On the effect of heavy water (D2O) on carbon isotope fractionation in photosynthesis
Guillaume Tcherkez A B D and Graham D. Farquhar CA Plateforme Métabolisme-Métabolome, IFR87, Université Paris-Sud XI, 91405 Orsay, France.
B Laboratoire d’écophysiologie végétale, CNRS UMR 8079, Université Paris-Sud XI, 91405 Orsay, France.
C Environmental Biology Group, Research School of Biological Sciences, Australian National University, Canberra, ACT 2601, Australia.
D Corresponding author. Email: guillaume.tcherkez@u-psud.fr
Functional Plant Biology 35(3) 201-212 https://doi.org/10.1071/FP07282
Submitted: 28 November 2007 Accepted: 18 February 2008 Published: 23 April 2008
Abstract
Internal conductance to carbon dioxide is a key aspect of leaf photosynthesis although is still not well understood. It is thought that it comprises two components, namely, a gas phase component (diffusion from intercellular spaces to cell walls) and a liquid phase component (dissolution, diffusion in water, hydration equilibrium). Here we use heavy water (D2O), which is known to slow down CO2 hydration by a factor of nearly three. Using 12C/13C stable isotope techniques and Xanthium strumarium L. leaves, we show that the on-line carbon isotope discrimination (Δ13C, or Δobs) associated with photosynthesis is not significantly decreased by heavy water, and that the internal conductance, estimated with relationships involving the deviation of Δ13C, decreased by 8–40% in 21% O2. It is concluded that in typical conditions, the CO2-hydration equilibrium does not exert an effect on CO2 assimilation larger than 9%. The carbon isotope discrimination associated with CO2 addition to ribulose-1,5,bisphosphate by Rubisco is slightly decreased by heavy water. This effect is proposed to originate from the use of solvent-derived proton/deuteron during the last step of the catalytic cycle of the enzyme (hydration/cleavage).
Acknowledgements
The authors wish to acknowledge the financial support provided by the franco-australian FAST project, under contract n°12795 WC. GT thanks Prof. Gabriel Cornic for valuable discussions about the manuscript. GD.F. acknowledges the support of the Australian Research Council.
Abdulkadirova KS,
Kostrowicka-Wyczalkowska A,
Anisimov MA, Sengers JV
(2002) Thermodynamic properties of mixtures of H2O and D2O in the critical region. Journal of Chemical Physics 116, 4597–4610.
| Crossref | GoogleScholarGoogle Scholar |
may be re-arranged to:
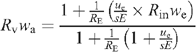
In the present study, RE = RS is 99.8/0.2, that is, 499, but inlet water is made of natural water (very small Rin). Here, ue/sERE is in the range 3.7 10−4 mol s–1/(10−2 m2 × 10−4 mol m–2 s–1 × 499) = 0.76, so that the right term in the numerator can be neglected and Rvwa ~1/1.76 = 0.57. With a value of wa = 0.01 mol mol–1, we find Rv~57. Using Eqn A1 we then have Re = 166, which is equivalent to 99.4% D2O. In other words, the dilution of heavy water in the leaf by inlet H2O is very small. This is consistent with the very small effect of heavy v. light inlet water on 12C/13C isotopic data in the present paper (Figs 1, 2).
Other steps possibly slowed down in heavy water
As already stated in the main text, heavy water has an effect on two main steps, namely, the Rubisco-catalysed reaction, and the CO2-HCO3– equilibrium, catalysed by carbonic anhydrase (Table 1). Other slowing effects caused by heavy water are negligible in the present study:
-
there is no effect of heavy water on the 12C/13C isotope effect associated with diffusion in water (Table 2), and
-
the effect of heavy water on the the 12C/13C isotope effect associated with dissolution in water is unknown, but one may assume it is small, as deuteration of water acts on dissolution through (a), the partition function of CO2 in the liquid phase and (b), the binding energy between solvent water molecules and CO2. The effect on binding is thought to be a negligible component (Vogel et al. 1970). Because the degrees of freedom and the motions of CO2 are similar, the partition function of CO2 in the liquid phase is likely not to be much influenced by deuteration of water, so that the change in the ratio of 13CO2 and 12CO2 solubility remains unchanged. This would be in agreement with the very small effect of atomic substitution changes on solubility in water: for example, the H2O/D2O isotope effect on the solubility of CH4 is 1.005 but that of (the much larger molecule) CF4 is 1.017 (Cosgrove and Walkley 1981). If an effect of heavy water on the 12C/13C isotope effect of dissolution were to occur, the overall impact would be very small, because of its order of magnitude (<1‰).
Thus, heavy water has roughly no effect on the carbon isotope fractionation by diffusion and dissolution (Table 2), and a change in the photosynthetic discrimination, if any, would have to be related to enzymatic effects.
Appendix II. Calculation of the conductance when inlet water is H2O
The outlet D2O vapour mole fraction, denoted as da, follows the mass-balance equation for heavy water, that is (as Rin is small compared with 1):

where ue is the entering air flow through the chamber, Φ is the water flux taken up by the leaf through the petiole, s is the leaf surface area, and Rs the D/H isotope ratio of source water. Rin and we are the D/H isotope ratio and the water mole fraction of inlet water, respectively. Because the ratio Rin is very small when natural water in used for inlet air, we have:

Although the leaf transpired heavy water, the natural vapour pressure (H2O of inlet air) had to be taken into account because it may have entered the leaf in the steady-state (see also above). At ambient temperatures, the combination of both natural and heavy waters behave linearly with respect to their relative quantities (Abdulkadirova et al. 2002) so that the saturation vapour pressure of the mixture at the evaporating sites is (1 − ε)di + εwi, where ε is the proportion of natural water at the evaporative sites, and wi and di are the saturation mole fractions of H2O and D2O at leaf temperature [in other words, the actual H2O vapour mole fraction in the leaf is εwi and that of D2O is (1 − ε)di]. Then the water exchange between the atmosphere and the leaf is made up of D2O loss, that is, gD2O ((1 − ε)di − da and H2O uptake, gH2O(wa − εwi), where wa is the outlet mole fraction of H2O. As the leaf compensated for the small diffusive influx of H2O by consuming less D2O through the petiole we have (assuming a mole-to-mole compensation):

where the diffusion ratio (H2O/D2O isotope effect) is denoted as gH2O/gD2O = αk (~1.040) and the saturation mole fraction pressure wi/di denoted as α+, The net heavy water uptake by the leaf is denoted as Φ. Rearranging, this gives:

If ε is small enough (see Appendix I), we define the ‘efficient’ heavy water mole fraction as:

The D2O saturation vapour pressure values (at leaf temperature), denoted as dsat, were taken from data compiled for the NIST chemistry webbook (http://webbook.nist.gov/chemistry) and from Besley and Bottomley (1973). Stomatal conductance to D2O is then given by:
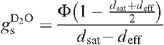
The CO2 conductance was then calculated with the ordinary equations (von Caemmerer and Farquhar 1981), with the ratio of diffusivity between CO2 and D2O of 1.5 (instead of 1.6 for H2O) (Marrero and Mason 1973). It is noted that the diffusion coefficients of CO2 in (heavy) water vapour and in air are not equal (the H2O-to-D2O ratio of CO2 diffusivity is of around ~1.07), in contrast with the usual case (von Caemmerer and Farquhar 1981). However, the effect on the internal CO2 concentration (ci) is very small, typically of around 0.2–0.5 μmol mol–1, and it was neglected in the present study.