Overexpression of forage millet (Setaria italica) SiER genes enhances drought resistance of Arabidopsis thaliana
Hanjing Dai A # , Xiaoyi Huang A # , Yingrun Wang A , Shoujing Zhu A , Jieqin Li A B , Zhaoshi Xu C * and Jiacheng Zheng
A
B
C
Abstract
ERECTA (ER) is a type of receptor-like kinase that contributes a crucial mission in various aspects of plant development, physiological metabolism, and abiotic stresses responses. This study aimed to explore the functional characteristics of the SiER family genes in millet (Setaria italica L.), focusing on the growth phenotype and drought resistance of Arabidopsis overexpressed SiER4_X1 and SiER1_X4 genes (SiERs). The results revealed that overexpression of SiER4_X1 and SiER1_X4 genes in Arabidopsis significantly enhanced the leaf number, expanded leaf length and width, further promoted the silique number, length and diameter, and plant height and main stem thickness, ultimately leading to a substantial increase in individual plant biomass. Compared to the wild-type (WT), through simulated drought stress, the expression level of SiER genes was notably upregulated, transgenic Arabidopsis seeds exhibited stronger germination rates and root development; after experiencing drought conditions, the activities of antioxidant enzymes (superoxide dismutase and peroxidase) increased, while the levels of malondialdehyde and relative electrical conductivity decreased. These results indicate that overexpression of SiERs significantly enhanced both biomass production and drought resistance in Arabidopsis. The SiER4_X1 and SiER1_X4 genes emerge as promising candidate genes for improving biomass production and drought resistance in forage plants.
Keywords: Arabidopsis thaliana L., biomass production, drought resistance, expression patterns, forage millet, genes structure, PEG6000, SiER genes.
Introduction
With high nutritional value and abundant protein content, millet (Setaria italica) is traditionally considered a dual-purpose crop utilised for both food and fodder. Millet is planted in the arid and semi-arid regions of northern China, and plays a substantial role in dryland ecological agriculture, diversification of food production, and national food security (Lata et al. 2013). Millet has stronger drought resistance and higher water-use efficiency than those of most gramineous crops, including wheat (Triticum aestivum), maize (Zea mays), and sorghum (Sorghum bicolor) (Tang et al. 2017; Shrestha et al. 2023). Consequently, uncovering and understanding the function of drought resistance genes of millet has significant implications for the advancement of crop genetics and development of drought-resistant varieties.
Receptor-like protein kinases (RLKs) are a substantial gene family of plants, these crucial transmembrane proteins are involved in various physiological processes, including organ development, signal transduction, and stress resistance (Yang et al. 2021). Overexpression of the rice (Oryza sativa) leucine-rich repeat (LRR)-RLK family gene OsLRK2 leads to an enhancement in the rice branch number, subsequently improving drought resistance and tiller quantity (Kang et al. 2017). The alfalfa (Medicago sativa) LRR-RLK gene MsSIK1 displays a potent expression in response to dehydration and has a strong correlation with water-use efficiency (Guo et al. 2016). The ERECTA (ER) gene, part of the LRR-RLK family, plays a key mission in regulating the plant development of ovules, leaves, and stomata, as well as in bolstering resistance to biotic and abiotic adversity (Shpak 2013). Overexpression of the grape (Vitis vinifera) VvER gene in Arabidopsis thaliana has been shown to markedly increase the length, width, and number of leaves, slenderise the flower stems, and noticeably enhance silique length and plant height (Liu et al. 2019). The stomatal density of Arabidopsis plants carrying the poplar (Populus spp.) PdER gene has been observed to decrease, leading to improvement in transpiration efficiency, water-use efficiency, and drought tolerance (Li et al. 2021). Furthermore, it has been discovered that the Arabidopsis AtER gene increases transpiration efficiency through affecting the distribution of leaves, stomatal density, and the growth of mesophyll and epidermal cells (Masle et al. 2005). The transgenic maize containing the sorghum SbER2-1 gene demonstrated the better water usage efficiency and enhanced drought resistance under drought environments (Li et al. 2019).
In northern arid regions where water resources are limited, millet serves as an essential fodder crop. Enhancing both the biomass and drought resistance of millet has become an imperative breeding goal, particularly for fodder millet. However, the function of the SiER genes of millet remains less reported. The study offered a comprehensive detection of the SiERs family of millet. SiER4_X1 and SiER1_X4, two SiER genes from millet, were cloned, and their expression patterns were elucidated in various tissues and under drought stress. Following their transformation into Arabidopsis, the agronomic indicators and drought resistance of the SiERs transgenic Arabidopsis were identified. This work lays the theoretical groundwork for more detailed investigations into the functional characteristics of the SiER family.
Materials and methods
Plant material
Fully matured seeds of millet (Setaria italica L.) variety ‘DunGu’ were selected and planted in sandy soil trays. At the embryonic period, embryos and coleoptiles were collected. At the four-leaf stage, some aboveground samples were gathered for the cloning of the target genes, some other plants were uprooted, rinsed with clean water, transiently dried on filter paper, and immediately submerged in a nutrient solution containing 20% PEG6000 (w/v) to simulate drought conditions. Cultivation in clean water served as the control group. The treatment durations were 0 h, 1 h, 2 h, 4 h, 6 h, 12 h, 24 h, 48 h, and 60 h. Root, stem, and leaf samples were combined for expression analysis. For tissue-specific expression analysis, the remaining plants continued to be cultivated. At the flowering stage, tissue samples from flag leaves, stems, roots, flag leaf sheaths, peduncles, and panicles were collected; at the harvest stage, seeds were collected.
cDNA sample preparation
All collected samples were quickly frozen in liquid nitrogen and stored at −80°C. These cryopreserved materials were then ground at low temperatures. Total RNA was prepared from these stored samples using Trizol reagent (AG21102, Aikorui), then processed the genomic DNA (gDNA) removal and transcribed into complementary DNA (cDNA) samples by the BeyoRT III cDNA Synthesis Kit (D7178S, Beyotime), and subsequently stored for future use.
Sequence analysis of millet SiERs and overexpression vector construction
Utilising the SbER10_X1 gene (from sorghum (Sorghum bicolor L.); XM_002437978.2) as a probe, two tags of the millet SiER genes were procured from the Phytozome database. These tags were then employed to search the NCBI database and download ER protein sequences from other species. The downloaded sequences were aligned using MEGA7 software, and a phylogenetic tree was subsequently constructed. The protein structure domains of SiER family were analysed using SMART database, and the exon‒intron structure of SiER family was predicted online through the GSDS database by comparing the millet genome in the Phytozome database (Setaria italica ver. 2.2). For details of all database used, see Supplementary Table S1.
From these, the genes SiER4_X1 and SiER1_X4 were selected, and primers SiER4_X1-F3/SiER4_X1-R3 and SiER1_X4-F2/SiER1_X4-R2 were designed for the SiERs isolation. The PCR reaction system was as follows: 1 μL of KOD FX High Fidelity Enzyme (KFX-101, Toyobo); 25 μL of 2 × KOD FX buffer; 10 μL of dNTPs (2 mM); 1.5 μL each of forward and reverse primers (10 mM); 5 μL of cDNA; and double distilled H2O up to a total of 50 μL. The amplification program involved 94°C for 2 min, 40 cycles (denaturation at 98°C for 10 s, annealing at 64°C for 30 s for SiER4_X1 or at 60°C for 30 s for SiER1_X4, and 68°C with subsequent extension for 4 min), followed by a final extension at 68°C for 10 min. PCR products were then ligated to the sequencing vector for sequencing confirmation.
Upon verification of correct sequencing, homology arm primers SiER4_X1-1302F2/SiER4_X1-1302R2 and SiER1_X4-1302F2/SiER1_X4-1302R2 were used to insert the SiER4_X1 and SiER1_X4 fragments into the pCAMBIA1302 expression vector (CaMV35S promoter), respectively. The resulting fusion expression vectors (pCAMBIA1302-SiER4_X1 and pCAMBIA1302-SiER1_X4) were then transformed into Agrobacterium tumefaciens EHA105. Details of all primers used are in Table S2.
Expression patterns analysis of millet SiERs
In accordance with real-time quantitative PCR (qPCR) requirements, specific primers were designed for the SiER4_X1 and SiER1_X4 genes: SiER4_X1-qRTF3/SiER4_X1-qRTR3, SiER1_X4-qRTF3/SiER1_X4-qRTR3, and for the reference gene SiActin (NM_001280818.1): SiActin-qRTF1/SiActin-qRTR1. The ABI Prism 7500 real time fluorescence qPCR instrument (Applied Biosystems, Waltham, USA) was used to detect the relative expression levels of the target genes. To guarantee the results correctness, each experiment was carried out with three technical replicates and three biological replicates. The reaction systems and amplification programs were used in accordance with procedures of Liu et al. (2013), the details of all primers and annealing temperature used are in Table S2.
Phenotype analysis and drought tolerance identification of transgenic Arabidopsis
The floral dip method (Clough and Bent 1998) was employed to transform pCAMBIA1302-SiER1_X4 and pCAMBIA1302-SiER4_X1 into Arabidopsis thaliana L. (Columbia-0; wild-type, WT line), to obtain the transgenic seeds in the T0 generation. Seeds from the T1 and T2 generations were successively cultivated on an antibiotic medium (Kan+) to screen positive plants. Using PCR detection, stable overexpressing lines were selected in the T3 generation. The T4 generation was further cultivated on MS medium (without antibiotics) for 7 days, then transferred to nutritional soil for pot planting. On Day 28, leaf count, leaf length and width were recorded. At harvest, plant height, stem thickness, silique length and diameter, and individual plant biomass were measured.
Seeds of WT, SiER4_X1 and SiER1_X4 transgenic Arabidopsis were sterilised and sown onto Murashige and Skoog (MS) media containing varying concentrations of mannitol (0, 75, 100, 150, 200, and 300 mmol L−1). Each Petri dish was divided into three equal parts, and spotted with three lines of Arabidopsis, with 30 seeds each part and three Petri dish replicates in total. The germination rate was recorded after 3 days, and the rate of green plant was recorded after 10 days. The young seedlings were grown on control medium (0 mmol L−1 mannitol) and divided into two groups. In the first group, young seedlings with the similar initial roots were transferred to MS medium (200 mmol L−1 mannitol), while the control was transferred to the empty MS medium. Cultivation continued vertically for 21 days, the root diameter, root length, and root surface area of WT lines and transgenic Arabidopsis were analysed using a root scan system (Regent instruments, Canada) (n = 20). In the second group, seedlings with uniform growth were transferred to flower pots (8 cm × 10 cm), with each pot containing four plants and 10 pots for each line. On Day 25 of greenhouse cultivation, watering was halted, and drought stress treatment was applied for 12 days. Samples were prepared to determine the malondialdehyde (MDA) concentration, activity of antioxidant enzyme (superoxide dismutase, SOD; peroxidase, POD), and relative electrolytic conductivity (Zheng et al. 2020). Watering was resumed after drought stress. The control group received well watering. The culture conditions were set at a temperature of 24°C, humidity of 40–60%, a light/dark cycle of 16 h/8 h with the light intensity of 525 μmol m−2 s−1.
Data processing and statistical analysis
Excel 2020 software was used to collate the agronomic traits-related data, SPSS 25.0 software was used for data statistical analysis, and Origin 2018 software was used for graphing. The 2−ΔΔCt method was utilised for the processing of qPCR data (Liu et al. 2013).
Results
Cloning and evolutionary analysis of the millet SiER family
A phylogenetic tree was constructed to distinctly divided ER proteins from various species into four classes (Fig. 1). Classes I and II are made up entirely of monocotyledonous plants. Class I includes ER proteins from wheat, maize, sorghum, barley (Hordeum vulgare), Brachypodium, and Aegilops, as well as two copies of the millet SiER4 family, with the SiER4 family more closely linking to sorghum and maize. The six copies of the SiER1 family and the rice ER protein make up Class II, SiER1_X3 and SiER1_X4 are the closer cousins. Class III includes the ER family from soybeans (Glycine max), grapes, and Arabidopsis. Class IV consists of millet SiERL4 and Arabidopsis AtERL. This suggests that in the evolution of different species, the SiER1 family, located on the first chromosome of millet, has a phylogeny closer to modern paddy aquatic crops, while the SiER4 family on the fourth chromosome is closer to field xerophytic crops. Two genes, SiER4_X1 and SiER1_X4, were selected to isolate target fragments. The open reading frames of SiER4_X1 and SiER1_X4 were found to be 2991 bp and 2973 bp, respectively, and encoding 996 and 990 amino acid residues, respectively.
Phylogeny and sequence characteristics of millet SiER family genes. (a) Phylophytic tree of SiER family proteins versus ER proteins of other species, numbers at the branch node represent the value of 1000 bootstraps. Plant species and NCBI accession numbers of proteins in phylogenetic tree are listed in Table S3. (b) PCR amplification of SiERs genes: M, DL15000 marker; 1, SiER1_X4; 2, SiER4_X1. (c) Exon–intron structure of the millet SiER family genes. The red boxes indicate the selective genes. UTR, untranslated region.
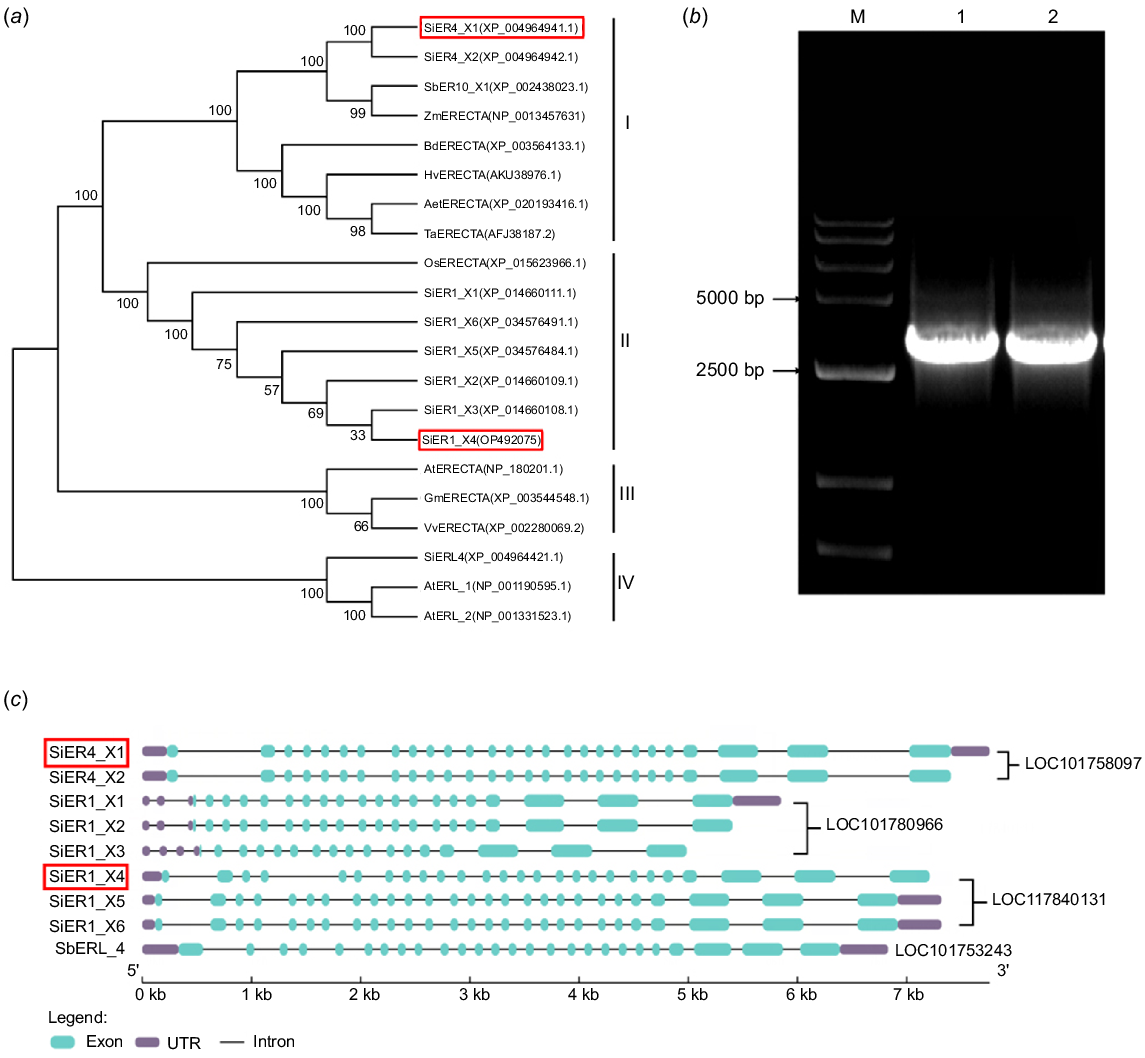
The genomic information and cDNA sequences of nine SiER family genes were obtained from the Phytozome database (Setaria italica ver. 2.2) (Fig. 1). By comparing the nucleotide sequence structure, it was found that the SiER family consisted of four genes, the nucleotide structure is highly conserved, but exon length and number vary, with a longer zone of the 25th, 26th, and 27th exon along the 3′ untranslated region (UTR), which encode the serine/threonine kinase domian of the ER protein. SiER4_X1, SiER4_X2, SiER1_X5, and SiER1_X6 showed 27 exons, while SiER1_X4 has 26 exons, the 2nd exon near the 5′ UTR region is larger and mainly encodes the LRR region of the ER protein. SiER1_X1, SiER1_X2, and SiER1_X3 displayed 20, 20, and 17 exons, respectively, with a shorter sequence of the 1st exon near the 5′ UTR region. The longest SiER4 sequence within the SiER family is produced by the bigger first intron of the SiER4 family, SiERL4 is a distinct branch with its 25 introns. The amino acid sequence comparison showed that the similarity of the SiER family reaches 82.93%. The sequences of the LRR region at the N-terminal and the kinase region at the C-terminus are conserved, but the transmembrane region is less conserved, while the conservation of the signal peptide region at the N-terminal and UTR region at the C-terminus is least pronounced (Fig. S1).
Expression patterns of SiER genes under simulated drought induction
As shown in Fig. 2, under simulated drought conditions with 20% PEG6000, the expression levels of SiER4_X1 and SiER1_X4 remained relatively stable in their respective normal growth condition. However, in the drought treatment samples, their expression significantly increased, the initial expression levels of the two genes were significantly induced, with their expression levels rising as the treatment time extended. Both genes reached their peak expression levels at 6 h, being 4.8-times and 12.1-times higher to the controls, respectively. As the treatment time continuously extended, the expression levels decreased noticeably but remained above the control levels. This suggests that the SiER4_X1 and SiER1_X4 genes possess a response mechanism to drought stress.
SiERs genes expression under 20% PEG6000 treatment. Roots, stems, and leaves were pooled to sample for the expression analysis of target genes under drought stress. The primers of the target genes (SiER1_X4 and SiER4_X1) and reference gene (SiActin) are in Table S2. The 2−ΔΔCt method was utilised for the processing of qPCR data. Bar, s.e. (n = 9).
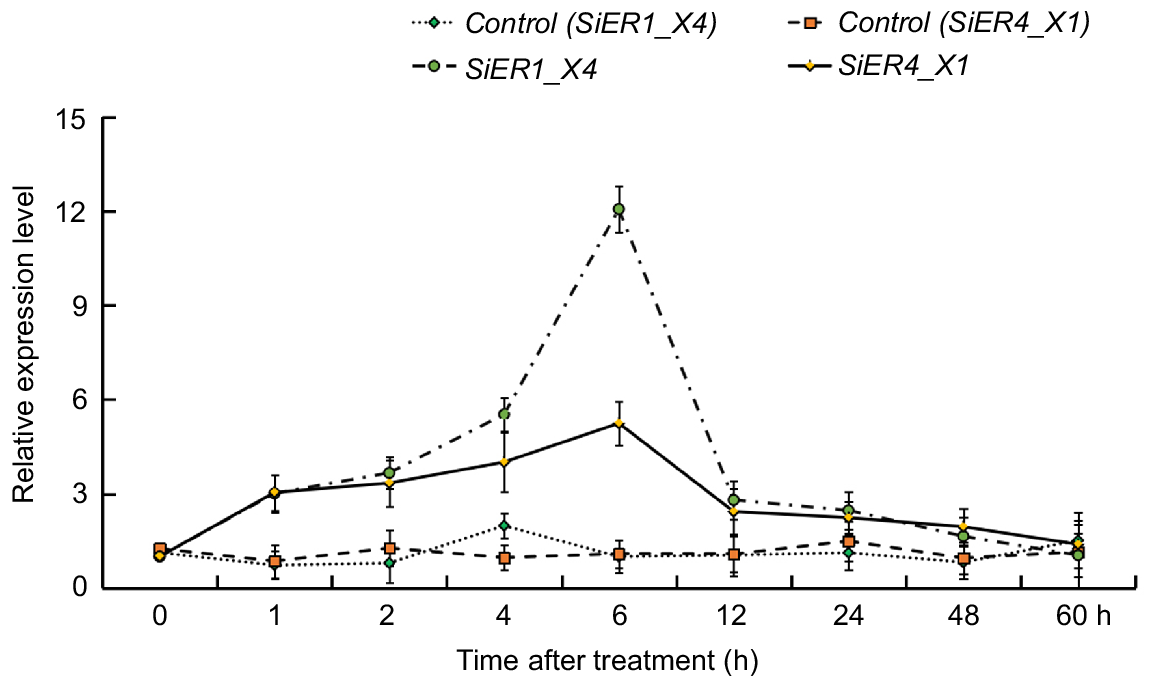
Tissue-specific expression of SiER genes
In various tissues, the expression levels of the SiER4_X1 and SiER1_X4 were higher in above-ground tissues and lower in subterranean roots (Fig. 3). In vegetative organs, the expression levels of SiER4_X1 and SiER1_X4 were more abundant in the embryos, coleoptiles, and stems, significantly exceeding those in the roots. In reproductive organs, the expression levels of both genes reached their peak in the peduncles, at 70-times and 61-times higher to the root level, respectively. This suggests that SiERs differentially modulate the diverse organs development in millet, implying the specialised regulator networks. Notably, SiER4_X1 exhibits substantial transcription prominence over SiER1_X4 across multiple tissues.
Expression level of SiERs in different tissues of millet. Embryos and coleoptiles were collected at the embryonic period. Roots, stems, flag leaves, flag leaf sheaths, peduncles, and panicles were collected at the flowering stage, as well as seeds at harvest stage. The primer details and qPCR data processing were the same as in Fig. 2. Blue and red bars indicate the expression level of target genes at the lowest and highest abundance, respectively.
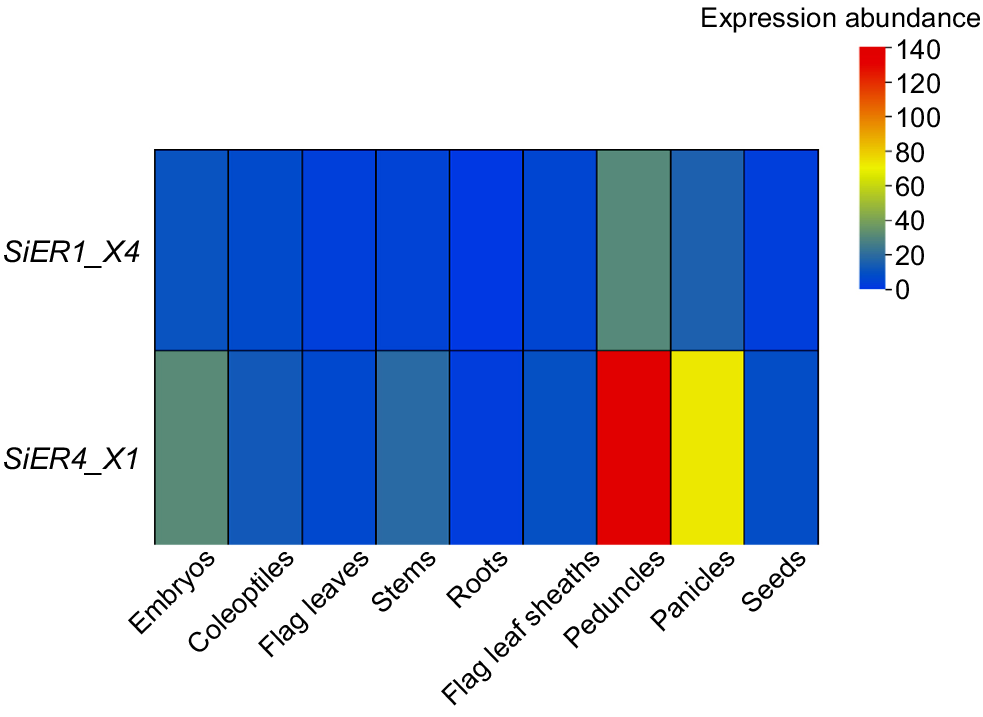
Phenotypic identification of transgenic Arabidopsis with SiER genes
Upon examining 4-week-old Arabidopsis plants (WT, overexpression lines of OxSiER4_X1#13 and OxSiER1_X4#3) (Fig. 4), referred to WT, the rosette leaves of both overexpression lines were larger, nearly elliptical, with relatively extended petioles. At maturity, the siliques of both overexpression lines were more longer and substantial, and the plant height increased, resulting in a higher biomass for the transgenic lines than for the WT. Data revealed that the lengths of the OxSiER4_X1#13 and OxSiER1_X4#3 leaves were 1.51-times and 1.47-times that of the WT leaves, respectively, and the leaf widths were 1.24-times and 1.22-times that of the WT leaves, respectively (Fig. 5). Each overexpression line holds the average 24 and 23 leaves, respectively, exhibiting significant differences from the WT. The main stem thicknesses of OxSiER4_X1#13 and OxSiER1_X4#3 were 1.33-times and 1.52-times that of the WT, respectively, and the silique lengths reached 18.16 mm and 19.03 mm, respectively, with the diameter of the siliques being 0.75 mm for both. The number of siliques reached 132.13 and 129.93, respectively. The plant heights of OxSiER4_X1#13 and OxSiER1_X4#3 were 1.43-times and 1.40-times that of the WT, respectively. These led to the single-plant biomass of the two transgenic lines reaching 1.02 g and 1.01 g, respectively, displaying a remarkable difference between transgenic lines and WT. Therefore, it is evident that overexpression of the SiER4_X1 and SiER1_X4 genes significantly increased the plant biomass of Arabidopsis.
Growth phenotype of WT and transgenic SiERs Arabidopsis. The overexpression vectors of pCAMBIA1302-SiER4_X1 and pCAMBIA1302-SiER1_X4 were transformed into Arabidopsis Columbia-0 (WT), to obtain the transgenic seeds in the T4 generation after antibiotic medium screening. (a) Rosette leaves of 4-week-old plants and the corresponding leaves at different development stages. (b) Arabidopsis siliques at early harvest stage. (c) The whole plants at early harvest stage. (Scale bar = 1 cm).
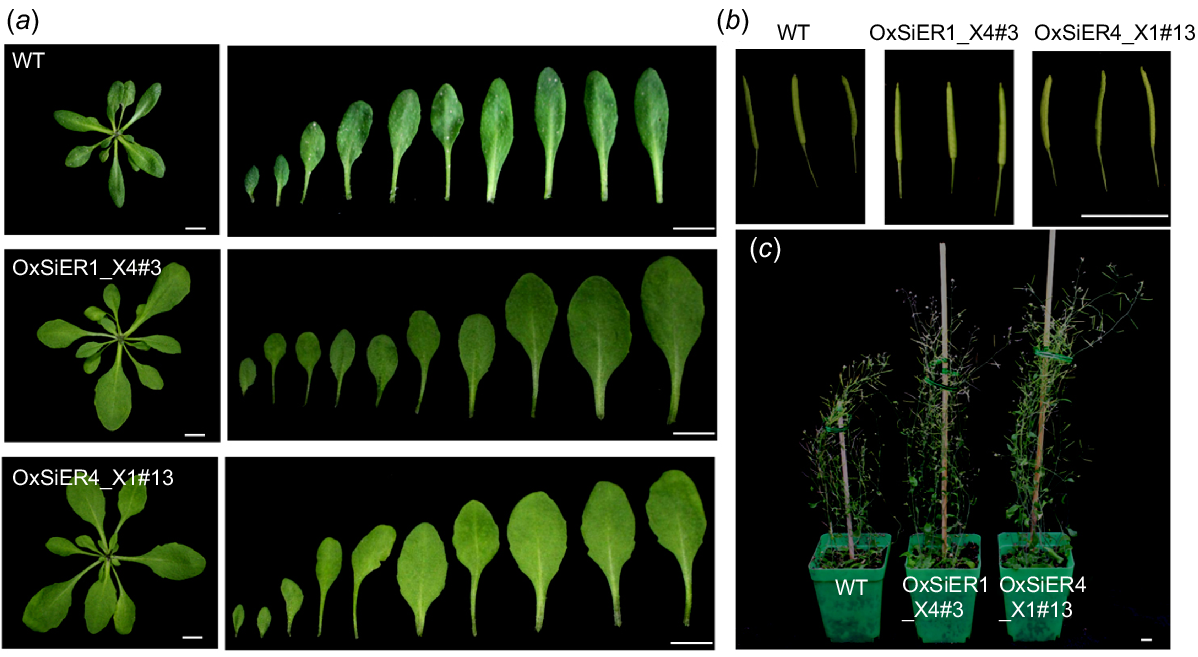
Morphological traits of WT and transgenic SiERs Arabidopsis plants. After the 28 days of Arabidopsis growth, leaf count, leaf length and width were recorded. At harvest, plant height, stem thickness, silique length and diameter, and individual plant biomass were measured. Lowercase letters indicate significant differences between WT and transgenic SiERs plants at P < 0.05.
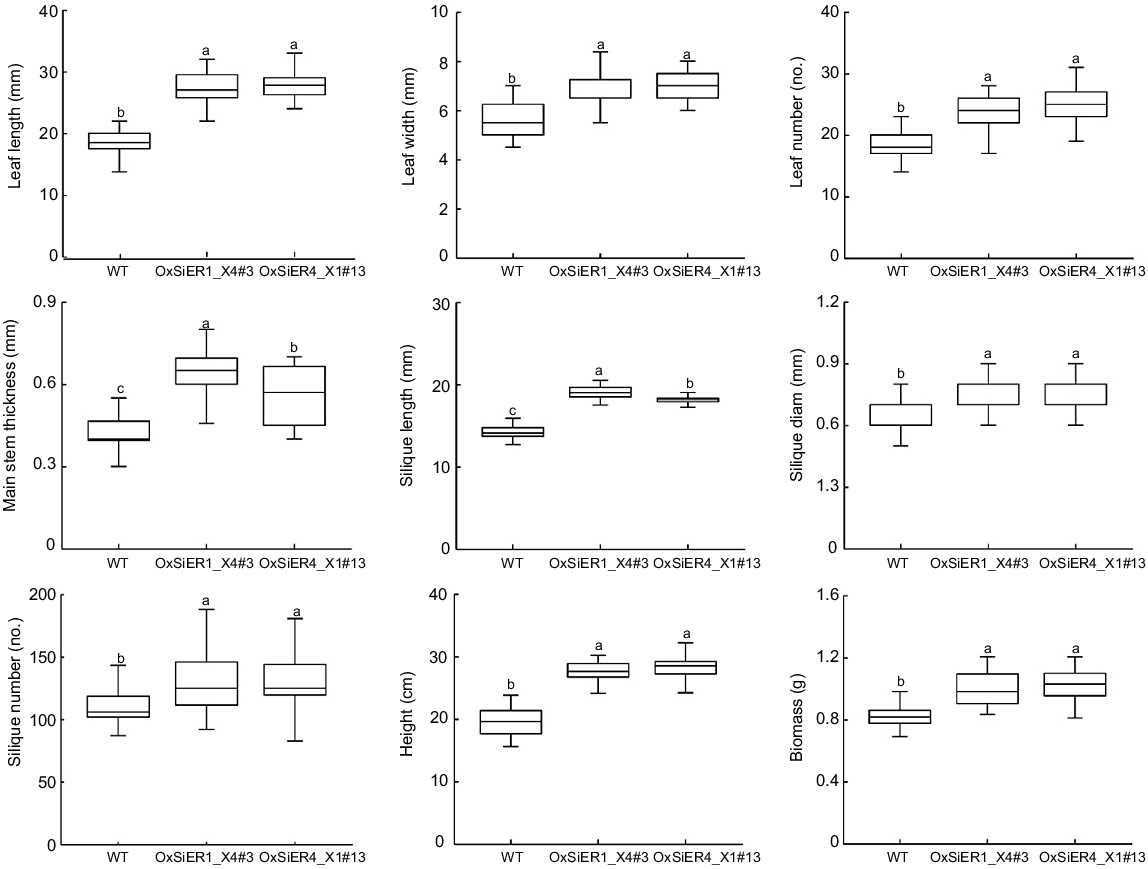
Germination rate, green plant rate, and root analysis of transgenic Arabidopsis with SiER genes under simulated drought stress
As the mannitol concentration increased, the germination and growth of Arabidopsis seeds were suppressed to varying degrees (Fig. 6). Compared to the WT line, under the 150 mmol L−1 treatment, the germination rates of the OxSiER4_X1#13 and OxSiER1_X4#3 lines increased by 1.08-times and 1.17-times, respectively, while the green plant rates increased by 1.11-times and 1.16-times, respectively. Under the 300 mmol L−1 treatment, the germination rates of both transgenic lines increased by 2.86-times and 3.57-times, respectively, and the green plant rates increased by 1.71-times and 2.14-times, respectively, with all differences being statistically significant. Under 200 and 300 mmol L−1 stress, the germination rate of the OxSiER1_X4#3 line was remarkably higher than that of the OxSiER4_X1#13 line. The results suggest that both SiER4_X1 and SiER1_X4 genes can enhance the drought resistance of Arabidopsis, with the regulatory effect of SiER1_X4 being superior to that of SiER4_X1.
Effect of mannitol treatment on germination of WT and transgenic SiERs Arabidopsis. WT and transgenic SiERs Arabidopsis were sown onto MS media containing varying concentrations of mannitol (0, 75, 100, 150, 200, and 300 mmol L−1). The germination rate was recorded after 3 days, as well as the green plant rate after 10 days. (a) Seeds germination of Arabidopsis. (b) Germination rate and green plant rate of Arabidopsis.
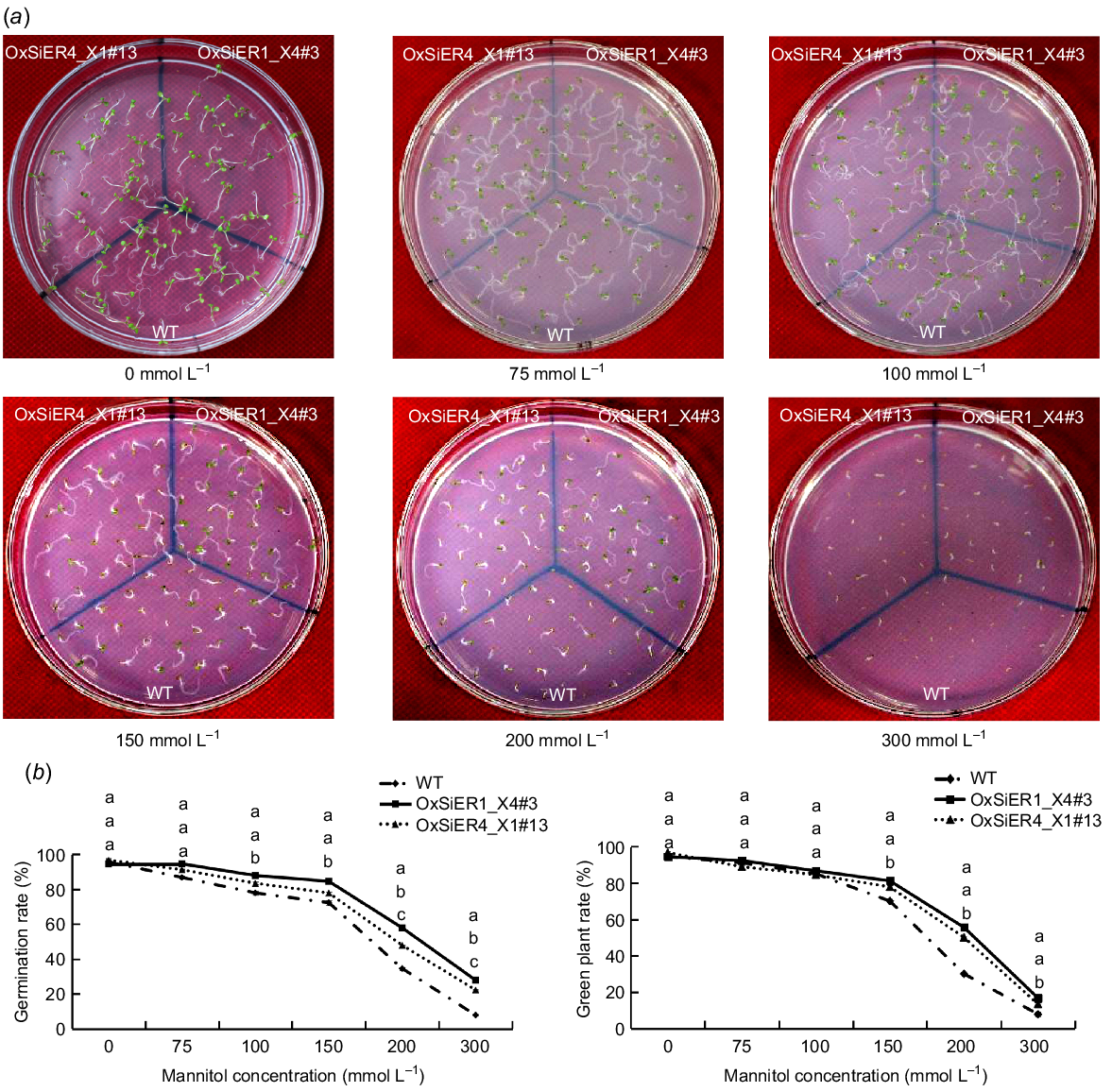
Overexpression of SiER4_X1 and SiER1_X4 significantly promoted root length and root diameter development in Arabidopsis seedlings, while the influence on root surface area was not significant (Fig. 7). Under the control treatment (0 mmol L−1 mannitol), the root lengths of the OxSiER4_X1#13 and OxSiER1_X4#3 lines were 1.03-times and 1.11-times that of the WT, respectively, while the root diameters were 1.17-times and 1.19-times that of the WT, respectively. Under the 200 mmol L−1 treatment, the root lengths of both transgenic lines were 1.17-times and 1.31-times that of the WT, respectively, and the root diameters were 1.53-times and 1.47-times that of the WT, respectively. These indicate that SiER4_X1 and SiER1_X4 can promote root development in Arabidopsis. After drought stress, they maintain a relatively longer, stronger root distribution, enhancing the drought resistance of Arabidopsis.
Effect of mannitol treatment on root development of WT and transgenic SiERs Arabidopsis. The young seedlings with the similar initial roots were transferred to medium containing 200 mmol L−1 mannitol and empty MS. After 21 days, the root length, root surface area, and root diameter were analysed using a root scan system (n = 20).
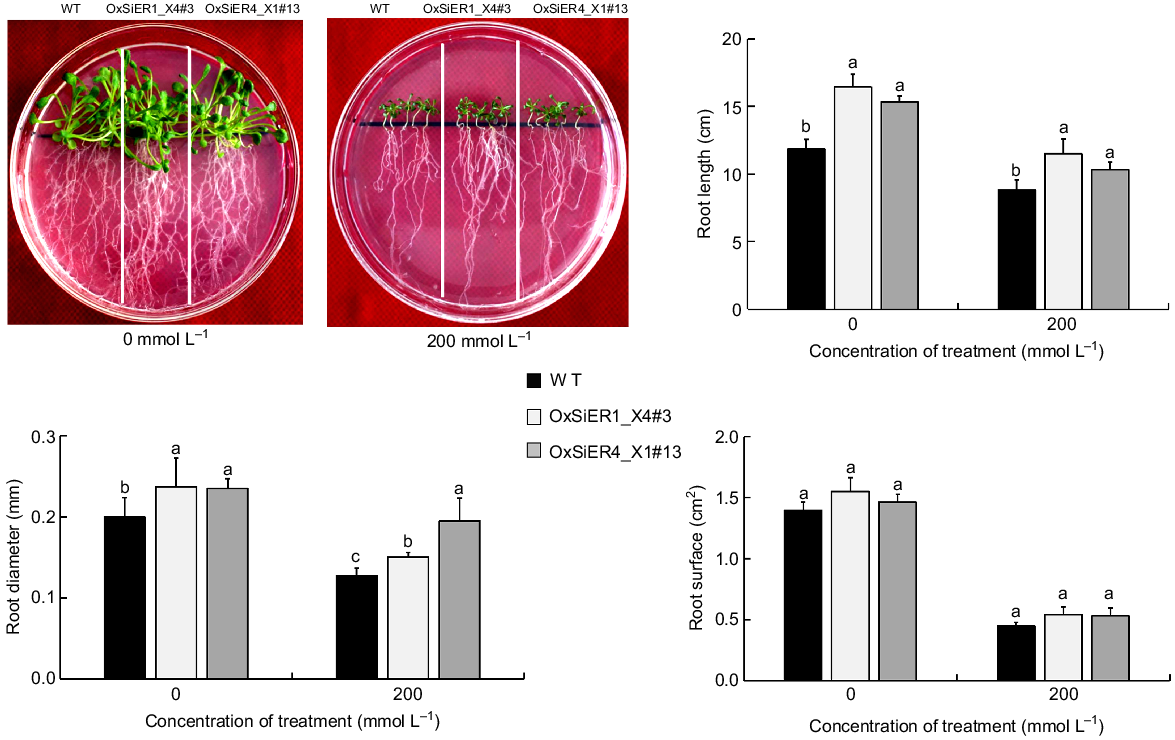
Analysis of drought resistance-related physiological traits of transgenic Arabidopsis with SiER genes
After rewatering Arabidopsis following drought stress, the WT lines wilted severely, with leaves wrinkling and turning purple. However, most transgenic lines survived, with only some leaves wilting, the degree of damage in transgenic lines was remarkably lighter than those of WT, indicating that the drought resistance of transgenic Arabidopsis with SiER genes is stronger than that of the WT lines (Fig. 8). Upon further measurement of drought resistance-related physiological traits, the results showed that under normal watering conditions, the SOD and POD activity, and relative electrical conductivity of the OxSiER4_X1#13 and OxSiER1_X4#3 lines were not significantly different from those of the WT lines, while the MDA concentration was significantly less than those of the WT lines. After drought treatment, the SOD activity in the OxSiER4_X1#13 and OxSiER1_X4#3 lines was 1.40-times and 1.39-times those in the WT plants, respectively, and the POD activity was 1.19-times and 1.23-times, respectively. The MDA concentration of the WT line was 1.73-times and 1.99-times that of OxSiER4_X1#13 and OxSiER1_X4#3, respectively, while the relative electrical conductivity of the WT line was 1.3-times and 1.28-times those of the two transgenic lines, respectively. The differences between the two transgenic lines and WT all reached significant levels, suggesting that transgenic Arabidopsis plants with the SiER genes have stronger drought resistance than WT plants.
Identification of drought resistance in WT and transgenic SiERs Arabidopsis. The young seedlings with the similar development after cultivation for 25 days were selected to halt watering for 12 days, leaves were collected to detect relative conductivity, MDA content, SOD and POD activities, then resuming water. The control group received well watering. (a) Arabidopsis phenotype. (b) SOD and POD activity, MDA content, and relative conductivity of Arabidopsis. Lowercase letters indicate significant difference (P < 0.05) (n = 40).
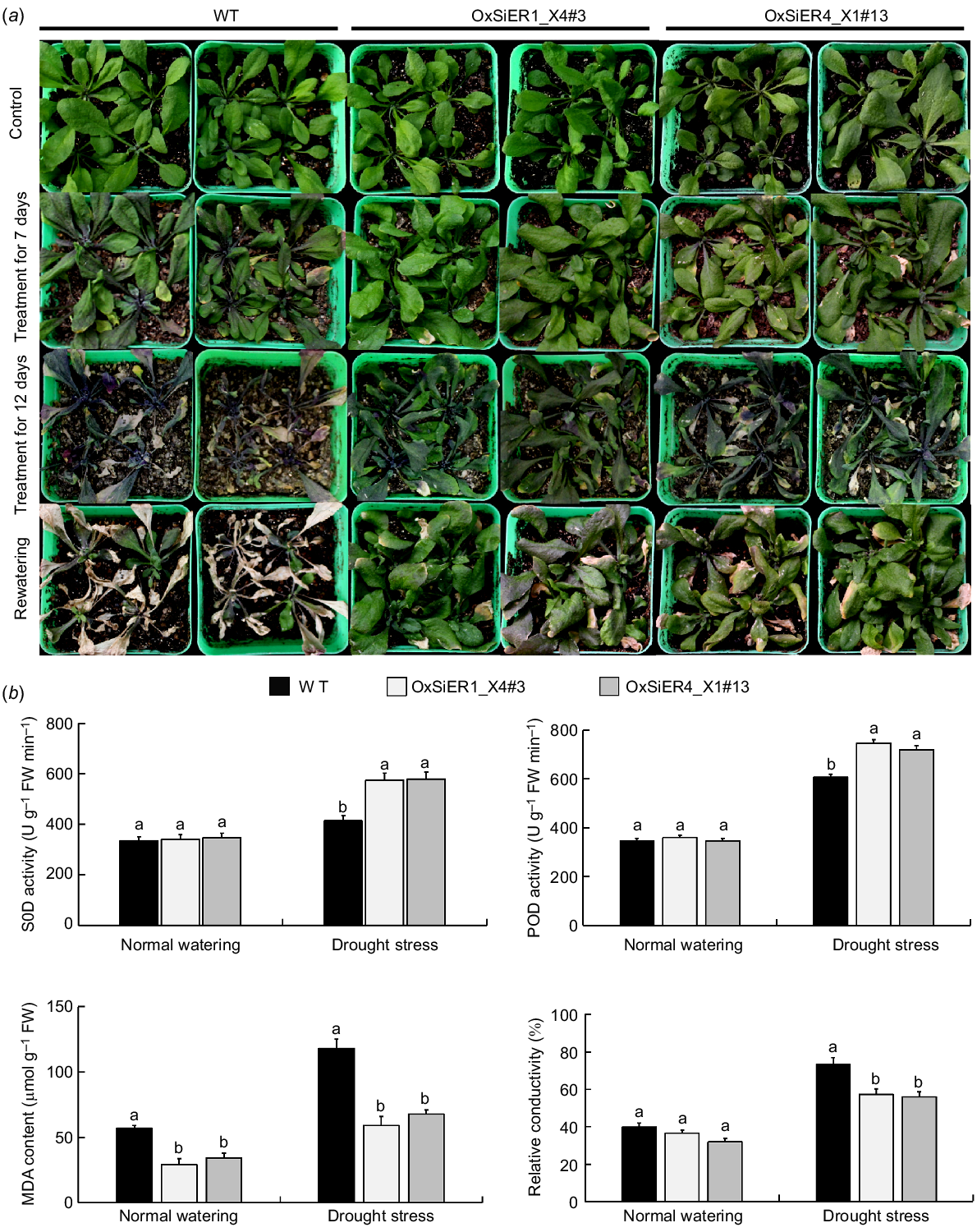
Discussion
Before monocot and dicot plants evolved, the ER family split into the ERL and ER families. The ER and ERL families are multicopy in plants as a result of this evolution and gene duplication events (Liu et al. 2019). Here, four SiER family members were found in the millet genome. Two genes, hosting a total of six copies, are located on the first chromosome, and two genes with three copies are situated on the fourth chromosome. There is great close relatedness between the six copies on the first chromosome and aquatic monocots, such as rice, while the two copies on the fourth chromosome and terrestrial monocots are more closer relations. The genome’s distribution of intron and exon numbers is unequal as a result of the considerable variation in the splice variants of the many SiER family members. This suggests that there is a complex evolutionary process within the millet SiERs. Previous studies have demonstrated that the mechanism of gene family evolution in eukaryotes is the acquisition or removal of introns (Roy and Penny 2006). The expression level of the target genes may be impacted by the number of introns. For example, there is a 500–900-fold decrease in target protein production when the Arabidopsis AtER gene is intron-free (Karve et al. 2011). Evidently, modulation of light conditions provokes a obvious response in transgenic Arabidopsis, which was observed through augmented hypocotyl length, leaf surface area, and petiole extension, particularly when the LRR tandem repeats diminish in the extracellular domain of soybean GmER (Du et al. 2018). This suggests that different splice variants of the SiER family may also lead to significant differences in regulatory function.
SiER genes exhibit a higher transcription level in aboveground millet organs. Notably, they are highly expressed in developing peduncles and panicles, less so in mature seeds, and barely expressed in the root system. ER genes have been found to show significantly higher expression in immature grapes and tomato fruits than in mature fruits (Villagarcia et al. 2012), indicating that SiER genes are essential for the quick tissue growth and differentiation in millet. Overexpression of Arabidopsis AtER gene in transgenic rice and tomato leads to a marked enhancement in their biomass, suggesting a pivotal role for this gene in regulating plant growth. When the AtER gene is deleted, the size of mesophyll cells, peduncle cortical cells, and petal epidermal cells decreases, whereas the number of cells per unit area increases. This suggests that ER can encourage cell enlargement, thereby influencing plant tissue morphology and augmenting plant biomass (Shen et al. 2015). The overexpression of the millet SiER4_X1 and SiER1_X4 genes in Arabidopsis results in a considerable increase in the leaf numbers, leaf area, silique length, and plant height compared to the wild type. This indicates that SiERs can stimulate an increase in plant biomass, which holds significant implications for enhancing the production potential of forage.
The germination stage is critical for plant growth and development, necessitating a substantial amount of water intake. Any stress factor that modifies the environmental water potential can impact seed germination. Traits such as germination rate, seedling establishment rate, and radicle length are instrumental in identifying drought resistance of plant varieties (Xing et al. 2011). Our study revealed that, under regular conditions, overexpression of SiERs markedly enhanced radicle length and diameter growth in Arabidopsis seedlings, with no significant change in the germination and seedling establishment rates. However, under intensely simulated drought stress, these parameters in the overexpressed lines significantly surpass those in the WT lines. Previous studies highlighted that ER genes are potential drought stress candidate genes. For instance, overexpressing the poplar PdER gene in Arabidopsis resulted in elongated primary roots during the seedling stage, a larger leaf area, and improved drought tolerance (Xing et al. 2011). Similar to this, the drought tolerance of Arabidopsis and maize was significantly increased by overexpressing the sorghum SbER2-1 gene, which also increased net photosynthetic rate and water use efficiency (Li et al. 2019).
Simultaneously, overexpressed lines showed increased SOD and POD activities, and reduced MDA concentration and relative electrolytic conductivity following drought stress. Compared to the WT lines, the results imply that transgenic SiERs lines suffered less severe harm to plant growth and development during drought stress. Under drought stress, the reactive oxygen metabolism system is disrupted, producing a vast amount of superoxide anions, amplifying cell membrane peroxidation, and imparting toxicity to plant cells (Du et al. 2013). To counter this, plants activate their antioxidant enzyme system to neutralise reactive oxygen molecules, effectively mitigating damage. The SiERs-overexpressing lines show higher antioxidant enzyme activity under post drought stress, implying the coregulated expression of antioxidant enzyme-encoding genes following SiER overexpression. This coregulation indirectly controls the activity of antioxidant enzymes, fortifying the capability to eliminate reactive oxygen and upholding cell membrane stability.
Conclusion
Overexpression of millet SiER4_X1 and SiER1_X4 genes in Arabidopsis led to increase in leaf length, width, and number, which further promoted the development of silique length, silique number, main stem thickness, and plant height, ultimately resulting in a significant increase in biomass per plant. After experiencing drought stress, the germination rate, green plant rate, and antioxidant enzyme activity of transgenic SiERs Arabidopsis were elevated, suggesting that SiER4_X1 and SiER1_X4 can bolster both the biomass and drought resistance of plants. These genes present substantial potential for use in breeding improvements to enhance drought resistance and biomass production in forage crops.
Data availability
The data in this study will be shared with the corresponding authors if reasonably requested for study.
Declaration of funding
This work was financially supported by the Natural Science Research Programs of Education of Anhui (2022AH051649 and 2023AH051846), the Bengbu Science and Technology Plan Project (2022ny02 and 2023tpt035), the National Natural Science Foundation of China (32372134) and the Collaborative Innovation Programs of Anhui University (GXXT-2023-103).
Author contributions
All authors contributed to the study conception and design. Material preparation, data collection, analysis, writing-original draft were performed by Hanjing Dai and Xiaoyi Huang. Data curation, formal analysis, investigation and methodolog were performed by Yingrun Wang, Shoujing Zhu, Jieqin Li. Conceptualisation, project administration, resources and supervision were performed by Zhao Shi Xu and Jiacheng Zheng.
References
Clough SJ, Bent AF (1998) Floral dip: a simplified method for Agrobacterium-mediated transformation of Arabidopsis thaliana. Plant Journal 16, 735-743.
| Crossref | Google Scholar |
Du YL, Wang ZY, Fan JW, Turner NC, He J, Wang T, Li FM (2013) Exogenous abscisic acid reduces water loss and improves antioxidant defence, desiccation tolerance and transpiration efficiency in two spring wheat cultivars subjected to a soil water deficit. Functional Plant Biology 40, 494-506.
| Crossref | Google Scholar |
Du J, Li Y, Sun X, Yu L, Jiang H, Cao Q, Shang J, Sun M, Liu Y, Shu K, Liu J, Yong T, Liu W, Yang F, Wang X, Liu C, Yang W (2018) Characterization of a splice variant of soybean ERECTA devoid of an intracellular kinase domain in response to shade stress. Journal of Genetics 97, 1353-1361.
| Crossref | Google Scholar | PubMed |
Guo P, Wei H, Zhang W, Yang B, Bao Y (2016) The dehydration-induced ERECTA gene, MsSIK1, from alfalfa improved water use efficiency in transgenic Arabidopsis. Acta Physiologiae Plantarum 38(2), 46.
| Crossref | Google Scholar |
Kang J, Li J, Gao S, Tian C, Zha X (2017) Overexpression of the leucine-rich receptor-like kinase gene LRK2 increases drought tolerance and tiller number in rice. Plant Biotechnology Journal 15(9), 1175-1185.
| Crossref | Google Scholar | PubMed |
Karve R, Liu W, Willet SG, Torii KU, Shpak ED (2011) The presence of multiple introns is essential for ERECTA expression in Arabidopsis. RNA 17(10), 1907-1921.
| Crossref | Google Scholar | PubMed |
Lata C, Gupta S, Prasad M (2013) Foxtail millet: a model crop for genetic and genomic studies in bioenergy grasses. Critical Reviews in Biotechnology 33(3), 328-343.
| Crossref | Google Scholar | PubMed |
Li H, Han X, Liu X, Zhou M, Ren W, Zhao B, Ju C, Liu Y, Zhao J (2019) A leucine-rich repeat-receptor-like kinase gene SbER2–1 from sorghum (Sorghum bicolor L.) confers drought tolerance in maize. BMC Genomics 20(1), 737.
| Crossref | Google Scholar | PubMed |
Li H, Yang Y, Wang H, Liu S, Jia F, Su Y, Li S, He F, Feng C, Niu M, Wang J, Liu C, Yin W, Xia X (2021) The receptor-like kinase ERECTA confers improved water use efficiency and drought tolerance to poplar via modulating stomatal density. International Journal Molecular Sciences 22(14), 7245.
| Crossref | Google Scholar | PubMed |
Liu P, Xu ZS, Lu PP, Hu D, Chen M, Li LC, Ma YZ (2013) A wheat PI4K gene whose product possesses threonine autophophorylation activity confers tolerance to drought and salt in Arabidopsis. Journal of Experimental Botany 64(10), 2915-2927.
| Crossref | Google Scholar | PubMed |
Liu M, Li W, Zhuo M, Cheng X, Fang Y (2019) Identification and expression analysis of ERECTA family genes in grape (Vitis vinifera L.). Genes Genomics 41(6), 723-735.
| Crossref | Google Scholar | PubMed |
Masle J, Gilmore SR, Farquhar GD (2005) The ERECTA gene regulates plant transpiration efficiency in Arabidopsis. Nature 436(7052), 866-870.
| Crossref | Google Scholar | PubMed |
Roy SW, Penny D (2006) Patterns of intron loss and gain in plants: intron loss-dominated evolution and genome-wide comparison of O. sativa and A. thaliana. Molecular Biology and Evolution 24(1), 171-181.
| Crossref | Google Scholar | PubMed |
Shen H, Zhong X, Zhao F, Wang Y, Yan B, Li Q, Chen G, Mao B, Wang J, Li Y, Xiao G, He Y, Xiao H, Li J, He Z (2015) Overexpression of receptor-like kinase ERECTA improves thermotolerance in rice and tomato. Nature Biotechnology 33(9), 996-1003.
| Crossref | Google Scholar | PubMed |
Shpak ED (2013) Diverse roles of ERECTA family genes in plant development. Journal of Integrative Plant Biology 55(12), 1238-1250.
| Crossref | Google Scholar | PubMed |
Shrestha N, Hu H, Shrestha K, Doust AN (2023) Pearl millet response to drought: a review. Frontiers in Plant Science 14, 1059574.
| Crossref | Google Scholar | PubMed |
Tang S, Li L, Wang Y, Chen Q, Zhang W, Jia G, Zhi H, Zhao B, Diao X (2017) Genotype-specific physiological and transcriptomic responses to drought stress in Setaria italica (an emerging model for Panicoideae grasses). Scientific Reports 7, 10009.
| Crossref | Google Scholar | PubMed |
Villagarcia H, Morin AC, Shpak ED, Khodakovskaya MV (2012) Modification of tomato growth by expression of truncated ERECTA protein from Arabidopsis thaliana. Journal Experiment Botany 63(18), 6493-6504.
| Crossref | Google Scholar |
Xing HT, Guo P, Xia XL, Yin WL (2011) PdERECTA, a leucine-rich repeat receptor-like kinase of poplar, confers enhanced water use efficiency in Arabidopsis. Planta 234(2), 229-241.
| Crossref | Google Scholar | PubMed |
Yang H, Bayer PE, Tirnaz S, Edwards D, Batley J (2021) Genome-wide identification and evolution of receptor-like kinases (RLKs) and receptor like proteins (RLPs) in Brassica juncea. Biology 10(1), 17.
| Crossref | Google Scholar |
Zheng JC, Liu T, Zheng QX, Li JQ, Qian YC, Li JC, Zhan QW (2020) Identification of cold tolerance and analysis of genetic diversity for major wheat varieties in Jianghuai Region of China. Pakistan Journal Botany 52(3), 839-849.
| Crossref | Google Scholar |