxidation (A + Z)/(V + A + Z) through the creation of VDE gene mutant strains. Following exposure to drought or salt stress, OE plants exhibited increased rates of photosynthesis, respiration, and transpiration compared to wild-type plants and npq1–2 mutants, suggesting enhanced resistance to stress.
As a result of the absence of a reliable genetic transformation mechanism in Hosta, the majority of investigations into gene functionality are carried out using model plant species (Küster et al. 2023). Therefore, we had to perform the functional analysis of HvVDE in tobacco (Fig. 4). When exposed to high light stress, both wild tobacco and transgenic tobacco exhibit an increasing NPQ trend over time, with the transgenic tobacco demonstrating higher NPQ levels compared to the wild tobacco (Fig. 5). This suggests that HvVDE transgenic tobacco possesses an enhanced ability to protect the photosynthetic apparatus from photodamage. The parameter Fv/Fm denotes the maximal photochemical efficiency of Photosystem II (PSII) when all reaction centers are in a closed state, providing a measure of photoinhibition in photosynthesis. Following exposure to high light conditions, Fv/Fm displays a declining pattern, indicating that the overexpression of HvVDE helps alleviate PSII photoinhibition under high light stress (Chen and Gallie 2012; Guan et al. 2015). These findings align with previous research on transgenic tomatoes, transgenic tobacco, and transgenic Arabidopsis under high light stress, which have shown similar alterations in NPQ and Fv/Fm values. Fv/Fm, as a metric, represents the peak photochemical efficiency of PSII when all reaction centers are fully active and can be utilized to assess the extent of photoinhibition in plants.
In high light illumination, qT quenching is expected to be suppressed. It is well known that qE is associated with the conversion of violaxanthin to zeaxanthin via the intermediate antheraxanthin, by the catalyst violaxanthin de-epoxidase (García-Plazaola et al. 2007) and the protonation of the PsbS protein under high light conditions. This was partly supported by our results whereby the induction of NPQ was determined by the expression of HvVDE under high light intensity. Previous studies have indicated that increased VDE expression enhanced the rate of NPQ induction under subsaturating light (Chen and Gallie 2012), which is consistent with our results (Fig. 5). The patterns observed in qE and NPQ exhibit a comparable trajectory, both rising rapidly under strong light, with qE serving as the primary constituent of NPQ (Fig. 6). In the OE lines, both NPQ and qE levels were elevated in comparison to the WT during light induction, which enhanced the contribution of xanthophyll cycle-dependent thermal dissipation to overall energy dissipation. This may be associated with the high expression of VDE in transgenic tobacco.
Conclusions
This study reports the identification of a conserved gene in H. ventricosa, which encodes a putative protein comprising 481 amino acids with a molecular weight of 54.304 kDa, responsible for encoding violaxanthin de-epoxidase (VDE). Through phylogenetic analysis, it was observed that HvVDE exhibited close similarity to JcVDE (Jatropha curcas). Analysis of HvVDE expression patterns across different tissues and light intensities revealed a high expression level in leaves, particularly under conditions of high light intensity. Subsequently, a recombinant vector was constructed and HvVDE was overexpressed in tobacco plants to elucidate the gene’s function. The results demonstrated that overexpression of HvVDE led to the restoration of NPQ in tobacco, suggesting that HvVDE plays a role in dissipating excess light energy as thermal energy in H. ventricosa. These findings underscore the significance of HvVDE in mitigating photoinhibition and enhancing photoprotection mechanisms in H. ventricosa. Nevertheless, further investigations are warranted to confirm the specific function of HvVDE in H. ventricosa. This study provides a new insight into the mechanism of light protection and for germplasm innovation and further breeding in H. ventricosa.
Data availability
The data that support this study will be shared upon reasonable request to the corresponding author.
Declaration of funding
This research was funded by Wuzhou University research project (2023A1004) and Achievements transformation project of the Ministry of Science and Technology (No. 2014GB2B100007).
Author contributions
Xiaojie Qu designed and performed most of the experiments and written the manuscript, Fangting Qin performed a part of qPCR, Gaung Huang constructed the vectors, Deyu Che contributed to photosynthesis index analysis, Hongzhang Liu modified the manuscript.
Acknowledgement
This research was funded by Wuzhou University research project (2023A1004) and Achievements transformation project of the Ministry of Science and Technology (No. 2014GB2B100007).
References
Abel S, Theologis A (1994) Transient transformation of Arabidopsis leaf protoplasts: a versatile experimental system to study gene expression. The Plant Journal 5(3), 421-427.
| Crossref | Google Scholar | PubMed |
Arnoux P, Morosinotto T, Saga G, Bassi R, Pignol D (2009) A structural basis for the pH-dependent xanthophyll cycle in Arabidopsis thaliana. The Plant Cell 21(7), 2036-2044.
| Crossref | Google Scholar | PubMed |
Badger MR, Fallahi H, Kaines S, Takahashi S (2009) Chlorophyll fluorescence screening of Arabidopsis thaliana for CO2 sensitive photorespiration and photoinhibition mutants. Functional Plant Biology 36(11), 867-873.
| Crossref | Google Scholar |
Bitterlich M, Franken P, Graefe J (2019) Atmospheric drought and low light impede mycorrhizal effects on leaf photosynthesis – a glasshouse study on tomato under naturally fluctuating environmental conditions. Mycorrhiza 29, 13-28.
| Crossref | Google Scholar | PubMed |
Chen Z, Gallie DR (2012) Violaxanthin de-epoxidase is rate-limiting for non-photochemical quenching under subsaturating light or during chilling in Arabidopsis. Plant Physiology and Biochemistry 58, 66-82.
| Crossref | Google Scholar | PubMed |
Chen Z, Gallie DR (2015) Ethylene regulates energy-dependent non-photochemical quenching in Arabidopsis through repression of the xanthophyll cycle. PLoS ONE 10(12), e0144209.
| Crossref | Google Scholar | PubMed |
Emanuelsson A, Eskling M, Åkerlund H-E (2003) Chemical and mutational modification of histidines in violaxanthin de-epoxidase from Spinacia oleracea. Physiologia Plantarum 119(1), 97-104.
| Crossref | Google Scholar |
García-Plazaola JI, Hernández A, Olano JM, Becerril JM (2003) The operation of the lutein epoxide cycle correlates with energy dissipation. Functional Plant Biology 30(3), 319-324.
| Crossref | Google Scholar | PubMed |
García-Plazaola JI, Matsubara S, Osmond CB (2007) The lutein epoxide cycle in higher plants: its relationships to other xanthophyll cycles and possible functions. Functional Plant Biology 34(9), 759-773.
| Crossref | Google Scholar | PubMed |
Gerganova MT, Faik AK, Velitchkova MY (2019) Acquired tolerance of the photosynthetic apparatus to photoinhibition as a result of growing Solanum lycopersicum at moderately higher temperature and light intensity. Functional Plant Biology 46(6), 555-566.
| Crossref | Google Scholar | PubMed |
Guan C, Ji J, Zhang X, Li X, Jin C, Guan W, Wang G (2015) Positive feedback regulation of a Lycium chinense-derived VDE gene by drought-induced endogenous ABA, and over-expression of this VDE gene improve drought-induced photo-damage in Arabidopsis. Journal of Plant Physiology 175, 26-36.
| Crossref | Google Scholar | PubMed |
Han H, Gao S, Li B, Dong X-C, Feng H-L, Meng Q-W (2010) Overexpression of violaxanthin de-epoxidase gene alleviates photoinhibition of PSII and PSI in tomato during high light and chilling stress. Journal of Plant Physiology 167(3), 176-183.
| Crossref | Google Scholar | PubMed |
Han J, Gu L, Warren JM, Guha A, Mclennan DA, Zhang W, Zhang Y (2022) The roles of photochemical and non-photochemical quenching in regulating photosynthesis depend on the phases of fluctuating light conditions. Tree Physiology 42(4), 848-861.
| Crossref | Google Scholar | PubMed |
Hernández I, Munné-Bosch S (2015) Linking phosphorus availability with photo-oxidative stress in plants. Journal of Experimental Botany 66(10), 2889-2900.
| Crossref | Google Scholar | PubMed |
Huang J-L, Cheng L-L, Zhang Z-X (2007) Molecular cloning and characterization of violaxanthin de-epoxidase (VDE) in Zingiber officinale. Plant Science 172(2), 228-235.
| Crossref | Google Scholar |
Hwang H-H, Liu Y-T, Huang S-C, Tung C-Y, Huang F-C, Tsai Y-L, Cheng T-F, Lai E-M (2015) Overexpression of the HspL promotes Agrobacterium tumefaciens virulence in Arabidopsis under heat shock conditions. Phytopathology 105, 160-168.
| Crossref | Google Scholar | PubMed |
Jahns P, Holzwarth AR (2012) The role of the xanthophyll cycle and of lutein in photoprotection of photosystem II. Biochimica et Biophysica Acta (BBA) – Bioenergetics 1817, 182-193.
| Crossref | Google Scholar |
Küster L, Lücke R, Brabender C, Bethmann S, Jahns P (2023) The amount of zeaxanthin epoxidase but not the amount of violaxanthin de-epoxidase is a critical determinant of zeaxanthin accumulation in Arabidopsis thaliana and Nicotiana tabacum. Plant and Cell Physiology 64(10), 1220-1230.
| Crossref | Google Scholar | PubMed |
Lacour T, Babin M, Lavaud J (2020) Diversity in xanthophyll cycle pigments content and related nonphotochemical quenching (NPQ) among microalgae: implications for growth strategy and ecology. Journal of Phycology 56(2), 245-263.
| Crossref | Google Scholar | PubMed |
Levin G, Kulikovsky S, Liveanu V, Eichenbaum B, Meir A, Isaacson T, Tadmor Y, Adir N, Schuster G (2021) The desert green algae Chlorella ohadii thrives at excessively high light intensities by exceptionally enhancing the mechanisms that protect photosynthesis from photoinhibition. The Plant Journal 106(5), 1260-1277.
| Crossref | Google Scholar | PubMed |
Li S, Yang W, Yang T, Chen Y, Ni W (2015) Effects of cadmium stress on leaf chlorophyll fluorescence and photosynthesis of Elsholtzia argyi – a cadmium accumulating plant. International Journal of Phytoremediation 17(1), 85-92.
| Crossref | Google Scholar |
Lou Y, Sun H, Zhu C, Yang K, Li X, Gao Z (2022) PeVDE, a violaxanthin de-epoxidase gene from moso bamboo, confers photoprotection ability in transgenic Arabidopsis under high light. Frontiers in Plant Science 13, 927949.
| Crossref | Google Scholar | PubMed |
Luo Q, Liu R, Zeng L, Wu Y, Jiang Y, Yang Q, Nie Q (2020) Isolation and molecular characterization of NtMYB4a, a putative transcription activation factor involved in anthocyanin synthesis in tobacco. Gene 760, 144990.
| Crossref | Google Scholar |
Nakamura S, Izumi M (2018) Regulation of chlorophagy during photoinhibition and senescence: lessons from mitophagy. Plant and Cell Physiology 59(6), 1135-1143.
| Crossref | Google Scholar | PubMed |
Okuzaki A, Tabei Y (2012) Improvement of the plastid transformation protocol by modifying tissue treatment at pre- and post-bombardment in tobacco. Plant Biotechnology 29(3), 307-310.
| Crossref | Google Scholar |
Pinnola A, Bassi R (2018) Molecular mechanisms involved in plant photoprotection. Biochemical Society Transactions 46(2), 467-482.
| Crossref | Google Scholar | PubMed |
Shi Y, Ke X, Yang X, Liu Y, Hou X (2022) Plants response to light stress. Journal of Genetics and Genomics 49(8), 735-747.
| Crossref | Google Scholar | PubMed |
Simkin AJ, Kapoor L, Doss CGP, Hofmann TA, Lawson T, Ramamoorthy S (2022) The role of photosynthesis related pigments in light harvesting, photoprotection and enhancement of photosynthetic yield in planta. Photosynthesis Research 152, 23-42.
| Crossref | Google Scholar | PubMed |
Sun LN, Wang F, Wang JW, Sun LJ, Gao WR, Song XS (2019) Overexpression of the ChVDE gene, encoding a violaxanthin de-epoxidase, improves tolerance to drought and salt stress in transgenic Arabidopsis. 3 Biotech 9(5), 197.
| Crossref | Google Scholar | PubMed |
Yang J, Choi M-J, Kim S-H, Choi H-J, Kim S-C (2021) Plastome characterization and phylogenomic analysis yield new insights into the evolutionary relationships among the species of the subgenus Bryocles (Hosta; asparagaceae) in East Asia. Plants 10(10), 1980.
| Crossref | Google Scholar |
Yoo M-J, Lee B-Y, Kim S, Lim CE (2021) Phylogenomics with Hyb-Seq unravels Korean Hosta evolution. Frontiers in Plant Science 12, 645735.
| Crossref | Google Scholar |
Zhang J, Sui C, Wang Y, Liu S, Liu H, Zhang Z, Liu H (2020) Transcriptome-wide analysis reveals key DEGs in flower color regulation of Hosta plantaginea (Lam.) Aschers. Genes 11(1), 31.
| Crossref | Google Scholar |
Zhao W, Wang S, Li X, Huang H, Sui X, Zhang Z (2012) Molecular cloning and characterization of the light-regulation and circadian-rhythm of the VDE gene promoter from Zingiber officinale. Plant Cell Reports 31, 1381-1392.
| Crossref | Google Scholar | PubMed |
Zia A, Gulzar S, Ruban AV (2024) Enhanced photochemical efficiency of PSII in Prosopis juliflora suggests contribution to invasion advantage over native C3 xero-halophytes under salt stress. Functional Plant Biology 51, FP23272.
| Crossref | Google Scholar |