Expression of heterosis in photosynthetic traits in F1 generation of sorghum (Sorghum bicolor) hybrids and relationship with yield traits
Renjie Zhao A , Yueqiao Li B , Chen Xu B , Zhian Zhang
A
B
C
Abstract
Heterosis is a crucial factor in enhancing crop yield, particularly in sorghum (Sorghum bicolor). This research utilised six sorghum restorer lines, six sorghum sterile lines, and 36 hybrid combinations created through the NCII incomplete double-row hybridisation method. We evaluated the performance of F1 generation hybrids for leaf photosynthesis-related parameters, carbon metabolism-related enzymes, and their correlation with yield traits during the flowering stage. Results showed that hybrid sorghum exhibited significant high-parent heterosis in net photosynthetic rate (Pn), transpiration rate (Tr), stomatal conductance (Gs), apparent leaf meat conductance (AMC), ribulose-1,5-bisphosphate (RuBP) carboxylase, phosphoenolpyruvate (PEP) carboxylase, and sucrose phosphate synthase (SPS). Conversely, inter-cellular carbon dioxide concentration (Ci), instantaneous water uses efficiency (WUE), and sucrose synthase (SuSy) displayed mostly negative heterosis. Traits such as 1000-grain weight (TGW), grain weight per spike (GWPS), and dry matter content (DMC) exhibited significant high-parent heterosis, with TGW reaching the highest value of 82.54%. Pn demonstrated positive correlations with Tr, Ci, Gs, RuBP carboxylase, PEP carboxylase, GWPS, TGW, and DMC, suggesting that Tr, Ci, and Gs could aid in identifying high-photosynthesis sorghum varieties. Concurrently, Pn could help select carbon-efficient sorghum varieties due to its close relationship with yield. Overall, the F1 generation of sorghum hybrids displayed notable heterosis during anthesis. Combined with field performance, Pn at athesis can serve as a valuable indicator for early prediction of the yield potential of the F1 generation of sorghum hybrids and for screening carbon-efficient sorghum varieties.
Keywords: carbon metabolising enzymes, F1 generation, heterosis, hybridisation method, photosynthesis, plant materials, sorghum, yield.
Introduction
Sorghum (Sorghum bicolor) is a C4 plant that was historically a significant grain crop in China (McCormick et al. 2018). While its primary use has shifted from food to brewing raw material due to advancements in maize (Zea mays) and rice (Oryza sativa) production (Li et al. 2021b), sorghum remains vital in arid and semi-arid regions for its high yield, salinity resistance, and drought tolerance (Shehzad and Okuno 2020). As demand rises, increasing sorghum yield on existing cropland is a crucial research focus (Follett 2001). Improving photosynthesis and leveraging heterosis in sorghum can boost yield per unit area (Li et al. 2021a), highlighting the importance of studying the biparental photosynthetic traits in sorghum hybrids to select varieties with strong heterosis and maximise yield potential. In sorghum hybrids, genetic variety improves photosynthetic efficiency, yield potential, tolerance to stress, and physiological processes inside the plant. It increases grain weight, dry matter content, and biomass while broadening the gene pool and encouraging complementing interactions. Detecting genetic markers and choosing better parents also helps breeding programs.
Heterosis, also known as hybrid vigour, is a phenomenon where the offspring of two different parents in the F1 generation exhibit superior traits such as growth potential (Huang et al. 2016), adaptability, and yield compared to their parents. Breeders commonly leverage hybrid dominance to enhance crops like maize and rice genetically, improving qualities like yield and resistance (Gou et al. 2021). Photosynthesis, a crucial process for plant growth and yield formation, is a central physiological activity in sorghum growth (Hall and Richards 2013; Fu et al. 2014). Photosynthesis in sorghum is essential for energy generation, growth, tolerance to stress, efficient use of water and nutrients, and yield formation. It produces ATP, transforms CO2 into glucose, encourages biomass build-up, and affects growth hormones. It also facilitates the uptake of nutrients and efficient utilisation of water. The formation of sorghum yield primarily involves starch and protein synthesis and accumulation, with carbon metabolising enzymes playing a pivotal role. Enhancing photosynthesis and carbon metabolising enzyme activities in sorghum leaves is an effective strategy to boost crop yield (Oguz et al. 2022; Wu et al. 2023). Breeders select sorghum materials with high photosynthetic rates for crossbreeding to improve photosynthetic efficiency and combine photosynthesis-related traits with other desirable qualities to achieve higher yields (Cakmak and Kirkby 2008; Saithalavi et al. 2021; Li et al. 2022). Some tactics include agronomic practice optimisation, hybrid maintenance breeding, genomic selection, marker-aided selection, backcross breeding, managing cross-pollination, sustaining hybrid seed output, and using biotechnology. The efficiency, stress tolerance, and yield potential of sorghum hybrids largely depend on stomatal conductance (Gs) and net photosynthetic rate (Pn). Because of their genetic and physiological adaptations, higher Gs and Pn are important breeding goals because they improve photosynthesis, water utilisation, and biomass accumulation. Improved photosynthetic sorghum hybrids support sustainable agriculture by offering ecological advantages such as better soil health, lower environmental impact, carbon sequestration, biodiversity conservation, climate resilience, and ecosystem services. Recent research has focused on studying hybrid dominance in photosynthetic physiological traits (Sharma et al. 2013), with findings showing significant differences in indica hybrid rice sword leaf photosynthetic characteristics compared to the parents, including positive ultra-high-parent heterosis in Pn and Gs (Liu et al. 2014; Reynolds et al. 2017). Photosynthesis, CO2 fixation, chloroplast activity, stomatal control, and vascular system efficiency are all enhanced by genetic complementation. It improves hormone balance, stress resistance, and drought tolerance, promoting strong growth and efficient water use. Increased energy production, improved nutrient uptake and transport, enhanced carbon assimilation, resilience to environmental stresses like heat and drought, and continuous biomass accumulation are all made possible by higher photosynthetic rates and Gs. By comparing the photosynthetic capacity of different sorghum varieties (Wu et al. 2023), demonstrated that high-yielding hybrids exhibited increased key enzyme activities, enhanced photosynthetic intensity, and lower CO2 compensation points compared to their parental lines. These hybrids also displayed a significant heterosis for photosynthetic traits compared to their parents. The findings from these studies offer valuable insights into understanding hybrid dominance in photosynthetic physiological characteristics. Selecting various sterile and restorer lines, managing cross-pollination, monitoring growth, optimising watering and fertilisation, implementing pest and disease control, testing hybrid seed purity, and using a randomised block design are all necessary for successfully implementing double-row hybridisation.
Current research on photosynthetic hybridisation in sorghum primarily focuses on leaf photosynthetic characteristics, with limited information on the hybridisation of enzymes linked to these characteristics. The study investigates how photosynthetic characteristics and enzyme activity affect plant performance and yield, helping to find superior hybrids, supporting breeding initiatives, enhancing yield, and assisting in stress resilience. Its physiological insights and data-driven breeding choices may achieve better productivity and sustainability. Investigating the hybrid dominance of photosynthetic traits and their associated enzymes in sorghum is crucial for identifying sorghum hybrids with highly dominant combinations and selecting suitable parents (Brown and Bouton 1993). We used a randomised block design to evaluate heterosis in photosynthetic attributes and yield characteristics of sorghum hybrids. In addition to assessing the activity of enzymes in leaf samples, the experiment measured the Pn, Gs, Tr, and intercellular CO2 concentration (Ci) on flag leaves. Software for statistical analysis was used to examine the data for heterosis values. In sorghum hybrids, environmental variables during anthesis significantly influence photosynthetic characteristics and heterosis. Efficiency and yield potential are determined by temperature, light intensity, availability of water, availability of nutrients, concentration of CO2, and humidity. Protective mechanism-equipped hybrids work better. High photosynthetic sorghum hybrids improve fertiliser usage efficiency, crop output, and resistance to environmental stresses. They support climate adaptability, lessen the demand for irrigation and fertiliser, and sustainable agriculture. In addition, they provide environmental protection, increased profitability and competitiveness in the market, and food security. This study utilised 36 sorghum hybrid F1 generations and their respective parents to assess hybrid dominance in leaf photosynthetic parameters, key enzyme activities during photosynthesis, yield formation, and other relevant factors. It also examined their correlation with yield in field conditions. The goal is to gain a comprehensive understanding of the critical indicators and criteria for identifying sorghum hybrids with strong dominant combinations and their parents, thereby establishing a theoretical foundation for leveraging sorghum hybrid dominance and facilitating the development of new hybrid sorghum varieties with robust dominance more efficiently and effectively.
Materials and methods
Plant materials
Table 1 shows six sterile sorghum (Sorghum bicolor L.) lines, six restorer lines, and 36 hybrid combinations within their respective groupings. The Institute of Crops and Resources at the Jilin Academy of Agricultural Sciences generously supplied these plant materials.
Sterile lines | Restorer lines | ||||||
---|---|---|---|---|---|---|---|
Nan133 | Ji318R | T40 | 0–30 | 501R | JiR107 | ||
Ji2055A | Ji2055A × Nan133 | Ji2055A × Ji318R | Ji2055A × T40 | Ji2055A × 0–30 | Ji2055A × 501R | Ji2055A × JiR107 | |
TAM428A | TAM428A × Nan133 | TAM428A × Ji318R | TAM428A × T40 | TAM428A × 0–30 | TAM428A × 501R | TAM428A × JiR107 | |
521A | 521A × Nan133 | 521A × Ji318R | 521A × T40 | 521A × 0–30 | 521A × 501R | 521A × JiR107 | |
I15A | I15A × Nan133 | I15A × Ji318R | I15A × T40 | I15A × 0–30 | I15A × 501R | I15A × JiR107 | |
170A | 170A × Nan133 | 170A × Ji318R | 170A × T40 | 170A × 0–30 | 170A × 501R | 170A × JiR107 | |
L407A | L407A × Nan133 | L407A × Ji318R | L407A × T40 | L407A × 0–30 | L407A × 501R | L407A × JiR107 |
Climatic conditions
The test site was black soil with soybean as the previous crop. Average organic matter content of the 0–40 cm layer was 21.6 g kg−1, average total nitrogen content was 0.946 g kg−1, average effective phosphorus content was 14.9 mg kg−1, average quick-acting potassium content was 129.6 mg kg−1, and the pH value was 6.43. The area belongs to the cold-temperate zone, with a continental semi-humid monsoon climate, a windy and less rainy spring, and a sunny autumn. The region has a cold-temperate, semi-humid monsoon continental climate, with windy spring and little rain, sunny autumn, significant temperature difference between morning and evening, and a long winter with little snow. In 2022, the effective cumulative temperature (≥10°C) was 3135.5°C, average daily temperature during the whole reproductive period (May–October) was 20.49 ± 4.59°C, and the total precipitation during the entire reproductive period (May–October) was 560.4 mm. Average daily temperature and precipitation are in Fig. 1. The meteorological data of the experimental area were obtained from the automatic meteorological monitoring system of the experimental base.
Test methods
The experiment was conducted at the Sorghum Germplasm Innovation Experimental Base in Gongzhuling Campus of Jilin Academy of Agricultural Sciences, China (43°29′N, 124°48′E). The field trial was in a randomised block design with a six-row area, row spacing of 0.65 m, row length of 5 m, and three replications, with a total of 144 plots, and the location of each plot was 19.5 m2. Seeds were sown on 13 May 2021, and seedlings were set at the five-leaf stage. The seedling retention density was 120,000 per ha, and the seedlings were harvested on 29 September 2021 using conventional field cultivation and management. Six sterile and restorer lines were utilised in the Sorghum Experiment to produce 36 different hybrid pairings. Following the sowing of seeds and thinning to produce 120,000 plants per ha, five plants with uniform leaf ages were chosen randomly for measurements.
Photosynthesis measurements were carried out during the flowering stage of sorghum. The cold-temperate semi-humid monsoon climate in which this experiment was carried out was unlike the usual warm, dry regions. The black soil had a specified organic matter, pH, nitrogen, phosphorus, and potassium. A total of 120,000 plants per ha were planted, with a set row spacing. Although photosynthetic metrics were manipulated, standard growth conditions were used when evaluating yield and biomass. Five plants of each variety with uniform leaf age were randomly selected for each replicate. Net photosynthetic rate (Pn), stomatal conductance (Gs), transpiration rate (Tr), and intercellular CO2 concentration (Ci) of the middle part of the flag leaves of sorghum were measured using the Li-6800 Photosynthetic Fluorescence Measurement System (Li-Cor Inc., Lincoln, NE, USA). It was used to calculate the transpiration and Pn in sorghum flag leaves. Instantaneous water use efficiency (WUE) and apparent chloroplastic conductance (AMC) was calculated from 08:00 hours to 12:00 hours on a sunny and windless day. Light intensity was set at 1800 μmol m−2 s−1, and the atmospheric CO2 concentration was set at 400 μmol mol−1. In photosynthetic measurements, the 1800 μmol m−2 s−1 light intensity mimics natural circumstances, maximises photosynthetic activity, standardises results, prevents photoinhibition, and assists breeding program research aims. After measuring photosynthesis, the leaves were removed and placed in liquid nitrogen cartridges for enzyme measurements.
Instantaneous WUE was obtained using the equation WUE = Pn/Tr (Zhang and Chen 2006), where Tr and Pn are the same leaf’s mean Tr and Pn, respectively.
AMC was obtained using the equation: AMC = Pn/Ci (Li et al. 2017), where Ci and Pn are the mean values of Ci and Pn, respectively, for the same leaf.
Ribulose-1,5-bisphosphate (RuBP) carboxylase, phosphoenolpyruvate (PEP) carboxylase, sucrose synthase (SuSy), and sucrose phosphate synthase (SPS) activities were determined using plant assay kits supplied by Shanghai Zhenke Bio-technology Co. (Fu et al. 2022).
Twenty plants were taken from each replication for indoor seed testing to investigate the dry matter content (DMC) and grain weight per spike (GWPS); 1000 grains were weighed after drying to determine 1000-grain weight (TGW) (Xu et al. 2022).
Data analysis
One-way ANOVA, honestly significant difference (HSD) comparisons, and correlation coefficient analysis were performed using Origin 2021 software plotting and SPSS 26.0 software. Hybrid dominance was calculated according to the formula, mid-parent heterosis = (F1-MP)/MP × 100%, and high-parent heterosis = (F1-HP)/HP × 100%, in which F1 is the average value of traits of hybrid combinations, and MP and HP denote the two-parent average and the high parental value, respectively. Since the sterile lines could not be calculated for yield, the yield hybrid dominance was all calculated with the restored lines as the high parental value (Xiong et al. 2022).
Results
Differences in photosynthetic traits between hybrid sorghum and parents
Table 2 shows the 12 sterile and restorer lines and 36 hybrid sorghum combinations, observing significant differences in six photosynthetic characteristics. Factors such as stomatal control, water availability, plant response to stress, and measurement difficulty were the reasons behind the most significant variance coefficient in Tr compared to Pn. Increased variability in Tr readings can result from environmental factors such as temperature, humidity, and wind speed. The coefficient of variation was highest for Tr at 14.39% and next highest for Pn at 13.08%. Among the traits, the sterile line TAM428A exhibited the highest Pn, Tr, and Gs, surpassing mean values by 19.9%, 29.1%, and 40.5%, respectively. Similarly, the restoration line JiR107 showed the highest values for these traits, exceeding mean values by 13.92%, 26.1%, and 34.91%, respectively. In the F1 generation of crosses in combination 521A/0–30, the highest values for Pn, Tr, and Gs were 56.17%, 49.87%, and 67.88% higher than the mean values. The hybrid dominance analysis of the F1 generation of crossings seeks to pinpoint superior hybrids, enhance crop output, increase stress resilience, maximise resource utilisation, and direct breeding initiatives. Significant heterosis in hybrids is identified by this research, improving crop production and nutrient utilisation.
Genotypes | Pn (μmol m−2 s−1) | Tr (mmol m−2 s−1) | Ci (μmol mol−1) | Gs (mmol m−2 s−1) | WUE | AMC | ||
---|---|---|---|---|---|---|---|---|
Male sterile lines | Ji2055A | 29.54 | 3.07 | 129.71 | 258.44 | 9.62 | 0.23 | |
TAM428A | 34.34 | 4.17 | 162.31 | 349.12 | 8.24 | 0.21 | ||
521A | 31.77 | 3.88 | 109.76 | 288.56 | 8.19 | 0.29 | ||
I15A | 30.62 | 3.44 | 123.23 | 227.51 | 8.90 | 0.25 | ||
170A | 18.38 | 1.83 | 97.22 | 164.54 | 10.04 | 0.19 | ||
L407A | 27.2 | 2.98 | 172.52 | 202.69 | 9.13 | 0.16 | ||
A-lines mean | 28.64 | 3.23 | 132.46 | 248.48 | 9.02 | 0.22 | ||
Restorer lines | Nan133 | 30.86 | 3.45 | 152.21 | 236.72 | 8.94 | 0.20 | |
Ji318R | 31.28 | 3.75 | 153.16 | 259.88 | 8.34 | 0.20 | ||
T40 | 27.32 | 2.98 | 182.99 | 192.82 | 9.17 | 0.15 | ||
0–30 | 35.81 | 4.38 | 176.35 | 354.82 | 8.18 | 0.20 | ||
501R | 32.16 | 3.78 | 167.13 | 280.52 | 8.51 | 0.19 | ||
JiR107 | 36.9 | 4.88 | 168.71 | 384.27 | 7.56 | 0.22 | ||
R-lines mean | 32.39 | 3.87 | 166.76 | 284.84 | 8.45 | 0.19 | ||
F1 hybrid | Ji2055A × Nan133 | 33.24 | 4.23 | 195.33 | 316.34 | 7.86 | 0.17 | |
Ji2055A × Ji318R | 38.56 | 4.46 | 220.58 | 296.54 | 8.65 | 0.17 | ||
Ji2055A × T40 | 34.59 | 4.00 | 167.97 | 339.12 | 8.65 | 0.21 | ||
Ji2055A × 0–30 | 40.70 | 4.83 | 232.45 | 402.29 | 8.43 | 0.18 | ||
Ji2055A × 501R | 31.36 | 3.87 | 142.77 | 279.32 | 8.10 | 0.22 | ||
Ji2055A × JiR107 | 43.19 | 5.45 | 112.75 | 426.72 | 7.92 | 0.38 | ||
TAM428A × Nan133 | 35.47 | 4.17 | 98.56 | 346.50 | 8.51 | 0.36 | ||
TAM428A × Ji318R | 31.63 | 3.57 | 84.67 | 230.14 | 8.86 | 0.37 | ||
TAM428A × T40 | 35.73 | 5.35 | 249.34 | 324.39 | 6.68 | 0.14 | ||
TAM428A × 0–30 | 37.24 | 3.22 | 100.43 | 371.84 | 11.57 | 0.37 | ||
TAM428A × 501R | 26.51 | 2.95 | 121.06 | 224.19 | 8.99 | 0.22 | ||
TAM428A × JiR107 | 40.53 | 5.01 | 254.62 | 394.54 | 8.09 | 0.16 | ||
521A × Nan133 | 37.25 | 3.72 | 129.39 | 387.10 | 10.01 | 0.29 | ||
521A × Ji318R | 21.63 | 2.48 | 79.71 | 165.89 | 8.72 | 0.27 | ||
521A × T40 | 30.39 | 3.92 | 131.92 | 261.41 | 7.75 | 0.23 | ||
521A × 0–30 | 49.79 | 5.74 | 218.22 | 487.80 | 8.67 | 0.23 | ||
521A × 501R | 31.05 | 4.04 | 191.18 | 326.10 | 7.69 | 0.16 | ||
521A × JiR107 | 40.81 | 5.12 | 187.23 | 387.78 | 7.97 | 0.22 | ||
I15A × Nan133 | 35.07 | 4.40 | 200.08 | 385.30 | 7.97 | 0.18 | ||
I15A × Ji318R | 40.49 | 5.23 | 193.77 | 398.67 | 7.74 | 0.21 | ||
I15A × T40 | 32.39 | 3.81 | 112.92 | 255.37 | 8.50 | 0.29 | ||
I15A × 0–30 | 39.55 | 4.32 | 139.04 | 375.77 | 9.16 | 0.28 | ||
I15A × 501R | 31.60 | 3.40 | 102.79 | 240.45 | 9.29 | 0.31 | ||
I15A × JiR107 | 31.49 | 3.88 | 121.13 | 298.08 | 8.12 | 0.26 | ||
170A × Nan133 | 33.81 | 4.07 | 103.27 | 255.06 | 8.31 | 0.33 | ||
170A × Ji318R | 21.69 | 3.01 | 100.82 | 180.29 | 7.21 | 0.22 | ||
170A × T40 | 18.95 | 2.84 | 120.00 | 285.11 | 6.67 | 0.16 | ||
170A × 0–30 | 24.37 | 2.69 | 125.07 | 210.88 | 9.06 | 0.19 | ||
170A × 501R | 19.26 | 2.89 | 88.28 | 159.12 | 6.66 | 0.22 | ||
170A × JiR107 | 16.09 | 2.00 | 54.37 | 101.94 | 8.05 | 0.30 | ||
L407A × Nan133 | 28.99 | 3.80 | 109.62 | 229.98 | 7.63 | 0.26 | ||
L407A × Ji318R | 32.07 | 3.88 | 229.17 | 247.97 | 8.27 | 0.14 | ||
L407A × T40 | 33.56 | 3.93 | 194.15 | 298.72 | 8.54 | 0.17 | ||
L407A × 0–30 | 30.95 | 3.20 | 109.69 | 242.24 | 9.67 | 0.28 | ||
L407A × 501R | 15.39 | 1.91 | 149.73 | 137.78 | 8.06 | 0.10 | ||
L407A × JiR107 | 22.38 | 2.65 | 140.86 | 189.44 | 8.45 | 0.16 | ||
Hybrids mean | 31.88 | 3.83 | 147.58 | 290.56 | 8.35 | 0.23 | ||
Mean | 31.54 | 3.76 | 148.09 | 284.58 | 8.44 | 0.23 | ||
s.d. | 4.125 | 0.541 | 10.233 | 15.282 | 0.891 | 0.017 | ||
CV (%) | 13.08 | 14.39 | 6.91 | 5.37 | 10.56 | 7.39 | ||
Difference in combinations (F) | 31.52** | 7.19** | 135.22** | 97.81** | 28.88** | 8.63** |
Pn, net photosynthetic rate; Tr, transpiration rate; Ci, intercellular CO2 concentration; Gs, stomatal conductance; WUE, water use efficiency; AMC, apparent leaf meat conductance.
*P < 0.05; **P < 0.01.
Relationship between hybrid sorghum combinations and parental photosynthetic traits
The correlation coefficients of 36 tested sorghum hybrid combinations with parental photosynthetic traits are in Table 3, showing that water use efficiency of the F1 generation of sorghum hybrids reached a significant level of negative correlation with the maternal values, Tr reached a considerable level of positive correlation with the parental values and the mid-parental values of both parents, and Pn reached a highly significant level of positive correlation with the mid-parental values of both parents. Other than that, none of the photosynthetic traits in the F1 generation of sorghum hybrids reached a significant correlation level with the parents and the mid-parent values of both parents.
Traits | Pn | Tr | Ci | Gs | WUE | AMC | |
---|---|---|---|---|---|---|---|
HMC | 0.424 | 0.163 | 0.012 | −0.25 | −0.882* | −0.626 | |
HRC | 0.720 | 0.861* | −0.215 | 0.727 | 0.284 | 0.401 | |
HMPC | 0.584** | 0.353* | 0.296 | 0.405* | −0.325 | 0.256 |
Correlation coefficients of hybrid F1 generation: HMC, maternal parent values; HRC, paternal parent values; HMPC, mid-parent values.
*P < 0.05; **P < 0.01.
Hybrid dominance analysis of photosynthetic traits in F1 generation of crosses
The hybrid dominance analysis of the photosynthetic characteristics of the F1 generation of crosses are in Fig. 2, showing that Pn, Tr, Gs, and AMC exhibited the highest number of favourable combinations of mid-parent heterosis and high-parent heterosis, along with a broader range of variability. Specifically, Pn was −56.4% to 39.04% for mid-parent heterosis and −48.15% to 47.35% for high-parent heterosis. Similarly, Tr displayed a range of −43.49% to 54.17% for mid-parent heterosis and −59.02% to 39.47% for high-parent heterosis. Gs and AMC also had significant ranges: from −62.85%−65.98% to −73.47%−62.77% for Gs; and −33.33%−80.49% to −47.37%−76.19% for AMC. The positive mid-parent and high-parent heterosis combinations were 22 and 19 for Pn, 25 and 19 for Tr, 24 and 18 for Gs, and 22 and 17 for AMC. In contrast, Ci and WUE had fewer favourable combinations, with 13 and 12 for Ci; and 12 and eight for WUE, respectively. These results suggest that not all photosynthetic traits in hybrid sorghum exhibit heterosis, with some indicators showing predominantly negative heterosis.
Hybrid dominance of (a) net photosynthetic rate, (b) transpiration rate, (c) intercellular CO2 concentration, (d) stomatal conductance, (e) water use efficiency, and (f) apparent leaf meat conductance. *P < 0.05.
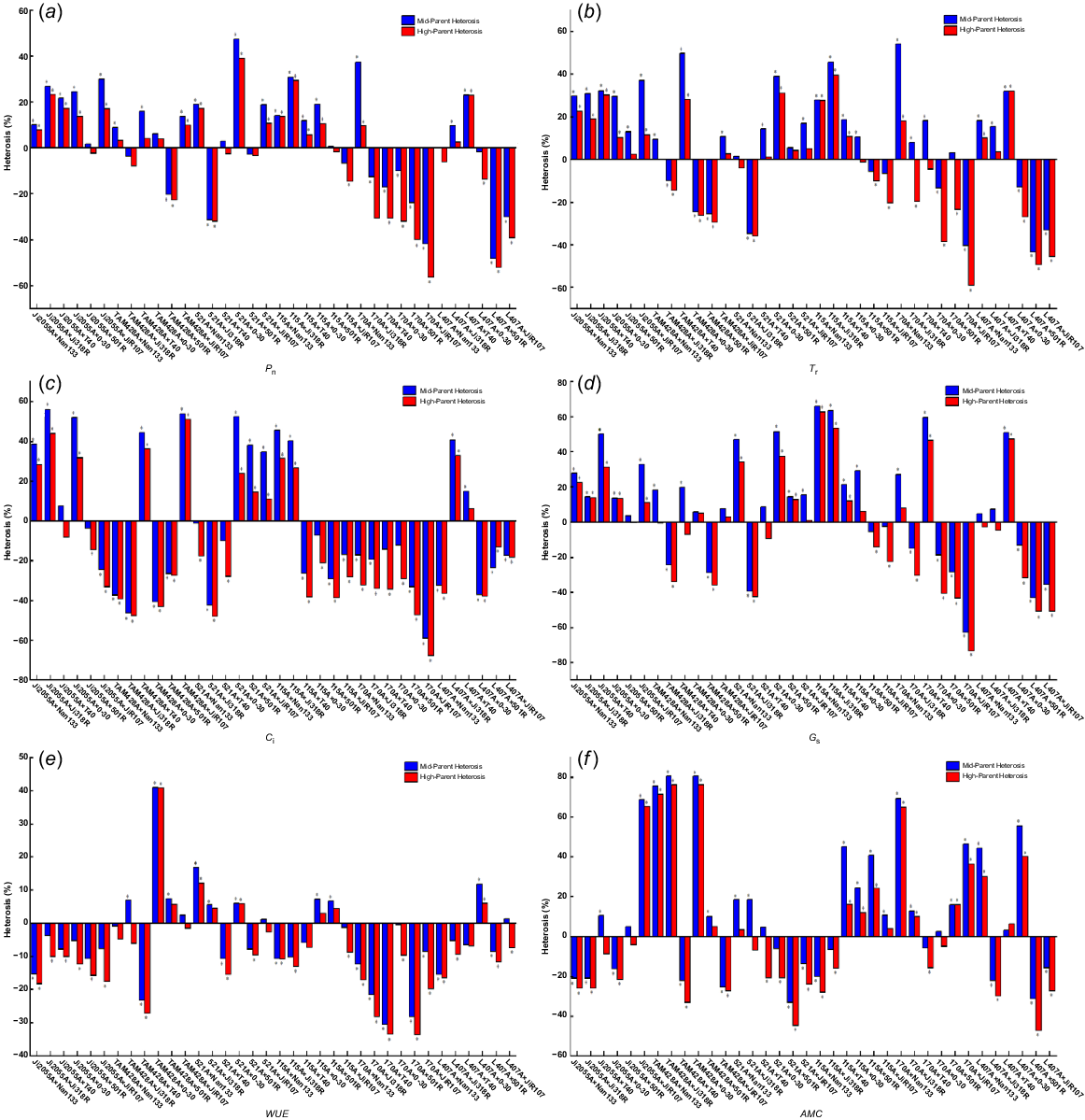
Regarding high-parent heterosis for the same photosynthetic trait, the variation depended on the specific hybridisation combinations. Due to genetic complementation, gene interactions, trait contributions, environmental adaptation, allele variation, and heterozygosity, high-parent heterosis for photosynthetic characteristics vary with hybridisation combinations, impacting the degree of heterosis in each hybrid combination. For instance, the combination 521A × 0–30 exhibited the highest Pn high-parent heterosis at 39.04%, whereas the combination L407A × Ji318R had a Pn high-parent heterosis of only 2.53%. Within the same hybrid combination, there were noticeable differences in the performance of hybrids for various photosynthetic traits. For example, TAM428A × Nan133 displayed a Pn high-parent heterosis of 3.29% and an AMC high-parent heterosis of 71.43%, while Tr, Ci, and Gs showed negative heterosis. Furthermore, when the infertile lines were consistent in hybrid combinations, but the restorer lines differed, the performance of the hybrids for photosynthetic traits also varied. Allelic interactions and distinct genetic contributions account for the high-parent heterosis variance observed in restorer lineages. These variations impact physiological characteristics like photosynthetic efficiency and stress resistance. The degree and kind of heterosis are determined by the restorer line used, highlighting the significance of choosing the right lines for hybrid vigour and trait improvement. Hybrids paired with Nan133 and T40 exhibited positive mid-parent heterosis in Tr and Gs. In contrast, hybrids paired with 501R showed negative high-parent heterosis in Pn, and those paired with T40 and JiR107 displayed negative high-parent heterosis in WUE.
Additionally, the Pn high-parent heterosis of hybrid sorghum with Nan133 and 0–30 as restorer lines was more significant than that of hybrid sorghum with 501R as the restorer line. Similarly, when the restorer lines were the same but the sterile lines differed, the performance of hybrids for each photosynthetic trait also varied. For instance, the hybrid with Ji2055A as the maternal parent showed positive heterosis in both Pn and Tr, whereas the hybrid with 170A as the maternal parent displayed negative heterosis in both Pn and Tr. Moreover, the Pn high-parent heterosis of hybrid sorghum with Ji2055A, 521A, and I15A as sterile lines was higher than that of hybrid sorghum with 170A and L407A as sterile lines. Among the 36 hybrid combinations, significant mesophilic and hyperphilic dominance levels were observed for most photosynthetic traits, with Pn, Tr, and Gs showing the most pronounced effects.
Delineation of heterozygous groups for parental photosynthetic traits
For the phenotypic values of the six photosynthetic traits, the clustering results of the 12 parental materials are in Fig. 3. At Euclidean Distance (ED) = 0.01, the ED-based cluster analysis classified the 12 sorghum parents into three categories: the first category contained seven parents, Ji2055A, 0–30, I15A, Ji318R, 501R, 170A, and Nan133, which generally had higher; the second category includes three parents, TAM428A, JiR107, and 521A, which have high photosynthetic and transpiration rates; and the third category consists of two parents, L407A and T40, which are mediocre in terms of performance of various traits.
Differences in carbon metabolising enzymes and yield-related traits between hybrid sorghum and parents
Table 4 shows significant differences in carbon metabolising enzymes and yield traits between the hybrid combinations and their parents. For instance, 521A exhibited the highest RuBP carboxylase and PEP carboxylase levels in the sterile line, surpassing the mean values by 3.32% and 14.5%, respectively. TAM428A displayed the highest dry matter content among the parents, exceeding the mean value by 12.11%. Among the restorer lines, JiR107 had the highest RuBP carboxylase levels, exceeding the mean by 10.1%. Similarly, 501R showed the highest sucrose synthase and grain weight per spike, surpassing the mean values by 5.24% and 9.83%, respectively. Additionally, 0–30 exhibited the highest sucrose phosphate synthase and dry matter content, exceeding the mean values by 6.72% and 18.74%, respectively. At the same time, Nan133 had the highest PEP carboxylase and 1000-grain weight, surpassing the mean values by 14.71% and 22%, respectively. The hybrid progeny also displayed significant differences in trait combinations, with TAM428A × 0–30 showing the highest RuBP carboxylase content, exceeding the mean by 12.43%. Regarding sucrose synthase, the combination TAM428A × 0–30 exhibited the highest content, surpassing the mean by 15.54%. Furthermore, the combination Ji2055A × 0–30 displayed the highest sucrose phosphate synthase content, exceeding the mean by 13.19%. The combination Ji2055A × Ji318R had the highest PEP carboxylase content, surpassing the mean by 17.43%. TAM428A × 0–30 showed the highest 1000-grain weight, exceeding the mean by 15.74%, while TAM428A × 501R exhibited the highest grain weight per spike, surpassing the mean by 22.35%. TAM428A × 0–30 displayed the highest dry matter content, exceeding the mean by 37%. Overall, the hybrid sorghums exhibited higher grain weight and dry matter content than their parents, with coefficients of variation of 12.57% and 18.77%, respectively. Hybrid sorghum, distinguished by heterosis, have higher grain weights and dry matter contents because of their genetic diversity, allelic interactions, improved photosynthesis, WUE, stress resilience, and optimal resource allocation. These traits all contribute to better physiological processes and performance.
Genotypes | RuBP (IU/g) | SuSy (U/g) | SPS (IU/g) | PEP (U/g) | TGW (g) | GWPS (g) | DMC (g) | ||
---|---|---|---|---|---|---|---|---|---|
Male sterile lines | Ji2055A | 72.09 | 31.85 | 14.78 | 3.50 | 59.71 | |||
TAM428A | 73.00 | 30.96 | 14.25 | 4.31 | 66.82 | ||||
521A | 74.04 | 28.78 | 13.19 | 4.58 | 63.06 | ||||
I15A | 70.05 | 32.16 | 14.97 | 4.11 | 61.18 | ||||
170A | 69.8 | 30.19 | 12.94 | 3.68 | 49.48 | ||||
L407A | 70.96 | 32.93 | 14.10 | 3.81 | 57.36 | ||||
A-lines mean | 71.66 | 31.15 | 14.04 | 4.00 | 59.60 | ||||
Restorer lines | Nan133 | 74.69 | 28.76 | 13.02 | 5.46 | 30.33 | 93.33 | 91.35 | |
Ji318R | 70.36 | 31.01 | 15.66 | 4.68 | 26.07 | 93.57 | 77.94 | ||
T40 | 69.29 | 29.40 | 13.52 | 3.74 | 23.32 | 96.3 | 88.93 | ||
0–30 | 79.77 | 30.22 | 15.72 | 5.27 | 21.18 | 88.57 | 105.19 | ||
501R | 70.99 | 32.13 | 14.85 | 4.27 | 27.77 | 102.75 | 87.42 | ||
JiR107 | 82.04 | 31.64 | 15.61 | 5.12 | 20.50 | 86.76 | 80.71 | ||
R-lines mean | 74.52 | 30.53 | 14.73 | 4.76 | 24.86 | 93.55 | 88.59 | ||
F1 hybrid | Ji2055A × Nan133 | 82.68 | 34.1 | 16.27 | 5.41 | 33.35 | 122.6 | 90.54 | |
Ji2055A × Ji318R | 83.47 | 30.23 | 16.48 | 5.58 | 35.00 | 135 | 105.02 | ||
Ji2055A × T40 | 75.44 | 27.86 | 15.78 | 4.92 | 31.47 | 119.68 | 94.12 | ||
Ji2055A × 0–30 | 86.74 | 29.60 | 16.82 | 5.48 | 36.20 | 115.42 | 101.88 | ||
Ji2055A × 501R | 74.86 | 29.50 | 16.23 | 3.58 | 33.43 | 132.65 | 92.96 | ||
Ji2055A × JiR107 | 75.36 | 30.09 | 12.81 | 5.28 | 32.00 | 136.59 | 107.91 | ||
TAM428A × Nan133 | 83.50 | 28.04 | 14.64 | 4.79 | 31.92 | 122.67 | 109.37 | ||
TAM428A × Ji318R | 73.70 | 28.28 | 15.08 | 3.94 | 26.85 | 119.81 | 97.77 | ||
TAM428A × T40 | 77.69 | 28.15 | 13.79 | 4.63 | 29.08 | 112.54 | 97.19 | ||
TAM428A × 0–30 | 86.86 | 34.42 | 16.11 | 5.34 | 38.68 | 126.2 | 140.88 | ||
TAM428A × 501R | 75.39 | 32.80 | 15.39 | 4.57 | 29.02 | 149.02 | 103.2 | ||
TAM428A × JiR107 | 69.80 | 30.27 | 13.12 | 4.85 | 27.23 | 131 | 103.65 | ||
521A × Nan133 | 85.71 | 28.24 | 15.61 | 5.34 | 37.40 | 141.02 | 118.39 | ||
521A × Ji318R | 76.80 | 28.26 | 13.02 | 3.94 | 31.03 | 124.52 | 106.44 | ||
521A × T40 | 77.82 | 28.41 | 13.03 | 5.20 | 30.00 | 122.02 | 103.77 | ||
521A × 0–30 | 82.85 | 32.68 | 14.24 | 5.47 | 36.33 | 145.5 | 129.31 | ||
521A × 501R | 80.67 | 33.07 | 15.77 | 4.75 | 35.22 | 131.75 | 109.83 | ||
521A × JiR107 | 79.36 | 29.08 | 13.97 | 5.27 | 32.30 | 125.21 | 105.74 | ||
I15A × Nan133 | 83.49 | 28.27 | 14.51 | 5.18 | 35.95 | 130.47 | 110.55 | ||
I15A × Ji318R | 80.54 | 28.14 | 13.79 | 4.57 | 32.37 | 116.56 | 100.98 | ||
I15A × T40 | 77.10 | 31.12 | 14.37 | 4.54 | 32.87 | 110.89 | 92.16 | ||
I15A × 0–30 | 83.09 | 30.6 | 15.24 | 5.39 | 37.85 | 126.63 | 111.32 | ||
I15A × 501R | 78.36 | 29.38 | 14.64 | 5.51 | 34.38 | 120.64 | 105.07 | ||
I15A × JiR107 | 80.35 | 32.30 | 15.2 | 4.9 | 35.35 | 126.3 | 93.61 | ||
170A × Nan133 | 78.38 | 30.91 | 13.77 | 4.42 | 27.23 | 105.68 | 93.94 | ||
170A × Ji318R | 71.72 | 28.68 | 15.84 | 4.77 | 27.53 | 97.45 | 96.52 | ||
170A × T40 | 73.04 | 28.71 | 15.14 | 4.09 | 28.33 | 104.17 | 81.13 | ||
170A × 0–30 | 76.00 | 32.02 | 15.01 | 4.51 | 32.97 | 115.22 | 95.05 | ||
170A × 501R | 71.54 | 27.31 | 14.91 | 4.50 | 29.32 | 114.41 | 90.37 | ||
170A × JiR107 | 73.94 | 30.78 | 15.42 | 4.84 | 28.53 | 104 | 95.15 | ||
L407A × Nan133 | 68.08 | 27.48 | 14.08 | 5.23 | 30.18 | 125 | 117.92 | ||
L407A × Ji318R | 66.39 | 26.76 | 14.45 | 3.86 | 26.55 | 111.71 | 98.51 | ||
L407A × T40 | 69.23 | 28.53 | 15.66 | 4.48 | 27.00 | 114.78 | 99.95 | ||
L407A × 0–30 | 79.05 | 30.35 | 15.6 | 5.04 | 32.57 | 118.24 | 109.67 | ||
L407A × 501R | 74.15 | 30.69 | 14.76 | 3.40 | 28.13 | 120.33 | 100.78 | ||
L407A × JiR107 | 68.12 | 27.4 | 14.29 | 3.47 | 27.17 | 108.98 | 91.08 | ||
Hybrids mean | 77.26 | 29.79 | 14.86 | 4.75 | 31.69 | 121.80 | 102.83 | ||
Mean | 76.21 | 30.05 | 14.74 | 4.66 | 30.71 | 117.76 | 95.64 | ||
s.d. | 5.422 | 1.907 | 1.045 | 0.635 | 4.296 | 14.81 | 17.954 | ||
CV (%) | 7.11 | 6.35 | 7.09 | 13.63 | 13.99 | 12.57 | 18.77 | ||
Difference in combinations (F) | 67.243** | 7.824* | 28.384* | 42.116** | 43.735** | 208.346** | 230.149** |
RuBP, ribulose-1,5-bisphosphate; SuSy, sucrose synthase; SPS, sucrose phosphate synthase; PEP, phosphoenolpyruvate; TWG, 1000-grain weight; GWPS, grain weight per spike; DMC, dry matter content.
*P < 0.05; **P < 0.01.
Relationship between hybrid sorghum combinations and parental leaf carbon metabolising enzymes
The correlation coefficients of 36 tested hybrid sorghum combinations with parental leaf carbon metabolism key enzymes are in Table 5, showing that PEP carboxylase in the F1 generation of sorghum hybrids reached a significant level of positive correlation with parental values and the mid-parental values of both parents. Other than that, all the carbon metabolism enzymes in the F1 generation of sorghum hybrids did not correlate significantly with the parents’ values and the mid-parent values of both parents.
Traits | RuBPCase | SuSy | SPS | PEPCase | |
---|---|---|---|---|---|
HMC | −0.203 | 0.245 | 0.051 | −0.111 | |
HRC | 0.198 | 0.119 | 0.050 | 0.355* | |
HMPC | 0.278 | −0.023 | 0.131 | 0.371* |
Correlation coefficients of hybrid F1 generation: HMC, maternal parent values; HRC, paternal parent values; and HMPC, mid-parent values.
RuBP, ribulose-1,5-bisphosphate; SuSy, sucrose synthase; SPS, sucrose phosphate synthase; PEPCase, PEP carboxylase.
*P < 0.05; **P < 0.01.
Heterosis analysis of carbon metabolising enzymes and yield-related traits in hybrid sorghum
As shown in Fig. 4, the mid-parental and high-parent heterosis of carbon metabolism-related enzymes and yield traits differed among the different hybrid sorghum combinations. High-parent heterosis variations were observed for several enzymes and traits, with ranges from −16.97% to 15.79% for RuBP carboxylase, from −18.74% to 11.18% for sucrose synthase, from −17.94% to 18.35% for sucrose phosphate synthase, from −32.23% to 31.55% for PEP carboxylase, from −10.21% to 82.54% for 1000-grain weight, from 4.15% to 64.28% for kernel weight per spike, and from −9.64% to 36.57% for dry matter content. Among the enzymes, RuBP carboxylase exhibited the highest number of favourable high-parent heterosis combinations, with the most significant combination being Ji2055A × Ji318R. PEP carboxylase had 18 favourable combinations, with Ji2055A × T40 being the highest. Sucrose phosphate synthase had 15 favourable combinations, with 521A × Nan133 being the highest. Sucrose synthase had the fewest favourable combinations at 8, with TAM428A × 0–30 being the highest. In terms of yield traits, most exhibited positive high-parent heterosis, with grain weight per spike in hybrid sorghum showing the highest at 64.28% for combination 521A × 0–30, 1000-grain weight reaching 82.54% with 34 combinations, and dry matter content at 36.57% with combination 521A × Ji318R.
Hybrid dominance of (a) RuBP carboxylase, (b) PEP carboxylase, (c) sucrose synthase, (d) sucrose phosphate synthase, (e) dry matter content, (f) grain weight per spike, (g) 1000-grain weight. *P < 0.05.
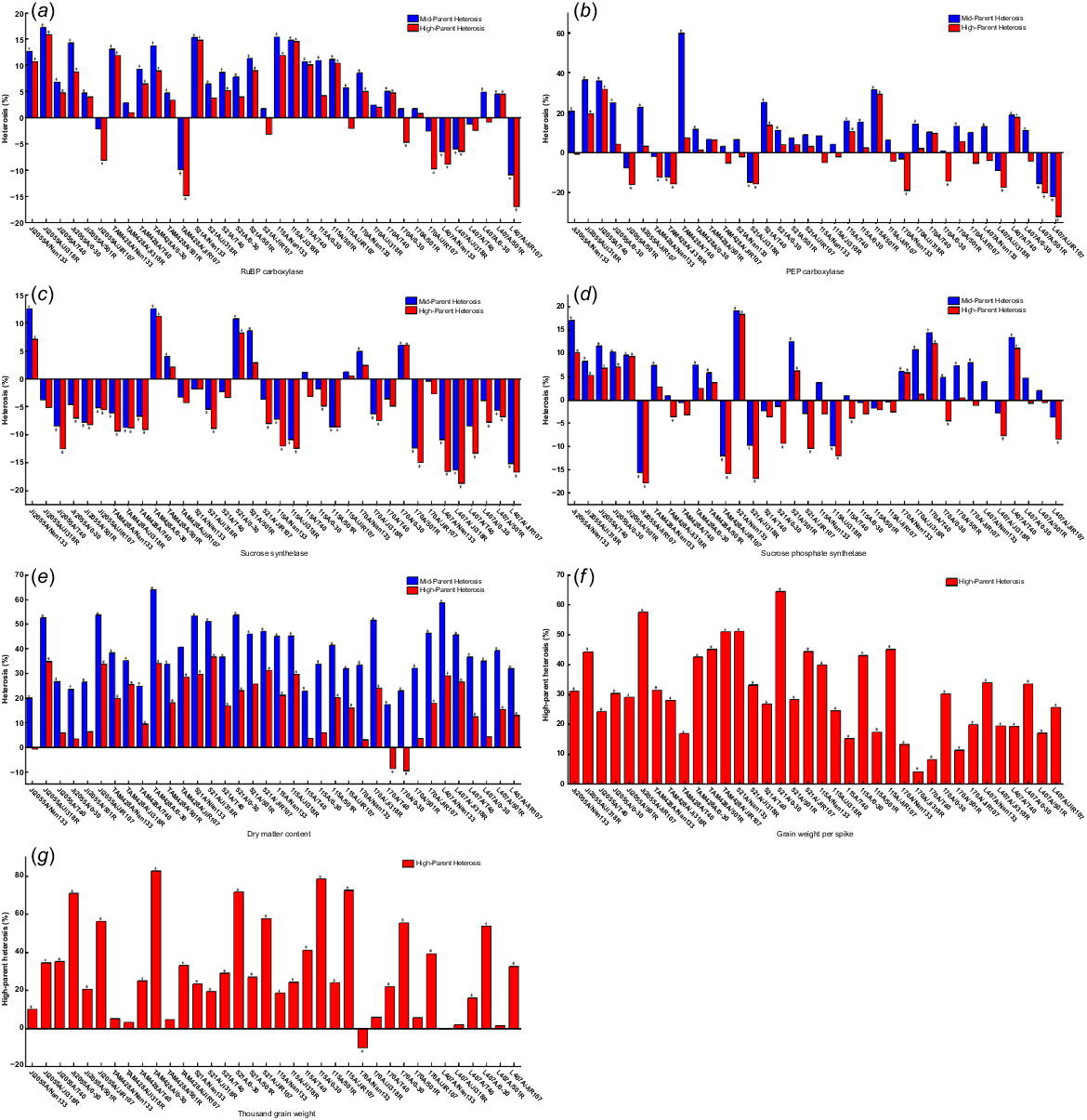
Significant differences were observed in the heterosis for various traits in the same hybrid sorghum combination. For instance, the combination TAM428A × JiR107 exhibited high-parent heterosis of −14.92%, −4.33%, −15.59%, and −5.27% for RuBP carboxylase, sucrose synthase, sucrose phosphate synthase, and PEP carboxylase, respectively. In contrast, it showed high-parent heterosis of 32.93%, 50.99%, and 28.42% for 1000-grain weight, kernel weight per spike, and dry matter content. Furthermore, variations in high-parent heterosis were also observed when the sterile lines remained the same, but the restorer lines differed in the hybrid combinations. For example, higher high-parent heterosis of 1000-grain weight and kernel weight per spike was noted in the hybrid combination with JiR107 and 0–30 as parents compared to other combinations. In contrast, negative heterosis was seen in the hybrid combination with JiR107 as the parent for RuBP carboxylase and sucrose phosphate synthase. Similarly, differences in the expression of hybrid dominance were observed when the restorer lines were the same, but the sterile lines differed. Among the 36 cross combinations, most showed significant mid-parent and high-parent heterosis levels, with 1000-grain weight, kernel weight per spike, and dry matter content being the most prominent.
Delineation of parental carbon metabolising enzyme content heterozygous dominant groups
Results of the cluster analysis of four leaf carbon metabolism enzyme contents of 12 parents of hybrid sorghum are in Fig. 5, at Euclidean Distance (ED) = 0.0005, the ED based cluster analysis divided the 12 sorghum parents into three classes: the first class contained four parents, Ji2055A, I15A, 501R, and L407A, which had higher sucrose synthase; the second class also contained four parents, TAM428A, T40, 170A, and Ji318R, which had a more mediocre performance; and the third category also contained four parents, 521A, Nan133, 0–30, and JiR107, which had a high level of RuBP carboxylase.
Correlation analysis of photosynthetic traits, carbon metabolising enzymes, and yield traits in hybrid sorghum
Fig. 6 shows significant positive correlations among yield traits of hybrid sorghum, such as grain weight per spike, 1000-grain weight, and dry matter content. These traits also showed substantial positive correlations with Pn, WUE, Gs, PEP carboxylase, and RuBP carboxylase. Additionally, Pn positively correlated with RuBP carboxylase, PEP carboxylase, Tr, Ci, and Gs (P < 0.01). RuBP carboxylase was positively correlated with PEP carboxylase and sucrose synthase, while Ci showed a highly significant negative correlation with AMC. In hybrid sorghums, RuBP carboxylase efficiency, carbon absorption, stress tolerance, resource allocation, and yield are all improved by positive correlations between PEP carboxylase and sucrose synthase. Because of the enhanced grain filling, these advantages help to increase crop quality and yields.
Discussion
Photosynthesis is crucial in determining plant growth and productivity, with approximately 95% of plant dry matter originating from the photosynthetic assimilation of CO2 (Xu 1999). Drought-tolerant sorghum effectively absorbs CO2 and transforms it into glucose, boosting biomass and photosynthesis. High biomass and grain yields from its effective CO2 absorption contribute to food security and the development of biofuels. During the flowering stage of sorghum, photosynthesis is of utmost importance for overall growth and can impact biomass at maturity and seed yield (Tollenaar et al. 2004; Ni et al. 2009). Previous research by the author demonstrated that the leaf photosynthetic physiological and metabolic capacity of the sorghum hybrid Jiza319 was significantly higher than that of its parent plants, highlighting its photosynthetic dominance (Zhao and Zhou 2020). The current experiment revealed significant heterosis in photosynthetic traits among 36 hybrid sorghums at anthesis, with characteristics such as Pn, Tr, Gs, and AMC showing notable high-parent heterosis.
Moreover, a strong positive correlation was observed between Pn, Tr, and Gs, suggesting that Tr and Gs could serve as indicators for identifying materials with high photosynthetic rates. Specifically, the progeny from crosses between sterile lines Ji2055A, 521A, and I15A exhibited higher net photosynthetic rates and more significant high-parent heterosis than those from crosses involving sterile lines 170A and L407A. Additionally, the net photosynthetic rates of Ji2055A, 521A, and I15A were significantly higher than those of 170A and L407A. Similarly, the progeny from crosses between restorer lines Nan133 and 0–30 displayed significantly higher net photosynthetic rates than from crosses involving 170A and L407A. Overall, the progeny from crosses between restorer lines Nan133 and 0–30 exhibited a higher parental advantage in Pn than hybrid sorghum with 501R as the restoration line. The findings of Zhang et al. (1998) and other researchers support the idea that the photosynthetic capacity of both parent plants plays a significant role in determining the dominance of photosynthetic traits in hybrid offspring. As a result, selecting lines with higher net photosynthetic rates as either sterile or restored lines could be a viable strategy for developing highly dominant sorghum hybrid combinations.
Carbon metabolism is a critical process that reflects plants’ physiological status and metabolism (Sinha and Khanna 1975). Exploring parental traits’ impact on offspring’s carbon metabolism is crucial for achieving high sorghum yields (Yang et al. 2020; Jangra et al. 2022). Key enzymes such as RuBP carboxylase, sucrose synthase, sucrose phosphate synthase, and PEP carboxylase play crucial roles in the photosynthetic pathway of plants, influencing carbon uptake and utilisation. RuBP carboxylase, in particular, is a multifunctional enzyme involved in CO2 fixation and the regulation of the photorespiratory pathway (Leegood 2016; Yuan et al. 2019; Bauwe 2022; Clapero et al. 2023) found that in wheat (Triticum aestivum), the activity of RuBP carboxylase in F1 hybrid generations was higher than in maintained lines but lower than in sterile lines during seedling and flowering stages. However, there was no difference in RuBP carboxylase activity among materials during the nodulation stage. Their results indicated that RuBP carboxylase activity in hybrid sorghum F1 generations was significantly higher than in the two parent lines at anthesis, showing significant high-parent heterosis. This is consistent with Wu et al. (1985) findings that sorghum hybrids exhibit heterosis in RuBP carboxylase and PEP carboxylase activities throughout the sorghum life cycle. Correlation analysis revealed that RuBP carboxylase and PEP carboxylase positively correlate with net photosynthetic rate, suggesting that higher activity of these enzymes may lead to increased photosynthetic utilisation. Elevated enzyme activity levels in hybrid sorghums facilitate photosynthetic utilisation using RuBisCO, PEP carboxylase, and sucrose synthase. These endeavours optimise the utilisation of resources, foster superior development and output, fortify resilience to stress, and sustain photosynthetic rates and energy reserves. Furthermore, most hybrid progeny derived from sterile lines Ji2055A and I15A or restorer T40 displayed mesophilic or high-parent heterosis in RuBP carboxylase and PEP carboxylase activities.
The formation of crop yield is closely related to organ differentiation, development, and the distribution and accumulation of photosynthetic products. Understanding this formation process is essential for implementing advanced cultivation techniques, conducting reasonable regulation, and achieving high yields (Tollenaar et al. 2004; Xing and Zhang 2010). The yield traits of the 36 hybrid combinations tested exhibited significant high-parent heterosis, particularly in 1000-grain weight and grain weight per spike, with average high-parent heterosis values of 30.21% and 30.54%, respectively. The average high-parent heterosis for dry matter content was also notable at 16.84%. The 1000-grain weight values demonstrated the highest high-parent heterosis value at 82.54%. Additionally, most combinations displayed positive high-parent heterosis values for 1000-grain weight, grain weight per spike, and dry matter content. Aisawi et al. (2015) found that increasing biomass can enhance yield, while Tausz-posch et al. (2015) highlighted that biotic yield is a crucial agronomic trait influencing wheat yield potential. They noted that the increase in biomass is primarily due to higher photosynthetic rate and Gs, findings that align with the results of this study. Correlation analysis indicated a strong positive correlation (P < 0.01) between the grain weight of a single spike and 1000-grain weight, dry matter content, and Pn in hybrid sorghum. A greater Pn during flowering increases yields because it produces more energy, improves biomass accumulation, strengthens the sink, sustains growth during critical times, and correlates with enhanced yield attributes like grain weight and dry matter content. This suggests that the increase in Pn during flowering is closely associated with higher yield at maturity and that Pn during flowering could be a valuable indicator for identifying superior hybrid combinations. Furthermore, the correlation analysis between Pn and carbon metabolism enzymes revealed a positive correlation with RuBP carboxylase and PEP carboxylase (P < 0.01), indicating that enhancing these enzymes could increase sorghum yield. Analysis of RuBP carboxylase and PEP carboxylase in hybrid sorghum and its parents showed a significant positive correlation, supporting the idea that using carbon-efficient sorghum lines as parents for hybrid combinations can be a successful strategy for developing new carbon-efficient sorghum varieties.
This study focused on measuring the photosynthetic rate and carbon metabolising enzyme content, specifically at the flowering stage. Further research is needed to investigate the relationship between carbon metabolising enzyme content and photosynthetic rate throughout the entire sorghum reproductive period. The study conducted a comparative analysis by combining photosynthetic performance, enzyme activity, and yield performance in the field but did not delve deeply into the mechanism of hybrid dominance. For robust, high-yielding hybrids, sorghum hybridisation strategies include superior parent selection, controlled hybridisation, genomic selection, backcross breeding, phenotypic selection, enhanced agronomic practices, biotechnological approaches, hybrid maintenance, and integrated breeding programs. Exploring the molecular basis for forming photosynthetic and yield advantages is essential.
Conclusion
The F1 generation of sorghum hybrids exhibits a notable photosynthetic advantage during anthesis. There is a highly significant and positive correlation between the Pn and factors such as 1000-grain weight at maturity, grain weight of a single spike, and dry matter content. These correlations can serve as a valuable reference for early screening of high-yielding varieties with dominant solid combinations. Additionally, they can aid in making simple predictions regarding the yield potential of hybrid combinations. When considering changes in the activities of RuBP carboxylesterase and PEP carboxylesterase, these results provide a theoretical basis for selecting carbon-efficient sorghum varieties.
Data availability
All data generated or analysed during this study are included in the manuscript. A preprint version of this article is available at https://doi.org/10.21203/rs.3.rs-3481811/v1.
Declaration of funding
This research was funded by the Jilin Agricultural Science and Technology Innovation Project (CXGC2024ZD008) of Ziyang Zhou.
Author contributions
All authors contributed to the design and methodology of this study, the assessment of the outcomes, and the writing of the manuscript.
References
Aisawi KAB, Reynolds MP, Singh RP, Foulkes MJ (2015) The physiological basis of the genetic progress in yield potential of CIMMYT spring wheat cultivars from 1966 to 2009. Crop Science 55, 1749-1764.
| Crossref | Google Scholar |
Bauwe H (2022) Photorespiration–Rubisco’s repair crew. Journal of Plant Physiology 280, 153899.
| Crossref | Google Scholar |
Brown HR, Bouton JH (1993) Physiology and genetics of interspecific hybrids between photosynthetic types. Annual Review of Plant Physiology and Plant Molecular Biology 44, 435-456.
| Crossref | Google Scholar |
Cakmak I, Kirkby EA (2008) Role of magnesium in carbon partitioning and alleviating photooxidative damage. Physiologia Plantarum 133, 692-704.
| Crossref | Google Scholar | PubMed |
Clapero V, Arrivault S, Stitt M (2023) Natural variation in metabolism of the Calvin-Benson cycle. Seminars in Cell & Developmental Biology 155, 23-36.
| Crossref | Google Scholar |
Follett RF (2001) Soil management concepts and carbon sequestration in cropland soils. Soil and Tillage Research 61, 77-92.
| Crossref | Google Scholar |
Fu D, Xiao M, Hayward A, Fu Y, Liu G, Jiang G, et al. (2014) Utilization of crop heterosis: a review. Euphytica 197, 161-173.
| Crossref | Google Scholar |
Fu J, Wang Y, Yang WB, Wang YT, Li BY, Wang FH, et al. (2022) Effects of alternate wetting and drying irrigation and nitrogen coupling on grain filling physiology and root physiology in rice. Acta Agron Sin 49, 808-820.
| Google Scholar |
Gou BD, Duan PP, Yang N, Zhao SF, Wang YF, Zhang GY, et al. (2021) Heterosis analysis of photosynthetic parameters of pepper seedlings responding to low temperature and low light stress. Acta Agriculturae Zhejiangensis 33, 429.
| Google Scholar |
Hall AJ, Richards RA (2013) Prognosis for genetic improvement of yield potential and water-limited yield of major grain crops. Field Crops Research 143, 18-33.
| Crossref | Google Scholar |
Huang X, Yang S, Gong J, Zhao Q, Feng Q, Zhan Q, et al. (2016) Genomic architecture of heterosis for yield traits in rice. Nature 537, 629-633.
| Crossref | Google Scholar | PubMed |
Jangra M, Devi S, Satpal , Kumar N, Goyal V, Mehrotra S (2022) Amelioration effect of salicylic acid under salt stress in Sorghum bicolor L. Applied Biochemistry and Biotechnology 194, 4400-4423.
| Crossref | Google Scholar | PubMed |
Li CX, He Z, Xu YH, Chen Z, Li D, Wang H, Zhang Z (2017) Seasonal changes of photosynthetic characteristics in farmland ginseng leaves under different shades. Journal of Jilin Agricultural University 39, 32-48.
| Google Scholar |
Li JL, Yang L, Ruan RW, Li ZA (2021a) Analysis of photosynthetic characteristics of hybrid wheat at seedling stage and its use for early prediction of strong heterosis combinations. Scientia Agricultura Sinica 54, 4996-5007.
| Google Scholar |
Li S, Liu M, Liu F, Zou J, Lu X, Diao X (2021b) Current status and future prospective of sorghum production and seed industry in China. Scientia Agricultura Sinica 54, 471-482.
| Google Scholar |
Li Y, Yin M, Li L, Zheng J, Yuan X, Wen Y (2022) Optimized potassium application rate increases foxtail millet grain yield by improving photosynthetic carbohydrate metabolism. Frontiers in Plant Science 13, 1044065.
| Crossref | Google Scholar | PubMed |
Liu HM, Zhou XY, Chen J, Li HL, Qiu YB, Liu JF (2014) Heterosis analysis of photosynthetic characteristics in indica hybrid rice. Acta Agriculturae Boreali-Sinica 29, 122-127.
| Google Scholar |
McCormick RF, Truong SK, Sreedasyam A, Jenkins J, Shu S, Sims D, et al. (2018) The Sorghum bicolor reference genome: improved assembly, gene annotations, a transcriptome atlas, and signatures of genome organization. The Plant Journal: For Cell and Molecular Biology 93, 338-354.
| Crossref | Google Scholar | PubMed |
Ni Z, Kim E-D, Ha M, Lackey E, Liu J, Zhang Y, et al. (2009) Altered circadian rhythms regulate growth vigour in hybrids and allopolyploids. Nature 457, 327-331.
| Crossref | Google Scholar | PubMed |
Oguz MC, Aycan M, Oguz E, Poyraz I, Yildiz M (2022) Drought stress tolerance in plants: interplay of molecular, biochemical and physiological responses in important development stages. Physiologia 2, 180-197.
| Crossref | Google Scholar |
Reynolds MP, Pask AJD, Hoppitt WJE, Sonder K, Sukumaran S, Molero G, et al. (2017) Strategic crossing of biomass and harvest index—source and sink—achieves genetic gains in wheat. Euphytica 213, 257.
| Crossref | Google Scholar |
Saithalavi KM, Bhasin A, Yaqoob M (2021) Impact of sprouting on physicochemical and nutritional properties of sorghum: a review. Journal of Food Measurement and Characterization 15, 4190-4204.
| Crossref | Google Scholar |
Sharma D, Sanghera GS, Sahu P, Sahu P, Parikh M, Sharma B, et al. (2013) Tailoring rice plants for sustainable yield through ideotype breeding and physiological interventions. African Journal of Agricultural Research 8, 5004-5019.
| Google Scholar |
Shehzad T, Okuno K (2020) Genetic analysis of QTLs controlling allelopathic characteristics in sorghum. PLoS ONE 15, e0235896.
| Crossref | Google Scholar | PubMed |
Sinha SK, Khanna R (1975) Physiological, biochemical, and genetic basis of heterosis. Advances in Agronomy 27, 123-174.
| Crossref | Google Scholar |
Tausz-Posch S, Dempsey RW, Seneweera S, Norton RM, Fitzgerald G, Tausz M (2015) Does a freely tillering wheat cultivar benefit more from elevated CO2 than a restricted tillering cultivar in a water-limited environment. European Journal of Agronomy 64, 21-28.
| Crossref | Google Scholar |
Tollenaar M, Ahmadzadeh A, Lee EA (2004) Physiological basis of heterosis for grain yield in maize. Crop Science 44, 2086-2094.
| Crossref | Google Scholar |
Wu GY, Huang QW, Wu XY (1985) Photosynthetic characteristics and hybrid dominance of sorghum. Plant Physiology Journal 11, 217-226.
| Google Scholar |
Wu A, Brider J, Busch FA, Chen M, Chenu K, Clarke VC, et al. (2023) A cross-scale analysis to understand and quantify the effects of photosynthetic enhancement on crop growth and yield across environments. Plant, Cell & Environment 46, 23-44.
| Crossref | Google Scholar |
Xing Y, Zhang Q (2010) Genetic and molecular bases of rice yield. Annual Review of Plant Biology 61, 421-442.
| Crossref | Google Scholar | PubMed |
Xiong J, Hu K, Shalby N, Zhuo C, Wen J, Yi B, et al. (2022) Comparative transcriptomic analysis reveals the molecular mechanism underlying seedling biomass heterosis in Brassica napus. BMC Plant Biology 22, 283.
| Crossref | Google Scholar |
Xu DQ (1999) Photosynthetic rate, photosynthetic efficiency, and crop yield. Chinese Bulletin of Biology 34, 8-10.
| Google Scholar |
Xu C, Zhang LH, Zhao HX, Yan WP, Liu XL, Sun N, et al. (2022) Characteristics of grain formation and leaf response to light and CO2 in semi-arid region under different cultivation patterns. Agricultural Research in the Arid Areas 40, 1-10.
| Google Scholar |
Yang Z, Li J-L, Liu L-N, Xie Q, Sui N (2020) Photosynthetic regulation under salt stress and salt-tolerance mechanism of sweet sorghum. Frontiers in Plant Science 10, 1722.
| Crossref | Google Scholar | PubMed |
Yuan K, Zhang T, Shi X, Xing C, Li N, Gao Q (2019) Comparison of chloroplast DNA and RuBP carboxylase (rubisco) activity with K, V, T-type cytoplasmic male-sterile wheat lines. Acta Botanica Boreali-Occidentalia Sinica 39, 472-479.
| Google Scholar |
Zhang QD, Lu CM, Zhang SP, Zhang QF (1998) The comparison of photosynthetic functions among hybrid rice and their parents in some heterosis and nonheterosis hybrid combinations. Chinese Bulletin of Botany 15, 51-56.
| Google Scholar |
Zhao RJ, Zhou ZY (2020) Comparison of photosynthetic parameters of sorghum hybrid Jiza 319 and its parents. Northeast Agricultural Science 45, 9-12.
| Google Scholar |