Small ubiquitin-like modifiers E3 ligases in plant stress
Shantwana Ghimire

A
Abstract
Plants regularly encounter various environmental stresses such as salt, drought, cold, heat, heavy metals and pathogens, leading to changes in their proteome. Of these, a post-translational modification, SUMOylation is particularly significant for its extensive involvement in regulating various plant molecular processes to counteract these external stressors. Small ubiquitin-like modifiers (SUMO) protein modification significantly contributes to various plant functions, encompassing growth, development and response to environmental stresses. The SUMO system has a limited number of ligases even in fully sequenced plant genomes but SUMO E3 ligases are pivotal in recognising substrates during the process of SUMOylation. E3 ligases play pivotal roles in numerous biological and developmental processes in plants, including DNA repair, photomorphogenesis, phytohormone signalling and responses to abiotic and biotic stress. A considerable number of targets for E3 ligases are proteins implicated in reactions to abiotic and biotic stressors. This review sheds light on how plants respond to environmental stresses by focusing on recent findings on the role of SUMO E3 ligases, contributing to a better understanding of how plants react at a molecular level to such stressors.
Keywords: abiotic stress, biotic stress, hormonal signalling, plant response, small ubiquitin-like modifiers, SUMO E3 ligase, SUMO targets, SUMOylation.
Introduction
Agricultural production globally has suffered significant yield losses due to exposure to abiotic stress conditions, such as drought, salt, heat and cold. Economic losses and damages in agrifood systems are significant. According to post-disaster assessments conducted by the Food and Agriculture Organization (FAO) between 2007 and 2022, agricultural losses made up an average of 23% of the total impact of disasters across agricultural and livestock sectors. Droughts were responsible for over 65% of losses in the agriculture sector (FAO 2023).
Abiotic stress encompasses environmental factors that impede plant growth and development, including mechanical disturbances, rapid light changes and severe conditions like soil salinity, extreme temperatures and drought (Zhang et al. 2022, 2023a). As plants cannot relocate to evade these stressors, they rely on strategies to swiftly adapt to environmental alterations for survival and sustained growth (Muhammad et al. 2024). Precise regulation of protein activity becomes crucial during rapid environmental changes, ensuring swift and reversible physiological adjustments for the organism’s functioning (Castro et al. 2012). The SUMOylation pathway is an example of a mechanism that allows plants to respond to a wide range of stressors (Vertegaal 2022). SUMOylation, a reversible post-translational modification (PTM) present in all eukaryotes, governs protein activity, stability, localisation and protein–protein interactions via conjugation with a small ubiquitin-like modifier (SUMO) (Celen and Sahin 2020). SUMOs are small proteins (10–15 kDa) featuring a conserved β-grasp structure comprising a five-stranded β-sheet enveloping a central α helix (Ghimire et al. 2020) (Fig. 1a). They bear a structural resemblance to ubiquitin, despite their limited sequence similarity with it (Cappadocia and Lima 2018). SUMOs slightly surpass ubiquitin in dimensions and have an N-terminal extension not found in ubiquitin (Ghimire et al. 2021). They exhibit versatility in conjugation to target proteins, existing either as single or multiple monomers or forming diverse polymer structures. Analogous to ubiquitin, SUMOs undergo modifications, such as phosphorylation and/or acetylation (Vertegaal 2022).
Plants SUMO E3 ligases structural organisation referencing Arabidopsis thaliana. (a) Structural complex of AtSUMO1 protein showing the SUMOylation and SUMO interacting sites. (b) Diagram illustrating three plant-based SUMO E3 ligase types: Arabidopsis thaliana SIZ1, MMS21 and PIAL1, showcasing their respective domains as different shade boxes. SAP, scaffold attachment factor (SAF)-A/B, acinus, PIAS; PHD-3, prolyl hydroxylase domain; MIZ, Myc-interacting zinc finger protein; PIIT, Pro-Ile-Ile-Thr; SP-RING, Siz/PIAS RING; SPL-RING, SP-like RING; PTNVT, Pro-Thr-Asn-Val-Thr.
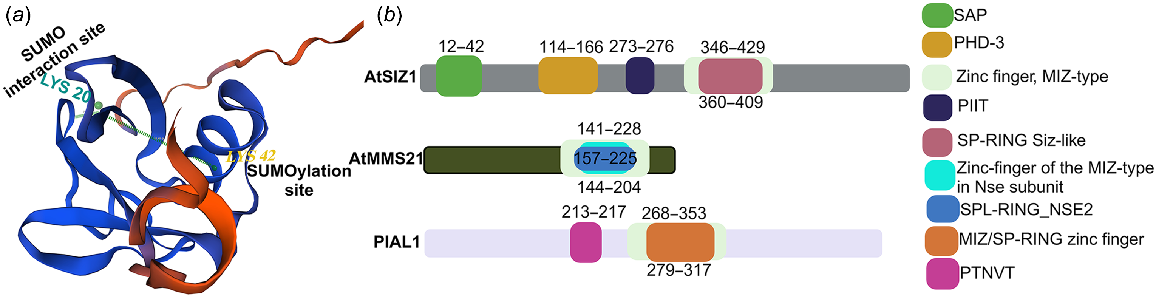
In plants, various stresses like heat, drought, cold, salt heavy metals and pathogens rapidly induce SUMOylation (Johnson 2004; Varejão et al. 2020). In contrast to ubiquitin, SUMO typically doesn’t relate to protein degradation. Instead, it regulates the target’s conformation, modulating protein activity and creating or blocking interacting interfaces based on the specific target (Wilkinson and Henley 2010). Initially proposed as antagonistic processes due to their targeting of the same amino acids, the understanding of SUMOylation and ubiquitination is evolving. Recent evidence in humans and yeast demonstrates the recruitment of ubiquitin by SUMO chains through SUMO-targeted ubiquitin ligases (STUbLs) (Geoffroy and Hay 2009; Han et al. 2023).
SUMO pathway
The SUMO conjugation pathway is a highly conserved mechanism found in eukaryotes and consists of four basic steps. The first step involves an ATP-dependent activation of SUMO by an E1 enzyme, which binds to the substrate protein and transfers it to an E2 enzyme in the second step. In the third step, the E2 enzyme associates with an E3 SUMO ligase, which marks the protein for SUMOylation by building up a chain of SUMO molecules. E2 enzymes can transfer SUMO to a target protein, primarily facilitated by SUMO E3 ligases, although E3-independent transfer is also feasible (Fig. 2). Conjugation typically happens at a lysine residue within the SUMOylation consensus motif ψKXE (where ψ represents a large hydrophobic residue, K stands for lysine, X signifies any amino acid and E denotes glutamic acid). Additionally, alternative SUMO conjugation sites like the inverted consensus motif, hydrophobic cluster motif, phosphorylation-dependent SUMO motif (PDSM) and the negatively charged amino acid-dependent SUMO motif (NDSM) have been identified (Varejão et al. 2020). The motif’s placement within the target is crucial. Verified SUMO consensus sites are commonly located in extended loops or intrinsically disordered regions outside the substrate’s globular fold. This placement allows the motif to adopt an extended conformation, facilitating effective interaction with the E2. Finally, the SUMO protease cleaves the protein. This is the fourth and final step, releasing free SUMO for the next cycle (Cappadocia and Lima 2018).
Fig. 2 provides a diagram illustrating the structures of specialised enzymes involved in the catalytic sequence leading to the formation of SUMO conjugates. Three enzymes for the conjugation pathway E1 (SAE1/2), E2 (SCE) and E3 (SIZ1) are presented in brown, green and purple colours, respectively. GG signifies the di-glycine pattern positioned at the C-terminus of SUMO. The ATP-dependent E1-activating enzyme triggers the activation of the SUMO precursor, previously processed to leave a Gly-Gly motif at the C-terminus, by forming a high-energy thioester bond between the SUMO C-terminus and an internal Cys. Subsequently, the SUMO-E1-thioester can be equivalently transferred to a Cys residue within the E2 conjugating enzyme. The E2-SUMO-thioester can then attach SUMO onto one or multiple lysine residues on target substrates, forming covalent isopeptide bonds. The discharge of E2-SUMO can be facilitated by a SUMO E3 ligase enzyme. Ultimately, the attachment of SUMO can be reversed by a specific family of SUMO proteases by cleaving SUMO precursors and SUMO-conjugated substrates releasing the free SUMO for another cycle.
Over time, two primary protein SUMOylation models have emerged. Firstly, the ’star effect’ model highlights the impactful biological outcomes resulting from the SUMOylation of a single protein (Sarangi and Zhao 2015). Conversely, the ‘ensemble effect model’ targets multiple complex subunits to enhance complex cohesion (Psakhye and Jentsch 2012; Sarangi and Zhao 2015; Augustine and Vierstra 2018). SUMOylation can regulate protein–protein interactions by either masking them or complementing existing interactions with non-covalent interactions between SUMO and SUMO interacting motifs (SIM) (Lascorz et al. 2022). SIMs facilitate non-covalent interactions between SUMO and proteins containing SIMs, increasing complexity within the network of SUMO-dependent protein interactions. SIMs typically consist of a brief sequence of hydrophobic amino acids (V/I)X(V/I)(V/I), bordered by acidic residues (Cappadocia and Lima 2018).
SUMO E3
Most eukaryotic SUMO E3 ligases, like Siz/PIAS-RING, feature an SP-RING domain (Siz for Saccharomyces cerevisiae and PIAS for vertebrates), like ubiquitin (Ub) E3 ligases’ RING domain (Johnson 2004). Four SUMO E3 ligases are reported in Arabidopsis thaliana (Catala et al. 2007; Zhang et al. 2023b). Among SUMO E3 ligases, the majority belong to the protein inhibitor of activated STAT (PIAS) type. These feature a SAP (scaffold attachment factor (SAF)-A/B, acinus, PIAS) motif, a PINIT motif (Pro-Ile-Asn-Ile-Thr), a SUMO interacting motif (SIM) or SXS (Ser-X-Ser), where X is any amino acid), and a C-terminal domain, alongside the SP-RING domain. The SAP motif is essential for DNA binding, the PINIT motif for nuclear retention/subcellular localisation, and the SIM or SXS motif for interacting with SUMO. A subset of SP-RING domain SUMO E3 ligases, lacking the SAP and PINIT domains present in PIAS proteins, is known as methyl methanesulfonate-sensitive 21 (MMS21), named after the canonical S. cerevisiae protein. PIAS and MMS21 SUMO E3 ligases exist across all organisms (Wilkinson and Henley 2010).
E3 ligases lacking an SP-RING domain are unique to animals but contain a domain enabling direct interaction with SUMO E2 (Varejão et al. 2021). This category includes SUMO E3 ligases like the animal-specific nucleoporin RanBP2/Nup358, playing a critical role in RanGAP SUMOylation and controlling nuclear translocation (Pichler et al. 2002). Pc2, a polycomb protein found in the polycomb body subnuclear protein complex, serves as another type of SUMO E3. It holds importance in regulating transcription and chromatin structure in animals (Tsuge et al. 2015; Lin et al. 2016).
Saccharomyces cerevisiae possesses two PIAS family SUMO E3 ligases: Siz1/Ull1 and Siz2/Nfi1 (Chen et al. 2007). SIZ1 and SIZ2 target different substrates and are essential for most of the Smt3p conjugation in S. cerevisiae. On the other hand, Saccharomyces pombe (fission yeast), fruit fly (Drosophila melanogaster) and worm (Caenorhabditis elegans) each have one PIAS family E3 ligase: Pli1, Su(var)2-10/zimp, and gei-17, respectively (Park and Yun 2013). Four genes in mammals encode five PIAS proteins: PIAS1, PIAS3, both α and β splice variants of PIASx, and PIASy. Across mammals, yeast, Drosophila and C. elegans, the PIAS family SUMO E3 ligases are involved in regulating gene expression, signal transduction and genome maintenance (Johnson 2004). Mutations in Dpli1 and Siz1Dsiz2D genes of Saccharomyces pombe and Su(var)2-10/zimp and gei-17 genes of S. cerevisiae result in embryonic lethality in fruit flies and worms, respectively (Andrews et al. 2005). Both S. cerevisiae and S. pombe have one MMS21 family SUMO E3 ligase each, which is required for DNA repair in both yeast and humans (Potts and Yu 2005), and deletion of MMS21 in S. cerevisiae and NSE2 in S. pombe leads to lethality (Potts and Yu 2005).
Four SUMO E3 ligases are known in Arabidopsis thaliana: SAP AND MIZ1 DOMAIN-CONTAINING LIGASE 1 (SIZ1) (Catala et al. 2007), METHYL METHANESULFONATE-SENSITIVITY PROTEIN 21 (MMS21) (Zhang et al. 2023b) and PROTEIN INHIBITOR OF ACTIVATED STAT-LIKE 1/2 (PIAL1/2) (Tomanov et al. 2014). These control the conjugation of over 100 proteins (Rytz et al. 2018) and fall into three main classes: SIZs, MMS21s/HPY2 and PIALs. The protein AtSIZ1 has four conserved domains that are shared with non-plant PIAS proteins, and a unique fifth domain called the plant homeodomain (PHD), which is found only in plant PIAS proteins. The four conserved domains of AtSIZ1 are SAP, PINIT, SP-RING and SXS (Cheong et al. 2010). The SIZ1 SIM, known as the SXS motif, is highly conserved in plants (Minty et al. 2000). However, it constitutes a segment of the C-terminal extension of the SIM (Park et al. 2011a). The PINIT domain consists of two interlaced β-sheets. Although it is a characteristic feature of this domain, the signature PINIT motif shows variations across evolution: PINIT in human PIAS1, PADLT in yeast SIZ1, PIIT (Pro-Ile-Ile-Thr) in Arabidopsis SIZ1, and PTNVT in Arabidopsis PIAL1/2. So, we have labelled the PINIT domain as PIIT and PTNVT (Pro-Thr-Asn-Val-Thr) in Fig. 1b. The PHD and SP-RING domains in AtSIZ1 are essential for both binding to the conjugating enzyme AtSCE1 and facilitating SUMO conjugation (Garcia-Dominguez et al. 2008). Through expressing motif-deleted mutant AtSIZ1 proteins (SIZ1sap, SIZ1phd, SIZ1pinit, SIZ1sp-ring and SIZ1sxs) in a siz1-2 mutant, it was determined that the SP-RING domain is crucial for in vivo SUMO conjugation and the subnuclear localisation of AtSIZ1 into punctate structures. Mutating the PIIT motif to PAAT in Arabidopsis SIZ1 reduces SUMO conjugation, leading to abnormal hypocotyl elongation in response to sugar and light (Cheong et al. 2009). The traits observed in Atsiz1 loss-of-function mutants encompass dwarfism, heightened salicylic acid (SA) levels and modified response to environmental stress (Zhang et al. 2020). AtMMS21/HYP2 participates in cell proliferation and maintains root meristem (Xu et al. 2013).
SUMO E3 functions in the growth and development of plants
SUMO E3 ligases are involved in regulating growth, development and responses to environmental stresses and are considered a strong candidate for improving crop yields (Elrouby 2017; Mishra et al. 2017). AtSIZ1 in Arabidopsis regulates gene methylation by sumoylating CHROMOMETHYLASE 3 (CMT3), enhancing its methyltransferase activity, and affects gene methylation linked to nitrate signalling, light perception, auxin signalling and flowering (Kim et al. 2015a).
Atsiz1-null mutants likely survive due to other E3 ligases but exhibit dwarfism due to fewer and smaller cells compared to the wild type (Cheong et al. 2009). Similarly, a study in rice (Orzya sativa) showed Null Ossiz1 rice plants lacking functional SIZ1 SUMO E3 ligase are shorter, with shorter primary roots and longer, more abundant adventitious roots compared to wild-type rice plants (Park et al. 2010). A similar result was found by Wang et al. (2011), where OsSIZ1 mutants generated with a T-DNA insertion were examined to elucidate their role in rice vegetative growth and reproductive development. The findings revealed significant alterations in various growth and developmental aspects, such as primary root length, adventitious root number, plant height, leaf and panicle length, flower formation and seed-setting rate compared to the wild type (Wang et al. 2011). AtSIZ1-driven SUMOylation activates NIA1 and NIA2, causing siz1-2 growth issues due to reduced nitrate reductase (NR) activity and ammonia limitations from faulty SUMO conjugation (Park et al. 2011b). SUMOylation, along with phosphorylation, crucially regulates Arabidopsis NR activity, and AtSIZ1-mediated SUMOylation profoundly impacts plant development through nitrogen assimilation. Another study in Arabidopsis showed that SIZ1 suppresses shoot regrowth in vitro, whereas siz1 mutants demonstrate excessive shoot production in tissue culture, suggesting that SIZ1 negatively regulates the shoot regeneration (Coleman et al. 2020). SIZ1 is involved in the formation of the apical hook (which safeguards the cotyledons and the shoot apical meristem from physical damage while seedlings emerge) by SUMOylating HOOKLESS1 (HLS1), which forms more active oligomers in Arabidopsis. Once exposed to light, ELONGATED HYPOCOTYL 5 (HY5) inhibits SIZ1 transcription, reducing HLS1’s SUMOylation and triggering a swift opening of the apical hook (Xiong et al. 2023). This study elucidated a post-translational modification of HLS1 and also uncovered a fast regulatory process governing apical hook opening through SIZ1-mediated HLS1 SUMOylation. A recent study in apples (Malus domestica) suggested that MdSIZ1 directly SUMOylated MdARF8, increasing its protein stability; and this interaction promoted lateral root development in apples (Zhang et al. 2021). In addition, MdSIZ1-mediated SUMOylation is found to be essential for MdCNR8 to regulate cell proliferation, controlling plant organ size in apple (Wang et al. 2022).
A study showed the OsMMS21 rice T-DNA mutant exhibited a short-root and dwarf phenotype, suggesting its crucial role in rice growth and development (Jiang et al. 2021). AtMMS21 plays a role in cytokinin signalling that regulates both root cell division and meristem development (Zhang et al. 2010). OsMMS21 deficiency in rice plants resulted in significant abnormalities in both shoot and root structures, uncovering its role in rice development (Xun et al. 2023).
An ectopic expression of the DiMMS21 gene from forage legume Desmodium intortum into the Arabidopsis mms21 mutant entirely restored root, leaf and silique development defects (Zhou et al. 2019a). A study on Arabidopsis revealed that AtMMS21 is a crucial regulator of cell proliferation and cytokinin signalling during root development where the mms21-1 mutant showed a short-root phenotype and altered cytokinin responses (Huang et al. 2009). A study showed that high PLOIDY2 (HPY2)/MMS21 is a nuclear-localised SUMO E3 ligase that plays a crucial role in regulating endocycle initiation in the meristems of Arabidopsis thaliana. The absence of HPY2 resulted in an early shift from the mitotic cycle to the endocycle, causing severe dwarfism and defective meristems (Ishida et al. 2009). This highlights the significant role of HPY2 in regulating cell cycle progression and meristem development in Arabidopsis thaliana by controlling endocycle initiation. IAA17, an auxin (Aux)/indole acetic acid (IAA) protein involved in root growth regulation, interacted with the AtMMS21 in Arabidopsis. Overexpressing IAA17 suppressed root length, but K41-mutated IAA17 transgenic plants showed no significant difference compared to wild-type roots. Biochemical analysis showed that K41-mutated IAA17 or IAA17 in the AtMMS21 knockout mutant had a higher probability of degradation compared to non-mutated IAA17 in wild-type plants (Zhang et al. 2023b). Collectively, these results emphasise the crucial role of SUMO E3 in governing plant growth and development.
In Arabidopsis, SIZ1 exhibited increased expression in female reproductive organs, but it was not detected in the anther or pollen. A defect in the siz1-2 maternal source resulted in reduced seed production, regardless of the plant’s high salicylic acid (SA) concentration (Ling et al. 2012). In rice, the ossiz2 mutant showed clear abnormalities in anther dehiscence, pollen fertility and seed set percentage (Pei et al. 2019), which indicated that OsSIZ2 plays a positive role in controlling various agronomic traits, including anther and seed development. AtSIZ1’s E3 ligase activity is also pivotal in governing the accumulation and stability of seed storage proteins CRUCIFERIN1 (CRU1), CRU2 and CRU3 (Kwak et al. 2019).
AtMMS21 also plays a crucial role in plant reproduction. Plants lacking AtMMS21 exhibited semi-sterility, characterised by shorter mature siliques and nonviable seeds. The mms21-1 mutant, specifically, showed decreased pollen count and viability, abnormal germination and disrupted pollen tube growth (Liu et al. 2014). Furthermore, the mutant experiences compromised embryo sac development, which contributes to its reproductive issues (Liu et al. 2014). This result highlights SUMO ligases’ role in plant reproduction and the impact of their absence on the reproductive process.
The function of SUMO proteases and SUMO E3 ligase is linked to flowering time control in Arabidopsis. AtSIZ1 regulates flowering by inhibiting the expression of FLOWERING LOCUS C (FLC) through SUMOylation of R proteins RRS1, reducing SA and FLOWERING LOCUS D (FLD) accumulation, thus impacting flowering time (Jin and Hasegawa 2008). Overexpressing AtSIZ1 increased FLC concentration and extended FLC’s post-translational stability. In contrast, mutant FLC (K154R) showed similar flowering times to the wild type, whereas FLC overexpression delayed flowering (Son et al. 2014). These findings emphasise the critical role of FLC SUMOylation in controlling flowering time and AtSIZ1’s positive regulation of FLC-mediated floral repression.
A study in Arabidopsis showed that SIZ1 regulates flowering time through both SA-dependent and SA-independent pathways. The siz1 mutant, characterised by increased SA levels, exhibits early flowering in short days compared to the wild type (Jin et al. 2008). Disabling rice SIZ1, responsible for encoding a SUMO E3 ligase, via mutation or transgenic RNA interference (RNAi)-mediated suppression, reduces seed production due to issues with anther dehiscence and endothecium development. However, this alteration does not impact pollen viability or flowering time (Park et al. 2010; Thangasamy et al. 2011). Ectopic expression of wintersweet (Chimonanthus praecox) CpSIZ1 in Arabidopsis, both in the siz1-2 mutant and wild-type (WT), resulted in enhanced vegetative growth, delayed flowering, accelerated leaf senescence and improved cold tolerance (Li et al. 2021). SIZ1 in other plants, like DnSIZ1 in dendrobium, also influences flowering negatively. However, the specific mechanism governing flowering time in dendrobium is not yet understood (Liu et al. 2015). Introducing ZmSIZ1c into wild-type Arabidopsis led to early flowering (Lai et al. 2022). Given that altering SIZ1 in Arabidopsis influences flowering time, it is crucial to further compare the functions of different plant lineages SUMO E3 ligases.
SIZ1 controls seed dormancy and germination. In Arabidopsis, after cold treatment or extended ripening, partially after-ripened siz1 mutant seeds showed improved germination rates comparable to wild-type. Supplying exogenous gibberellic acid (GA) also raised siz1 seed germination to wild-type levels. AtSIZ1 maintained SLEEPY1 (SLY1) stability during germination, while mSLY1 didn’t impact germination. In siz1 mutants, SLY1 expression decreased during imbibition, whereas DELAY OF GERMINATION 1 (DOG1), ABSCISIC ACID INSENSITIVE 3 (ABI3) and DELLA gene expressions increased (Kim et al. 2016). IbSIZ1 genes’ expression in the Arabidopsis atsiz1 mutant restored normal germination and growth (Zhang et al. 2023c). These findings give insights into E3 SUMO ligase activity that regulates germination and growth.
SUMO E3 functions in plant abiotic stresses
Plants have evolved complex mechanisms to adapt to harsh environmental conditions due to their immobility. These include regulating ion levels, producing antioxidants and generating compatible substances. Plants also activate stress-related elements, transcription factors, and stress response genes to cope with stress. Abiotic stressors such as soil salinity, drought and extreme temperatures reduce crop productivity, resulting in lower crop yields. SUMOylation plays a significant role in plant responses to environmental stress, supported by substantial evidence. When plants face stress like cold, heat, drought, salt or heavy metals, the levels of sumoylated proteins in plant tissues notably increase.
Ectopically expressing apple MdSIZ1 in Arabidopsis siz1-2 mutants partly restored the mutant phenotype and increased SUMO conjugation levels (Zhang et al. 2016). These findings highlight the significance of MdSIZ1-mediated SUMO conjugation in regulating apple plant adaptation to diverse environmental stresses. In tomatoes (Solanum lycopersicum), the gene SlSIZ1 exhibited increased activity in response to various stressors like cold, NaCl (sodium chloride), PEG (polyethylene glycol), H2O2 (hydrogen peroxide) and ABA (abscisic acid). When introduced into Arabidopsis siz1-2 mutants, SlSIZ1 expression partly reversed their stunted growth, sensitivity to cold and heightened ABA response (Zhang et al. 2017). It functioned as a SUMO E3 ligase, elevating SUMO conjugate levels. In drought-stressed transgenic tobacco (Nicotiana tabacum), SlSIZ1 expression enhanced germination, reduced reactive oxygen species, increased growth, free proline levels and SUMO conjugates. As a functional counterpart to Arabidopsis SIZ1, SlSIZ1 improved drought tolerance in transgenic tobacco when overexpressed (Zhang et al. 2017). The transgenic cotton plants overexpressing OsSIZ1 exhibit increased tolerance to abiotic stresses, as evidenced by higher fibre yield in both controlled environments and rainfed field trials with reduced irrigation (Mishra et al. 2017). Overexpressing OsSIZ1 in Arabidopsis enhanced stress tolerance, as transgenic plants maintain higher chlorophyll content and produce greater seed yields compared to wild-type plants when exposed to drought, heat and salt stress individually or in combination (Mishra et al. 2018). This suggests that OsSIZ1 has the potential to enhance crop resilience to environmental stressors and improve overall crop performance.
CaDSIZ1-mediated SUMOylation in Capsicum annuum has been shown to regulate the stability of CaDRHB1 protein in pepper plants. When CaDSIZ1 is overexpressed or knocked down, the resulting phenotypes differ significantly. Pepper plants with reduced CaDSIZ1 expression (CaDSIZ1-silenced) display decreased ABA sensitivity and drought tolerance, whereas those overexpressing CaDSIZ1 exhibit increased ABA sensitivity and drought tolerance (Joo et al. 2022). These findings highlight the importance of CaDSIZ1 in regulating plant responses to drought stress and ABA signalling.
Overexpressing OsSIZ1 in creeping bentgrass (Agrostis stolonifera) enhanced photosynthesis and plant growth. Under drought and heat stress conditions, OsSIZ1 plants showed superior performance, characterised by stronger root growth, improved water retention, and better cell membrane integrity compared to wild-type controls. Moreover, a higher uptake of minerals including phosphate potassium and zinc was observed in the transgenic bentgrass (Li et al. 2013). MdSIZ1 facilitated MdMYB30’s SUMOylation and accumulation by impeding its degradation via the 26S proteasome pathway. Additionally, MdMYB30 directly interacted with the β-KETOACYL-COA SYNTHASE 1 (MdKCS1) promoter, stimulating its expression and enhancing wax biosynthesis (Zhang et al. 2023d). As cuticular wax is reportedly associated with drought tolerance (Guo et al. 2016), this finding suggests that MdSIZ1 positively regulates drought tolerance in apples. This strongly affirms SUMOylation’s role in regulating various molecular pathways in plant stress response, establishing a direct connection between SUMOylation and plants’ adaptation to environmental challenges.
In Arabidopsis, it was demonstrated that SIZ1 enhances basic thermotolerance through processes not reliant on salicylic acid (SA) (Yoo et al. 2006). Subsequent research revealed that the presence of natural SA influenced by SIZ1 affects stomatal closure and drought resistance in Arabidopsis. SIZ1 mutants showed reduced stomatal openings, regulated by SA-related reactive oxygen species (ROS) production. Introducing salicylate hydroxylase (NahG) in siz1 reverses these effects (Miura et al. 2013). Drought-induced SA-related gene expression and siz1 enhanced drought tolerance, counteracted by nahG (Miura et al. 2013). Overall, SIZ1’s role in promoting SA accumulation negatively impacts stomatal closure and drought tolerance.
AtSIZ1 underwent ubiquitination in response to heat stress and SUMOylation in response to drought. Modification of AtSIZ1 depended on constitutive photomorphogenic 1 (COP1) and early in short days 4 (ESD4) activities. Moreover, siz1–2 and siz1–3 mutants displayed tolerance to both heat and drought stresses (Kim et al. 2017). It highlights how distinct abiotic stresses can selectively regulate AtSIZ1’s activity and stability. A recent study disclosed that SIZ1 functions as a crucial promoter of growth and serves as a suppressor of a suppressor of npr1-1, constitutive 1 (SNC1)-dependent immune response in high-temperature conditions, creating a dual role for SIZ1 in both growth promotion and immune regulation under elevated ambient temperature, showcasing interdependent functions (Hammoudi et al. 2018). Ectopic expression of sweet potato genes IbSIZ1a/b/c improved drought tolerance in Arabidopsis (Zhang et al. 2023c).
Given the significant roles of H+-pyrophosphatase gene (AVP1) and SIZ1 in plant abiotic stress response, a study in Arabidopsis hypothesised that co-overexpressing AVP1 and OsSIZ1 has an additive effect on tolerance to water deficit, salinity and high-temperature stress (Esmaeili et al. 2019). AVP1/OsSIZ1 co-overexpression significantly enhanced tolerance to these stresses and co-overexpressing these genes could potentially improve crop yields substantially.
SlSIZ1 overexpression in tomatoes improved heat stress tolerance, whereas RNA interference (RNAi) plants exhibited increased wilting compared to the wild type under heat treatment (Zhang et al. 2018). Overexpressing OsSIZ1 in cotton has been shown to result in improved growth and enhanced net photosynthesis compared to wild-type cotton, particularly under drought and heat stresses (Mishra et al. 2017).
In a study on Arabidopsis, it was observed that plants lacking MMS21 exhibit increased drought tolerance, whereas those with constant MMS21 expression show reduced drought tolerance. The expression of MMS21 decreased under various stress conditions, such as treatment with abscisic acid (ABA), polyethylene glycol (PEG), or actual drought stress. During drought conditions, mms21 mutant plants had the highest survival rate, the slowest rate of water loss, and accumulated higher levels of free proline compared to wild-type (WT) and MMS21 overexpression plants (Zhang et al. 2013). This suggests that MMS21 plays a role in modulating drought tolerance in plants. In a recent study in Arabidopsis, the chromatin-remodelling factor PICKLE (PKL) mutant showed resistance to drought and inhibited the expression of the drought-resistant gene AFL1 by facilitating the deposition of H3K27me3 at AFL1 chromatin sites. Genetic evidence supports PKL’s role in plant drought resilience, relying on AFL1. Furthermore, MMS21 interacted directly with and reinforced PKL, contributing to additional regulation of drought tolerance (Jing et al. 2023). Overall, this study revealed an MMS21-PKL-AFL1 module governing Arabidopsis’ ability to tolerate drought.
AtSIZ1 was identified through genetic screening aimed at discovering suppressors of the salt overly sensitive phenotype seen in Atsos3 mutants. The siz1-1 mutation, resulting in loss of function, was observed to alleviate the sensitivity to NaCl in sos3-1 seedlings, suggesting a role for SIZ1 in salt stress response (Miura et al. 2005). The overexpressed ZmSIZ1 Arabidopsis showed high tolerance to salinity (Lai et al. 2022). Ectopic expression of sweet potato genes IbSIZ1a/b/c improved salt tolerance (Zhang et al. 2023c). In pepper, CaSIZ1 interacted with CaABI5, and ABA promoted SUMO conjugate accumulation. Knockdown of CaSIZ1 reduced ABA-induced SUMOylation and impaired salt tolerance, suggesting that CaSIZ1 positively regulates salt tolerance in pepper (Lei et al. 2022). Overexpressing MdSIZ1a in vivo appears to enhance the process of SUMO conjugation in MdSIZ1a callus when treated with NaCl and ABA. Conversely, reducing MdSIZ1a expression diminishes this effect (Li et al. 2021). These findings suggest that the overexpression of MdSIZ1a promotes protein SUMOylation to help plants enhance salt tolerance capacity. In salty environments, OsSIZ1-transgenic plants prevent sodium ion buildup in the cytoplasm, steering clear of sodium’s harmful impact inside the cell, unlike wild-type plants (Mishra et al. 2018).
Mutant analysis in Arabidopsis revealed that PIAL1 and PIAL2 participate in regulating responses to salt and osmotic stress while contributing to sulfate assimilation and sulfur metabolism (Tomanov et al. 2014).
The low-temperature (LT)-responsive MYB transcription factor (TF) MdMYB2 in apple was found to directly interact with the MdSIZ1 promoter, thereby activating MdSIZ1 transcriptionally. Additionally, MdMYB2 was shown to influence the SUMOylation of MdMYB1 (Jiang et al. 2022). This research has unveiled the mechanism by which MdMYB2 interacting with MdSIZ1 regulates anthocyanin biosynthesis and cold tolerance under low-temperature conditions. Ectopic expression of CpSIZ1 in Arabidopsis, both in siz1-2 mutant and WT, resulted in improved cold tolerance (Li et al. 2021). SIZ1 modulates the process of cold acclimation by managing ICE1 (inducer of CBF expression) activity, CBF/DREB1 expression (dehydration responsive element-binding 1), specifically CBF3/DREB1A, and the function of target genes (Miura et al. 2007). The K393R mutation impedes ICE1 SUMOylation, suppresses CBF3/DREB1A expression, and its associated group of genes, and diminishes the ability to withstand freezing (Miura et al. 2007).
AtSIZ1 was isolated through a genetic screening aimed at identifying suppressors of the salt overly sensitive phenotype observed in Atsos3 mutants. The loss-of-function mutation siz1-1 was found to alleviate the NaCl sensitivity in sos3-1 seedlings, indicating that SIZ1 plays a role in salt stress response. However, when SIZ1 was combined with SOS3 in the sos3-1siz1-2 double mutant, the plants displayed hypersensitivity to phosphate (Pi) limitation. This was evident through various phenotypic changes such as shorter primary roots, increased lateral roots and root hairs, a higher root/shoot mass ratio, and greater anthocyanin accumulation compared to wild-type plants (Miura et al. 2005). These results suggest that the interplay between SIZ1 and SOS3 is crucial for maintaining proper plant growth and development under both salt and phosphate-limited conditions. In an in vitro assay, it was observed that AtSIZ1 (a protein) facilitated the process of SUMOylation of AtPHR1. AtPHR1 is a transcriptional regulator involved in phosphate starvation response and is known to regulate the expression of genes such as AtIPS1, AtPNS1 and the target of miRNA399-Pho2 (Rubio et al. 2001; Bari et al. 2006; Duan et al. 2008). This finding suggests that AtSIZ1 plays a role in the regulation of phosphate starvation response through its interaction with AtPHR1. Auxin receptor TIR1-dependent signalling is connected to the development of lateral roots, which is initiated by phosphate starvation (Perez-Torres et al. 2008). This discovery highlights the role of TIR1-dependent signalling in the plant’s response to phosphate scarcity and its subsequent adaptation through lateral root development. Miura et al. (2011) showed that in low-phosphate conditions, auxin intensified the suppression of primary root growth and markedly increased lateral root density in siz1 compared to the wild type. Conversely, inhibition of auxin efflux carriers normalised lateral root growth in siz1 to wild-type levels (Miura et al. 2011). These findings suggest that SIZ1’s control of phosphate starvation responses includes alterations in auxin accumulation.
Another study investigated the OsSIZ1’s roles in balancing phosphorus (Pi) and nitrogen (N) in rice plants. OsSIZ1 consistently expressed throughout the plant’s life stages, showing limited responsiveness to Pi or N deficiencies at the transcript level. Mutations in OsSIZ1 led to increased P or N concentration and altered Pi distribution in various ossiz1 mutant tissues compared to wild-type (WT) plants. Quantitative reverse transcription–polymerase chain reaction (qRT–PCR) analysis of ossiz1 mutants highlighted OsSIZ1’s diverse effects on genes related to Pi starvation signalling, N transport, and assimilation (Miura et al. 2005). These findings offer insights into how rice plants regulate Pi and N homeostasis. A similar study was conducted on rice suggesting the role of OsSIZ2 in regulating Pi and N homeostasis (Pei et al. 2017; Pei et al. 2019).
Similarly, genetic analyses conducted on Arabidopsis revealed the existence of SIZ1-independent pathways for the low-phosphate response. By comparing root phenotypes and gene expression in low-phosphate conditions among Phosphate Starvation-Insensitive (PSI), siz1 and siz1psi1 mutants, it was demonstrated that PSI operates independently of SIZ1 (Yuan and Liu 2008; Wang et al. 2010). This finding suggests that there are multiple pathways involved in the low-phosphate response in Arabidopsis, with SIZ1 and PSI being part of distinct and separate pathways.
Research on apples demonstrated that MdSIZ1 transgenic apple callus exhibited robust growth in phosphate-deficient conditions, whereas MdSIZ1 sense apple callus did not. This finding indicates that MdSIZ1 plays a crucial role in regulating the phosphate-deficiency response in apples (Zhang et al. 2019a). A similar study was carried out in an iron deficit condition where MdSIZ1 played a role in the plasma membrane (PM) H+-ATPase response to iron deficiency. Overexpressing MdSIZ1 positively impacted PM H+-ATPase-mediated rhizosphere acidification and iron uptake (Zhou et al. 2019b).
The precise mechanism of how SUMOylation and SUMO E3 activity regulate nutrient starvation responses is still unknown. However, the observed connections between this process, root architecture changes, and alterations in different hormone accumulation patterns in the various organs may provide a pathway toward understanding this mechanism.
Research in Arabidopsis demonstrated that malfunctioning SUMO E3 ligase SIZ1 enhances arsenite resistance in the plant. The dominant-negative SUMO E2 variant’s overexpression mirrored the arsenite-resistant characteristics of the siz1 mutant, suggesting that SUMOylation negatively influences plant arsenite detoxification. The siz1 mutant accumulated higher glutathione (GSH) levels than the wild type during arsenite stress. Inhibition of GSH production diminished the arsenite-resistant traits of siz1. Additionally, the siz1 mutant exhibited increased transcript levels of genes involved in GSH biosynthesis compared to the wild type when exposed to arsenite (Hong et al. 2022). The discoveries unveiled a new role of SIZ1 in controlling plant arsenite response by regulating GSH-dependent detoxification. A study in Arabidopsis showed heightened sensitivity to copper in the siz1 mutant, along with a SIZ1-dependent increase in SUMO1 conjugates during excessive copper treatment. Notably, the siz1 mutant displayed an increased shoot-to-root copper concentration ratio. Analysis of gene expression patterns and mutant studies suggest the potential role of YSL1 and YSL3 in regulating copper translocation (Lai et al. 2022).
The mutants SIZ1 displayed enhanced Al resistance in Arabidopsis through STOP1 (sensitive to proton rhizotoxicity 1) partial SUMOylation, where STOP1 protein levels decreased in siz1 mutants while the expression of STOP1-target gene AtALMT1 increased. These results suggest that SIZ1 likely modulates Al resistance by regulating AtALMT1 expression (Fang et al. 2021; Xu et al. 2021). However, a contrasting result was reported by Mercier et al. (2021), where the root tips of siz1 mutants accumulated more STOP1 protein than in the WT. Mercier and the team explained that the difference might stem from varying experimental approaches where Fang et al. (2021) and Xu et al. (2021) analysed proteins obtained from entire roots, whereas Mercier’s team specifically used proteins from the root tip. The siz1-2 mutant of Arabidopsis, with reduced function, exhibited decreased resistance to Cd and increased accumulation of reactive oxygen species (ROS), which suggested that SIZ1 positively regulates plant tolerance to Cd exposure (Zheng et al. 2022).
SUMO E3 functions in plant biotic stresses
Mutants lacking AtSIZ1 in A. thaliana show increased resistance to Pseudomonas syringae pv. tomato DC3000 due to higher SA levels and amplified expression of immune-related genes, indicating AtSIZ1’s role as a negative regulator of immunity (Lee et al. 2007). A recent maize (Zea mays) study demonstrated the essential and redundant role of ZmSIZ1a and ZmSIZ1b in countering FER (fusarium ear rot) (Liao et al. 2023). There is a scarcity of literature that explores the involvement of plant SUMO E3 ligases in biotic stress responses.
SUMO E3 functions in hormonal signalling
In a study of Arabidopsis, the heightened ABA sensitivity seen in siz1 mutants partly involves ABI5 (ABA insensitive 5). In siz1 mutants, there’s increased ABA-triggered expression of ABI5-dependent genes like RD29A (responsive to desiccation 29A) compared to the wild type (Miura et al. 2009). SIZ1-mediated SUMOylation of ABI5 takes place at Lys-391. Introducing ABI5K391R in the abi5-4 mutant increased ABA sensitivity and boosted the expression of ABA-responsive genes more than expressing ABI5 at a similar level in the mutant, indicating that ABI5 SUMOylation by SIZ1 regulates ABA response (Miura and Hasegawa 2009). Collectively, this information demonstrates that the capability to withstand ABA’s suppressive impact on Arabidopsis seed germination and the elongation of seedling roots relies on SUMOylation.
myb30 mutant of Arabidopsis exhibited heightened sensitivity to ABA during germination and seedling growth. Expressing MYB30K283R in myb30 partially restored the mutant ABA-hypersensitive phenotype, whereas wild-type MYB30 fully complemented the mutant phenotype (Zheng et al. 2012). Overexpressing MYB30 in wild-type plants resulted in an ABA-insensitive phenotype, whereas overexpressing MYB30 in the siz1 mutant did not alter the hypersensitivity to ABA. The siz1-2 myb30-2 double mutant displayed greater ABA sensitivity than either single mutant, but a mutation in the SIZ1-sumoylated ABI5 transcription factor suppressed the ABA sensitivity and aligned with the wild-type response (Zheng et al. 2012). These findings suggest that the coordination of ABI5 and MYB30 SUMOylation by SIZ1 may balance gene expression, which is essential for regulating ABA signalling during seed germination.
AtMMS21-mediated SUMOylation of IAA17 stabilised the IAA17 protein, influencing the auxin signalling transduction and root development (Zhang et al. 2023b). The OsMMS21 mutant’s shortened roots and dwarfism highlight its importance in rice development. The presence of additional physiological and transcriptomic evidence suggests a possible association between OsMMS21 and auxin responses in rice (Jiang et al. 2021). The involvement of AtMMS21 in auxin signalling positively influences root meristem size by promoting cell division within the root meristem (Zhang et al. 2010).
While studying Arabidopsis roots, B2-type cyclin-dependent kinase (CDKB2;1) and HIGH HPY2 were accumulated in the stele (vascular cylinder) when exposed to external auxin, indicating that CDKB2; 1 accumulation may depend on HPY2-mediated SUMOylation, which is typically sustained by higher auxin levels in the meristem (Okushima et al. 2014). CDKB2 accumulation is regulated by multiple methods, including transcriptional control, protein degradation, and likely SUMOylation, all of which are connected to auxin signalling (Okushima et al. 2014).
GmSIZ1RNAi soybean (Glycine max) plants displayed decreased plant height and leaf size. Unlike Arabidopsis siz1-2 mutant plants, flowering time and SA levels remained largely unchanged in GmSIZ1RNAi plants. These findings suggest that GmSIZ1 positively regulates vegetative growth in soybeans by mediating SUMO modification (Cai et al. 2017). Arabidopsis siz1 mutant plants exhibited constant systemic-acquired resistance (SAR) with heightened salicylic acid (SA) levels, increased pathogenesis related (PR) gene expression, and resistance to Pseudomonas syringae pv. tomato (Pst) DC3000. These findings establish SIZ1 as a suppressor of SA-mediated signalling in plants. The activation of SA signalling caused by SIZ1 mutations leads to elevated SA levels, continuous defence responses, and improved resistance against pathogens (Lee et al. 2007). Furthermore, the expression of the bacterial NahG gene, which encodes a salicylate hydroxylase, resulted in decreased SA accumulation (Lee et al. 2007). Further research found that nahG not only inhibited the cell division and expansion issues induced by siz1 but also restored regular plant growth and development. The introduction of nahG gene in siz1-2 plants increased the expression of XTH8 and XTH31 genes, which encode xyloglucan endotransglycosylase/hydrolases (XTHs), to levels observed in wild-type plants (Miura et al. 2010). This study confirms that SIZ1 contributes to vegetative growth and development by regulating leaf cell division and expansion through SA signalling, which is associated with XTH gene expression. Moreover, during the early developmental stages of Arabidopsis, SA influences the SIZ1-dependent germinative or osmotic response to sugars but does not influence SIZ1 involvement in glucose signalling (Castro et al. 2018). AtSIZ1 controls gibberellic acid signalling in Arabidopsis by SUMOylating SLY1 (Kim et al. 2015b).
The siz1-2 mutant of Arabidopsis thaliana demonstrated hypersensitivity to brassinosteroids (BRs). SIZ1 negatively impacts the BR pathway by SUMOylating BES1 at K302, which results in BES1 degradation and activity inhibition (Zhang et al. 2019b). This finding sheds new light on SUMO E3 mediated SUMOylation’s role in regulating BR signalling.
Conclusion and future perspectives
Analysis of mutants with defective SUMOylation has provided a significant understanding of the role of SUMO E3 ligases and SUMOylation mechanism in plants. These studies have revealed that SUMOylation controls various plant processes such as environmental stress responses, plant growth, flowering, and hormonal signalling. Although SUMO E3 ligases play a role in plant responses to various stressors such as salt, drought, cold, and heat, the specific substrates involved in these pathways, particularly under different conditions and in single cell types, remain unexplored. Further investigation is needed to explore the interaction between SUMOylation and other protein modifications, as well as the physiological consequences of this crosstalk. Additionally, understanding the impact of SUMOylation on transcriptional complex activity and assembly, and its role in controlling substrate subcellular location, could be important areas of future research in plants.
The advent of sophisticated molecular tools, such as CRISPR-based genome editing (clustered regularly interspaced short palindromic repeats), provides the capability to intricately engineer the SUMOylation machinery within plants. With cutting-edge molecular tools like CRISPR-based genome editing, there is a newfound potential to intricately manipulate the SUMOylation machinery in plants. This paves the way for crafting crop traits tailored to specific environmental scenarios, fostering the development of crops that exhibit resilience to stress while being finely tuned for heightened growth and yield. This provides the platform for exploring the manipulation of SUMO E3 ligases to augment the production of bioactive compounds in plants. Through a comprehensive understanding and targeted modification of SUMOylation in key regulatory proteins involved in secondary metabolite biosynthesis, there is potential to amplify the accumulation of valuable compounds with applications in pharmaceutical or industrial sectors too.
Declaration of funding
This research was partially supported by the National Natural Science Foundation of China (Nos 32325036, 32171491), Gansu Science and Technology Project (22ZD6FA052, 23JRRA1037, 22ZD6NA007, 22JR5RA531, 23JRRA1161), Introduction plan for high-end foreign experts (G2022175007L), and top leading talents in Gansu Province.
Author contributions
S.G. conceived and designed the study. S.G. and M.M.H. critically revised the manuscript. X.W.F. critically edited and revised the manuscript and approved the final version.
References
Andrews EA, Palecek J, Sergeant J, Taylor E, Lehmann AR, Watts FZ (2005) Nse2, a component of the Smc5-6 complex, is a SUMO ligase required for the response to DNA damage. Molecular and Cellular Biology 25, 185-196.
| Crossref | Google Scholar | PubMed |
Augustine RC, Vierstra RD (2018) SUMOylation: re-wiring the plant nucleus during stress and development. Current Opinion in Plant Biology 45, 143-154.
| Crossref | Google Scholar | PubMed |
Bari R, Pant BD, Stitt M, Scheible W-R (2006) PHO2, microRNA399, and PHR1 define a phosphate-signaling pathway in plants. Plant Physiology 141, 988-999.
| Crossref | Google Scholar | PubMed |
Cai B, Kong X, Zhong C, Sun S, Zhou XF, Jin YH, Wang Y, Li X, Zhu Z, Jin JB (2017) SUMO E3 Ligases GmSIZ1a and GmSIZ1b regulate vegetative growth in soybean. Journal of Integrative Plant Biology 59, 2-14.
| Crossref | Google Scholar | PubMed |
Cappadocia L, Lima CD (2018) Ubiquitin-like protein conjugation: structures, chemistry, and mechanism. Chemical Reviews 118, 889-918.
| Crossref | Google Scholar | PubMed |
Castro PH, Tavares RM, Bejarano ER, Azevedo H (2012) SUMO, a heavyweight player in plant abiotic stress responses. Cellular and Molecular Life Sciences 69, 3269-3283.
| Crossref | Google Scholar | PubMed |
Castro PH, Verde N, Tavares RM, Bejarano ER, Azevedo H (2018) Sugar signaling regulation by Arabidopsis SIZ1-driven sumoylation is independent of salicylic acid. Plant Signaling & Behavior 13, e1179417.
| Crossref | Google Scholar | PubMed |
Catala R, Ouyang J, Abreu IA, Hu Y, Seo H, Zhang X, Chua N-H (2007) The Arabidopsis E3 SUMO ligase SIZ1 regulates plant growth and drought responses. The Plant Cell 19, 2952-2966.
| Crossref | Google Scholar | PubMed |
Celen AB, Sahin U (2020) Sumoylation on its 25th anniversary: mechanisms, pathology, and emerging concepts. The FEBS Journal 287, 3110-3140.
| Crossref | Google Scholar | PubMed |
Chen XL, Silver HR, Xiong L, Belichenko I, Adegite C, Johnson ES (2007) Topoisomerase I-dependent viability loss in Saccharomyces cerevisiae mutants defective in both SUMO conjugation and DNA repair. Genetics 177, 17-30.
| Crossref | Google Scholar | PubMed |
Cheong MS, Park HC, Hong MJ, Lee J, Choi W, Jin JB, Bohnert HJ, Lee SY, Bressan RA, Yun D-J (2009) Specific domain structures control abscisic acid-, salicylic acid-, and stress-mediated SIZ1 phenotypes. Plant Physiology 151, 1930-1942.
| Crossref | Google Scholar | PubMed |
Cheong MS, Park HC, Bohnert HJ, Bressan RA, Yun D-J (2010) Structural and functional studies of SIZ1, a PIAS-type SUMO E3 ligase from Arabidopsis. Plant Signaling & Behavior 5, 567-569.
| Crossref | Google Scholar | PubMed |
Coleman D, Kawamura A, Ikeuchi M, Favero DS, Lambolez A, Rymen B, Iwase A, Suzuki T, Sugimoto K (2020) The SUMO E3 ligase SIZ1 negatively regulates shoot regeneration. Plant Physiology 184, 330-344.
| Crossref | Google Scholar | PubMed |
Duan K, Yi K, Dang L, Huang H, Wu W, Wu P (2008) Characterization of a sub-family of Arabidopsis genes with the SPX domain reveals their diverse functions in plant tolerance to phosphorus starvation. The Plant Journal 54, 965-975.
| Crossref | Google Scholar | PubMed |
Elrouby N (2017) Regulation of plant cellular and organismal development by SUMO. Advances in Experimental Medicine and Biology 963, 227-247.
| Crossref | Google Scholar | PubMed |
Esmaeili N, Yang X, Cai Y, Sun L, Zhu X, Shen G, Payton P, Fang W, Zhang H (2019) Co-overexpression of AVP1 and OsSIZ1 in Arabidopsis substantially enhances plant tolerance to drought, salt, and heat stresses. Scientific Reports 9, 7642.
| Crossref | Google Scholar | PubMed |
Fang Q, Zhang J, Yang D-L, Huang C-F (2021) The SUMO E3 ligase SIZ1 partially regulates STOP1 SUMOylation and stability in Arabidopsis thaliana. Plant Signaling & Behavior 16, 1899487.
| Crossref | Google Scholar | PubMed |
FAO (2023) FAO report: agrifood sector faces growing threat from climate change-induced loss and damage. Food and Agriculture Organization. Available at https://www.fao.org/newsroom/detail/fao-report-agrifood-sector-faces-growing-threat-from-climate-change-induced-loss-and-damage/en
Garcia-Dominguez M, March-Diaz R, Reyes JC (2008) The PHD domain of plant PIAS proteins mediates sumoylation of bromodomain GTE proteins. Journal of Biological Chemistry 283, 21469-21477.
| Crossref | Google Scholar | PubMed |
Geoffroy M-C, Hay RT (2009) An additional role for SUMO in ubiquitin-mediated proteolysis. Nature Reviews: Molecular Cell Biology 10, 564-568.
| Crossref | Google Scholar | PubMed |
Ghimire S, Tang X, Zhang N, Liu W, Si H (2020) SUMO and SUMOylation in plant abiotic stress. Plant Growth Regulation 91, 317-325.
| Crossref | Google Scholar |
Ghimire S, Tang X, Liu W, Fu X, Zhang H, Zhang N, Si H (2021) SUMO conjugating enzyme: a vital player of SUMO pathway in plants. Physiology and Molecular Biology of Plants 27, 2421-2431.
| Crossref | Google Scholar | PubMed |
Guo J, Xu W, Yu X, Shen H, Li H, Cheng D, Liu A, Liu J, Liu C, Zhao S, Song J (2016) Cuticular wax accumulation is associated with drought tolerance in wheat near-isogenic lines. Frontiers in Plant Science 7, 1809.
| Crossref | Google Scholar | PubMed |
Hammoudi V, Fokkens L, Beerens B, Vlachakis G, Chatterjee S, Arroyo-Mateos M, Wackers PFK, Jonker MJ, van den Burg HA (2018) The Arabidopsis SUMO E3 ligase SIZ1 mediates the temperature dependent trade-off between plant immunity and growth. PLoS Genetics 14, e1007157.
| Crossref | Google Scholar | PubMed |
Han J, Mu Y, Huang J (2023) Preserving genome integrity: the vital role of SUMO-targeted ubiquitin ligases. Cell Insight 2, 100128.
| Crossref | Google Scholar | PubMed |
Hong Y, Chen Y, Shi H, Kong X, Yao J, Lei M, Zhu J-K, Wang Z (2022) SUMO E3 ligase SIZ1 negatively regulates arsenite resistance via depressing GSH biosynthesis in Arabidopsis. Stress Biology 2, 9.
| Crossref | Google Scholar | PubMed |
Huang L, Yang S, Zhang S, Liu M, Lai J, Qi Y, Shi S, Wang J, Wang Y, Xie Q, Yang C (2009) The Arabidopsis SUMO E3 ligase AtMMS21, a homologue of NSE2/MMS21, regulates cell proliferation in the root. The Plant Journal 60, 666-678.
| Crossref | Google Scholar | PubMed |
Ishida T, Fujiwara S, Miura K, Stacey N, Yoshimura M, Schneider K, Adachi S, Minamisawa K, Umeda M, Sugimoto K (2009) SUMO E3 ligase HIGH PLOIDY2 regulates endocycle onset and meristem maintenance in Arabidopsis. The Plant Cell 21, 2284-2297.
| Crossref | Google Scholar | PubMed |
Jiang J, Xie Y, Du J, Yang C, Lai J (2021) A SUMO ligase OsMMS21 regulates rice development and auxin response. Journal of Plant Physiology 263, 153447.
| Crossref | Google Scholar | PubMed |
Jiang H, Zhou L-J, Gao H-N, Wang X-F, Li Z-W, Li Y-Y (2022) The transcription factor MdMYB2 influences cold tolerance and anthocyanin accumulation by activating SUMO E3 ligase MdSIZ1 in apple. Plant Physiology 189, 2044-2060.
| Crossref | Google Scholar | PubMed |
Jin JB, Hasegawa PM (2008) Flowering time regulation by the SUMO E3 ligase SIZ1. Plant Signaling & Behavior 3, 891-892.
| Crossref | Google Scholar | PubMed |
Jin JB, Jin YH, Lee J, et al. (2008) The SUMO E3 ligase, AtSIZ1, regulates flowering by controlling a salicylic acid-mediated floral promotion pathway and through affects on FLC chromatin structure. The Plant Journal 53, 530-540.
| Crossref | Google Scholar | PubMed |
Jing Y, Yang Z, Yang R, Zhang Y, Qiao W, Zhou Y, Sun J (2023) PKL is stabilized by MMS21 to negatively regulate Arabidopsis drought tolerance through directly repressing AFL1 transcription. New Phytologist 239, 920-935.
| Crossref | Google Scholar | PubMed |
Johnson ES (2004) Protein Modification by SUMO. Annual Review of Biochemistry 73, 355-382.
| Crossref | Google Scholar | PubMed |
Joo H, Lim CW, Lee SC (2022) Pepper SUMO E3 ligase CaDSIZ1 enhances drought tolerance by stabilizing the transcription factor CaDRHB1. New Phytologist 235, 2313-2330.
| Crossref | Google Scholar | PubMed |
Kim DY, Han YJ, Kim S-I, Song JT, Seo HS (2015a) Arabidopsis CMT3 activity is positively regulated by AtSIZ1-mediated sumoylation. Plant Science 239, 209-215.
| Crossref | Google Scholar | PubMed |
Kim S-I, Park BS, Kim DY, Yeu SY, Song SI, Song JT, Seo HS (2015b) E3 SUMO ligase AtSIZ1 positively regulates SLY1-mediated GA signalling and plant development. Biochemical Journal 469, 299-314.
| Crossref | Google Scholar | PubMed |
Kim S-I, Kwak JS, Song JT, Seo HS (2016) The E3 SUMO ligase AtSIZ1 functions in seed germination in Arabidopsis. Physiologia Plantarum 158, 256-271.
| Crossref | Google Scholar | PubMed |
Kim JY, Song JT, Seo HS (2017) Post-translational modifications of Arabidopsis E3 SUMO ligase AtSIZ1 are controlled by environmental conditions. FEBS Open Bio 7, 1622-1634.
| Crossref | Google Scholar | PubMed |
Kwak JS, Kim S-I, Park SW, Song JT, Seo HS (2019) E3 SUMO ligase AtSIZ1 regulates the cruciferin content of Arabidopsis seeds. Biochemical and Biophysical Research Communications 519, 761-766.
| Crossref | Google Scholar | PubMed |
Lai R, Jiang J, Wang J, Du J, Lai J, Yang C (2022) Functional characterization of three maize SIZ/PIAS-type SUMO E3 ligases. Journal of Plant Physiology 268, 153588.
| Crossref | Google Scholar | PubMed |
Lascorz J, Codina-Fabra J, Reverter D, Torres-Rosell J (2022) SUMO-SIM interactions: from structure to biological functions. Seminars in Cell & Developmental Biology 132, 193-202.
| Crossref | Google Scholar | PubMed |
Lee J, Nam J, Park HC, et al. (2007) Salicylic acid-mediated innate immunity in Arabidopsis is regulated by SIZ1 SUMO E3 ligase. The Plant Journal 49, 79-90.
| Crossref | Google Scholar | PubMed |
Lei S, Wang Q, Chen Y, Song Y, Zheng M, Hsu Y-F (2022) Capsicum SIZ1 contributes to ABA-induced SUMOylation in pepper. Plant Science 314, 111099.
| Crossref | Google Scholar | PubMed |
Li Z, Hu Q, Zhou M, Vandenbrink J, Li D, Menchyk N, Reighard S, Norris A, Liu H, Sun D, Luo H (2013) Heterologous expression of OsSIZ1, a rice SUMO E3 ligase, enhances broad abiotic stress tolerance in transgenic creeping bentgrass. Plant Biotechnology Journal 11, 432-445.
| Crossref | Google Scholar | PubMed |
Li R, Ma J, Liu H, Wang X, Li J, Li Z, Li M, Sui S, Liu D (2021) Overexpression of CpSIZ1, a SIZ/PIAS-type SUMO E3 ligase from wintersweet (Chimonanthus praecox), delays flowering, accelerates leaf senescence and enhances cold tolerance in Arabidopsis. Plant Molecular Biology Reporter 39, 301-316.
| Crossref | Google Scholar |
Liao X, Sun J, Li Q, Ding W, Zhao B, Wang B, Zhou S, Wang H (2023) ZmSIZ1a and ZmSIZ1b play an indispensable role in resistance against fusarium ear rot in maize. Molecular Plant Pathology 24, 711-724.
| Crossref | Google Scholar | PubMed |
Lin F-M, Kumar S, Ren J, Karami S, Bahnassy S, Li Y, Zheng X, Wang J, Bawa-Khalfe T (2016) SUMOylation of HP1α supports association with ncRNA to define responsiveness of breast cancer cells to chemotherapy. Oncotarget 7, 30336-30349.
| Crossref | Google Scholar | PubMed |
Ling Y, Zhang C, Chen T, Hao H, Liu P, Bressan RA, Hasegawa PM, Jin JB, Lin J (2012) Mutation in SUMO E3 ligase, SIZ1, disrupts the mature female gametophyte in Arabidopsis. PLoS ONE 7, e29470.
| Crossref | Google Scholar | PubMed |
Liu M, Shi S, Zhang S, Xu P, Lai J, Liu Y, Yuan D, Wang Y, Du J, Yang C (2014) SUMO E3 ligase AtMMS21 is required for normal meiosis and gametophyte development in Arabidopsis. BMC Plant Biology 14, 153.
| Crossref | Google Scholar | PubMed |
Liu F, Wang X, Su M, Yu M, Zhang S, Lai J, Yang C, Wang Y (2015) Functional characterization of DnSIZ1, a SIZ/PIAS-type SUMO E3 ligase from Dendrobium. BMC Plant Biology 15, 225.
| Crossref | Google Scholar | PubMed |
Mercier C, Roux B, Have M, et al. (2021) Root responses to aluminium and iron stresses require the SIZ1 SUMO ligase to modulate the STOP1 transcription factor. The Plant Journal 108, 1507-1521.
| Crossref | Google Scholar | PubMed |
Minty A, Dumont X, Kaghad M, Caput D (2000) Covalent modification of p73α by SUMO-1: two-hybrid screening with p73 identifies novel SUMO-1-interacting proteins and a SUMO-1 interaction motif. Journal of Biological Chemistry 275, 36316-36323.
| Crossref | Google Scholar | PubMed |
Mishra N, Sun L, Zhu X, et al. (2017) Overexpression of the rice SUMO E3 ligase gene OsSIZ1 in cotton enhances drought and heat tolerance, and substantially improves fiber yields in the field under reduced irrigation and rainfed conditions. Plant and Cell Physiology 58, 735-746.
| Crossref | Google Scholar | PubMed |
Mishra N, Srivastava AP, Esmaeili N, Hu W, Shen G (2018) Overexpression of the rice gene OsSIZ1 in Arabidopsis improves drought-, heat-, and salt-tolerance simultaneously. PLoS ONE 13, e0201716.
| Crossref | Google Scholar | PubMed |
Miura K, Hasegawa PM (2009) Sumoylation and abscisic acid signaling. Plant Signaling & Behavior 4, 1176-1178.
| Crossref | Google Scholar | PubMed |
Miura K, Rus A, Sharkhuu A, Yokoi S, Karthikeyan AS, Raghothama KG, Baek D, Koo YD, Jin JB, Bressan RA, Yun D-J, Hasegawa PM (2005) The Arabidopsis SUMO E3 ligase SIZ1 controls phosphate deficiency responses. Proceedings of the National Academy of Sciences United States of America 102, 7760-7765.
| Crossref | Google Scholar |
Miura K, Jin JB, Lee J, Yoo CY, Stirm V, Miura T, Ashworth EN, Bressan RA, Yun D-J, Hasegawa P (2007) SIZ1-mediated sumoylation of ICE1 controls CBF3/DREB1A expression and freezing tolerance in Arabidopsis. The Plant Cell 19, 1403-1414.
| Crossref | Google Scholar | PubMed |
Miura K, Lee J, Jin JB, Yoo CY, Miura T, Hasegawa PM (2009) Sumoylation of ABI5 by the Arabidopsis SUMO E3 ligase SIZ1 negatively regulates abscisic acid signaling. Proceedings of the National Academy of Sciences United States of America 106, 5418-5423.
| Crossref | Google Scholar |
Miura K, Lee J, Miura T, Hasegawa PM (2010) SIZ1 controls cell growth and plant development in Arabidopsis through salicylic acid. Plant and Cell Physiology 51, 103-113.
| Crossref | Google Scholar | PubMed |
Miura K, Lee J, Gong Q, Ma S, Jin JB, Yoo CY, Miura T, Sato A, Bohnert HJ, Hasegawa PM (2011) SIZ1 regulation of phosphate starvation-induced root architecture remodeling involves the control of auxin accumulation. Plant Physiology 155, 1000-1012.
| Crossref | Google Scholar | PubMed |
Miura K, Okamoto H, Okuma E, Shiba H, Kamada H, Hasegawa PM, Murata Y (2013) SIZ1 deficiency causes reduced stomatal aperture and enhanced drought tolerance via controlling salicylic acid-induced accumulation of reactive oxygen species in Arabidopsis. The Plant Journal 73, 91-104.
| Crossref | Google Scholar | PubMed |
Muhammad W, Mehtab MA, Sunil KS (2024) Understanding the mechanistic basis of plant adaptation to salinity and drought. Functional Plant Biology 51, FP23216,.
| Crossref | Google Scholar |
Okushima Y, Shimizu K, Ishida T, Sugimoto K, Umeda M (2014) Differential regulation of B2-type CDK accumulation in Arabidopsis roots. Plant Cell Reports 33, 1033-1040.
| Crossref | Google Scholar | PubMed |
Park HJ, Yun D-J (2013) New insights into the role of the small ubiquitin-like modifier (SUMO) in plants. International Review of Cell and Molecular Biology 300, 161-209.
| Crossref | Google Scholar | PubMed |
Park HC, Kim H, Koo SC, et al. (2010) Functional characterization of the SIZ/PIAS-type SUMO E3 ligases, OsSIZ1 and OsSIZ2 in rice. Plant, Cell & Environment 33, 1923-1934.
| Crossref | Google Scholar | PubMed |
Park HJ, Kim W-Y, Park HC, Lee SY, Bohnert HJ, Yun D-J (2011a) SUMO and SUMOylation in plants. Molecules and Cells 32, 305-316.
| Crossref | Google Scholar | PubMed |
Park BS, Song JT, Seo HS (2011b) Arabidopsis nitrate reductase activity is stimulated by the E3 SUMO ligase AtSIZ1. Nature Communications 2, 400.
| Crossref | Google Scholar | PubMed |
Pei W, Jain A, Sun Y, Zhang Z, Ai H, Liu X, Wang H, Feng B, Sun R, Zhou H, Xu G, Sun S (2017) OsSIZ2 exerts regulatory influences on the developmental responses and phosphate homeostasis in rice. Scientific Reports 7, 12280.
| Crossref | Google Scholar | PubMed |
Pei W, Jain A, Ai H, Liu X, Feng B, Wang X, Sun Y, Xu G, Sun S (2019) OsSIZ2 regulates nitrogen homeostasis and some of the reproductive traits in rice. Journal of Plant Physiology 232, 51-60.
| Crossref | Google Scholar | PubMed |
Perez-Torres C-A, Lopez-Bucio J, Cruz-Ramı’rez A, Ibarra-Laclette E, Dharmasiri S, Estelle M, Herrera-Estrella L (2008) Phosphate availability alters lateral root development in Arabidopsis by modulating auxin sensitivity via a mechanism involving the TIR1 auxin receptor. The Plant Cell 20, 3258-3272.
| Crossref | Google Scholar |
Pichler A, Gast A, Seeler JS, Dejean A, Melchior F (2002) The nucleoporin RanBP2 has SUMO1 E3 ligase activity. Cell 108, 109-120.
| Crossref | Google Scholar | PubMed |
Potts PR, Yu H (2005) Human MMS21/NSE2 is a SUMO ligase required for DNA repair. Molecular and Cellular Biology 25, 7021-7032.
| Crossref | Google Scholar |
Psakhye I, Jentsch S (2012) Protein group modification and synergy in the SUMO pathway as exemplified in DNA repair. Cell 151, 807-820.
| Crossref | Google Scholar | PubMed |
Rubio V, Linhares F, Solano R, Martín AC, Iglesias J, Leyva A, Paz-Ares J (2001) A conserved MYB transcription factor involved in phosphate starvation signaling both in vascular plants and in unicellular algae. Genes & Development 15, 2122-2133.
| Crossref | Google Scholar | PubMed |
Rytz TC, Miller MJ, McLoughlin F, Augustine RC, Marshall RS, Juan Y-T, Charng Y-Y, Scalf M, Smith LM, Vierstra RD (2018) SUMOylome profiling reveals a diverse array of nuclear targets modified by the SUMO ligase SIZ1 during heat stress. The Plant Cell 30, 1077-1099.
| Crossref | Google Scholar | PubMed |
Sarangi P, Zhao X (2015) SUMO-mediated regulation of DNA damage repair and responses. Trends in Biochemical Sciences 40, 233-242.
| Crossref | Google Scholar | PubMed |
Son GH, Park BS, Song JT, Seo HS (2014) FLC-mediated flowering repression is positively regulated by sumoylation. Journal of Experimental Botany 65, 339-351.
| Crossref | Google Scholar | PubMed |
Thangasamy S, Guo C-L, Chuang M-H, Lai M-H, Chen J, Jauh G-Y (2011) Rice SIZ1, a SUMO E3 ligase, controls spikelet fertility through regulation of anther dehiscence. New Phytologist 189, 869-882.
| Crossref | Google Scholar | PubMed |
Tomanov K, Zeschmann A, Hermkes R, Eifler K, Ziba I, Grieco M, Novatchkova M, Hofmann K, Hesse H, Bachmair A (2014) Arabidopsis PIAL1 and 2 promote SUMO chain formation as E4-type SUMO ligases and are involved in stress responses and sulfur metabolism. The Plant Cell 26, 4547-4560.
| Crossref | Google Scholar | PubMed |
Tsuge M, Kaneoka H, Masuda Y, Ito H, Miyake K, Iijima S (2015) Implication of SUMO E3 ligases in nucleotide excision repair. Cytotechnology 67, 681-687.
| Crossref | Google Scholar | PubMed |
Varejão N, Lascorz J, Li Y, Reverter D (2020) Molecular mechanisms in SUMO conjugation. Biochemical Society Transactions 48, 123-135.
| Crossref | Google Scholar | PubMed |
Varejão N, Lascorz J, Codina-Fabra J, Bellí G, Borràs-Gas H, Torres-Rosell J, Reverter D (2021) Structural basis for the E3 ligase activity enhancement of yeast Nse2 by SUMO-interacting motifs. Nature Communications 12, 7013.
| Crossref | Google Scholar | PubMed |
Vertegaal ACO (2022) Signalling mechanisms and cellular functions of SUMO. Nature Reviews Molecular Cell Biology 23, 715-731.
| Crossref | Google Scholar | PubMed |
Wang X, Du G, Wang X, Meng Y, Li Y, Wu P, Yi K (2010) The function of LPR1 is controlled by an element in the promoter and is independent of SUMO E3 ligase SIZ1 in response to low Pi stress in Arabidopsis thaliana. Plant and Cell Physiology 51, 380-394.
| Crossref | Google Scholar | PubMed |
Wang H, Makeen K, Yan Y, Cao Y, Sun S, Xu G (2011) OsSIZ1 regulates the vegetative growth and reproductive development in rice. Plant Molecular Biology Reporter 29, 411-417.
| Crossref | Google Scholar |
Wang G-L, Zhang C-L, Huo H-Q, Sun X-S, Zhang Y-L, Hao Y-J, You C-X (2022) The SUMO E3 ligase MdSIZ1 sumoylates a cell number regulator MdCNR8 to control organ size. Frontiers in Plant Science 13, 836935.
| Crossref | Google Scholar | PubMed |
Wilkinson KA, Henley JM (2010) Mechanisms, regulation and consequences of protein SUMOylation. Biochemical Journal 428, 133-145.
| Crossref | Google Scholar | PubMed |
Xiong J, Yang F, Wei F, Yang F, Lin H, Zhang D (2023) Inhibition of SIZ1-mediated SUMOylation of HOOKLESS1 promotes light-induced apical hook opening in Arabidopsis. The Plant Cell 35, 2027-2043.
| Crossref | Google Scholar | PubMed |
Xu P, Yuan D, Liu M, Li C, Liu Y, Zhang S, Yao N, Yang C (2013) AtMMS21, an SMC5/6 complex subunit, is involved in stem cell niche maintenance and DNA damage responses in Arabidopsis roots. Plant Physiology 161, 1755-1768.
| Crossref | Google Scholar | PubMed |
Xu J, Zhu J, Liu J, Wang J, Ding Z, Tian H (2021) SIZ1 negatively regulates aluminum resistance by mediating the STOP1-ALMT1 pathway in Arabidopsis. Journal of Integrative Plant Biology 63, 1147-1160.
| Crossref | Google Scholar | PubMed |
Xun Q, Song Y, Mei M, Ding Y, Ding C (2023) The SMC5/6 complex subunit MMS21 regulates stem cell proliferation in rice. Plant Cell Reports 42, 1279-1290.
| Crossref | Google Scholar | PubMed |
Yoo CY, Miura K, Jin JB, Lee J, Park HC, Salt DE, Yun D-J, Bressan RA, Hasegawa PM (2006) SIZ1 small ubiquitin-like modifier E3 ligase facilitates basal thermotolerance in Arabidopsis independent of salicylic acid. Plant Physiology 142, 1548-1558.
| Crossref | Google Scholar | PubMed |
Yuan H, Liu D (2008) Signaling components involved in plant responses to phosphate starvation. Journal of Integrative Plant Biology 50, 849-859.
| Crossref | Google Scholar | PubMed |
Zhang S, Qi Y, Yang C (2010) Arabidopsis SUMO E3 ligase AtMMS21 regulates root meristem development. Plant Signaling & Behavior 5, 53-55.
| Crossref | Google Scholar | PubMed |
Zhang S, Qi Y, Liu M, Yang C (2013) SUMO E3 ligase AtMMS21 regulates drought tolerance in Arabidopsis thaliana. Journal of Integrative Plant Biology 55, 83-95.
| Crossref | Google Scholar | PubMed |
Zhang R-F, Guo Y, Li Y-Y, Zhou L-J, Hao Y-J, You C-X (2016) Functional identification of MdSIZ1 as a SUMO E3 ligase in apple. Journal of Plant Physiology 198, 69-80.
| Crossref | Google Scholar | PubMed |
Zhang S, Zhuang K, Wang S, Lv J, Ma N, Meng Q (2017) A novel tomato SUMO E3 ligase, SlSIZ1, confers drought tolerance in transgenic tobacco. Journal of Integrative Plant Biology 59, 102-117.
| Crossref | Google Scholar | PubMed |
Zhang S, Wang S, Lv J, Liu Z, Wang Y, Ma N, Meng Q (2018) SUMO E3 ligase SlSIZ1 facilitates heat tolerance in tomato. Plant and Cell Physiology 59, 58-71.
| Crossref | Google Scholar | PubMed |
Zhang R-F, Zhou L-J, Li Y-Y, You C-X, Sha G-L, Hao Y-J (2019) Apple SUMO E3 ligase MdSIZ1 is involved in the response to phosphate deficiency. Journal of Plant Physiology 232, 216-225.
| Crossref | Google Scholar | PubMed |
Zhang L, Han Q, Xiong J, Zheng T, Han J, Zhou H, Lin H, Yin Y, Zhang D (2019b) Sumoylation of BRI1-EMS-SUPPRESSOR 1 (BES1) by the SUMO E3 ligase SIZ1 negatively regulates brassinosteroids signaling in Arabidopsis thaliana. Plant and Cell Physiology 60, 2282-2292.
| Crossref | Google Scholar |
Zhang X, Huai J, Liu S, Jin JB, Lin R (2020) SIZ1-mediated SUMO modification of SEUSS regulates photomorphogenesis in Arabidopsis. Plant Communications 1, 100080.
| Crossref | Google Scholar | PubMed |
Zhang C-L, Wang G-L, Zhang Y-L, Hu X, Zhou L-J, You C-X, Li Y-Y, Hao Y-J (2021) Apple SUMO E3 ligase MdSIZ1 facilitates SUMOylation of MdARF8 to regulate lateral root formation. New Phytologist 229, 2206-2222.
| Crossref | Google Scholar | PubMed |
Zhang H, Zhu J, Gong Z, Zhu J-K (2022) Abiotic stress responses in plants. Nature Reviews Genetics 23, 104-119.
| Crossref | Google Scholar | PubMed |
Zhang Y, Xu J, Li R, Ge Y, Li Y, Li R (2023a) Plants’ response to abiotic stress: mechanisms and strategies. International Journal of Molecular Sciences 24, 10915.
| Crossref | Google Scholar | PubMed |
Zhang C, Yang Y, Yu Z, Wang J, Huang R, Zhan Q, Li S, Lai J, Zhang S, Yang C (2023b) SUMO E3 ligase AtMMS21-dependent SUMOylation of AUXIN/INDOLE-3-ACETIC ACID 17 regulates auxin signaling. Plant Physiology 191, 1871-1883.
| Crossref | Google Scholar | PubMed |
Zhang Y, Lyu S, Hu Z, Yang X, Zhu H, Deng S (2023c) Identification and functional characterization of the SUMO system in sweet potato under salt and drought stress. Plant Science 330, 111645.
| Crossref | Google Scholar | PubMed |
Zhang Y-L, Tian Y, Man Y-Y, Zhang C-L, Wang Y, You C-X, Li Y-Y (2023d) Apple SUMO E3 ligase MdSIZ1 regulates cuticular wax biosynthesis by SUMOylating transcription factor MdMYB30. Plant Physiology 191, 1771-1788.
| Crossref | Google Scholar | PubMed |
Zheng Y, Schumaker KS, Guo Y (2012) Sumoylation of transcription factor MYB30 by the small ubiquitin-like modifier E3 ligase SIZ1 mediates abscisic acid response in Arabidopsis thaliana. Proceedings of the National Academy of Sciences, USA 109, 12822-12827.
| Crossref | Google Scholar |
Zheng T, Wu G, Tao X, He B (2022) Arabidopsis SUMO E3 ligase SIZ1 enhances cadmium tolerance via the glutathione-dependent phytochelatin synthesis pathway. Plant Science 322, 111357.
| Crossref | Google Scholar | PubMed |
Zhou X, Du J, Liu Y, Yang C, Lai J (2019a) Functional characterization of DiMMS21, a SUMO ligase from Desmodium intortum. Plant Physiology and Biochemistry 141, 206-214.
| Crossref | Google Scholar | PubMed |
Zhou L-J, Zhang C-L, Zhang R-F, Wang G-L, Li Y-Y, Hao Y-J (2019b) The SUMO E3 ligase MdSIZ1 targets MdbHLH104 to regulate plasma membrane H+-ATPase activity and iron homeostasis. Plant Physiology 179, 88-106.
| Crossref | Google Scholar | PubMed |