Upregulation of TaHSP90A transcripts enhances heat tolerance and increases grain yield in wheat under changing climate conditions
Ali Ammar A , Zulfiqar Ali
A
B
C
D
E
Handling Editor: Inzamam Haq
Abstract
Plants have certain adaptation mechanisms to combat temperature extremes and fluctuations. The heat shock protein (HSP90A) plays a crucial role in plant defence mechanisms under heat stress. In silico analysis of the eight TaHSP90A transcripts showed diverse structural patterns in terms of intron/exons, domains, motifs and cis elements in the promoter region in wheat. These regions contained cis elements related to hormones, biotic and abiotic stress and development. To validate these findings, two contrasting wheat genotypes E-01 (thermo-tolerant) and SHP-52 (thermo-sensitive) were used to evaluate the expression pattern of three transcripts TraesCS2A02G033700.1, TraesCS5B02G258900.3 and TraesCS5D02G268000.2 in five different tissues at five different temperature regimes. Expression of TraesCS2A02G033700.1 was upregulated (2-fold) in flag leaf tissue after 1 and 4 h of heat treatment in E-01. In contrast, SHP-52 showed downregulated expression after 1 h of heat treatment. Additionally, it was shown that under heat stress, the increased expression of TaHSP90A led to an increase in grain production. As the molecular mechanism of genes involved in heat tolerance at the reproductive stage is mostly unknown, these results provide new insights into the role of TaHSP90A transcripts in developing phenotypic plasticity in wheat to develop heat-tolerant cultivars under the current changing climate scenario.
Keywords: abiotic stress, climate change, food security, functional genomics, heat shock proteins, heat tolerance, spatial and temporal expression, Triticum aestivum L.
Introduction
Global warming has increased temperature stress in recent years due to its incessant progress (Zhao et al. 2017), which continuously affects plant growth (Lesk et al. 2016) and development (Ahuja et al. 2010). Among the grains, wheat is the most widely grown crop in Pakistan, both in terms of acreage and productivity (Tahir et al. 2023). Wheat yield is impacted by changes in the environment, such as stress from water, heat, cold and salt (Irshad et al. 2022, Mohammadi et al. 2023). The growth and development of wheat are adversely affected by heat stress at almost all stages (Chauhan et al. 2011). Crop production and quality are also affected by regular small spells of high temperatures (Liu et al. 2014). The high temperatures experienced during flowering and early grain-filling (Maphosa et al. 2014) negatively impact wheat production through reduced grain number and size (Gouache et al. 2012). At post-anthesis, wheat grain-filling rate can decrease with an increase in temperature (Al-Khatib and Paulsen 1990), and the same stressor imposed before anthesis (Farooq et al. 2011) can also decrease yield (Tashiro and Wardlaw 1990) by reducing the number of grains per spike (Wheeler et al. 1996). Higher temperatures can decrease yields and grain quality in wheat (Maestri et al. 2002).
As a response to maintaining cell stability, a large amount of heat shock proteins (HSPs) (De Maio 1999) are accumulated and activated (Wang et al. 2016) in several organisms under heat stress (Richter et al. 2010). In this regard, the role of heat shock proteins in heat tolerance is crucial. At high temperatures, HSP transcripts are upregulated, which plays a crucial role in heat-stress response (Zuehlke and Johnson 2010). They are the most abundant, comprising 1–2% of the total cellular proteins, and play a role in cellular functions (Rizzolo et al. 2014). HSP90A acts as a multichaperone machine in plants, but its level increases under stressed conditions. HSP90A is expressed under light, dark, cold, heat, and in the presence of phytohormones, heavy metals and salt stress (Krishna and Gloor 2001). These proteins increase up to 10 fold compared to those in normal conditions (Wahid et al. 2007). Several HSP90 genes have been recognised, and they are strongly induced by fluctuations in temperature (Majoul et al. 2004). The manifestation of HSP90A is driven by temperature variation, which also exhibited a substantial association with the expression of HSP90A (Ammar et al. 2023). There are 44 plant species carrying the HSP90 gene family (Li et al. 2020), including Nicotiana tabacum (Song et al. 2019), Hordeum vulgare (Chaudhary et al. 2019) and Camellia sinensis (Chen et al. 2018). Upregulation of TaHSP90A transcripts in wheat genotypes resulted in enhanced grain production and heat tolerance (Ammar et al. 2023). The previous studies indicated that the HSP90A family genes are multifunctional in the life cycle of plants.
The current study thus hypothesised that the TaHSP90A transcripts express differentially when exposed to high temperature and may lead to cell membrane thermal stability, lower canopy temperature and higher thousand-kernel weight and grain yield. The transcript abundance of TaHSP90A transcripts was studied in two wheat genotypes (of which E-01 was the most tolerant, and SHP-52 the most sensitive) with different morphological, physiological and yield traits. Furthermore, the spatiotemporal expression of TaHSP90A transcripts was differentially induced by heat across different tissues and time intervals. This study provides information about the roles of TaHSP90A transcripts in response to heat stress, and may provide the basis for further research on the function of these transcripts and wheat resistance breeding.
Materials and methods
In silico analysis of TaHSP90A transcripts
To evaluate the evolutionary relationships of TaHSP90A, phylogenetic analysis was conducted with Arabidopsis, rice (Oryza sativa), soya bean (Glycine max), unicellular (Chlamydomonas) and wheat (Triticum aestivum) by using protein sequences. The tree was constructed based on multiple aligned protein sequences by maximum likelihood method in MEGA 6.05. The MEGA 6.05 Clustal W program was used to align multiple sequences.
For visualising the features, the intron and exons of TaHSP90A were studied using Gene Structure Display Server (http://gsds.cbi.pku.edu.cn/). The conserved motifs in the TaHSP90A proteins identified in the wheat crop were analysed with MEME server8 (Bailey et al. 2006).
TaHSP90A proteins were submitted to the National Center for Biotechnology Information (NCBI) conserved domain database and visualised using the TB tool. Fifteen conserved motifs were predicted by using the MEME web server. Based on the annotation information in the Pfam database.
The promoter sequences of each TaHSP90A member were retrieved from the wheat genome database. The Plant CARE database (Lescot et al. 2002) was used for analysing the cis-acting regulatory elements in the promoters of each wheat TaHSP90A, and a map was constructed by using TB tool software.
Plant materials and environmental conditions
The present experiment was conducted in the molecular breeding laboratory and experimental area of the Department of Plant Breeding and Genetics, Faculty of Agriculture and Environmental Sciences, MNS University of Agriculture, Multan, Pakistan, located at 29°24′0″N, 71°41′60 0″E. Experimental material comprised of two wheat (Triticum aestivum L.) genotypes, E-01 (heat tolerant) and SHP-52 (heat sensitive, Supplementary Table S1) obtained from MNS University of Agriculture. Both genotypes were grown under two treatments (normal as a control and with a high temperature regime up to +5°C from ambient). The temperature was controlled by a small tunnel containing a plastic sheath, cooling pads, fans and a digital thermometer. Heat treatment was given from 10:00 am to 4:00 pm for heat (high temperature). For spatiotemporal transcription of TaHSP90A transcripts, samples were collected at two leaf stages, from anthers, flag leaf, leaves at grain-filling and from grains. For temporal expression, samples were collected at 0, 1, 2, 4 and 6 h of heat treatment.
Sample collection and RNA isolation
Protein sequences of all the transcripts were downloaded from the plant genomic resource Phytozome (https://phytozome-next.jgi.doe.gov/info/Taestivum_v2_2) and primers were designed using AmplifX (ver. 1.7.0). RNA samples were taken from leaves (two leaf stage), anthers, flag leaf, leaves at grain-filling and grains, at 0, 1, 2, 4 and 6 h after heat treatment. RNA was extracted from the samples using the TRIzol protocol mentioned by Liu et al. (2018) and a spectrophotometer (nanodrop 2000c, Thermo Fisher Scientific, WA, USA) was used for the recording of quantification, before complementary DNA (cDNA) synthesis concentrations were optimised. cDNA synthesis was done following manufacturer guidelines (Thermo Fisher Scientific, 2000). Semi-quantitative polymerase chain reaction (semi-qPCR) was performed on synthesised cDNA obtained from plant material for the expression analysis of TaHSP90A transcripts as described by Ali et al. (2012).
cDNA synthesis
The total isolated RNA was quantified by spectrophotometer (nanodrop 2000c, Thermo Fisher Scientific) to calculate its concentration. The loaded samples were further examined under ultraviolet (UV) light in a gel documentation apparatus (Omega Fluor™ Plus Gel Documentation System) to access the integrity of total isolated RNA. After measuring the concentration of total isolated RNA, 500 ng of total isolated RNA per sample was used for the synthesis of the first strand of cDNA. Taking 5 μL RNA in the PCR tube, 6.5 μL of nuclease free water and 1 μL oligo dT were added to make the total volume 12.5 μL. Samples were incubated at 65°C for 5 min in a thermocycler and kept on ice to lower the temperature to 4°C. To make the volume up to 20 μL, 0.5 μL Ribo lock RNase inhibitor, 4 μL 5× reaction buffer, 2 μL 10 mM dNTP’s Mix and 1 μL of RevertAid Reverse Transcriptase were added. The samples were run in a thermocycler at 42°C for 60 min, 70°C for 10 min, 10°C for 15 min and final termination at 72°C for 1 min.
Semi-qPCR
An optimisation for semi-qPCR cycles was done at 30, 32, 34, 35, 36, 39 and 40 cycles to obtain the product at the plateau stage. Primers used in expression analysis are listed in Table 1. The final concentration of reagents used in the PCR are listed in Table S2. The analysis was repeated in three independent experiments. The PCR product was stained with ethidium bromide, run on 1% of agarose gel and observed under UV radiance using the gel documentation apparatus (Omega Fluor™ Plus Gel Documentation System). The results were quantified through ImageJ software (Saddique et al. 2020) to examine the expression of TaHSP90A transcripts under normal and heat stress. Numerical values showing band strength were used to calculate the change in expression of TaHSP90A transcripts.
Sr. no. | Gene locus | Temp. (°C) | Amplicon size | 5′F | 5′R | |
---|---|---|---|---|---|---|
T1 | TraesCS2A02G033700.1 | 58.3 | 2448 | AAGCTTCGGGACAAGGCTC | TCAGTCGACCTCCTCCATCT | |
T2 | TraesCS5B02G258900.3 | 54.3 | 2063 | ATGTATACAGATAAAACTTCATCG | CTACCTTTCAGTGGAGATCGA | |
T3 | TraesCS5D02G268000.2 | 61.6 | 1276 | ATGAGGAAGCCAGAGGAGAT | CCACAAACAGCTAACCGCG |
Results
Phylogenetic analysis of TaHSP90A transcripts
Phylogenetic tree clustered transcripts into three groups: I, II and III. There are two, one and three TaHSP90A transcripts in the I, II and III groups, respectively, which are further divided into sub groups (Fig. 1). Whereas, two TaHSP90A transcripts remained ungrouped, indicating that these were different from the genes in Arabidopsis, rice and soya bean.
Gene structure analysis of TaHSP90A transcripts
Gene structure and functional similarity were found among TaHSP90A transcripts, with an evolutionary relationship suggesting that the function of TaHSP90A transcripts could be predicted based on similarity in Arabidopsis and rice. The exon/intron distribution results showed that number of introns ranged from one to two and exons ranged from one to three. Most of them showed both upstream and downstream regions. It was observed that the closely related transcripts showed similar numbers of exons and lengths of introns, whereas distantly related transcripts had variable numbers and lengths of exons and introns, respectively. The length of exons and introns of TaHSP90A transcripts in wheat showed the diversity in gene structure shown in Fig. 2. Closely related TaHSP90A transcripts showed similar distributions of exon and intron, suggesting that the functions of TaHSP90A transcripts in the same group were similar.
Motif analysis of TaHSP90A transcripts
Motifs 1, 2, 3, 5 and 8 were most conserved and shared by most members of TaHSP90A proteins. Motif composition varied among the different transcripts and it is similar among the similar transcripts, showing that these have conserved domains. However, the differences in motif distribution show that the functions of these genes might have diverged during evolution as shown in Fig. 3.
Promoter analysis of TaHSP90A transcripts
For TaHSP90A transcripts, 21 main types of cis elements were identified in their promoter sequences. The number of various cis elements in the promoters of each TaHSP90A transcript is shown in Fig. 4. The number of cis elements observed across the TaHSP90A transcripts was associated with growth and development, such as MRE, G-box and Box 4. In addition, various cis elements are involved in phytohormone response and biotic and abiotic stress responses (e.g. heat and defence). The different cis elements were present in the promoter regions of different TaHSP90A transcripts, indicating that these performed multiple functions.
Morphophysiological and yield traits of tolerant and sensitive genotypes
Significant differences were observed between heat-tolerant and sensitive genotypes for various morphopysiological and yield related traits (Fig. 5). Tolerant wheat genotype (G1) showed a positive response to yield and yield contributing traits along with physiological traits as compared to sensitive genotype (G2). Days to heading (DH) and maturity (DM) were reduced by −5 and −7%, respectively, for the heat-tolerant genotype (G1). Similarly, for plant height (PH) and peduncle length (PdL), reductions were observed of −5 and −27%, respectively, as compared to the sensitive genotype (G2). In contrast, a significant increase was observed in yield (Y) (343%), spike length (SL) (51%), spikelets/spike (SPS) (44%), grains per spike (GPS) (22%) and 1000-grain weight (TGW) (25%) for tolerant genotype. In the case of physiological traits, tolerant genotype showed a 34% increase in photosynthetic rate (PR) and 12% for stomatal conductance (SC). For traits like canopy temperature (CT) and relative cell injury (RCI), a reduction was observed of 37% and 63%, respectively, in the tolerant genotype (Fig. 6).
Morphophysiological and yield traits of tolerant and sensitive genotypes. E-01, heat tolerant; SHP-52, heat sensitive; DH, days to heading; DM, days to maturity; PH, plant height (cm); SL, spike length (cm); PdL, peduncle length (cm); SPS, spikelets/spike; FLA, flag leaf area (cm2); GPS, grains/spike; TGW, 1000-grain weight (g); Y, grain yield (g).
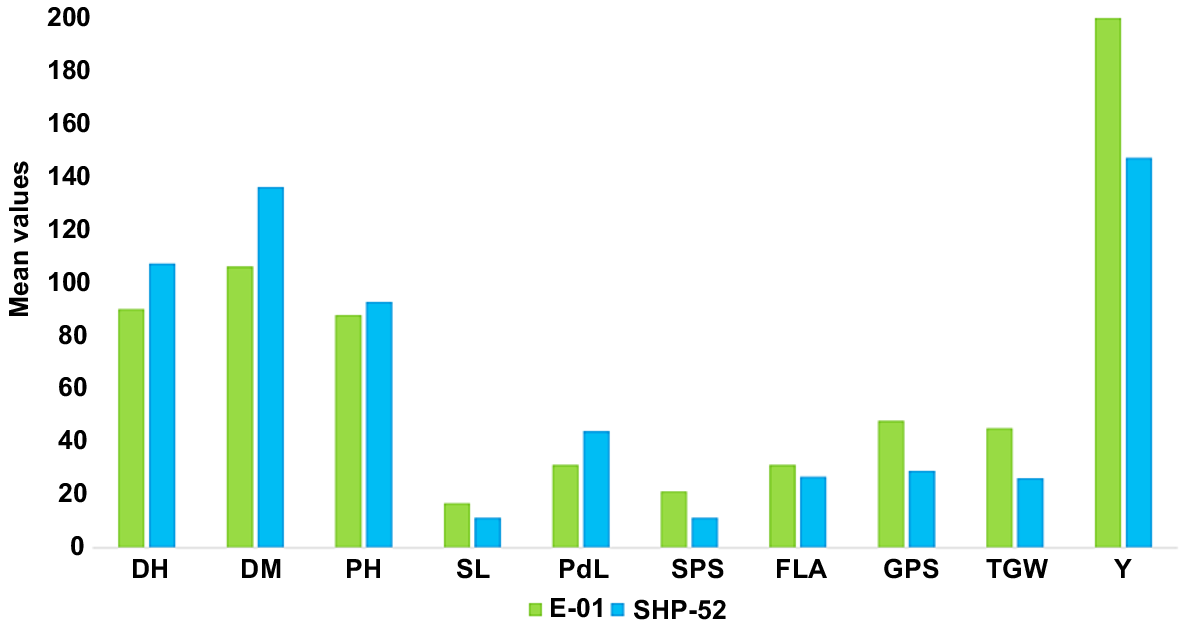
Expression profiling of TaHSP90A transcripts
Some heat shock proteins were generally upregulated under heat and other stresses. In the case of wheat, TaHSP90A transcripts showed variable expression in different tissues at different time intervals for tested wheat genotypes under both normal and heat-stress conditions (Fig. 7). Under normal conditions, tolerant genotype G1 (E-01) showed differential expression for all three T1 (TraesCS2A02G033700.1), T2 (TraesCS5B02G258900.3) and T3 (TraesCS5D02G268000.2) transcripts from flag leaf, anthers and leaves at grain-filling. However it showed no expression in leaves from the two leaf stage nor in grains. On the other hand, sensitive genotype G2 (SHP-52) showed expression from flag leaf and anthers for transcripts T1 and T2, respectively, and showed no significant expression from other tissues. Under high temperatures, genotype G1 (E-01) also showed differential expression from flag leaf, anthers and leaves at grain-filling after 1, 2 and 4 h of heat treatment for all three transcripts. The genotype E-01 showed 2-fold upregulation of Transcripts 1, 2 and 3 from the flag leaf tissue after 1 h and 4 h of heat treatment. In contrast, SHP-52 showed downregulated expression in flag leaf tissue after 1 h of heat treatment (Fig. 8).
Transcript abundance of two wheat genotypes (G1 and G2) under normal (N) and high temperature (HT) from different tissues, where T1 indicates Transcript 1; T2, Transcript 2; T3, Transcript 3; and 0, 1, 2, 4 and 6 indicate the time in hours after treatment when samples were investigated. UB, ubiquitin (internal control).
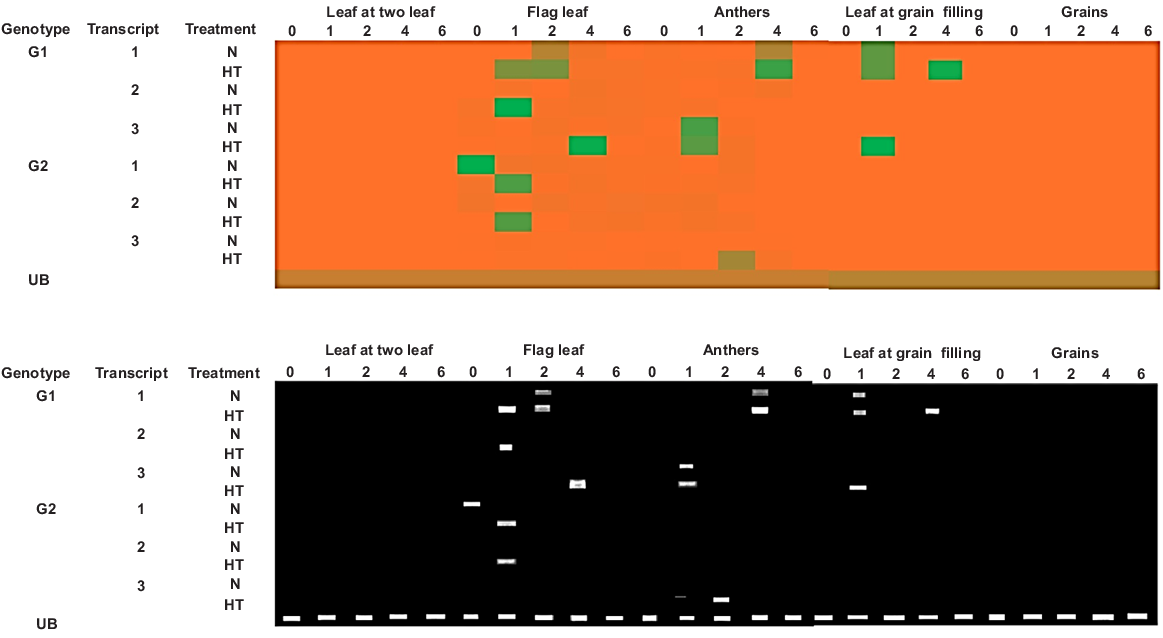
Relative expression of two wheat genotypes (G1 and G2) under normal and high temperature from different tissues, where T1 indicates Transcript 1; T2, Transcript 2; T3, Transcript 3; and 0, 1, 2, 4 and 6 indicate the time in hours after treatment when samples were investigated. Ubiqutin was used as a housekeeping gene. Values represent data from three biological replicates and three technical replicates. Error bars indicate values ± s.d.
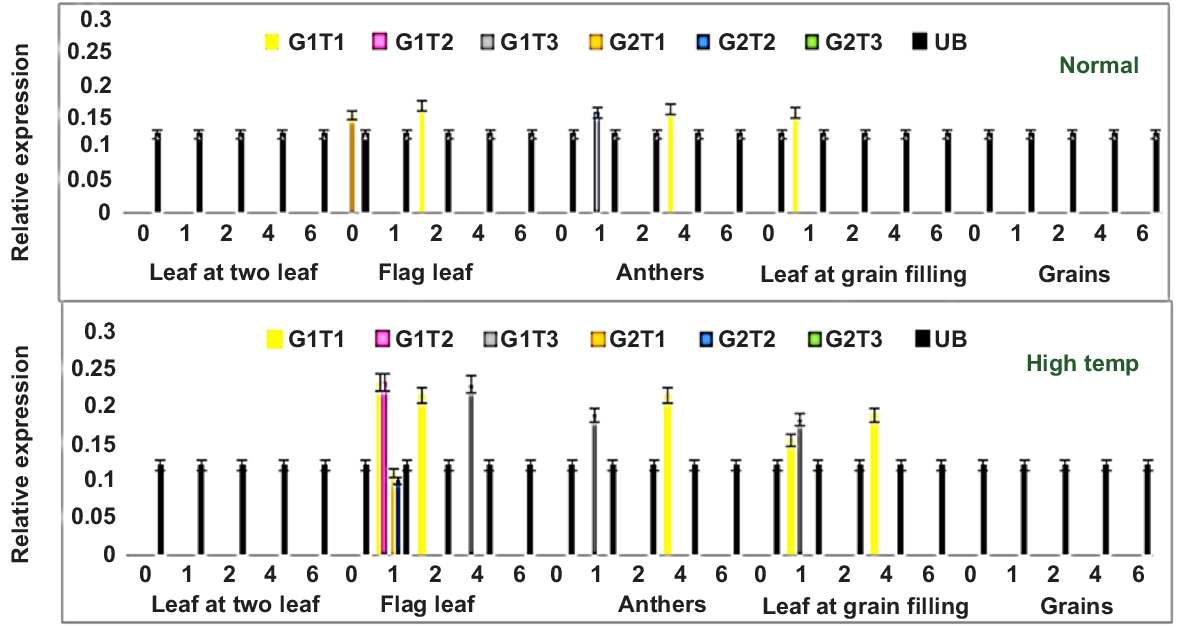
It is clear that G1 (E-01) showed the upregulation of these transcripts in different tissues at different time intervals under heat stress. In contrast, G2 (SHP-52) showed very little or no significant expression for all transcripts from different tissues after several time intervals, both under normal and heat stress.
Discussion
Wheat and other economically significant crops are particularly vulnerable to heat stress, which is often regarded as the most harmful abiotic stress factor (Lee et al. 2022). Environmental stress is countered by HSP90s in plants (Gil et al. 2017), which are extensively involved in many significant biological processes (Pearl and Prodromou 2006). In animal and fungal systems, the functions of HSP90 genes have been widely studied (Yabe et al. 1994), but little is known about their function in plants. More plant genome information is becoming available now, due to the continuous development of genome sequencing technology. Identification of HSP90 was reported in Arabidopsis (Krishna and Gloor 2001) and rice (Hu et al. 2009) as well as in some vegetables like tomato (Solanum lycopersicum) (Liu et al. 2014). In several crops, HSP90s have been identified and their expression patterns extensively analysed (Swindell et al. 2007).
Considering wheat’s short ploidy history, it is used in the study of polyploidy evolution as a model plant (Borrill et al. 2015). Wheat genes’ expression partitioning can be observed at the transcriptional level, and approximately 68% of the genes are partially expressed under stress (Zheng et al. 2016) such as heat, drought and salt (Leach et al. 2014). Thus, in wheat, gene expression partitioning among homologous genes is considered an approach for abiotic stress tolerance (Zuehlke and Johnson 2010).
In this study, a total of eight TaHSP90A transcripts were investigated in wheat. According to the phylogenetic and gene structure analysis, most of the TaHSP90A transcripts showed a similar exon/intron structure and conserved motif. It is possible that the evolution of some TaHSP90A transcripts is affecting not only the functional aspects of the genes, but the structure as well (Babenko et al. 2004; Roy and Penny 2007). Promoter analysis of wheat TaHSP90As indicated that they contained plant growth and development, phytohormone responsiveness and biotic and abiotic stress related cis-elements, suggesting that these play crucial roles in protein degradation and protein trafficking (Richter and Buchner 2001; Young et al. 2001).
Three TaHSP90A transcripts (TraesCS2A02G033700.1, TraesCS5B02G258900.3 and TraesCS5D02G268000.2) were investigated for their expression in the different tissues, where they were required for growth and development and were essential for heat tolerance. Results revealed that all three TaHSP90A transcripts responded to heat stress. According to certain reports, HSP90As were markedly increased when under heat stress (Lu et al. 2020). In several tissues, TaHSP90A transcripts had low expression under normal conditions, whereas their expression was sharply upregulated after 1 h, 2 h and 4 h of heat treatment, suggesting that they played a vital role in combating heat stress. Previous studies also showed that HSP90As were upregulated during heat stress in Arabidopsis (Borrill et al. 2015). TaHSP90A transcripts were used in the wheat breeding programme, which significantly increased grain yield and tolerance to heat stress (Ammar et al. 2023). All three TaHSP90A transcripts were heat responsive in flag leaf tissue, anthers and leaves at grain-filling, indicating that the functions of these transcripts were diverse in different tissues. However, it is also worth noting that TaHSP90A transcripts did not respond to heat stress in leaf at two leaf stages and in grains, suggesting that these two tissues have distinct response networks. The data we used were mainly from short term stress, and the TaHSP90A transcripts that do not respond in grains may also respond to heat stress in other conditions. Additionally, it was shown that under heat stress, TaHSP90A gene expression was increased, and this increased expression led to an increase in grain production.
All transcripts were significantly expressed after 1 h of heat treatment, and TaHSP90A transcripts rapidly responded to heat stress. It was found that the expression levels of TaHSP90A Transcript 1 (TraesCS2A02G033700.1) at high temperatures were higher, signifying that the transcript responded to high temperature stress. Two-fold upregulations of all TaHSP90A transcripts were observed in the flag leaf after 1 h and 4 h of heat treatment in the case of tolerant genotype E-01. The sensitive genotype (SHP-52) showed less significant expression as compared to the tolerant (E-01) genotype. Upregulation of TaHSP90A transcripts improves heat tolerance in wheat, which occurs mainly in flag leaf in response to stress conditions. The results indicated that wheat plants with these TaHSP90A transcripts had the potential to cope with heat stress.
Conclusions
Based on the morphological, physiological and spatiotemporal expression analysis, we concluded that TaHSP90A transcripts were upregulated in wheat genotypes, and these genotypes produced more grain and were more heat tolerant. This study not only provided an understanding of the TaHSP90A transcripts, but also showed the expression patterns in different tissues, under various conditions, including normal and high temperature stress. It could also help in selecting new wheat varieties with high yields and better tolerance to heat stress under changing climate conditions. This work advances knowledge of the wheat heat-stress response during the early growth stage as well as the variations in heat tolerance. This data can provide an important reference for further studies of the functions of TaHSP90A transcripts and contribute to the genetic improvement of wheat.
Author contributions
Z.A, conceptualisation of study; A.A and M.A.B.S, experimental design; A.A, performing experiments, data curation and writing original draft; A.A, I.A and M.A.B.S, data analysis; Z.A. and M.H.R, critical review and editing.
Acknowledgements
This research was funded by the Institute of Plant Breeding and Biotechnology, MNS University of Agriculture, Multan, Punjab Agriculture Research Board and Government of Punjab, Pakistan.
References
Ahuja I, de Vos RCH, Bones AM, Hall RD (2010) Plant molecular stress responses face climate change. Trends in Plant Science 15(12), 664-674.
| Crossref | Google Scholar | PubMed |
Ali Z, Zhang DY, Xu ZL, Yi JX, He XL, Huang YH, Liu XU, Khan AA, Trethowan RM, Ma HX (2012) Uncovering the salt response of soybean by unraveling its wild and cultivated functional genomes using tag sequencing. PLoS ONE 7(11), e48819.
| Crossref | Google Scholar | PubMed |
Al-Khatib K, Paulsen GM (1990) Photosynthesis and productivity during high temperature stress of wheat genotypes from major world regions. Journal of Crop Science 30(5), 1127-1132.
| Crossref | Google Scholar |
Ammar A, Ali Z, Saddique MAB, Habib-Ur-Rahman M, Ali I (2023) Genetic analysis and expression profiling of TaHSP90A transcripts confer heat tolerance in wheat. SABRAO Journal of Breeding and Genetics 55(3), 653-670.
| Crossref | Google Scholar |
Babenko VN, Rogozin IB, Mekhedov SL, Koonin EV (2004) Prevalence of intron gain over intron loss in the evolution of paralogous gene families. Nucleic Acids Research 32(12), 3724-3733.
| Crossref | Google Scholar | PubMed |
Bailey TL, Williams N, Misleh C, Li WW (2006) MEME: discovering and analyzing DNA and protein sequence motifs. Nucleic Acids Research 34, W369-W373.
| Crossref | Google Scholar | PubMed |
Borrill P, Adamski N, Uauy C (2015) Genomics as the key to unlocking the polyploid potential of wheat. New Phytologist 208(4), 1008-1022.
| Crossref | Google Scholar | PubMed |
Chaudhary R, Baranwal VK, Kumar R, Sircar D, Chauhan H (2019) Genome-wide identification and expression analysis of Hsp70, Hsp90, and Hsp100 heat shock protein genes in barley under stress conditions and reproductive development. Functional and Integrative Genomics 19, 1007-1022.
| Crossref | Google Scholar | PubMed |
Chauhan H, Khurana N, Tyagi AK, Khurana JP, Khurana P (2011) Identification and characterization of high temperature stress responsive genes in bread wheat (Triticum aestivum L.) and their regulation at various stages of development. Plant Molecular Biology 75, 35-51.
| Crossref | Google Scholar | PubMed |
Chen J, Gao T, Wan S, Zhang Y, Yang J, Yu Y, et al. (2018) Genome-wide identification, classification and expression analysis of the HSP gene superfamily in tea plant (Camellia sinensis). International Journal of Molecular Sciences 19(9), 2633.
| Crossref | Google Scholar | PubMed |
De Maio A (1999) Heat shock proteins: facts, thoughts, and dreams. Shock 11(1), 1-12.
| Crossref | Google Scholar | PubMed |
Farooq M, Bramley H, Palta JA, Siddique KHM (2011) Heat stress in wheat during reproductive and grain-filling phases. Critical Reviews in Plant Sciences 30(6), 491-507.
| Crossref | Google Scholar |
Gil KE, Kim WY, Lee HJ, Faisal M, Saquib Q, Alatar AA, Park CM (2017) Zeitlupe contributes to a thermoresponsive protein quality control system in Arabidopsis. The Plant Cell 29(11), 2882-2894.
| Crossref | Google Scholar | PubMed |
Gouache D, Bris XL, Bogard M, Deudon O, Page C, Gate P (2012) Evaluating agronomic adaptation options to increasing heat stress under climate change during wheat grain filling in France. European Journal of Agronomy 39, 62-70.
| Crossref | Google Scholar |
Hu W, Hu G, Han B (2009) Genome-wide survey and expression profiling of heat shock proteins and heat shock factors revealed overlapped and stress specific response under abiotic stresses in rice. Plant Science 176((4)), 583-590.
| Crossref | Google Scholar |
Irshad A, Ahmed RI, Ur Rehman S, Sun G, Ahmad F, Sher MA, Aslam MZ, Hassan MM, Qari SH, Aziz MK, Khan Z (2022) Characterization of salt tolerant wheat genotypes by using morpho-physiological, biochemical, and molecular analysis. Frontier in Plant Science 13, 956298.
| Crossref | Google Scholar |
Krishna P, Gloor G (2001) The HSP90 family of proteins in Arabidopsis thaliana. Cell Stress and Chaperones 6(3), 238-246.
| Crossref | Google Scholar | PubMed |
Leach LJ, Belfield EJ, Jiang C, Brown C, Mithani A, Harberd NP (2014) Patterns of homoeologous gene expression shown by RNA sequencing in hexaploid bread wheat. BMC Genomics 15(1), 276.
| Crossref | Google Scholar |
Lee MH, Kim KM, Sang WG, Kang CS, Choi C (2022) Comparison of gene expression changes in three wheat varieties with different susceptibilities to heat stress using RNA Seq analysis. Internal Journal of Molecular Sciences 23, 10734.
| Crossref | Google Scholar | PubMed |
Lescot M, Déhais P, Thijs G, Marchal K, Moreau Y, Van de Peer Y, Rouzé P, Rombauts S (2002) Plant care, a database of plant cis-acting regulatory elements and a portal to tools for in silico analysis of promoter sequences. Nucleic Acids Research 30(1), 325-327.
| Crossref | Google Scholar | PubMed |
Lesk C, Rowhani P, Ramankutty N (2016) Influence of extreme weather disasters on global crop production. Nature 529(7584), 84-87.
| Crossref | Google Scholar | PubMed |
Li W, Chen Y, Ye M, Wang D, Chen Q (2020) Evolutionary history of the heat shock protein 90 (Hsp90) family of 43 plants and characterization of Hsp90s in Solanum tuberosum. Molecular Biological Reports 47(9), 6679-6691.
| Crossref | Google Scholar | PubMed |
Liu Y, Wan H, Yang Y, Wei Y, Li Z, Ye Q, Wang R, Ruan M, Yao Z, Zhou G (2014) Genome-wide identification and analysis of heat shock protein 90 in tomato. Hereditas 36(10), 1043-1052.
| Google Scholar | PubMed |
Liu L, Han R, Yu N, Zhang W, Xing L, Xie D, Peng D (2018) A method for extracting high-quality total RNA from plant rich in polysaccharides and polyphenols using Dendrobium huoshanense. PLoS ONE 13(5), e0196592.
| Crossref | Google Scholar | PubMed |
Lu Y, Zhao P, Zhang A, Ma L, Xu S, Wang X (2020) Alternative splicing diversified the heat response and evolutionary strategy of conserved Heat Shock Protein 90s in hexaploid wheat (Triticum aestivum L.). Frontier in Genetics 11, 577897.
| Crossref | Google Scholar |
Maestri E, Klueva N, Perrotta C, Gulli M, Nguyen HT, Marmiroli N (2002) Molecular genetics of heat tolerance and heat shock proteins in cereals. Plant Molecular Biology 48, 667-681.
| Crossref | Google Scholar | PubMed |
Majoul T, Bancel E, Triboï E, Ben Hamida J, Branlard G (2004) Proteomic analysis of the effect of heat stress on hexaploid wheat grain: characterization of heat-responsive proteins from non-prolamins fraction. Proteomics 4(2), 505-513.
| Crossref | Google Scholar | PubMed |
Maphosa L, Langridge P, Taylor H, Parent B, Emebiri LC, Kuchel H, Reynolds MP, Chalmers KJ, Okada A, Edwards J, Mather DE (2014) Genetic control of grain yield and grain physical characteristics in a bread wheat population grown under a range of environmental conditions. Theoratical & Applied Genetics 127(7), 1607-1624.
| Crossref | Google Scholar | PubMed |
Mohammadi AS, Hadi H, Toorchi M, Pawłowski TA, Asgari LB, Price GW, Farooq M, Astatkie T (2023) Morpho-physiological responses and growth indices of triticale to drought and salt stresses. Scientific Reports 13(1), 8896.
| Crossref | Google Scholar |
Pearl LH, Prodromou C (2006) Structure and mechanism of the HSP90 molecular chaperone machinery. Annual Review of Biochemistry 75, 271-294.
| Crossref | Google Scholar |
Richter K, Buchner J (2001) Hsp90: chaperoning signal transduction. Journal of Cellular Physiology 188(3), 281-290.
| Crossref | Google Scholar | PubMed |
Richter K, Haslbeck M, Buchner J (2010) The heat shock response: life on the verge of death. Molecular Cell 40(2), 253-266.
| Crossref | Google Scholar | PubMed |
Rizzolo K, Wong P, Tillier ERM, Houry WA (2014) The interaction network of the HSP90 molecular chaperone. In ‘The molecular chaperones interaction networks in protein folding and degradation’. (Ed. W Houry) pp. 111–131. (Springer) doi:10.1007/978-1-4939-1130-1_5
Roy SW, Penny D (2007) On the incidence of intron loss and gain in paralogous gene families. Molecular Biology and Evolution 24(8), 1579-1581.
| Crossref | Google Scholar | PubMed |
Saddique MAB, Ali Z, Sher MA, Farid B, Ikram RM, Ahmad MS (2020) Proline, total antioxidant capacity, and OsP5CS gene activity in radical and plumule of rice are efficient drought tolerance indicator traits. International Journal of Agronomy 2020, 8862792.
| Crossref | Google Scholar |
Song Z, Pan F, Yang C, Jia H, Jiang H, He F, et al. (2019) Genome-wide identification and expression analysis of HSP90 gene family in Nicotiana tabacum. BMC Genetics 20, 35.
| Crossref | Google Scholar | PubMed |
Swindell WR, Huebner M, Weber AP (2007) Transcriptional profiling of Arabidopsis heat shock proteins and transcription factors reveals extensive overlap between heat and non-heat stress response pathways. BMC Genomics 8, 125.
| Crossref | Google Scholar | PubMed |
Tahir T, Rasheed A, Kayani S, Shahzad A (2023) High-throughput digital imaging analysis for grain morphology of historical wheat cultivars of Pakistan. Genetic Resources and Crop Evolution
| Crossref | Google Scholar |
Tashiro T, Wardlaw IF (1990) The response to high temperature shock and humidity changes prior to and during the early stages of grain development in wheat. Functional Plant Biology 17(5), 551-561.
| Crossref | Google Scholar |
Wahid A, Gelani S, Ashraf M, Foolad MR (2007) Heat tolerance in plants: an overview. Environmental & Experimental Botany 61(3), 199-223.
| Crossref | Google Scholar |
Wang R, Zhang Y, Kieffer M, Yu H, Kepinski S, Estelle M (2016) HSP90 regulates temperature-dependent seedling growth in Arabidopsis by stabilizing the auxin co-receptor F-box protein TIR1. Nature Communications 7, 10269.
| Crossref | Google Scholar |
Wheeler TR, Batts GR, Ellis RH, Hadley P, Morison JIL (1996) Growth and yield of winter wheat (Triticum aestivum) crops in response to CO2 and temperature. Journal of Agricultural Sciences 127(1), 37-48.
| Crossref | Google Scholar |
Yabe N, Takahashi T, Komeda Y (1994) Analysis of tissue-specific expression of Arabidopsis thaliana HSP90-family gene HSP81. Plant Cell Physiology 35(8), 1207-1219.
| Crossref | Google Scholar | PubMed |
Young JC, Moarefi I, Hartl FU (2001) Hsp90: a specialized but essential protein-folding tool. The Journal of Cell Biology 154(2), 267-273.
| Crossref | Google Scholar | PubMed |
Zhao C, Liu B, Piao S, Wang X, Lobell DB, Huang Y, Huang M, Yao Y, Bassu S, Ciais P, Durand J-L, Elliott J, Ewert F, Janssens IA, Li T, Lin E, Liu Q, Martre P, Müller C, Peng S, Peñuelas J, Ruane AC, Wallach D, Wang T, Wu D, Liu Z, Zhu Y, Zhu Z, Asseng S (2017) Temperature increase reduces global yields of major crops in four independent estimates. Proceedings of the National Academy of Sciences 114(35), 9326-9331.
| Crossref | Google Scholar |
Zheng B, Chenu K, Chapman SC (2016) Velocity of temperature and flowering time in wheat – assisting breeders to keep pace with climate change. Global Change Biology 22(2), 921-933.
| Crossref | Google Scholar | PubMed |
Zuehlke A, Johnson JL (2010) HSP90 and co-chaperones twist the functions of diverse client proteins. Biopolymers: Original Research on Biomolecules 93(3), 211-217.
| Crossref | Google Scholar |