Identification of cysteine-rich receptor-like kinase gene family in potato: revealed StCRLK9 in response to heat, salt and drought stresses
Roshan Zameer
A
B
C
D
Handling Editor: Sajid Fiaz
Abstract
The investigation into cysteine-rich receptor-like kinases (CRLKs) holds pivotal significance as these conserved, upstream signalling molecules intricately regulate fundamental biological processes such as plant growth, development and stress adaptation. This study undertakes a comprehensive characterisation of CRLKs in Solanum tuberosum (potato), a staple food crop of immense economic importance. Employing comparative genomics and evolutionary analyses, we identified 10 distinct CRLK genes in potato. Further categorisation into three major groups based on sequence similarity was performed. Each CRLK member in potato was systematically named according to its chromosomal position. Multiple sequence alignment and phylogenetic analyses unveiled conserved gene structures and motifs within the same groups. The genomic distribution of CRLKs was observed across Chromosomes 2–5, 8 and 12. Gene duplication analysis highlighted a noteworthy trend, with most gene pairs exhibiting a Ka/Ks ratio greater than one, indicating positive selection of StCRLKs in potato. Salt and drought stresses significantly impacted peroxidase and catalase activities in potato seedlings. The presence of diverse cis-regulatory elements, including hormone-responsive elements, underscored their involvement in myriad biotic and abiotic stress responses. Interestingly, interactions between the phytohormone auxin and CRLK proteins unveiled a potential auxin-mediated regulatory mechanism. A holistic approach combining transcriptomics and quantitative PCR validation identified StCRLK9 as a potential candidate involved in plant response to heat, salt and drought stresses. This study lays a robust foundation for future research on the functional roles of the CRLK gene family in potatoes, offering valuable insights into their diverse regulatory mechanisms and potential applications in stress management.
Keywords: 3D structure, abiotic stresses, auxin, CRLKs, docking, expression, Solanum tuberosum.
Introduction
Potato (Solanum tuberosum) is the world’s third-largest staple food crop, followed by rice and wheat (Wang et al. 2023). The highly nutritious nature and large number of essential nutrients enable the Chinese government to consider potato as a staple food (Hill et al. 2021; Han et al. 2023). China globally ranks third in salinised soil, the northeastern region being particularly affected (Yang et al. 2018). Salinised soil conditions cause delayed germination and severe root damage, which lead to overall yield loss (Shabala et al. 2015). Global warming-induced temperature rises can significantly impact plant growth, particularly heat-sensitive crops like potatoes, leading to reduced leaf size and disrupted tuber initiation, and affecting physiological processes (Wang et al. 2003; Mittler 2006; Tang et al. 2018; Azeem et al. 2021). The drought condition can directly impact photosynthesis, i.e. lowering chlorophyll content (Nasir and Toth 2022); the vegetative stage, i.e. reducing plant size (Deblonde and Ledent 2001); and the reproductive stage, i.e. shortening the growth cycle (Kumar et al. 2007). Although extensive research has focused on developing resilient crops, the latest potato genome assemblies enable more thorough investigations to develop resilient crops to mitigate climate-induced losses, to enhance the crop yield and reduce input resources (Huang et al. 2021). This balance is crucial for economic sustainability and food security for the increasing population.
Receptor-like protein kinases (RLKs), a large group of plant-specific membrane proteins, have a crucial role in plant development, abiotic stress responses, plant immunity and symbiosis (Cui et al. 2022). ZmPK1 was the first identified RLK family member from maize (Walker and Zhang 1990). A total of approximately 600 members of this family have been identified in model plant Arabidopsis thaliana and 1100 in rice (Oryza sativa) (Shiu and Bleecker 2003; Shiu et al. 2004). In general, a C-terminal cytoplasmic domain (CD), N-terminal extracellular domain (ED) and single-pass transmembrane domain are present in RLKs (Ou et al. 2021).
Cysteine-rich receptor-like kinases (CRLKs) are the largest subgroup family of RLKs with a highly conserved DUF26-related domain (Gnk2) in higher plants. This domain consists of three consecutive conserved cysteine residues (C-X8-C-X2-C) (Chen 2001). Previous studies indicate the dynamic regulation of expression patterns of CRLK genes in response to different abiotic stresses, e.g. salt stress (Tanaka et al. 2012), drought stress (Shumayla et al. 2019), visible light and heat stress (Liu et al. 2021) and cold stress (Campos Mantello et al. 2019). Some genes are upregulated at specific stages, whereas others are downregulated at the same stages. For instance, in particular stress conditions, certain genes are initially upregulated, but their expression decreases as the stress duration changes. Additionally, AtPR5Ks were identified in abscisic acid (ABA)-mediated drought stress responses. Only the atpr5k2-1 mutant exhibited drought tolerance, whereas AtPR5K2-overexpressing plants showed drought hypersensitivity. AtPR5K2 interacts with key ABA signalling components (ABI1, ABI2 and SnRK2.6), and this interaction is disrupted by exogenous ABA, suggesting AtPR5K2 acts as a negative regulator of ABA signalling in drought conditions (Umezawa et al. 2010; Baek et al. 2019). Similarly, OsSRK1, an unconventional S-receptor-like kinase, displays increased expression under ABA, salt and polyethylene glycol (PEG) 4000 exposure. Although OsSRK1-overexpressing (OsSRK1-OX) plants exhibit ABA sensitivity, they surprisingly demonstrate enhanced salt stress tolerance compared to the wild type (Jinjun et al. 2020). Whereas the CRLK gene family is extensively studied in model plants like Arabidopsis (Burdiak et al. 2015) and rice (Chern et al. 2016), information regarding their role in potatoes is lacking. The advanced genomics and omics-assisted techniques allow investigation of the CRLK gene family, its functional significance, and the molecular mechanism of stress regulation in potato.
Auxin plays a pivotal role in plant growth and development. It was first discovered by Went in 1928 and is recognised as a key positive regulator in various physiological processes in plants (Went 1928; Caumon and Vernoux 2023). Numerous studies have previously reported that auxin mediates the regulation of various genes that are involved in plant development and growth (Shin et al. 2007; Koyama et al. 2010; Sakamoto et al. 2013; Li et al. 2021). In Populus, PagFBL1 regulates adventitious root growth through auxin/indole-3-acetic acid interaction (Shu et al. 2019). Another study highlights heat shock protein 90 (HSP90) stabilising the auxin receptor for enhanced plant plasticity under abiotic stress (Watanabe et al. 2017). Particularly for improving drought stress resistance in plants, auxin acts as a mediator in promoting the root branches by regulating YUC and PIN in tobacco (Nicotiana tabacum) (Wang et al. 2018). Various stress-related pathways interact with auxin-related gene regulatory networks. This highlights the auxin’s role as a central hub that integrates genetic and environmental information, ultimately leading to the appropriate developmental and physiological responses in plants (Shani et al. 2017).
The current study was purposed for homology-based identification and conserved structural-based characterisation of CRLK family members in Solanum tuberosum (potato). In this study, we analyse the gene structures, identify conserved motifs, and elucidate the phylogenetic relationship with model plants. Furthermore, transcriptome data analysis for expression profiling and quantitative PCR (qPCR) validation provides an in-depth understanding of CRLK family members’ involvement in response to abiotic stress conditions. Various biochemical studies were performed to analyse the impact of abiotic stresses on physiological activities in potato. 3D structure prediction and its potential binding with auxin-related elements were also performed. The present work establishes the groundwork for further investigations into the role of the potato CRLKs gene family in engineering climate-resilient future crop plants.
Materials and methods
Identification of StCRLK genes in potato
The CRKs in Arabidopsis thaliana L. were used to identify this family in potato. All the published protein sequences for CRKs in Arabidopsis were retrieved and subjected to the BLASTP program (https://blast.ncbi.nlm.nih.gov/Blast.cgi?PAGE=Proteins) as a query against the potato genome. The largest open reading frames were taken as candidates, with a threshold of e < 1e−5 and 50% identity. After removing the redundant sequences manually, the potential candidate genes for CRLKs were screened for the presence of a conserved motif consisting of three cysteine residues (C-X8-C-X2-C) (Chen 2001). Weblogo3 (https://weblogo.threeplusone.com/create.cgi) is an online tool used for the graphical representation of this conserved motif. Meanwhile various domain prediction tools, namely SMART (http://smart.embl-heidelberg.de/), Pfam (https://pfam.xfam.org/) and NCBI-CDD web server (https://www.ncbi.nlm.nih.gov/Structure/cdd/wrpsb.cgi), were used to further confirm the presence of CRLK-related domains and construct a domain architecture accordingly. The putative confirmed members were renamed according to their chromosomal position. The genomic information, consisting of start site, chromosome number, and translated protein length, was retrieved from NCBI-gene database. The amino acid sequences of putative candidates genes were analysed in the ExPASy server (https://web.expasy.org/) to detect various physiochemical properties, i.e. theoretical isoelectric point (PI) and molecular weight (MW), and a web server ‘cello’ (http://cello.life.nctu.edu.tw/) was utilised to detect the sub-cellular location of these proteins.
Phylogeny, gene structure and motif prediction of StCRLK in potato
The published protein sequences of CRKs in Arabidopsis were retrieved from the TAIR database (https://www.arabidopsis.org/) (Idänheimo et al. 2014). The multiple sequence alignment of these CRKs protein sequences along with potential candidates of StCRLKs were performed using CLUSTALW (https://www.genome.jp/tools-bin/clustalw) with default parameters. The phylogenetic tree was constructed using MEGA 7.0 software with a Neighbor-Joining method, and bootstrap analysis was conducted using 1000 iterations with a pair-wise gap deletion mode (Kumar et al. 2016). The interactive editing was performed by using an online tool iTOL (https://itol.embl.de/). The full-length protein sequence of CRLKs from Arabidopsis and potato (Solanum tuberosum L.) were subjected to an online tool MEME (https://meme-suite.org/meme/) for motif-based comparison. The following parameters were adjusted: the length of motif 10–300 aa, the maximum number of motifs was limited to 15, with other parameters at the default setting. The NCBI-Gene database was used to retrieve the genomic sequences and their corresponding coding sequences for Arabidopsis and potato. These sequences were subjected to an online server GSDS (https://gsds.gao-lab.org/) to examine the comparative intron–exon distribution in Arabidopsis and potato.
Chromosomal mapping, comparative phylogenetics and evolutionary analysis
The genomic coordinates of all the genes, i.e. start and end positions, were subjected to the MapChart program (Voorrips 2002). This program provides an interactive visualisation of the physical locations of genes on chromosomes. To investigate the adaptation of genes across diverse species, we retrieve the already published sequence of CRKs in Arabidopsis, rice (Oryza sativa L.) and cucumber (Cucumis sativus L.). These sequences from diverse species along with the sequences in potato were subjected to CLUSTALW for alignment, and then MEGA 7.0 software was utilised to construct a phylogenetic tree with maximum likelihood algorithm with bootstrap method at 1000 replicates. The DNAsp tool was used to calculate non-synonymous (ka) and synonymous (ks) substitution rates between the members of CRLKs to investigate the gene duplication events in potato (Rozas et al. 2017). The divergence time was also calculated [T = Ks/2λ × 10−6 Mya (λ = 6.5 × 10−9)] (Zhu et al. 2019).
In silico promoter analysis and expression profiling of StCRLKs in potato
The upstream region of 1000 bp of all StCRLK genes were subjected to PlantCARE (https://bioinformatics.psb.ugent.be/webtools/plantcare/html/) to detect the cis-acting regulatory elements. These elements are regulatory regions that control how genes respond to various signals, i.e. abiotic or biotic stresses (Lescot et al. 2002). The publicly available RNA sequencing (RNA-seq) data was utilised to detect the expression pattern of CRLKs in potato. A bioproject of RNA-seq data with accession number PRJNA556372 was used to detect the expression in response to heat stress at various stages, i.e. 0 h, 6 h, 24 h, 48 h, 72 h and 96 h (Xi et al. 2020). Another project with accession number PRJNA882516 was used to detect the expression under salt stress at various stages, i.e. 0 h, 3 h, 6 h, 12 h and 24 h (Jing et al. 2022). A project with accession number PRJNA879907 was used to detect the expression under drought stress in control, moderate (5% PEG) and severe (10% PEG) conditions. For all the replicated experiments, statistics are presented as the average of all the replicates ± s.d. (standard deviation). All clean reads were mapped to the reference genome using the bowtie2 program (Langmead and Salzberg 2012). The SolTub_3.0 genome assembly was used as reference genome (Xu et al. 2011). The expression was estimated using the FPKM (fragments per kilobase of transcript per million fragments mapped) value according to the total number of query reads mapped to the corresponding sequence in the reference genome using the cufflinks program (Trapnell et al. 2012). TBtools software was utilised for graphical visualisation of expression data (Chen et al. 2020).
Experimental setup and stress treatments
A potato cultivar Desiree was sown in pots. The pots were maintained in a growth chamber controlled at 21 ± 2°C with a 16 h light/8 h dark photoperiod. Hoagland’s solution was provided once per week. The seedlings (height ~20 cm) were placed into three groups I, II and III for application of different treatments, i.e. heat, salt and drought, respectively. For heat stress, the pots in Group I were placed in a growth chamber with a rise in temperature to 37°C. For salt stress, 100 mM NaCl solution was directly applied to the pots in Group II. For drought stress, Hoagland’s solution containing 25% PEG-6000 was applied to the pots in Group III. The matured leaf samples in triplicates were collected at various treatment stages: (1) 0 h, 6 h, 12 h and 24 h; (2) 0, 2 days, 4 days and 8 days; (3) 0, 1 days, 2 days, 4 days for heat stress, salt stress and drought stress, respectively. The collected samples were quickly frozen in liquid nitrogen and stored at −80°C until further use.
Quantitative reverse transcription PCR (qRT-PCR) validation
Total RNA was extracted from all the samples using RNAprep Pure Plant Plus Kit (Cat# DP441, TIANGEN). The reverse transcription was performed using ReverTra Ace™ qPCR RT Master Mix with gDNA Remover (Cat# FSD-301_226900) by following the manufacturer’s instructions. The gene-specific primers were designed for 10 family members of CRLKs in potato using the NCBI primer-blast program and the specificity was confirmed using the online tool oligo calculator (Table S1). qRT-PCR was performed using a real-time PCR detection system (The LightCycler® 480 II, Roche) with ChamQ Universal SYBR qPCR Master Mix. A housekeeping gene β-actin from potato was used as an internal control (Locus tag: LOC102582178: TTTCGCTGTATGCCAGTGGT-F, AAGAATGGCATGTGGGAGGG-R) to normalise the expression data. The qRT-PCR analysis was repeated in three independent experiments.
Biochemical studies
The antioxidant activities of peroxidase (POD), superoxide dismutase (SOD), catalase (CAT) and total soluble protein were measured in response to heat, salt and drought stress. The fresh leaves (0.5 g) were ground in 1 mL phosphate buffer. The 50 μL of the homogenised sample was added into the cuvette and then 700 μL potassium phosphate buffer, 100 μL guaiacol and 100 μL H2O2 were added. After gently mixing the solution, a spectrophotometer was used to record the absorbance at 470 nm to measure the POD activity (Chance and Maehly 1955). Another 50 μL of the homogenised sample was added into the cuvette and then 50 μL nitro blue tetrazolium (NBT), 50 μL riboflavin, 250 μL potassium phosphate buffer, 100 μL methionine, 100 μL triton-X and 400 μL distilled water were added and kept under heavy light for 15 min. After gently mixing, the solution was used to measure SOD activity by recording the absorbances at 560 nm with a spectrophotometer (Kono 1978). A total of 0.1 mL of above enzyme extract was mixed with 1 mL H2O2 and 2.8 mL phosphate buffer. The absorbance was measured at 240 nm for catalase estimation (Chance and Maehly 1955). Next, 0.5 g of plant fresh leaf was ground in 10 mL buffer and centrifuged at 13,500g for 10 min at 4°C. Then, 100 μL aqueous phase with 2 mL Bradford reagent was added to the test tube and kept for 15 min. The absorbance was taken at 595 nm under a spectrophotometer for measuring the total soluble proteins (Bradford 1976). The statistics analysis was performed on all replicates.
Protein structure modelling and molecular docking with auxin
The functional characterisation of proteins heavily relies on their three-dimensional (3D) structures, pivotal for understanding their interactions with other proteins or molecules. These interactions may unveil their potential roles in mediating crucial processes associated with plant growth and development. The protein sequences of all the CRLK family members were subjected as input to AlphaFold2 for 3D structure prediction (Jumper et al. 2021). All the 3D structures were further validated by PROCHECK and ERRAT servers. These predicted structures were subjected to UCSF Chimera software for energy minimisation (Pettersen et al. 2004). Then the crystal structure of indole-3-acetic acid CCDC number 141657, (C10H9NO2) was downloaded from PubChem-NCBI database (https://pubchem.ncbi.nlm.nih.gov/). The minimised 3D structures and ligands were subjected to the CB-DOCK online server for blind docking (https://cadd.labshare.cn/cb-dock2/php/index.php). The interactions between protein and ligand were visualised on Cytoscape software (Shannon et al. 2003).
Results
Identification of StCRLKs in potato
A whole genome-wide identification revealed that a total of 10 CRLKs were identified in potato. In this study the BLASTP program was used to detect potential candidates and then cross-verified through the presence of CRLK-related domains. The results revealed that all the family members contain two Gnk2-homologous domains present at the N-terminus and a protein kinase domain at the C-terminus; except StCRLK2, which consists of one Gnk2-homologous domain at the N-terminus and one protein kinase domain at the C-terminus. Gnk2-homologous domains contain signature motifs for CRLKs family members (C-X8-C-X2-C). In this domain cysteine residues form three intramolecular disulfide bridges. Multiple sequence alignment of CRLKs in potato revealed that the above signature motif is conserved among all family members, a logo for this alignment was generated and is presented (Fig. 1). These 10 proteins were renamed from StCRLK1 to StCRLK10 according to the arrangement on the chromosomes. The members of CRLK family are distributed on six out of twelve chromosomes. These family members were subjected to the NCBI database to get the basic information, i.e. chromosome number (chr), amino acid length (aa) and gene start site (gene S. site). The amino acid lengths of StCRLKs vary, with the shortest length of 595 residues and the longest length extending to 676 residues. Furthermore, these family members were subjected to the Expasy protparam tool to detect physiochemical properties, i.e. molecular weight (MWs) and isoelectric point (pI). The results revealed that the molecular weight varied from 70102.04 to 75641.61 Da and the isoelectric point varied from 5.40 to 8.81. The sub-cellular localisation revealed that these family members are present in various cells, i.e. plasma membrane, cytoplasmic and nuclear, but the higher reliability index was found in plasma membrane (Table 1).
Graphical representation of conserved core domains (top) and sequence logos (bottom) of the StCRLKs.
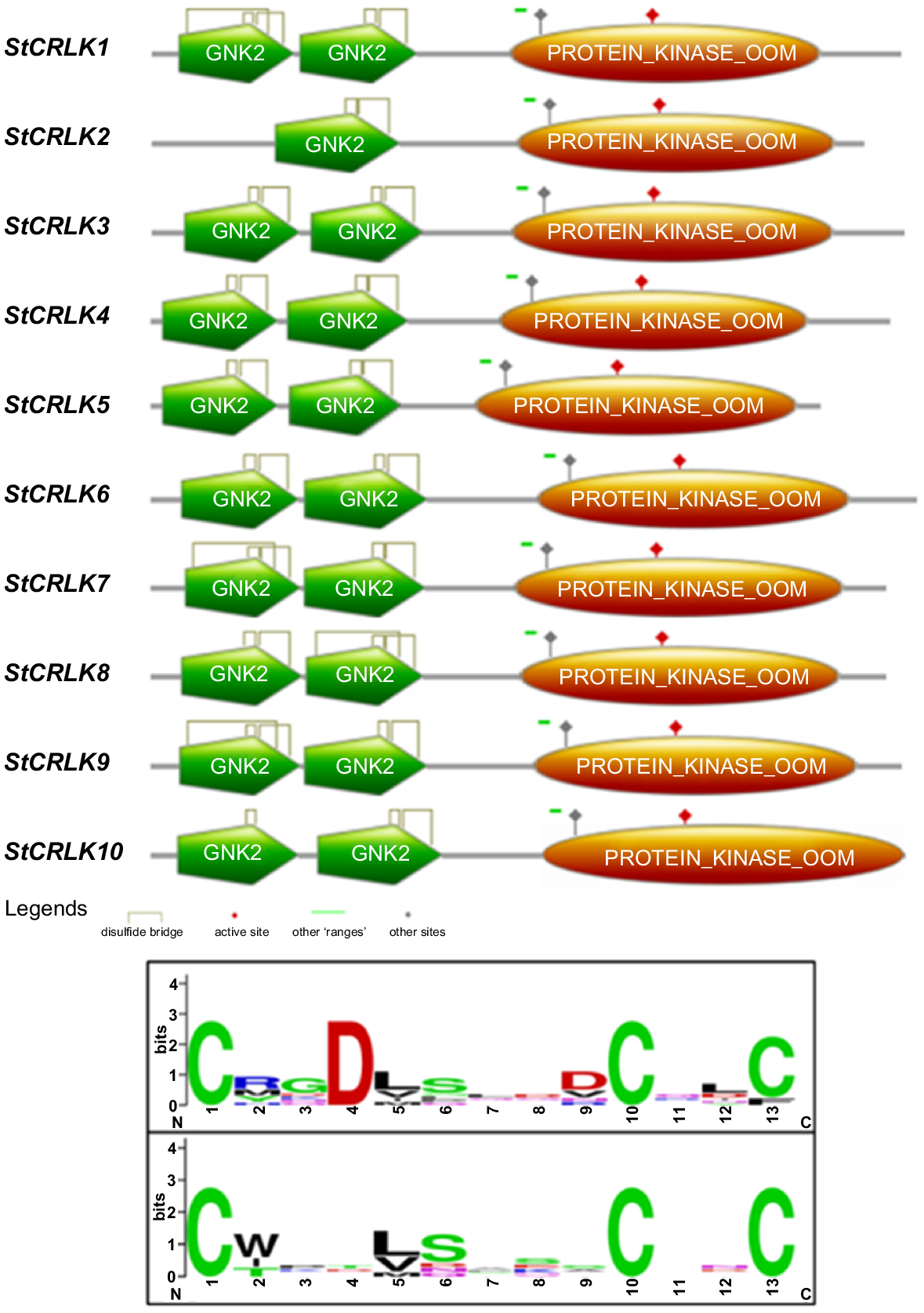
Name | Gene ID | Chr | Length (aa) | Gene S. site | MW (Da) | pI | Sub-cell location | |
---|---|---|---|---|---|---|---|---|
StCRLK1 | PGSC0003DMG400006912 | 2 | 661 | 26500044 | 74525.08 | 5.89 | Plasma membrane | |
StCRLK2 | PGSC0003DMG400013882 | 2 | 629 | 35389834 | 70102.04 | 8.31 | Plasma membrane | |
StCRLK3 | PGSC0003DMG402000515 | 2 | 664 | 47239539 | 72809.02 | 8.63 | Plasma membrane | |
StCRLK4 | PGSC0003DMG400018101 | 3 | 653 | 52493703 | 72274.74 | 6.45 | Plasma membrane | |
StCRLK5 | PGSC0003DMG400029520 | 4 | 595 | 2527219 | 74224.50 | 7.67 | Plasma membrane | |
StCRLK6 | PGSC0003DMG400030553 | 5 | 676 | 3975677 | 75619.14 | 6.31 | Plasma membrane | |
StCRLK7 | PGSC0003DMG400013524 | 5 | 649 | 9453059 | 72526.60 | 6.51 | Plasma membrane | |
StCRLK8 | PGSC0003DMG400013525 | 5 | 649 | 9465568 | 72039.30 | 8.81 | Plasma membrane | |
StCRLK9 | PGSC0003DMG401009323 | 8 | 662 | 48648273 | 73726.84 | 5.40 | Plasma membrane | |
StCRLK10 | PGSC0003DMG400002045 | 12 | 665 | 55752036 | 75641.61 | 6.24 | Plasma membrane |
Comparative phylogenetics, gene structure and motif analysis
The CRLK proteins from Arabidopsis and potato were collectively used for phylogenetic analysis, to study the conserved evolutionary relationship among both plants. Based on these results, the CRLKs were divided into three clades, these clades were named as Class I, Class II and Class III. In Class I, only one family member was found in potato, named StCRLK5. The closest homologous of this family member in Arabidopsis was AtCRK43. In Class II, five family members were found in potato, named as StCRLK1, StCRLK7, StCRLK8, StCRLK3 and StCRLK4. The closest homologues of these family members in Arabidopsis were AtCRK46, AtCRK2, AtCRK3, AtCRK42 and AtCRK1, respectively. In Class III, four family members were found in potato, named StCRLK6, StCRLK2, StCRLK10 and StCRLK9. The closest homologues of these family members in Arabidopsis was AtCRK33 (Fig. 2a). The genomic and coding sequences of all the putative members of CRLK gene family in Arabidopsis and potato were retrieved from the NCBI database. These sequences were submitted to GSDS server to predict the intron–exon organisation. These results revealed that total length of genomic sequence varied from 2712 bp to 4324 bp, and the total length of the coding sequence varied from 1890 bp to 2506 bp. The intron–exon organisation revealed that StCRLK4 has almost the same pattern and number of introns and exons, but is different in size from its counterpart in Arabidopsis (AtCRK1). Similarly, SrCRLK3 has the same pattern and number as AtCRK42, but there is a difference in the size of the first intron. StCRLK8 has the same distribution as AtCRK3, but the latter has an extra exon at Position 2. StCRLK7 has the same pattern as AtCRK2, except StCRLK7 has an extra exon and intron at Position 1. The gene pairs StCRLK1 with AtCRK46, and StCRLK5 with AtCRK43, have variations in their intron–exon structure; although the number of exons and introns are same, there was variation in size and position. Both StCRLK10 and StCRLK9 have the same pattern of introns and exons, except the size of a few introns and exons were different (Fig. 2b). All the protein sequences from Arabidopsis and potato were scanned through the MEME suite. The result revealed that the family members in potato are highly conserved within the sub-classification (Fig. 2c).
Phylogenetic tree, gene structure, and conserved motifs (a) phylogenetic tree among Arabidopsis and potato, Class I represented with black lines, Class II represented with green lines, Class III represented with purple lines. (b) Intron–exon organisation of CRLKs in Arabidopsis and potato, exons presented in green (Arabidopsis) and red (potato), untranslated regions presented in blue, introns presented in black lines. (c) conserved motif organisation in Arabidopsis and potato.
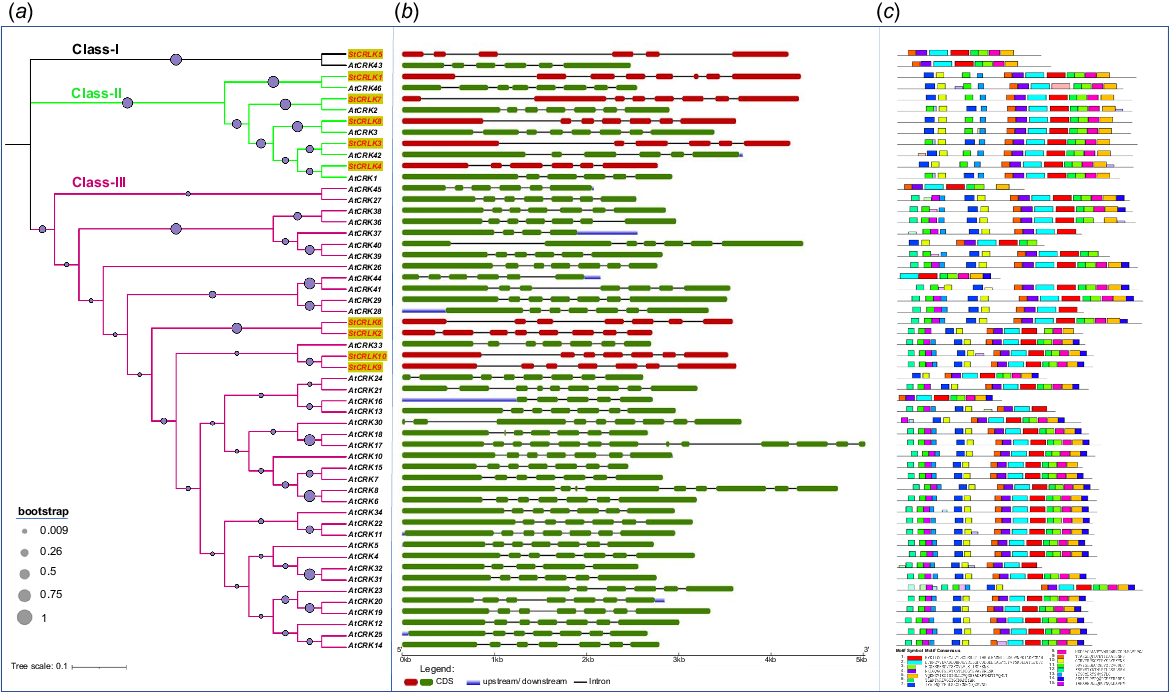
Evolutionary studies in diverse plant species and chromosomal localisation
A phylogenetic tree was constructed based on evolutionary relationships among various plant species, i.e. Arabidopsis, rice, cucumber and potato. The result revealed that all the CRLK family members in potato with their orthologues in Arabidopsis, rice and cucumber were divided into three classes: Class I, Class II and Class III. Class III is further divided into three sub-classes, Class IIIA, Class IIIB and Class IIIC. In Class I, a member of the potato CRLK family, named StCRLK5, had 65% identity with the closest orthologue of this family member in Arabidopsis, named AtCRK43. In the case of Class II, StCRLK8 and StCRLK3 family members from potato had 66% and 57% identity with their closest orthologues in cucumber, named as CsCRK2 and CsCRK1, respectively. Additionally, StCRLK7 had 70% identity with CsCRK3. StCRLK1 had 53% identity with its closest orthologue in Arabidopsis, named AtCRK46. Furthermore, in Class III, two members StCRLK2 and StCRLK6 were found to be in a paralogous relationship with 59% identity, and with their closest orthologue in Arabidopsis, named as AtCRK21, these genes had respectively 51% and 53% identity (Fig. 3a). We constructed a graphical representation of physical location of chromosomes for all the CRLK family members in potato. The results revealed that 10 family members are distributed on six out of twelve chromosomes. Chromosome numbers 3, 4, 8 and 12 have only one family member on each chromosome, named as StCRLK4, StCRLK5, StCRLK9 and StCRLK12 respectively. Meanwhile, Chromosomes 2 and 5 have three family members on each chromosome, named StCRLK1-3 and StCRLK6-8, respectively. The Ka/Ks ratios of most of the segmental duplicates were greater than 1 except between StCRLK1 and StCRLK4, indicating that most gene pairs evolved under the effect of positive selection. Segmentally duplicated pairs are highlighted in various colours (Fig. 3b).
Phylogenetic analysis and chromosomal localisation. (a) Phylogenetic tree between Arabidopsis, rice, cucumber and potato was constructed with bootstrap iterations set to 1000. CRLK family members from potato are presented with green label background. (b) Distribution of CRLK family members in potato on different chromosomes, same colours for different genes represent the duplicated pairs in potato.
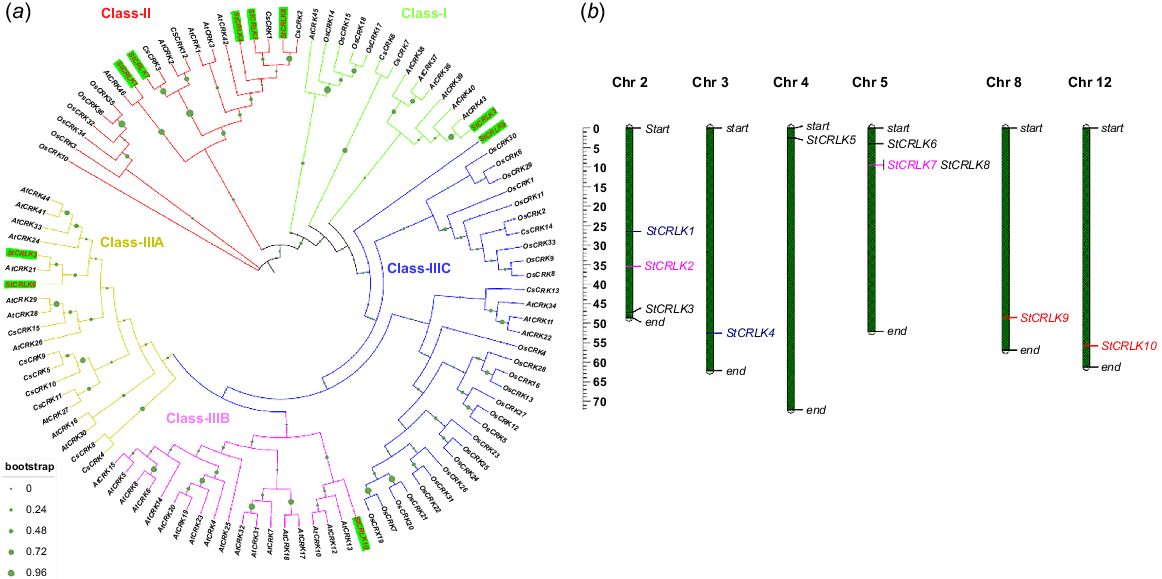
Promoter analysis
In functional genomic studies, exploring genomic regions is crucial to identify transcription factor binding sites and cis-acting elements in the upstream region (typically 1000 to 2000 base pairs) of a gene, providing valuable insights into its regulation and helping to predict its function. In this study, we used 1000 bp upstream region of all the members of CRLKs in potato to predict potential cis-regulatory elements. The results revealed that a large number of cis-regulatory elements were found in upstream region of all the CRLK family members in potato. In particular, 178 CAAT-Box elements and 320 TATA-Box elements were identified in the upstream regions of all the CRLK family members in potato. The ratio of CAAT-Box element is highest in StCRLK6 (24 out of 178), whereas the ratio of TATA-Box element is highest in StCRLK7 (60 out of 320). Furthermore, all the family members contain the following important cis-acting elements in the upstream regions, i.e. 16 ABRE involved in the abscisic acid response, 16G-box involved in light response, 45 MYB binding site involved in light response and drought-inducibility, 20 box 4 involved in light response, and 20 MYC. The above results indicate the potential regulatory involvement of these genes in biological processes including various abiotic stress responses (Fig. 4).
(a) Graphical representation of cis-acting regulatory elements. Heat map for digital expression in response to (b) heat stress, (c) salt stress, and (d) drought stress. qRT-PCR based relative expression levels of StCRLKs in response to (e) heat, (f) salt, and (g) drought stress. Error bar indicates standard deviation (s.d.); *P < 0.05.
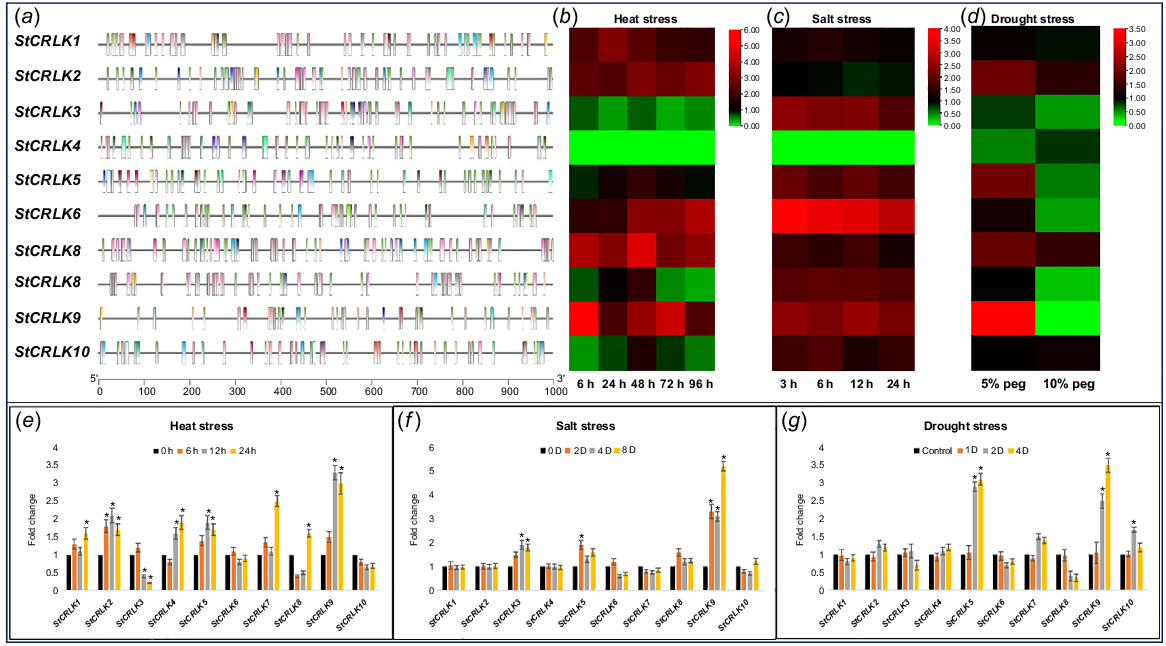
Expression profiling
A set of various cis-acting elements and their involvement in regulation within this gene family turned our focus to investigating the gene expression patterns, to gain insights into their functional roles. For this purpose, we used already published transcriptome sequences for three particular conditions, (1) heat stress, (2) salt stress and (3) drought stress. The transcriptome data was processed using bioinformatics techniques. We have derived FPKM (fragments per kilobase of transcript per million mapped reads) values, which serve as reliable measures of gene expression levels. Our analysis revealed the following results.
In the case of heat stress, two CRLK family members, StCRLK9 and StCRLK7, were found to be differentially upregulated at all the time intervals analysed. Meanwhile, StCRLK1, StCRLK2 and StCRLK6 were also found to be upregulated in all the analysed time intervals. A family member StCRLK5 was found to be slightly downregulated at a time frame of 6 h, but in later stages, it was slightly upregulated with no significant change in expression. On the other hand, StCRLK3 was found to be differentially downregulated at all the analysed time intervals. StCRLK10 and StCRLK8 were found to be differentially downregulated at initial and later time intervals, whereas at 48 h both genes were found to be upregulated (Fig. 4). In the case of salt stress, three CRLK family members, StCRLK6, StCRLK3 and StCRLK9, were found to be differentially expressed throughout the analysed time intervals. Other family members StCRLK5, StCRLK8, StCRLK7 and StCRLK10 were also found to be upregulated throughout the analysed time intervals. Meanwhile, no significant change was found in the expression of StCRLK1 and StCRLK2. StCRLK4 was shown to have zero expression (Fig. 4). In the case of drought stress, a CRLK family member StCRLK9 was found to be highly upregulated in response to moderate drought stress but significantly downregulated in response to severe drought stress. A similar pattern was found in family member StCRLK5. Moreover, StCRLK2 and StCRLK7 were found to upregulated in response to both moderate and severe drought but the degree of upregulation is notably higher when drought is moderate than when it is severe, our results highlighting a distinct expression pattern between these two conditions. StCRLK3, StCRLK6 and StCRLK8 were also found to differentially downregulate in response to severe drought stress (Fig. 4). The expression level of all the CRLK family members in potato was validated through qRT-PCR for the accuracy of transcriptome sequence data. The overall expression pattern through qRT-PCR revealed a similar expression trend as compared to transcriptome data (Fig. 4). Understanding the interplay between these regulatory elements and the dynamic expression profiles of the genes reveals their potential involvement in various biological processes.
Biochemical studies in potato under different abiotic stresses
In the current study, we performed a series of biochemical analyses to measure the antioxidant activities of peroxidase (POD), superoxide dismutase (SOD), catalase (CAT) and the levels of total soluble protein in potato. Our objective was to compare the level of these biochemicals under various abiotic stresses, i.e. heat, salt and drought. The results revealed that in the case of POD measurement, salt stress causes a significant increase, although drought and heat stress also cause an increase in the total POD content as compared to control. In the case of SOD estimation, the result revealed that drought stress causes a slight increase and heat stress a slight decrease, whereas salt stress causes a significant decrease in total activity as compared to control. In the case of CAT, our results revealed that the total measurement of CAT was significantly increased in response to salt and drought stress; in response to heat stress, CAT content were slightly increased as compared to control. The total soluble protein content was found to slightly decrease in response to heat stress and increase in response to drought stress, but no significant changes were found in response to salt stress (Fig. 5). The overall estimation of these enzyme activities under various abiotic stresses could be the basis for a more in-depth understanding of molecular mechanisms, required to develop climate-resilient crops.
Change in various antioxidant levels under different abiotic stress responses. (a) POD activity, (b) SOD activity, (c) CAT activity, and (d) total soluble proteins. The asterisk sign indicates the significant changes as compared to control, salt, heat and drought stress (*P < 0.05, **P < 0.01).
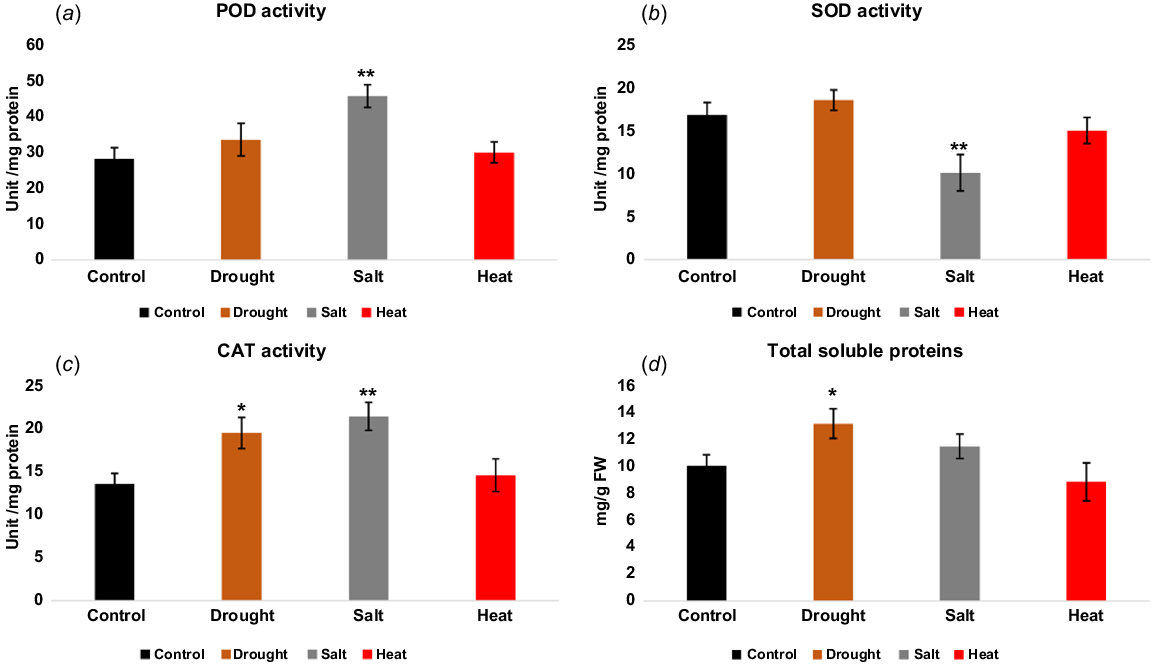
3D structure prediction and molecular docking analysis with auxin-based ligand
The 3D protein structures were predicted using AlphaFold2, and subsequent validation revealed that all protein structures attained a quality factor of over 90% and these structures are frequently closer to their original structure. Upon visualisation in UCSF Chimera, it was observed that these structures exhibited similar configurations of alpha helices, beta sheets, and loops across all proteins (Fig. 6). Indole-3-acetic acid was docked with the protein structure of CRLK family members to check their binding interaction with each other. Molecular docking analysis was performed through CB-DOCK and the visualisation and binding interaction of structures were displayed through Chimera. The docking results revealed that indole-3-acetic acid has shown very good binding capability with StCRLK3, StCRLK4, StCRLK6, StCRLK7 and StCRLK9. Family member StCRLK4 had the least vina score as compared to other family members, i.e. −7.0. The docking complexes overall exhibited an impressive range of vina scores, varying from −6.5 to −7.0 with cavity volume from 500 to 3229 Å3. Family member StCRLK9 was found to have the least root mean square deviation (RMSD) value as compared to other family members, i.e. 0.54. Meanwhile, the overall RMSD value varied from 0.54 to 1.67 for StCRLK10 (Table 2).
Phylogenetic tree and 3D structures of putative StCRLKs in potato, docking analysis and visualisation of predicted ligand-binding sites.
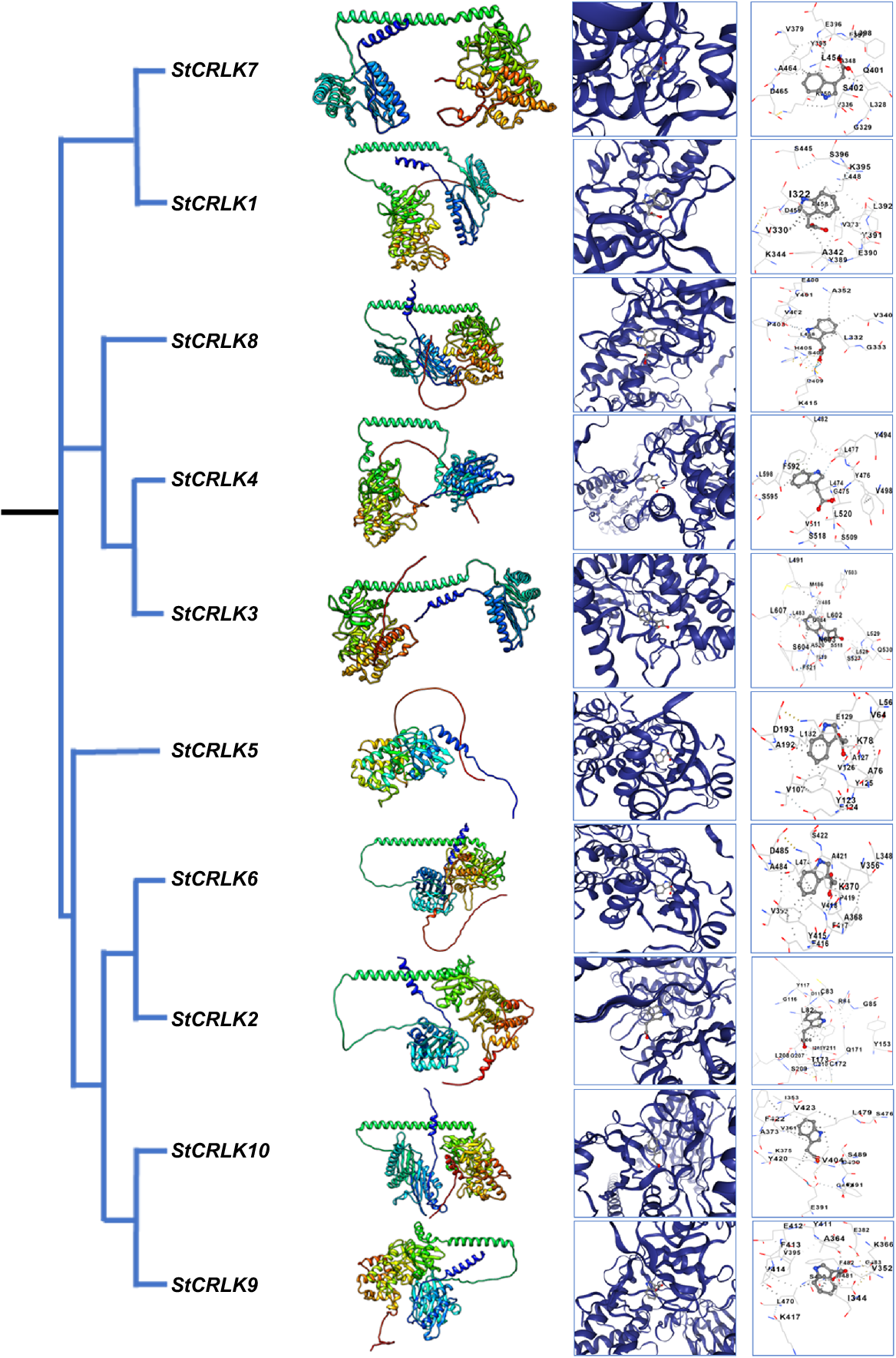
Sr no. | Protein name | Vina score | Cavity volume (Å3) | Contact residues | RMSD | |
---|---|---|---|---|---|---|
1 | StCRLK1 | −6.7 | 652 | ILE322 VAL330 ALA342 VAL373 TYR389 GLU390 TYR391 LEU392 LYS395 SER396 SER445 LEU448 ALA458 ASP459 | 1.58 | |
2 | StCRLK2 | −6.8 | 2234 | LEU82 CYS83 ARG84 GLY85 GLY116 TYR117 ASP118 GLN171 THR173 ILE205 ILE206 GLY207 LEU208 SER209 CYS210 TYR211 | 1.31 | |
3 | StCRLK3 | −6.9 | 894 | LEU483 GLY484 MET486 LEU491 TYR503 SER518 ILE519 ALA520 PHE521 SER527 LEU528 LEU529 LEU602 ASN603 SER604 LEU607 | 0.78 | |
4 | StCRLK4 | −7.0 | 3229 | LEU474 GLY475 TYR476 LEU477 ALA478 LEU482 TYR494 VAL498 SER509 VAL511 SER518 LEU520 PHE592 LEU598 | 0.9 | |
5 | StCRLK5 | −6.6 | 839 | LEU56 VAL64 ALA76 VAL107 TYR123 GLU124 TYR125 VAL126 GLU129 LEU182 ALA192 ASP193 | 1.27 | |
6 | StCRLK6 | −6.5 | 500 | LEU348 VAL356 ALA368 VAL399 TYR415 GLU416 PHE417 VAL418 PRO419 ALA421 SER422 LEU474 ALA484 ASP485 | 0.71 | |
7 | StCRLK7 | −6.9 | 1019 | LEU328 VAL336 ALA348 LYS350 VAL379 TYR395 GLU396 PHE397 LEU398 PRO399 GLN401 SER402 LEU454 ALA464 ASP465 | 0.83 | |
8 | StCRLK8 | −6.8 | 1480 | LEU332 GLY333 VAL340 ALA352 GLU400 TYR401 VAL402 PRO403 HIS405 SER406 ASP409 LYS415 LEU458 | 1.06 | |
9 | StCRLK9 | −6.7 | 622 | ILE344 VAL352 ALA364 LYS366 GLU382 VAL395 TYR411 GLU412 VAL414 LEU470 SER480 ASP481 PHE482 | 0.54 | |
10 | StCRLK10 | −6.5 | 534 | PHE166 LYS167 GLN168 LEU170 GLY171 MET174 ILE228 LEU231 ASN232 CYS235 ASP236 GLY237 TYR238 ALA241 HIS252 | 1.61 |
Discussion
CRLKs play a key role in plant responses to environmental changes, are involved in diverse signalling pathways, and have potential to regulate phytohormones and mitogen-activated protein kinases (MAPKs) (Pelagio-Flores et al. 2020). These CRLKs were also involved in regulating flowering, fruit development, and biotic stress responses (Acharya et al. 2007; Hunter et al. 2019; Theine et al. 2021). The biochemical function of the CRLK’s signature domain is unknown but the structure consists of antifungal protein ginkbilolobin-2 (Gnk2) from Ginkgo biloba (Miyakawa et al. 2009). Several studies suggest the involvement of CRKs in ROS (reactive oxygen species) signalling, indicating the potential for redox regulation of their extracellular thiol groups, and have observed elevated transcript levels in response to oxidative stress, pathogens and salicylic acid (SA) (Czernic et al. 1999; Wrzaczek et al. 2010; Shapiguzov et al. 2012; Vainonen and Kangasjärvi 2015). Although genetic redundancy complicates the characterisation of CRK mutants, overexpression studies have suggested their roles in disease resistance and cell death (Chen et al. 2003; Acharya et al. 2007). A comprehensive analysis of a transfer DNA (T-DNA) insertion collection for CRKs reveals their participation in various processes, i.e. both biotic and abiotic stress responses, as well as developmental pathways (Idänheimo et al. 2014).
Recent research underscores their participation in plant development control, yet the precise molecular mechanisms that underlie this role remain unclear, especially in potato. Genome assemblies and bioinformatics analyses of diverse plant gene families, including CRLKs, which have been extensively characterised in crops such as Arabidopsis thaliana, Capsicum annuum, Gossypium barbadense, Malus domestica, Prunus avium, Brassica napus and Cucumis sativus, served as models for characterising CRLKs in the potato (Li et al. 2018; Zuo et al. 2020; Sarwar et al. 2023; Nanda et al. 2023; Rout et al. 2023). The comprehensive genome-wide identification of 10 CRLKs in potato, each featuring two Gnk2-homologous domains at the N-terminus and a protein kinase domain at the C-terminus, is consistent with the structural characteristics reported for CRLK family members in other plant species (Zeiner et al. 2023). Notably, the unique domain arrangement observed in StCRLK2, with one Gnk2-homologous domain and one protein kinase domain, deviates from the canonical pattern seen in other family members, suggesting potential functional divergence that aligns with the adaptability of these kinases to diverse stress conditions in potatoes (Liu et al. 2024).
The theoretical isoelectric point in our results revealed that most StCRLKs have a slightly acidic pH, with the remaining having an alkaline natural pH (Table 1). Since plants have a rapid pH response system that can regulate the gene expression pattern (Lager et al. 2010). The variation in total number of CRLKs in potato as compared to Arabidopsis (44), rice (39) and cucumber (15), and inter-family conserved intron–exon organisation, suggests its relation with their function and conservation (Liu et al. 2013). Chromosomal mapping reveals the uneven distribution on potato chromosomes; with a maximum number of genes on Chromosome number 5. In contrast, in Arabidopsis the maximum genes were localised on Chromosome number 4 (Wrzaczek et al. 2010). Phylogenetic analysis revealed a conserved evolutionary relationship with CRLK family members from other plants, i.e. Arabidopsis, rice and cucumber. These findings serve as a basis and suggest more in-depth studies on the biological and functional involvement of CRLKs in potato.
In plants, the production of ROS is regulated by several abiotic stresses that cause oxidative damage to lipids, proteins, and nucleic acid (Rahnama and Ebrahimzadeh 2005). A set of enzymes, SOD, POD and CAT, are potentially involved in avoiding the oxidative damage of plants that is caused by ROS (Zameer et al. 2022). It was proposed in 2010, that the increase in these enzymatic activities might contribute to the tuber yield of potato (Wang et al. 2010). In Arabidopsis, the overexpression of AtRCD1 gene was involved in maintaining the SOD and POD enzyme activity in response to salt stress (Tao et al. 2023). In Medicago truncatula, a drought sensitive gene MtCBL13 causes a significant decrease in SOD and POD activity by overexpressing (Wassie et al. 2023). In Arabidopsis, the overexpression of TaGPX1-D causes a significant increase in SOD, CAT and carotenoids in response to salt stress (Tyagi et al. 2023). In current research, our findings revealed that abiotic stresses, i.e. salt, heat and drought, significantly affect the physiology of potato seedlings, as evident from increased POD and CAT activity in salt and drought stress.
External stimuli are crucial in regulating gene expression during development and in response to stress. Gene transcription is controlled by cis-acting elements located upstream of the transcription start site, which respond to external cues, leading to either gene activation or repression (Shafique et al. 2023). In the current study, the highest number of cis-elements, i.e. TATA-Box and CAAT-Box, were abundantly present in the promoter region of all the identified CRLKs in potato. The stress-related genes are enriched with TATA-box, and its binding with RNA polymerase II is involved in the regulation of the initiation site for transcription (Bae et al. 2015; Ijaz et al. 2023). ABRE-elements, considered as hormone-responsive cis-elements, have been identified in the promoter regions of StCRLK genes, indicating the potential regulation of gene expression by hormones (Gómez-Porras et al. 2007). G-box consists of ACGT-motif and ranks high across ABA, drought, cold and salinity-inducible genes (Zhang et al. 2005). Two stress-related transcription factors MYB and MYC play a pivotal role in stress tolerance, with their binding sites occurring 45 and 20 times, respectively, in StCRLKs (Feng et al. 2013; Li et al. 2019). Various numbers of cis-elements, especially stress-related cis-elements, present in the upstream region of CRLKs in potato indicate the functional diversity and biological significance that contribute to the plant’s ability to cope with different environmental stresses.
Previously, various phytohormones such as jasmonic acid (JA), ethylene and SA have been identified as being involved in the regulation of both biotic and abiotic stresses (Pieterse et al. 2009). Recent insights underscore the importance of auxin in various stages of tuber development, including initiation, growth and sprouting, as well as for stress resistance; suggesting a potential for auxin-mediated potato improvement (Kolachevskaya et al. 2017, 2019). The conserved 3D structures support the phylogenetic and conserved domain analysis (Fig. 6). The interaction of auxin-related compounds with CRLK protein suggests the auxin-mediated involvement of CRLKs in potato.
Understanding and manipulating gene expression levels in plants have become key strategies in biotechnology and breeding programs aimed at developing stress-tolerant crop varieties to reduce the risk of yield losses (Pérez-de-Castro et al. 2012). Various studies in A. thaliana indicate the involvement of AtCRK5 family members in regulation of ABA signalling, ROS and SA, suggesting the regulation of growth, development and stress responses (Burdiak et al. 2015; Lu et al. 2016). AtCRK28 is involved in modulating plant growth by stimulating abscisic acid responses (Pelagio-Flores et al. 2020). Two transcription factors, StHsfA1b and StHsfA2, are positively regulated in response to heat stress in potato (Tang et al. 2016). The overexpressing of StERF94 improves tolerance to various abiotic stresses, e.g. heat and drought (Charfeddine et al. 2023). Various studies reveal the importance of CRKs in response to biotic stress including, CRKs in cotton and chilli that respond to fungal infections, TaCRK2 which enhances wheat resistance to leaf rust, and AtCRK45 which boosts defence against Pseudomonas syringae (Zhang et al. 2013; Li et al. 2018; Gu et al. 2020; Rout et al. 2023). The literature suggests that CRKs play a role in defence responses against both abiotic and biotic stresses. The expression dynamics of CRKs exhibit diverse and time-specific trends in response to specific stressors. In the current study, heat stress showed a mixed gene expression response, salt stress predominantly upregulated genes, and drought stress induced a shift from upregulation at moderate levels to downregulation at severe levels. StCRLK9 is a common gene influenced by these stresses, suggesting its potential involvement in the plant’s response to various stress conditions.
Conclusion
In summary, the present study shows the first systematic and comprehensive analysis of StCRKs in potato. A total of 10 genes were identified through genome-wide analysis in the potato genome, and divided into three classes based on comparative phylogenetic studies. All StCRLK proteins have specific Gnk2-homologous domains present at the N-terminus and protein kinase domain at the C-terminus. Gene duplication events in the potato were caused by tandem duplication. Significantly, StCRLKs genes showed diversity in protein properties such as motifs, length, and size of gene structure. The current study provides a more in-depth understanding of phylogenetic relationships of StCRLKs, their distribution on chromosomes, conserved exon–intron structure, and domain structure as well as evolutionary history. StCRLK9 is differentially expressed in response to abiotic stress. Collectively this study will provide comprehensive information about the CRLKs in potato and will provide a theoretical basis for future targets in further characterisation of StCRLKs against abiotic stress response.
Data availability
All the sequence data used in the study were downloaded from the nucleotide repository of the National Center for Biotechnology Information (NCBI; www.ncbi.nlm.nih.gov) and Ensemble plants database (http://plants.ensembl.org/index.html). RNA sequencing data (BioProject: PRJNA556372 for heat stress, BioProject: PRJNA882516 for salt stress, and BioProject: PRJNA879907 for drought stress) was downloaded from NCBI Sequence Read Archive (SRA) database (https://www.ncbi.nlm.nih.gov/sra). The genome and gtf files were downloaded directly from NCBI Genome assembly database using RefSeq: GCF_000226075.1 (https://www.ncbi.nlm.nih.gov/assembly/SolTub_3.0/).
Declaration of funding
Princess Nourah bint Abdulrahman University Researchers Supporting Project number (PNURSP2024R402), Princess Nourah bint Abdulrahman University, Riyadh, Saudi Arabia.
Author contributions
RZ, CY and ZL conceived and designed the research project. RZ, MSM, KMA and CL performed the experiments. RZ, KMA, DA and CY prepared a draft manuscript. MSM, DA and ZL revised and finalised the manuscript. All authors discussed the results, provided critical feedback and agreed to the final manuscript.
Acknowledgements
The authors acknowledge Princess Nourah bint Abdulrahman University Researchers Supporting Project number (PNURSP2024R402), Princess Nourah bint Abdulrahman University, Riyadh, Saudi Arabia.
References
Acharya BR, Raina S, Maqbool SB, Jagadeeswaran G, Mosher SL, Appel HM, Schultz JC, Klessig DF, Raina R (2007) Overexpression of CRK13, an Arabidopsis cysteine-rich receptor-like kinase, results in enhanced resistance to Pseudomonas syringae. The Plant Journal 50, 488-499.
| Crossref | Google Scholar | PubMed |
Azeem F, Ijaz U, Ali MA, Hussain S, Zubair M, Manzoor H, Abid M, Zameer R, Kim D-S, Golokhvast KS, Chung G, Sun S, Nawaz MA (2021) Genome-wide identification and expression profiling of potassium transport-related genes in Vigna radiata under abiotic stresses. Plants 11(1), 2.
| Crossref | Google Scholar |
Bae S-H, Han HW, Moon J (2015) Functional analysis of the molecular interactions of TATA box-containing genes and essential genes. PLoS ONE 10, e0120848.
| Crossref | Google Scholar | PubMed |
Baek D, Kim MC, Kumar D, Park B, Cheong MS, Choi W, Park HC, Chun HJ, Park HJ, Lee SY, Bressan RA, Kim J-Y, Yun D-J (2019) AtPR5K2, a PR5-Like receptor kinase, modulates plant responses to drought stress by phosphorylating protein phosphatase 2Cs. Frontiers in Plant Science 10, 1146.
| Crossref | Google Scholar |
Bradford MM (1976) A rapid and sensitive method for the quantitation of microgram quantities of protein utilizing the principle of protein-dye binding. Analytical Biochemistry 72, 248-254.
| Crossref | Google Scholar | PubMed |
Burdiak P, Rusaczonek A, Witoń D, Głów D, Karpiński S (2015) Cysteine-rich receptor-like kinase CRK5 as a regulator of growth, development, and ultraviolet radiation responses in Arabidopsis thaliana. Journal of Experimental Botany 66, 3325-3337.
| Crossref | Google Scholar | PubMed |
Campos Mantello C, Boatwright L, da Silva CC, Scaloppi EJ, de Souza Goncalves P, Barbazuk WB, Pereira de Souza A (2019) Deep expression analysis reveals distinct cold-response strategies in rubber tree (Hevea brasiliensis). BMC Genomics 20, 455.
| Crossref | Google Scholar |
Caumon H, Vernoux T (2023) A matter of time: auxin signaling dynamics and the regulation of auxin responses during plant development. Journal of Experimental Botany 74, 3887-3902.
| Crossref | Google Scholar |
Chance B, Maehly AC (1955) Assay of catalases and peroxidases. Methods in Enzymology 2, 764-775.
| Crossref | Google Scholar |
Charfeddine M, Chiab N, Charfeddine S, Ferjani A, Gargouri-Bouzid R (2023) Heat, drought, and combined stress effect on transgenic potato plants overexpressing the StERF94 transcription factor. Journal of Plant Research 136, 549-562.
| Crossref | Google Scholar | PubMed |
Chen Z (2001) A superfamily of proteins with novel cysteine-rich repeats. Plant Physiology 126, 473-476.
| Crossref | Google Scholar | PubMed |
Chen K, Du L, Chen Z (2003) Sensitization of defense responses and activation of programmed cell death by a pathogen-induced receptor-like protein kinase in Arabidopsis. Plant Molecular Biology 53, 61-74.
| Crossref | Google Scholar | PubMed |
Chen C, Chen H, Zhang Y, Thomas HR, Frank MH, He Y, Xia R (2020) TBtools: an integrative toolkit developed for interactive analyses of big biological data. Molecular Plant 13, 1194-1202.
| Crossref | Google Scholar |
Chern M, Xu Q, Bart RS, Bai W, Ruan D, Sze-To WH, Canlas PE, Jain R, Chen X, Ronald PC (2016) A genetic screen identifies a requirement for cysteine-rich–receptor-like kinases in rice NH1 (OsNPR1)-mediated immunity. PLoS Genetics 12, e1006049.
| Crossref | Google Scholar | PubMed |
Cui Y, Lu X, Gou X (2022) Receptor-like protein kinases in plant reproduction: current understanding and future perspectives. Plant Communications 3, 100273.
| Crossref | Google Scholar |
Czernic P, Visser B, Sun W, Savouré A, Deslandes L, Marco Y, Van Montagu M, Verbruggen N (1999) Characterization of an Arabidopsis thaliana receptor-like protein kinase gene activated by oxidative stress and pathogen attack. The Plant Journal 18, 321-327.
| Crossref | Google Scholar | PubMed |
Deblonde PMK, Ledent J-F (2001) Effects of moderate drought conditions on green leaf number, stem height, leaf length and tuber yield of potato cultivars. European Journal of Agronomy 14, 31-41.
| Crossref | Google Scholar |
Feng H-L, Ma N-N, Meng X, Zhang S, Wang J-R, Chai S, Meng Q-W (2013) A novel tomato MYC-type ICE1-like transcription factor, SlICE1a, confers cold, osmotic and salt tolerance in transgenic tobacco. Plant Physiology and Biochemistry 73, 309-320.
| Crossref | Google Scholar | PubMed |
Gu J, Sun J, Liu N, Sun X, Liu C, Wu L, Liu G, Zeng F, Hou C, Han S, Zhen W, Wang D (2020) A novel cysteine-rich receptor-like kinase gene, TaCRK2, contributes to leaf rust resistance in wheat. Molecular Plant Pathology 21, 732-746.
| Crossref | Google Scholar | PubMed |
Gómez-Porras JL, Riaño-Pachón DM, Dreyer I, Mayer JE, Mueller-Roeber B (2007) Genome-wide analysis of ABA-responsive elements ABRE and CE3 reveals divergent patterns in Arabidopsis and rice. BMC Genomics 8, 260.
| Crossref | Google Scholar |
Han X, Yang R, Zhang L, Wei Q, Zhang Y, Wang Y, Shi Y (2023) A review of potato salt tolerance. International Journal of Molecular Sciences 24, 10726.
| Crossref | Google Scholar |
Hill D, Nelson D, Hammond J, Bell L (2021) Morphophysiology of potato (Solanum tuberosum) in response to drought stress: paving the way forward. Frontiers in Plant Science 11, 597554.
| Crossref | Google Scholar |
Huang Y, Gao B, Huang W, Wang L, Fang X, Xu S, Cui S (2021) Producing more potatoes with lower inputs and greenhouse gases emissions by regionalized cooperation in China. Journal of Cleaner Production 299, 126883.
| Crossref | Google Scholar |
Hunter K, Kimura S, Rokka A, Tran HC, Toyota M, Kukkonen JP, Wrzaczek M (2019) CRK2 enhances salt tolerance by regulating callose deposition in connection with PLD α 1. Plant Physiology 180, 2004-2021.
| Crossref | Google Scholar | PubMed |
Idänheimo N, Gauthier A, Salojärvi J, Siligato R, Brosché M, Kollist H, Mähönen AP, Kangasjärvi J, Wrzaczek M (2014) The Arabidopsis thaliana cysteine-rich receptor-like kinases CRK6 and CRK7 protect against apoplastic oxidative stress. Biochemical and Biophysical Research Communications 445, 457-462.
| Crossref | Google Scholar | PubMed |
Ijaz U, Pervaiz T, Ahmed T, Seemab R, Shahid M, Noman M, Nadeem M, Azeem F (2023) AJAB. Asian Journal of Agriculture & Biology 4(4), 2023110.
| Google Scholar |
Jing Q, Hou H, Meng X, Chen A, Wang L, Zhu H, Zheng S, Lv Z, Zhu X (2022) Transcriptome analysis reveals the proline metabolic pathway and its potential regulation TF-hub genes in salt-stressed potato. Frontiers in Plant Science 13, 1030138.
| Crossref | Google Scholar | PubMed |
Jinjun Z, Peina J, Fang Z, Chongke Z, Bo B, Yaping L, Haifeng W, Fan C, Xianzhi X (2020) OsSRK1, an Atypical S-receptor-like kinase positively regulates leaf width and salt tolerance in rice. Rice Science 27, 133-142.
| Crossref | Google Scholar |
Jumper J, Evans R, Pritzel A, Green T, Figurnov M, Ronneberger O, Tunyasuvunakool K, Bates R, Žídek A, Potapenko A, Bridgland A, Meyer C, Kohl SAA, Ballard AJ, Cowie A, Romera-Paredes B, Nikolov S, Jain R, Adler J, Back T, Petersen S, Reiman D, Clancy E, Zielinski M, Steinegger M, Pacholska M, Berghammer T, Bodenstein S, Silver D, Vinyals O, Senior AW, Kavukcuoglu K (2021) Highly accurate protein structure prediction with AlphaFold. Nature 596, 583-589.
| Crossref | Google Scholar | PubMed |
Kolachevskaya OO, Sergeeva LI, Floková K, Getman IA, Lomin SN, Alekseeva V V, Rukavtsova EB, Buryanov YI, Romanov GA (2017) Auxin synthesis gene tms1 driven by tuber-specific promoter alters hormonal status of transgenic potato plants and their responses to exogenous phytohormones. Plant Cell Reports 36, 419-435.
| Crossref | Google Scholar | PubMed |
Kolachevskaya OO, Lomin SN, Arkhipov DV, Romanov GA (2019) Auxins in potato: molecular aspects and emerging roles in tuber formation and stress resistance. Plant Cell Reports 38, 681-698.
| Crossref | Google Scholar | PubMed |
Kono Y (1978) Generation of superoxide radical during autoxidation of hydroxylamine and an assay for superoxide dismutase. Archives of Biochemistry and Biophysics 186, 189-195.
| Crossref | Google Scholar | PubMed |
Koyama T, Mitsuda N, Seki M, Shinozaki K, Ohme-Takagi M (2010) TCP transcription factors regulate the activities of ASYMMETRIC LEAVES1 and miR164, as well as the auxin response, during differentiation of leaves in Arabidopsis. The Plant Cell 22, 3574-3588.
| Crossref | Google Scholar | PubMed |
Kumar S, Asrey R, Mandal G (2007) Effect of differential irrigation regimes on potato (Solanum tuberosum) yield and post-harvest attributes. Indian Journal of Agricultural Sciences 77(6), 336-338.
| Google Scholar |
Kumar S, Stecher G, Tamura K (2016) MEGA7: molecular evolutionary genetics analysis version 7.0 for bigger datasets. Molecular Biology and Evolution 33, 1870-1874.
| Crossref | Google Scholar | PubMed |
Lager I, Andréasson O, Dunbar TL, Andreasson E, Escobar MA, Rasmusson AG (2010) Changes in external pH rapidly alter plant gene expression and modulate auxin and elicitor responses. Plant, Cell & Environment 33, 1513-1528.
| Crossref | Google Scholar | PubMed |
Langmead B, Salzberg SL (2012) Fast gapped-read alignment with Bowtie 2. Nature Methods 9, 357-359.
| Crossref | Google Scholar |
Lescot M, Déhais P, Thijs G, Marchal K, Moreau Y, Van de Peer Y, Rouzé P, Rombauts S (2002) PlantCARE, a database of plant cis-acting regulatory elements and a portal to tools for in silico analysis of promoter sequences. Nucleic Acids Research 30, 325-327.
| Crossref | Google Scholar | PubMed |
Li T-G, Zhang D-D, Zhou L, Kong Z-Q, Hussaini AS, Wang D, Li J-J, Short DPG, Dhar N, Klosterman SJ, Wang BL, Yin CM, Subbarao KV, Chen JY, Dai XF (2018) Genome-wide identification and functional analyses of the CRK gene family in cotton reveals GbCRK18 confers verticillium wilt resistance in Gossypium barbadense. Frontiers in Plant Science 9, 1266.
| Crossref | Google Scholar | PubMed |
Li J, Han G, Sun C, Sui N (2019) Research advances of MYB transcription factors in plant stress resistance and breeding. Plant Signaling & Behavior 14, 1613131.
| Crossref | Google Scholar | PubMed |
Li X, Shen F, Xu X, Zheng Q, Wang Y, Wu T, Li W, Qiu C, Xu X, Han Z, Zhang X (2021) An HD-ZIP transcription factor, MxHB13, integrates auxin-regulated and juvenility-determined control of adventitious rooting in Malus xiaojinensis. The Plant Journal 107, 1663-1680.
| Crossref | Google Scholar | PubMed |
Liu Y, Zhang C, Chen J, Guo L, Li X, Li W, Yu Z, Deng J, Zhang P, Zhang K, Zhang L (2013) Arabidopsis heat shock factor HsfA1a directly senses heat stress, pH changes, and hydrogen peroxide via the engagement of redox state. Plant Physiology and Biochemistry 64, 92-98.
| Crossref | Google Scholar | PubMed |
Liu Y, Feng Z, Zhu W, Liu J, Zhang Y (2021) Genome-wide identification and characterization of cysteine-rich receptor-like protein kinase genes in tomato and their expression profile in response to heat stress. Diversity 13, 258.
| Crossref | Google Scholar |
Liu J, Li W, Wu G, Ali K (2024) An update on evolutionary, structural, and functional studies of receptor-like kinases in plants. Frontiers in Plant Science 15, 1305599.
| Crossref | Google Scholar |
Lu K, Liang S, Wu Z, Bi C, Yu Y-T, Wang X-F, Zhang D-P (2016) Overexpression of an Arabidopsis cysteine-rich receptor-like protein kinase, CRK5, enhances abscisic acid sensitivity and confers drought tolerance. Journal of Experimental Botany 67, 5009-5027.
| Crossref | Google Scholar | PubMed |
Mittler R (2006) Abiotic stress, the field environment and stress combination. Trends in Plant Science 11, 15-19.
| Crossref | Google Scholar | PubMed |
Miyakawa T, Miyazono K, Sawano Y, Hatano K, Tanokura M (2009) Crystal structure of ginkbilobin-2 with homology to the extracellular domain of plant cysteine-rich receptor-like kinases. Proteins: Structure, Function, and Bioinformatics 77, 247-251.
| Crossref | Google Scholar |
Nanda S, Rout P, Ullah I, Nag SR, Reddy VV, Kumar G, Kumar R, He S, Wu H (2023) Genome-wide identification and molecular characterization of CRK gene family in cucumber (Cucumis sativus L.) under cold stress and sclerotium rolfsii infection. BMC Genomics 24, 219.
| Crossref | Google Scholar | PubMed |
Nasir MW, Toth Z (2022) Effect of drought stress on potato production: a review. Agronomy 12, 635.
| Crossref | Google Scholar |
Ou Y, Kui H, Li J (2021) Receptor-like kinases in root development: current progress and future directions. Molecular Plant 14, 166-185.
| Crossref | Google Scholar | PubMed |
Pelagio-Flores R, Muñoz-Parra E, Barrera-Ortiz S, Ortiz-Castro R, Saenz-Mata J, Ortega-Amaro MA, Jiménez-Bremont JF, López-Bucio J (2020) The cysteine-rich receptor-like protein kinase CRK28 modulates Arabidopsis growth and development and influences abscisic acid responses. Planta 251, 1-12.
| Crossref | Google Scholar |
Pettersen EF, Goddard TD, Huang CC, Couch GS, Greenblatt DM, Meng EC, Ferrin TE (2004) UCSF Chimera—a visualization system for exploratory research and analysis. Journal of Computational Chemistry 25, 1605-1612.
| Crossref | Google Scholar | PubMed |
Pieterse CMJ, Leon-Reyes A, Van der Ent S, Van Wees SCM (2009) Networking by small-molecule hormones in plant immunity. Nature Chemical Biology 5, 308-316.
| Crossref | Google Scholar | PubMed |
Pérez-de-Castro AM, Vilanova S, Cañizares J, Pascual L, Blanca JM, Diez MJ, Prohens J, Picó B (2012) Application of genomic tools in plant breeding. Current Genomics 13, 179-195.
| Crossref | Google Scholar |
Rahnama H, Ebrahimzadeh H (2005) The effect of NaCl on antioxidant enzyme activities in potato seedlings. Biologia Plantarum 49, 93-97.
| Crossref | Google Scholar |
Rout SS, Rout P, Uzair M, Kumar G, Nanda S (2023) Genome-wide identification and expression analysis of CRK gene family in chili pepper (Capsicum annuum L.) in response to Colletotrichum truncatum infection. The Journal of Horticultural Science and Biotechnology 98, 194-206.
| Crossref | Google Scholar |
Rozas J, Ferrer-mata A, Sánchez-DelBarrio JC, Guirao-Rico S, Librado P, Ramos-Onsins SE, Sánchez-Gracia A (2017) DnaSP 6: DNA sequence polymorphism analysis of large data sets. Molecular Biology and Evolution 34, 3299-3302.
| Crossref | Google Scholar |
Sakamoto T, Morinaka Y, Inukai Y, Kitano H, Fujioka S (2013) Auxin signal transcription factor regulates expression of the brassinosteroid receptor gene in rice. The Plant Journal 73, 676-688.
| Crossref | Google Scholar | PubMed |
Sarwar R, Li L, Yu J, Zhang Y, Geng R, Meng Q, Zhu K, Tan X-L (2023) Functional characterization of the cystine-rich-receptor-like kinases (CRKs) and their expression response to Sclerotinia sclerotiorum and abiotic stresses in Brassica napus. International Journal of Molecular Sciences 24, 511.
| Crossref | Google Scholar | PubMed |
Shabala S, Wu H, Bose J (2015) Salt stress sensing and early signalling events in plant roots: current knowledge and hypothesis. Plant Science 241, 109-119.
| Crossref | Google Scholar | PubMed |
Shafique A, Batool R, Rizwan M, Zameer R, Arshad H, Xu H, Alwutayd K, AbdElgawad H, Azeem F (2023) Integrative omics analysis of Rosa chinensis reveals insights into its transcriptome and in silico characterization of potassium transport genes. Plant Stress 10, 100202.
| Crossref | Google Scholar |
Shani E, Salehin M, Zhang Y, Sanchez SE, Doherty C, Wang R, Mangado CC, Song L, Tal I, Pisanty O, Ecker JR, Kay SA, Pruneda-Paz J, Estelle M (2017) Plant stress tolerance requires auxin-sensitive Aux/IAA transcriptional repressors. Current Biology 27, 437-444.
| Crossref | Google Scholar | PubMed |
Shannon P, Markiel A, Ozier O, Baliga NS, Wang JT, Ramage D, Amin N, Schwikowski B, Ideker T (2003) Cytoscape: a software environment for integrated models of biomolecular interaction networks. Genome Research 13, 2498-2504.
| Crossref | Google Scholar | PubMed |
Shapiguzov A, Vainonen JP, Wrzaczek M, Kangasjärvi J (2012) ROS-talk–how the apoplast, the chloroplast, and the nucleus get the message through. Frontiers in Plant Science 3, 292.
| Crossref | Google Scholar |
Shin R, Burch AY, Huppert KA, Tiwari SB, Murphy AS, Guilfoyle TJ, Schachtman DP (2007) The Arabidopsis transcription factor MYB77 modulates auxin signal transduction. The Plant Cell 19, 2440-2453.
| Crossref | Google Scholar | PubMed |
Shiu S-H, Bleecker AB (2003) Expansion of the receptor-like kinase/Pelle gene family and receptor-like proteins in Arabidopsis. Plant Physiology 132, 530-543.
| Crossref | Google Scholar | PubMed |
Shiu S-H, Karlowski WM, Pan R, Tzeng Y-H, Mayer KFX, Li W-H (2004) Comparative analysis of the receptor-like kinase family in Arabidopsis and rice. The Plant Cell 16, 1220-1234.
| Crossref | Google Scholar | PubMed |
Shu W, Zhou H, Jiang C, Zhao S, Wang L, Li Q, Yang Z, Groover A, Lu M-Z (2019) The auxin receptor TIR 1 homolog (Pag FBL 1) regulates adventitious rooting through interactions with Aux/IAA 28 in Populus. Plant Biotechnology Journal 17, 338-349.
| Crossref | Google Scholar | PubMed |
Shumayla , Tyagi S, Sharma A, Singh K, Upadhyay SK (2019) Genomic dissection and transcriptional profiling of cysteine-rich receptor-like kinases in five cereals and functional characterization of TaCRK68-A. International Journal of Biological Macromolecules 134, 316-329.
| Crossref | Google Scholar |
Tanaka H, Osakabe Y, Katsura S, Mizuno S, Maruyama K, Kusakabe K, Mizoi J, Shinozaki K, Yamaguchi-Shinozaki K (2012) Abiotic stress-inducible receptor-like kinases negatively control ABA signaling in Arabidopsis. The Plant Journal 70, 599-613.
| Crossref | Google Scholar | PubMed |
Tang R, Zhu W, Song X, Lin X, Cai J, Wang M, Yang Q (2016) Genome-wide identification and function analyses of heat shock transcription factors in potato. Frontiers in Plant Science 7, 490.
| Google Scholar | PubMed |
Tang R, Niu S, Zhang G, Chen G, Haroon M, Yang Q, Rajora OP, Li X-Q (2018) Physiological and growth responses of potato cultivars to heat stress. Botany 96, 897-912.
| Crossref | Google Scholar |
Tao J, Wu F, Wen H, Liu X, Luo W, Gao L, Jiang Z, Mo B, Chen X, Kong W, Yu Y (2023) RCD1 promotes salt stress tolerance in Arabidopsis by repressing ANAC017 activity. International Journal of Molecular Sciences 24, 9793.
| Crossref | Google Scholar | PubMed |
Theine J, Holtgräwe D, Herzog K, Schwander F, Kicherer A, Hausmann L, Viehöver P, Töpfer R, Weisshaar B (2021) Transcriptomic analysis of temporal shifts in berry development between two grapevine cultivars of the Pinot family reveals potential genes controlling ripening time. BMC Plant Biology 21, 327.
| Crossref | Google Scholar |
Trapnell C, Roberts A, Goff L, Pertea G, Kim D, Kelley DR, Pimentel H, Salzberg SL, Rinn JL, Pachter L (2012) Differential gene and transcript expression analysis of RNA-seq experiments with TopHat and Cufflinks. Nature Protocols 7, 562-578.
| Crossref | Google Scholar |
Tyagi S, Shumayla , Sharma Y, Madhu , Sharma A, Pandey A, Singh K, Upadhyay SK (2023) TaGPX1-D overexpression provides salinity and osmotic stress tolerance in Arabidopsis. Plant Science 337, 111881.
| Crossref | Google Scholar |
Umezawa T, Nakashima K, Miyakawa T, Kuromori T, Tanokura M, Shinozaki K, Yamaguchi-Shinozaki K (2010) Molecular basis of the core regulatory network in ABA responses: sensing, signaling and transport. Plant & Cell Physiology 51, 1821-1839.
| Crossref | Google Scholar |
Vainonen JP, Kangasjärvi J (2015) Plant signalling in acute ozone exposure. Plant, Cell & Environment 38, 240-252.
| Crossref | Google Scholar | PubMed |
Voorrips RE (2002) MapChart: software for the graphical presentation of linkage maps and QTLs. Journal of Heredity 93, 77-78.
| Crossref | Google Scholar | PubMed |
Walker JC, Zhang R (1990) Relationship of a putative receptor protein kinase from maize to the S-locus glycoproteins of Brassica. Nature 345, 743-746.
| Crossref | Google Scholar | PubMed |
Wang W, Vinocur B, Altman A (2003) Plant responses to drought, salinity and extreme temperatures: towards genetic engineering for stress tolerance. Planta 218, 1-14.
| Crossref | Google Scholar | PubMed |
Wang H, Xiao L, Tong J, Liu F (2010) Foliar application of chlorocholine chloride improves leaf mineral nutrition, antioxidant enzyme activity, and tuber yield of potato (Solanum tuberosum L.). Scientia Horticulturae 125, 521-523.
| Crossref | Google Scholar |
Wang C, Zhao Y, Gu P, Zou F, Meng L, Song W, Yang Y, Wang S, Zhang Y (2018) Auxin is involved in lateral root formation induced by drought stress in tobacco seedlings. Journal of Plant Growth Regulation 37, 539-549.
| Crossref | Google Scholar |
Wang Z-J, Liu H, Zeng F-K, Yang Y-C, Xu D, Zhao Y-C, Liu X, Kaur L, Liu G, Singh J (2023) Potato processing industry in China: current scenario, future trends and global impact. Potato Research 66, 543-562.
| Crossref | Google Scholar | PubMed |
Wassie M, Song S, Cao L, Chen L (2023) A Medicago truncatula calcineurin b-like protein, MtCBL13 confers drought sensitivity in arabidopsis through ABA-dependent pathway. Environmental and Experimental Botany 206, 105141.
| Crossref | Google Scholar |
Watanabe E, Mano S, Hara-Nishimura I, Nishimura M, Yamada K (2017) HSP90 stabilizes auxin receptor TIR1 and ensures plasticity of auxin responses. Plant Signaling & Behavior 12, e1311439.
| Crossref | Google Scholar | PubMed |
Went FW (1928) Wuchsstoff und wachstum. Recueil Des Travaux Botaniques Néerlandais 25, 1-116.
| Google Scholar |
Wrzaczek M, Brosché M, Salojärvi J, Kangasjärvi S, Idänheimo N, Mersmann S, Robatzek S, Karpiński S, Karpińska B, Kangasjärvi J (2010) Transcriptional regulation of the CRK/DUF26 group of receptor-like protein kinases by ozone and plant hormones in Arabidopsis. BMC Plant Biology 10, 95.
| Crossref | Google Scholar |
Xi Y, Han X, Zhang Z, Joshi J, Borza T, Aqa MM, Zhang B, Yuan H, Wang-Pruski G (2020) Exogenous phosphite application alleviates the adverse effects of heat stress and improves thermotolerance of potato (Solanum tuberosum L.) seedlings. Ecotoxicology and Environmental Safety 190, 110048.
| Crossref | Google Scholar | PubMed |
Xu X, Pan S, Cheng S, Zhang B, Mu D, Ni P, Zhang G, Yang S, Li R, Wang J, Orjeda G, Guzman F, Torres M, Lozano R, Ponce O, Martinez D, De La Cruz G, Chakrabarti SK, Patil VU, Skryabin G, Kuznetsov BB, Ravin NV, Kolganova TV, Beletsky AV, Mardanov AV, Di Genova A, Bolser DM, Martin DMA, Li G, Yang Y, Kuang H, Hu Q, Xiong X, Bishop GJ, Sagredo B, Mejía N, Zagorski W, Gromadka R, Gawor J, Szczesny P, Huang S, Zhang Z, Liang C, He J, Li Y, He Y, Xu J, Zhang Y, Xie B, Du Y, Qu D, Bonierbale M, Ghislain M, Herrera MDR, Giuliano G, Pietrella M, Perrotta G, Facella P, O’Brien K, Feingold SE, Barreiro LE, Massa GA, Diambra L, Whitty BR, Vaillancourt B, Lin H, Massa AN, Geoffroy M, Lundback S, DellaPenna D, Buell CR, Sharma SK, Marshall DF, Waugh R, Bryan GJ, Destefanis M, Nagy I, Milbourne D, Thomson SJ, Fiers M, Jacobs JME, Nielsen KL, Sønderkær M, Iovene M, Torres GA, Jiang J, Veilleux RE, Bachem CWB, De Boer J, Borm T, Kloosterman B, Van Eck H, Datema E, Hekkert BL, Goverse A, Van Ham RCHJ, Visser RGF (2011) Genome sequence and analysis of the tuber crop potato. Nature 475, 465-470.
| Google Scholar |
Yang W, Yang M, Wen H, Jiao Y (2018) Global warming potential of CH4 uptake and N2O emissions in saline–alkaline soils. Atmospheric Environment 191, 172-180.
| Crossref | Google Scholar |
Zameer R, Fatima K, Azeem F, ALgwaiz HIM, Sadaqat M, Rasheed A, Batool R, Shah AN, Zaynab M, Shah AA, Attia KA, AlKahtani MDF, Fiaz S (2022) Genome-wide characterization of superoxide dismutase (SOD) genes in Daucus carota: novel insights into structure, expression, and binding interaction with hydrogen peroxide (H2O2) under abiotic stress condition. Frontiers in Plant Science 13, 870241.
| Crossref | Google Scholar | PubMed |
Zeiner A, Colina FJ, Citterico M, Wrzaczek M (2023) CYSTEINE-RICH RECEPTOR-LIKE PROTEIN KINASES: their evolution, structure, and roles in stress response and development. Journal of Experimental Botany 74, 4910-4927.
| Crossref | Google Scholar |
Zhang W, Ruan J, Ho TD, You Y, Yu T, Quatrano RS (2005) Cis-regulatory element based targeted gene finding: genome-wide identification of abscisic acid-and abiotic stress-responsive genes in Arabidopsis thaliana. Bioinformatics 21, 3074-3081.
| Crossref | Google Scholar | PubMed |
Zhang X, Han X, Shi R, Yang G, Qi L, Wang R, Li G (2013) Arabidopsis cysteine-rich receptor-like kinase 45 positively regulates disease resistance to Pseudomonas syringae. Plant Physiology and Biochemistry 73, 383-391.
| Crossref | Google Scholar | PubMed |
Zhu T, Xin F, Wei S, Liu Y, Han YC, Xie J, Ding Q, Ma L (2019) Genome-wide identification, phylogeny and expression profiling of class III peroxidases gene family in Brachypodium distachyon. Gene 700, 149-162.
| Crossref | Google Scholar | PubMed |
Zuo C, Liu H, Lv Q, Chen Z, Tian Y, Mao J, Chu M, Ma Z, An Z, Chen B (2020) Genome-wide analysis of the apple (Malus domestica) cysteine-rich receptor-like kinase (CRK) family: annotation, genomic organization, and expression profiles in response to fungal infection. Plant Molecular Biology Reporter 38, 14-24.
| Crossref | Google Scholar |