New opportunities for molecular photoswitches as wearable ultraviolet radiation dosimeters
Lauren E. Blackwell A , Sandra Wiedbrauk A B and Nathan R. B. Boase
A
B
![]() Lauren Blackwell completed undergraduate studies in chemistry and software engineering from the Queensland University of Technology in 2023. Since 2020, she has worked on a variety of organic synthesis and analysis projects under the supervision of Dr Nathan Boase. Specifically, she has an interest in organic small molecules for use in health and medical applications. |
![]() Sandra Wiedbrauk received her PhD in organic chemistry from Ludwig-Maximilians-Universität in Munich in 2018 under the supervision of Prof. Henry Dube. In 2018, she joined Queensland University of Technology as a postdoctoral fellow to work on advanced materials. Since 2021, she has been working in the research group of Kathryn E. Fairfull-Smith on anti-biofilm molecules. |
![]() Nathan Boase is a senior lecturer at the Queensland University of Technology, a member of the Centre for Materials Science, and a co-leader of the Medicinal Molecules and Materials Group. He completed his PhD at The University of Queensland in 2015. In 2019, he was recognised as a CAS Future Leader in chemistry and received an Australian Institute of Policy and Science (AIPS) Tall Poppy Science Award in 2023. His current research explores controlled synthetic strategies to design materials that can respond to their environment. These materials are applied to solve significant challenges in health care, such as antibiotic coatings, environmental sensing, nanomedicine and antiviral therapies. |
Abstract
Australians have the highest incidence of melanoma globally, despite increasing awareness of the risks of excessive sun exposure. Although excess ultraviolet radiation (UVR) can cause irreparable cell damage and lead to cancer, some exposure is vital to maintain bodily processes such as vitamin D production. For an individual, finding the balance between healthy exposure and skin damage is largely guesswork. The ability to provide a simple, individualised indicator of cumulative UVR dosage could be transformative in preventing skin cancer. This review will provide a brief overview of the variety of UVR sensor technologies and explain the important role of colourimetric dosimeters. The chemistry behind some recent examples of colourimetric dosimeters will be discussed, identifying that molecular photoswitches are ideal candidates to enable this technology. We discuss the chemical mechanisms of photoswitches and how to modify their chemical structure to optimise their properties for use as dosimeters. Through this lens, diarylethenes have been identified as prime dosimeter candidates, owing to their sensitivity, stability, adaptability and the variety of visually striking colours possible. Finally, some specific challenges are identified in the design and fabrication of personalised colourimetric dosimeters that can equitably meet the requirements of all users in our community.
Keywords: diarylethene, dosimeter, melanoma, photochrome, photoswitch, skin cancer, UV radiation, wearable.
Introduction
With 54 in every 100,000 Australians being diagnosed with melanoma each year, the motivation to develop new preventative healthcare technologies is clear.1 Overexposure to ultraviolet radiation (UVR), particularly in childhood, is known to increase an individual’s risk of skin cancer later in life.2 There is a well-established link between over-exposure to UVR and a range of skin and eye diseases.3 UVR is a high-frequency band of solar radiation that can be divided into UV-A (315–399 nm), UV-B (280–314 nm) and UV-C (100–279 nm) subcategories. Fig. 1 shows a typical summer solar spectrum (solid), and the actual risk from the solar spectrum (dashed).4 Although UV-C radiation is entirely filtered by the ozone in the earth’s atmosphere, UV-A and UV-B reach the earth’s surface in a ratio of ~20:1.5 UV-B has been directly implicated with direct cell and DNA damage and heightened cancer risks.5 The contribution of UV-A to cancer risk is not as clear, but it has been shown that UV-A is responsible for the generation of toxic reactive oxygen species which are synergistically mutagenic with UV-B.6 Despite the known risks, regular exposure to sunlight is vital, with critical bodily functions such as vitamin D production being stimulated by UV-B radiation.7,8 Furthermore, emerging research suggests that some UVR exposure can reduce risks for other diseases, including ovarian and prostate cancer, diabetes and hypertension.9 Evidently, purely advising patients to limit sun exposure to avoid melanoma is not satisfactory health advice.10 In Australia, where the risk from UVR exposure is high, despite sustained educational campaigns since the 1980s warning of the risk of sun exposure, the rates of melanoma remain extremely high.11,12 A clear method of monitoring and communicating UVR exposure to an individual may help to unlock the health benefits of sunlight while minimising the risk of skin cancer and damage.8
Comparison of the action spectrum and risk from UVR compared to the terrestrial solar spectrum (solid line, right axis, Brisbane, Australia, noon summer day).13 Risk profiles are presented as The International Commission on Non-Ionizing Radiation Protection (ICNIRP) action spectrum for the risk to human skin and eyes from UVR (dashed line, left axis) and the International Commission on Illumination (CIE) erythema reference action spectrum (dotted line, left axis).14,15
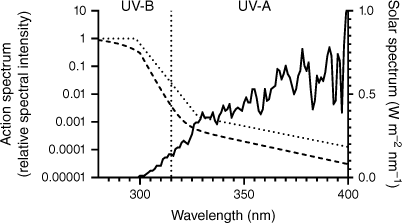
The need for personalised UVR sensor devices has been recognised by many groups, leading to production of a variety of different devices. Spanning a wide range of price, sensitivity and form, these sensors largely fall into two categories – electrical or unpowered.16 Unpowered devices change appearance, usually colour, with UVR exposure. Electrical devices emit a varying voltage or current, which relates to the irradiance of UVR exposure.17 In addition to their mechanism of detection, they can also be classified as exposure sensors or dosimeters. Exposure sensors are defined in this review as materials or devices that respond instantaneously and qualitatively to UVR, usually with a visual response when the sensor detects radiation at sufficient irradiance. They are often marketed as sunscreen reminders. The irradiance sensitivity varies between different devices and technologies. Dosimeters, however, respond to radiant exposure of UVR detected over time, with the aim of informing a user of their risk.18 This is a rapidly evolving field, and a selection of innovations for wearable sensors are detailed in Table 1, to provide a broad overview of different technologies on the market or in development, and readers are directed to more detailed overviews of technological development and testing of UVR sensor technologies.17,19 Each device is listed with an indicative price if available, as price is likely to be a significant barrier to end-user engagement.16 The difference in price between electronic and unpowered colourimetric sensors makes colourimetric sensors an attractive and economical solution.
The summary table above shows that there are no reusable colourimetric dosimeter devices currently available on the market, with only one prototype published.20 A reusable device is ideal to improve sustainability and will also result in a reduced long-term cost to the user. Additionally, the colour-changing devices have no power requirements for use and are compatible with a wide range of forms.17 Research into the use of an optical exposure sensor sticker, designed to provide a sunscreen reapplication reminder, shows that users are receptive and will change behaviours from a visual prompt.21 This suggests that a reusable optical dosimeter will likely have similar outcomes.
To enable the development of reusable colourimetric dosimeters, several key parameters for the chromophore system need to be defined and optimised (Fig. 2). First is the colourimetric response of the chromophore, with a significant visual contrast between the two states necessary to allow for easy reading of accumulated dose. A colourless to coloured change upon exposure to UVR is ideal for easiest interpretation. The sensitivity and specificity of the colourimetric system to varying radiant exposure of UVR, the solar spectrum at the given time and the action spectrum for erythema are key to allow for skin-relevant UVR doses to be detected. To develop a reusable device, the system must be reversible, allowing the colourimetric response to be reset. Ideally for a dosimeter, the colour response should be stable until a defined trigger mechanism is applied. This allows the user to choose when to reset the device, once they have finished acquiring their accumulated dose information. Finally, the overall stability of the colourimetric system must be high, to prevent degradation of the chromophores into non-responsive or discoloured states, making them inactive as a dosimeter. In this report, stability will refer to the tendency of the chromophore to resist degradation or spontaneous reactions with other species to form side products. The overall reusability of the system is evaluated as the combination of reversibility and stability allowing repeated switching with limited degradation of the products. This review paper will use the framework presented in Fig. 2 to evaluate recent reusable colourimetric UVR dosimeter technologies and to understand their strengths and weaknesses. It will also present a case that organic photoswitches are ideal chemical candidates for reusable dosimeters, and review the photoswitch literature to understand key structure–activity relationships to further improve this technology. Finally, key design parameters for wearable colourimetric devices including skin tone and fabrication will be discussed.
Chemistries for reusable colourimetric dosimeters
A wide variety of chemistries have been considered for use in colourimetric UVR dosimeters. Here we review some recent examples that have potential to be incorporated into reusable devices. Wide bandgap semiconductors have often been employed in both electronic and colourimetric UVR sensors.22 One recent example of a colourimetric device uses titania and hydrated tungsten oxide (THTO) hetero-nanoparticles to create a redox system that switches between colourless and blue forms.23 This mechanism is photo-reversible, and colouration occurs through the reduction of the THTO, initiated by UVR irradiation. Oxidation back to the colourless form occurs spontaneously in the dark under ambient conditions, and the system is stable for at least 60 reversal cycles.23 This technology has been proposed within a sticker design, although it could also be utilised in a more robust, reusable form. One key drawback of this system is its spontaneous decolouration. Although the colour progressively darkens as UVR exposure increases, it will slowly bleach back to its colourless form when removed from the sun. This means that its usefulness as a cumulative dosimeter over a full day, or multiple days, may be limited.
Another approach utilises a BaMgSiO4 ceramic that transitions from white to pink when irradiated with UVR.24 This occurs due to electron trapping and detrapping at oxygen vacancies within the substrate. As the dose of UVR irradiation increases, electrons are trapped by oxygen vacancies and the BaMgSiO4 darkens in colour (pink), making it an effective dosimeter. Interestingly this system was also found to respond to X-ray exposure, providing for more potential applications of this technology. Visible light or heat (~285°C) can then reverse the colouration through detrapping of the electrons. Although reversible, this system was found to maintain its colour when removed from UVR, with minimal bleaching observed over a 36-h period, overcoming the limitation of the THTO example above. Importantly the compound appears to be relatively stable over multiple cycles, and was able to be quantified either spectrophotometrically, or with simple digital photography.24 The BaMgSiO4 devices were produced as pressed ceramic pellets, and their ability to be developed into a variety of shapes and forms for wearable devices may be limited.
Organic materials offer great promise as UVR dosimeters, with full freedom of design of the devices. The earliest examples are polysulfone films, which rely on degradation of the polymer matrix leading to sample darkening.25 These have been used as occupational monitors for UVR exposure, but require spectrophotometric analysis for determination of dose, and are not reusable. Other elegant examples of organic-based colourimetric UVR sensors, readable by the naked eye, either function as exposure sensors or dosimeters that are not reusable.26–29 Our group recently developed the first fully organic, reusable, colourimetric dosimeters using diarylethene photoswitches as UVR-sensitive chromophores.20 These chromophores were shown to be highly sensitive to UVR and visually striking, providing quantitative estimations of dose, while simply being reset using low-powered green LEDs. The chromophores could easily be incorporated into a range of materials allowing for production of flexible silicone devices, or imbedding in 3-D printing resins for advanced manufacturing of any form. This new approach highlights the untapped potential of photoswitches for use in UVR dosimeters, but it requires a careful understanding of the photochemistry of these chromophores. In the next section, we discuss the chemistry of photoswitches and where they have been used in a range of UVR sensing technologies, highlighting opportunities to improve their use in both colourimetric UVR exposure sensors and dosimeters.
Untapped potential of photoswitches for colourimetric dosimeters
Photoswitches can change their chemical structure when irradiated with light, and transform back to their original state, either when excited with light or heat. Depending on the energy barrier between the isomers, photoswitches are classified as either T-type (thermal relaxation due to low energy barrier) or P-type (relaxation by light irradiation). These properties make them well-suited for usage in colourimetric UVR dosimeters. However, as discussed above, to function as efficient dosimeters photoswitches need a few key properties to be optimised: (1) intense colourimetric response when irradiated with UV-A and UV-B radiation; (2) increased sensitivity towards UV-B over UV-A radiation; (3) reversibility of colour that is only triggered after dose has been measured (stable during usage ~12–24 h); (4) high stability to heat and light to prevent degradation.
An additional challenge for using photoswitches in UVR-sensitive wearables is switching in the solid state (or a solid matrix), as photoswitches often have large volume changes due to structural isomerisation, e.g. azobenzene.30 This was a significant challenge in the development of organic photochromic lenses. In this context, it was shown that the kinetics of the isomerisation process of a range of photoswitches (T-type: spirooxazine, chromene, azobenzene) in a rigid polymer matrix could be increased by attaching flexible oligomers such as poly(dimethylsiloxane) to the photoswitch.31 Other photoswitches that have a small volume change during the photoswitching process such as cyclisation (e.g. diarylethene) are well suited for switching in the solid state, and therefore amenable to applications such as solid sun sensors.30,32
The most common classes of photoswitches are azobenzene, spirobenzopyran and diarylethene (DAE), but others have been investigated for a wide range of applications (Fig. 3).33 Azobenzene and spirobenzopyran are classified as T-type photoswitches, due to the high energy difference (large ΔGr,T) between the stable and metastable states, with a small energy barrier (small ΔG‡T) between both isomers (Fig. 3b). Furylfulgide and diarylethene molecules, however, switch to an isomer with a similar energy state (small ΔGr,P) with a higher energy barrier (large ΔG‡P), and therefore are more thermodynamically stable and are referred to as P-type photoswitches (Fig. 3b).33 However, most photoswitches can be structurally modified to modulate their photophysical and photochemical properties. DAEs, for example, can be converted into a T-type switch by addition of electron-withdrawing substituents on the aryl groups, or increasing steric bulk at the 3-thiophene position. These changes greatly decrease the thermal stability and can allow switching below 100°C with thermal half-lives in the order of minutes.34
Molecular photoswitches can be divided into two classes based on thermodynamics of isomerisation mechanisms. (a) Exemplary T-type and P-type photoswitch molecular structures, with the coloured state highlighted. (b) Qualitative potential energy diagram for the isomerisation of photoswitches, highlighting the key energy differences between metastable T-type (solid red) and stable P-type (dashed blue) photoswitches.
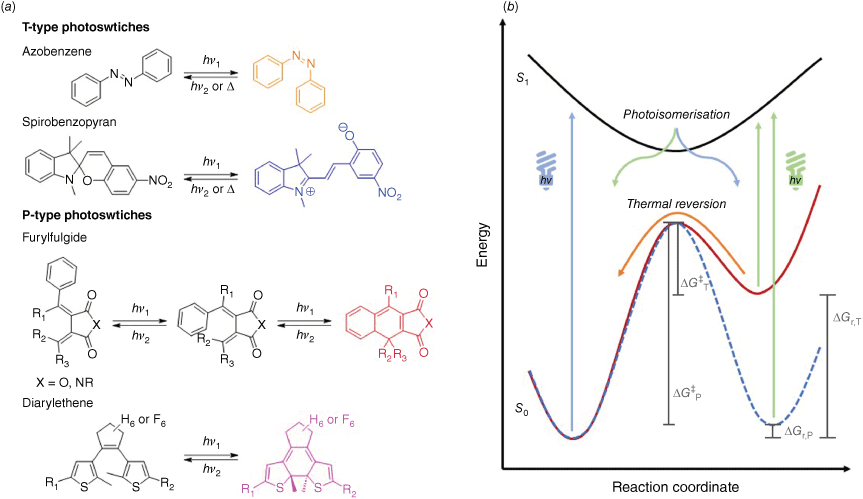
Spirobenzopyrans are thermally reversible T-type photoswitches. As seen in Fig. 3, these molecules ring-open rapidly under UVR, forming a coloured merocyanine molecule. At room temperature, once the radiation source is removed, they recyclise to the colourless form again.35 Spirobenzopyrans can natively switch in the solid state.30 Therefore, they have been used in the design of photochromic personal products, such as sunscreen reminders.36 Studies have shown that many spirobenzopyrans are unstable and prone to undergo side reactions leading to degradation and unusable products.35 Spirobenzopyrans are not suitable for use in a reusable sun dosimeter, as the device will spontaneously revert to the colourless form through thermal relaxation, and will not record dose across 1 day.35 Furthermore, their tendency to fatigue after multiple cycles makes these molecules better suited to single-use applications such as sunscreen reminder stickers (Table 1).
UVR sensor technology and devices | Appearance | Cost (A$) | Exposure sensors or dosimeter | Reusable | Electrical or unpowered | |
---|---|---|---|---|---|---|
Wide bandgap semiconductors | ||||||
Nanostructuring of wide bandgap semiconductors, such as GaN, ZnO, and SiC, leads to UVR photodetectors | ||||||
La Roche-Posay My Skin Track UVA | ![]() | $89 | Dosimeter | Yes | Electrical | |
QSun UV exposure tracker37,B | ![]() | $220 | Dosimeter | Yes | Electrical | |
Scienterra UV dosimeter38 | ![]() | US$300 | Dosimeter | Yes | Electrical | |
Scientific research only | ||||||
SunWatch39 | ![]() | Prototype (large user trial planned) | Dosimeter | Yes | Electrical | |
Redox couples | ||||||
A coloured substance is discoloured by oxidation processes, catalysed by a UV photosensitiser | ||||||
Redox colour-changing stickers | ![]() | Experimental | Dosimeter | No | Unpowered | |
Different sections of the sticker require different intensities of irradiation to change colour23,C | ||||||
Inorganic ceramics and nanoparticles | ||||||
Inorganic materials undergo modulation under UVR to change colour | ||||||
Colour-changing nanoparticle stickers40 | ![]() | Experimental | Dosimeter | No | Unpowered | |
Photochromic ceramic24,C | ![]() | Experimental | Dosimeter | Yes | Unpowered (requires heat or LED irradiation to reverse) | |
Photodegradation of organic molecules | ||||||
Light-sensitive molecules that undergo an irreversible colour change or photobleaching when exposed to UVR | ||||||
UVR dose wristband26,C | ![]() | Experimental | Dosimeter | No | Unpowered | |
Photoswitchable photochromes | ||||||
Molecules change structure when irradiated with UVR, leading to a colour change. Many different classes of photoswitch molecules exist, some reversible and others irreversible | ||||||
Sundicator UV | ![]() | $20 for 5 | Dosimeter | No (reversible but not reusable) | Unpowered | |
WristbandD | ||||||
Sundicator sunscreen reminder stickerD | ![]() | $20 for 10 | Exposure | No (reversible but not reusable) | Unpowered | |
Rayminder UV awareness braceletE | ![]() | $48 | Exposure | Yes | Unpowered | |
UV wear20 | ![]() | Prototype | Dosimeter | Yes | Unpowered (requires LED lightbox to reverse) |
The demands for (semi)quantitative UVR dosimeters require a colourimetric response that is stable during use. By adding a second, independent stimuli to trigger rapid reversion, these devices can become reusable products, rather than single use. Both furylfulgides and DAEs fit this category (Fig. 3). Furylfulgides are an interesting class of photoswitches, consisting of a 1,3,5-hexatriene structure with at least one aromatic ring.41 The open and colourless forms exist as either a Z- or E-isomer (Fig. 3). Under irradiation with UVR, it undergoes a Z–E isomerisation before a 6π-electrocyclisation to a coloured ring-closed state. The Z-isomer cannot cyclise directly towards the closed form, and the quantum yield of this reaction is low (>0.11), which is a significant disadvantage for dosimetric applications.42 The E and Z isomers of furylfulgides absorb strongly in the UVR region, with maximum absorption between 330 and 370 nm and relatively high photochemical quantum yield to the ring-closed state (φEC 0.17–0.53).42 Ring-opening can be achieved using visible light between 470 and 670 nm, with low photochemical quantum yields (φCE 0.09–0.16).42–44 Furylfulgides have also been demonstrated to photoswitch in the solid state.45 The photochemical properties, and solid state switching, makes furylfulgides an interesting class of molecule that have not yet been exploited as UVR dosimeters. Unfortunately, their synthesis can be challenging and expensive which makes them a less attractive candidate for commercial UVR dosimeters.46
DAEs are also based on a central 1,3,5-hexatriene framework with either phenyl or heterocyclic rings connected. Irie synthesised the first reversible T-type DAE in 1989, which changed from colourless to coloured when exposed to UVR.47 Substitution of the aryl rings with five-membered heterocycles (e.g. thiophene) can convert DAEs into P-type photoswitches.48 They undergo photocyclisation (Fig. 3) and this can only occur when in the antiparallel rotational state.49 The closed form of the DAE creates a conjugated system, shifting the absorbance wavelength to the visible light region and rendering it coloured.50,51 These molecules will not reopen until irradiated with visible light, and so are well suited to dosimetric applications.52 In addition, DAE photoswitches are extremely fatigue resistant, reported to be reversible more than 105 times in the solid state.32,48 As discussed previously, these are desirable properties for a UVR dosimeter (Fig. 2).
Another advantage of DAEs is that the colour of the ring-closed state can be altered by modifying the substituents on the rings as shown in Fig. 4.48,51 This is desirable for creating dosimeters with multiple sensor panels, or to cater for the user’s individual taste. In addition to changing colour, the substituents can also change the polarity and solvent interactions of the molecule.53 Depending on the solvent polarity, some DAEs possess solvatochromic behaviour. This is caused by the change in dipole moment occurring as the molecule cyclises.53 This solvatochromic behaviour may also effect the DAE in a solid matrix and must be considered when testing a molecule and designing a UVR dosimeter. The polarity and effect of the substrates in testing and manufacture must be comparable to yield useful, consistent results.
The visible colour of photoisomerised diarylethene photoswitches is highly dependent on their molecular structure and provides the potential to tune the colour of a UVR dosimeter to the desires of the end user. Reproduced from fig. 2a of Irie et al.,51 with permission, copyright 2014 American Chemical Society.
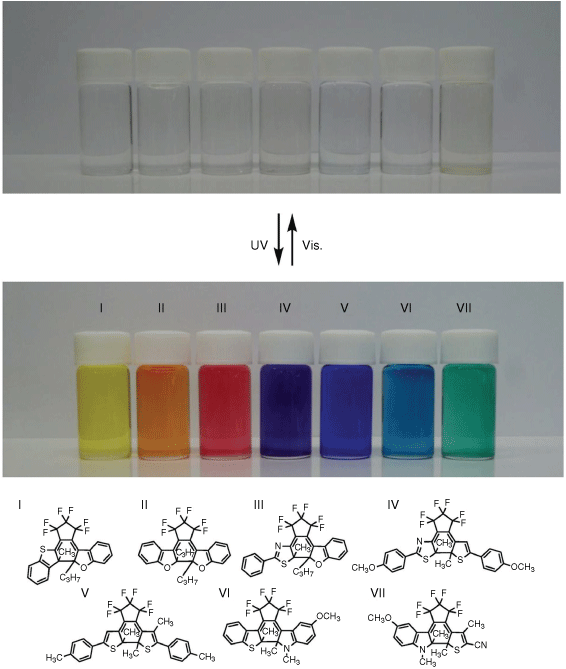
One of the major drawbacks of DAEs in practical use is their tendency to fatigue after multiple cycles, particularly in solution or under uncontrolled atmospheric conditions, as the molecule can degrade into undesirable side products.54 Fig. 5b shows the fatigue of a DAE photoswitch as measured by changes in absorbance over cycles of use. As the switching cycle is repeated, there is a gradual decrease in the population of switchable molecules, due to the formation of inactive by-products. It is hypothesised that this is caused by oxidation and elimination side reactions while in the closed state.55 Fig. 5b shows the proposed degradation mechanism for DAEs, where by-product C is unable to return to either A or B, the two active photoswitch forms. To create a truly reusable material, this side reaction must be minimised.
(a) Fatigue of a diarylethene photoswitch in an oxygenated solution of acetonitrile measured by UV-Vis spectroscopy over repeated cycles of photoisomerisation. (b) Mechanism of photoisomerisation and formation of inactive by-product from the ring-closed isomer. Part (a) reproduced from fig. 1c and (b) from scheme 1 of Herder et al.,55 with permission, copyright 2015 American Chemical Society.
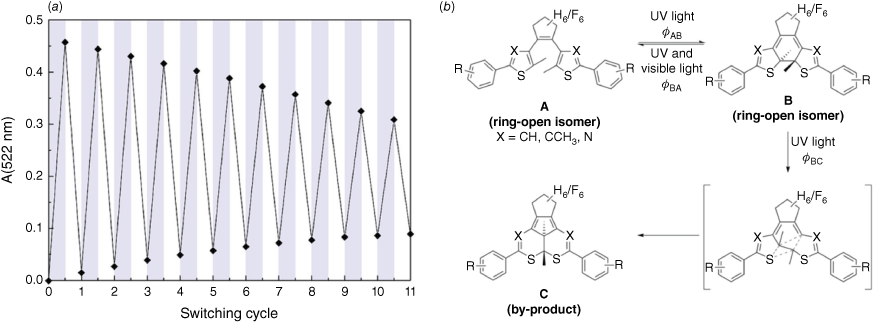
Studies have shown that using perfluorinated cyclopentene rather than the standard hydrogenated form can reduce the rate of fatigue in DAEs (Fig. 6).55 Molecules 1a–1h have a hydrogenated cyclopentene, and generally show very high levels of degradation. The exception to this is 1h, which has two trifluoromethyl substituents on each phenyl ring and shows very little by-product formation. Compounds 2c, 2h and 2i all have perfluorinated cyclopentene, and all show relatively low levels of degradation, further enhanced by CF3 or SF5 substituents. Compounds 3 and 4 replace the thiophene units with thiazole, and show poorer performance than the fluorinated molecules. This study demonstrates that addition of fluorine atoms can make DAEs more robust, which may improve reusability in a UVR dosimeter.55
(a) Introduction of fluorine atoms on the aryl ring and perfluorination of the cyclopentene ring can (b) decrease formation of inactive by-product, and improve the fatigue resistance of diarylethene photoswitches. Part (a) reproduced from scheme 3 and (b) from fig. 2 of Herder et al.,55 with permission, copyright 2015 American Chemical Society.
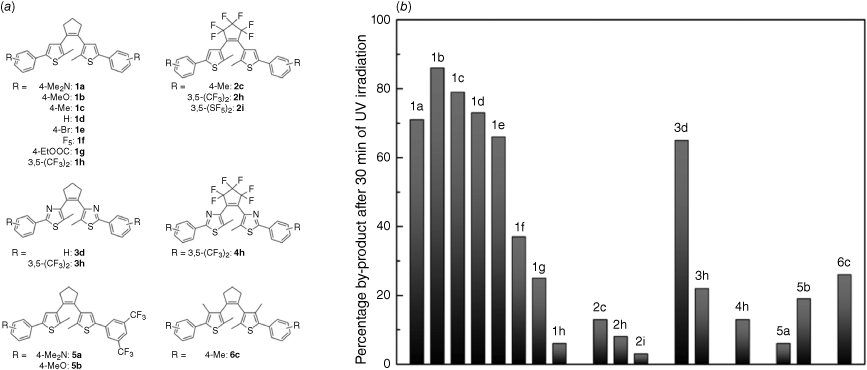
To be used as a UVR dosimeter, photoswitch behaviour should be activated by wavelengths within the UV-A to UV-B spectrum. Ideally the sensor will have a higher sensitivity to UV-B than UV-A, as UV-B has between 100 to more than 1000-fold higher risk for disease and erythema (Fig. 1). 1,2-Bis(2-methyl-5-phenyl-3-thienyl)perfluorocyclopentene has an absorption maximum of 280 nm within the UV-B range.56 Irradiation at this wavelength stimulates cyclisation most efficiently and was found to have a quantum yield of 0.59.56 The reverse process, ring-opening, was found to be catalysed by irradiation with visible light at wavelengths above 500 nm, with a quantum yield of 0.0013 at 492-nm irradiation.56 Owing to the favourable photophysical properties, 1,2-bis(2-methyl-5-phenyl-3-thienyl)perfluorocyclopentene was recently used to produce a reusable UVR dosimeter, with devices being reset by low-powered green LED light.20 Sunlight contains visible light, which can induce ring-opening, although ring-opening is ~64 times less efficient than cyclisation. Sunlight contains more visible light in the green region ~600 nm than UVR, however, the ratio is not sufficient to overcome the difference in quantum yield between cyclisation and ring-opening, and allows for these devices to be used as dosimeters under real sunlight conditions.20 The study demonstrated that the photoswitch could be easily integrated into a variety of polymer matrices, including 3D printing resins. This ease of fabrication allowed for a wide range of dosimeter forms to be used, to meet the desired needs of end users (Table 1).
Requirements and challenges of personalised UVR dosimeters
One major challenge in the development of a personal UVR sensor is that different skin types show different sensitivity to UV-A and UV-B radiation, as measured by their minimal erythema dose.17,57 The minimal erythemal dose is the exposure level at which the skin begins to burn. If a UVR sensor product is designed to provide health advice to the user, their individual skin type must be factored into the output reading or interpretation to provide equitable protection suitable for the whole population. Without this consideration, the readouts could under- or overestimate the health risks, potentially increasing risk of skin damage and cancer or discouraging adequate exposure for vitamin D production. In addition to skin type, skin is between 100 to more than 1000 times more sensitive to UV-B than UV-A (Fig. 1). This indicates that the sensitivity spectrum for a sensor must match the action spectrum for human skin erythema, or at least be equivalently weighted towards UV-B dosage or factor this ratio into its output. This sensitivity to UVR should be relatively temperature independent, within a standard environmental range, to ensure consistent measurements in any conditions. This is best achieved by the advantageous photoisomerisation mechanism of P-type photoswitches such as DAEs.58
Different approaches to tuning sensitivity for different skin tones have been presented in the literature.17,57 The use of filtering layers above the UVR sensitive layer has been commonly and successfully employed, but requires complicated multi-layered fabrication (Fig. 7).27 In addition, it can be important to tune the sensitivity between UV-A and UV-B radiation, to ensure the measured dose can accurately reflect the level of relative risk. In our recent work, we presented a new and simple method of tuning the response of the material to the UVR radiant exposure, by mixing in non-responsive UV-A absorbing molecules into the dosimeter matrix.20 It was found that inclusion of 7-diethylamino-4-methylcoumarin was able to tune the response of the DAE for maximum sensitivity for UV-B over UV-A radiation. While this approach hasn’t yet been demonstrated to make skin tone specific devices or tailor their specific action spectrum for erythema, the mechanism should allow for one or more filtering molecules to be integrated into a device to improve UVR specificity.
Tuning the UVR sensitivity of spirobenzopyran UVR exposure sensors to provide skin tone specific warnings, by applying a top UVR filtering layer with increasing weight ratio of UVR absorptive material. The colourimetric materials and the thickness of the filtering layers are the same in all examples. Scale bar: 1 cm. Reproduced from fig. 4 of Yang et al.,27 with permission.
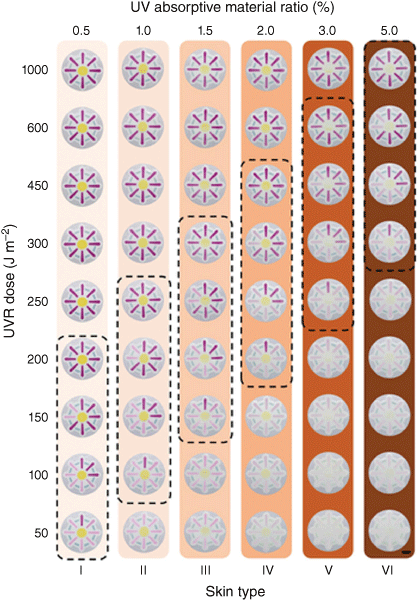
To maximise the effect of UVR dosimeters on the community, they must be cost effective and desirable to the individual end-user. Despite the wide availability of a variety of UVR sensors, there remains behavioural challenges in how effectively end users engage with the devices and sun protection strategies broadly.11,16,21 The design and fabrication of devices to ensure pleasing aesthetics, personal customisation and compatibility with personal identity, are real opportunities to improve engagement with wearable health technologies.59,60 The advantage of using photoswitches is they can be integrated into nearly any material and fabrication process, similar to any other organic pigment or chromophore.61,62 Careful material design, and attachment of photochromes to polymers, can even enhance their photoswitching properties in solid devices.31 These advantages enable the use of modern additive manufacturing techniques to be employed to create UVR dosimeters of nearly infinite shape and form.20 Interestingly, the use of 3D printing also provides an avenue of collaboration across disciplines to address the multifaceted issue of sun protection. It is clear based on the challenges detailed above, that a purely scientific approach cannot solve this challenge alone. Our work has found that 3D printing of UVR sensitive materials can act as a boundary between the fields of chemistry and design, by successfully mediating and embodying each discipline’s contributions to this project.63 This interdisciplinary approach is enabling research into the photochemistry of DAE chromophores to emerge from the laboratory, and begin to have an effect on the communities it aims to protect. Finally, quantification and long-term tracking of dosimetric information may be useful for users. Quantitative photographic analysis can enable this approach in colourimetric dosimeters, and integration into smartphone applications could help simplify provision of the information to users.20,64
Conclusions
The risks of UVR from sunshine continues to be a major risk factor for Australians, as seen in our world leading rates of skin cancer. In other regions of the world, vitamin D deficiency due to a lack of sun exposure can be a challenge. Cheap, simple and reliable UVR dosimeters may be a solution to allow people to make informed decisions about their level of sun exposure and risk. Colourimetric dosimeters that can be easily read by the naked eye and simply integrate into people’s everyday lives, offer a unique opportunity to inform the broader public of their UVR exposure. Molecular photoswitches, and in particular diarylethenes, offer reliable, sensitive and highly versatile photoswitching that is uniquely suited to the demands of UVR dosimeters. Their amenability to current and future manufacturing technologies provides flexibility of design, allowing for the creation of fashionable and highly desirable products, that will engage all community members in sun safe behaviours. To achieve a truly transformative effect, challenges remain in understanding how people interact with these devices and what they learn from them. Ensuring that the sensitivity of devices match the action spectra for both erythema and overall risk, and can match the risk for all skin tones, is critical for their accurate and safe usage. Combining this with a better understanding of behavioural and cultural attitudes towards the dosimeters and sun protection strategies, will ensure equitable protection among all members of our community.
Data availability
Data sharing is not applicable as no new data were generated or analysed during this study.
Declaration of funding
This work was funded by an Early Career Researcher Scheme team grant (2021) and the Boost Scheme (2020) to Dr Nathan Boase, both generously provided by Queensland University of Technology.
Acknowledgements
The authors acknowledge the ongoing support from the School of Chemistry and Physics and the Centre for Material Science at Queensland University of Technology.
References
1 Australian Institute of Health and Welfare, Cancer data in Australia. Catalogue number CAN 122. Canberra, ACT, Australia: Australian Government; 2023. Available at https://www.aihw.gov.au/reports/cancer/cancer-data-in-australia
2 Volkmer B, Greinert R. UV and children’s skin. Prog Biophys Mol Biol 2011; 107: 386-388.
| Crossref | Google Scholar | PubMed |
3 D’Orazio J, Jarrett S, Amaro-Ortiz A, Scott T. UV radiation and the skin. Int J Mol Sci 2013; 14: 12222-12248.
| Crossref | Google Scholar | PubMed |
4 Huang X, Chalmers AN. Review of wearable and portable sensors for monitoring personal solar UV exposure. Ann Biomed Eng 2021; 49: 964-978.
| Crossref | Google Scholar | PubMed |
5 Ichihashi M, Ueda M, Budiyanto A, Bito T, Oka M, Fukunaga M, et al. UV-induced skin damage. Toxicology 2003; 189: 21-39.
| Crossref | Google Scholar | PubMed |
6 McAdam E, Brem R, Karran P. Oxidative stress-induced protein damage inhibits DNA repair and determines mutation risk and therapeutic efficacy. Mol Cancer Res 2016; 14: 612-622.
| Crossref | Google Scholar | PubMed |
7 Jablonski NG, Chaplin G. The roles of vitamin D and cutaneous vitamin D production in human evolution and health. Int J Paleopathol 2018; 23: 54-59.
| Crossref | Google Scholar | PubMed |
8 Cherrie JW, Cherrie MPC. Workplace exposure to UV radiation and strategies to minimize cancer risk. Br Med Bull 2022; 144: 45-56.
| Crossref | Google Scholar | PubMed |
9 van der Rhee HJ, de Vries E, Coebergh JW. Regular sun exposure benefits health. Med Hypotheses 2016; 97: 34-37.
| Crossref | Google Scholar | PubMed |
10 Alfredsson L, Armstrong BK, Butterfield DA, Chowdhury R, de Gruijl FR, Feelisch M, et al. Insufficient sun exposure has become a real public health problem. Int J Environ Res Public Health 2020; 17: 5014.
| Crossref | Google Scholar | PubMed |
11 Morton SK, Harrison SL. Slip, slop, slap, slide, seek and sport: a systematic scoping review of sun protection in sport in Australasia. Curr Oncol 2023; 30: 401-415.
| Crossref | Google Scholar | PubMed |
12 Insight Economics. State of the Nation. A Report into Melanoma – A National Health Priority; Melbourne, Vic., Australia: Insight Economics; 2022. https://www.insighteconomics.com.au/?pgid=jhhk4hfh-256ba9f0-14f7-45e9-8b61-4b6a386b5c1a
13 Diffey B. Solar spectral irradiance and summary outputs using Excel. Photochem Photobiol 2015; 91: 553-557.
| Crossref | Google Scholar | PubMed |
15 The International Commission on Non-Ionizing Radiation Protection. Guidelines on limits of exposure to ultraviolet radiation of wavelengths between 180 nm and 400 nm (incoherent optical radiation). Health Phys 2004; 87: 171-186.
| Crossref | Google Scholar | PubMed |
16 Henning A, J. Downs N, Vanos JK. Wearable ultraviolet radiation sensors for research and personal use. Int J Biometeorol 2022; 66: 627-640.
| Crossref | Google Scholar | PubMed |
17 Zou W, Sastry M, Gooding JJ, Ramanathan R, Bansal V. Recent advances and a roadmap to wearable UV sensor technologies. Adv Mat Technol 2020; 5: 1901036.
| Crossref | Google Scholar |
18 Zou W, González A, Jampaiah D, Ramanathan R, Taha M, Walia S, et al. Skin color-specific and spectrally selective naked-eye dosimetry of UVA, B and C radiations. Nat Commun 2018; 9: 3743.
| Crossref | Google Scholar | PubMed |
19 Banerjee S, Hoch EG, Kaplan PD, Dumont ELP. A comparative study of wearable ultraviolet radiometers. In: 2017 IEEE Life Sciences Conference (LSC); 13–15 December 2017; Sydney, NSW, Australia. IEEE; 2017. pp. 9–12. 10.1109/LSC.2017.8268131
20 Wiedbrauk S, McKinnon H, Swann L, Boase NRB. Diarylethene photoswitches and 3D printing to fabricate rewearable colorimetric UV sensors for sun protection. Adv Mat Technol 2023; 8: 2201918.
| Crossref | Google Scholar |
21 Hacker E, Horsham C, Ford H, Hartel G, Olsen CM, Pandeya N, et al. UV detection stickers can assist people to reapply sunscreen. Prev Med 2019; 124: 67-74.
| Crossref | Google Scholar | PubMed |
22 Nasiri N, Jin D, Tricoli A. Nanoarchitechtonics of visible–blind ultraviolet photodetector materials: critical features and nano-microfabrication. Adv Opt Mat 2019; 7: 1800580.
| Crossref | Google Scholar |
23 Zhao J, Zhang Y, Liu F, Yan M, Wang X, Wang W. Sunlight-responsive titania-hydrated tungsten oxide heteronanoparticles/paper-based color-switching film for solar ultraviolet radiation monitors. ACS App Nano Mat 2022; 5: 4009-4017.
| Crossref | Google Scholar |
24 Yang Z, Hu J, Van der Heggen D, Jiao M, Feng A, Vrielinck H, et al. A versatile photochromic dosimeter enabling detection of X-ray, ultraviolet, and visible photons. Laser Photonics Rev 2023; 17: 2200809.
| Crossref | Google Scholar |
25 Diffey B. The early days of personal solar ultraviolet dosimetry. Atmosphere 2020; 11: 125.
| Crossref | Google Scholar |
26 Wang J, Jeevarathinam AS, Jhunjhunwala A, Ren H, Lemaster J, Luo Y, et al. A wearable colorimetric dosimeter to monitor sunlight exposure. Adv Mat Technol 2018; 3: 1800037.
| Crossref | Google Scholar | PubMed |
27 Yang Z, Zhao J, Liang C, Jiang H. Materials and device design for epidermal UV sensors with real-time, skin-color specific, and naked-eye quasi-quantitative monitoring capabilities. Adv Mat Technol 2023; 8: 2201481.
| Crossref | Google Scholar |
28 Lee ME, Armani AM. Flexible UV exposure sensor based on UV responsive polymer. ACS Sens 2016; 1: 1251-1255.
| Crossref | Google Scholar |
29 Khiabani PS, Soeriyadi AH, Nam EV, Peterson JR, Webb JEA, Thordarson P, et al. Understanding the performance of a paper-based UV exposure sensor: the photodegradation mechanism of brilliant blue FCF in the presence of TiO2 photocatalysts in both the solid state and solution. Rapid Commun Mass Spectrom 2019; 33: 1076-1083.
| Crossref | Google Scholar | PubMed |
30 Gonzalez A, Kengmana ES, Fonseca MV, Han GGD. Solid-state photoswitching molecules: structural design for isomerization in condensed phase. Mat Today Adv 2020; 6: 100058.
| Crossref | Google Scholar |
31 Evans RA, Hanley TL, Skidmore MA, Davis TP, Such GK, Yee LH, et al. The generic enhancement of photochromic dye switching speeds in a rigid polymer matrix. Nat Mater 2005; 4: 249-253.
| Crossref | Google Scholar | PubMed |
32 Fukaminato T, Kobatake S, Kawai T, Irie M. Three-dimensional erasable optical memory using a photochromic diarylethene single crystal as the recording medium. Proc Jpn Acad – B Phys Biol Sci 2001; 77: 30-35.
| Crossref | Google Scholar |
34 Kitagawa D, Sasaki K, Kobatake S. Correlation between steric substituent constants and thermal cycloreversion reactivity of diarylethene closed-ring isomers. Bull Chem Soc Jpn 2011; 84: 141-147.
| Crossref | Google Scholar |
35 Tomasulo M, Sortino S, White AJ, Raymo FM. Fast and stable photochromic oxazines. J Org Chem 2005; 70: 8180-8189.
| Crossref | Google Scholar | PubMed |
37 Heydenreich J, Wulf HC. Miniature personal electronic UVR dosimeter with erythema response and time-stamped readings in a wristwatch. Photochem Photobiol 2005; 81: 1138-1144.
| Crossref | Google Scholar | PubMed |
38 Grandahl K, Mortensen OS, Sherman DZ, Køster B, Lund P-A, Ibler KS, et al. Solar UV exposure among outdoor workers in Denmark measured with personal UV-B dosimeters: technical and practical feasibility. Biomed Eng Online 2017; 16: 119.
| Crossref | Google Scholar | PubMed |
40 Francisco-Aldana L, Morales-Narváez E. Plasmonic colored nanopaper: a potential preventive healthcare tool against threats emerging from uncontrolled UV exposure. J Phys Photonics 2019; 1: 04LT01.
| Crossref | Google Scholar |
41 Yokoyama Y. Fulgides for memories and switches. Chem Rev 2000; 100: 1717-1740.
| Crossref | Google Scholar | PubMed |
42 Strübe F, Siewertsen R, Sönnichsen FD, Renth F, Temps F, Mattay J. Photochromism of rotation-hindered furylfulgides influenced by steric modifications. Eur J Org Chem 2011; 2011: 1947-1955.
| Crossref | Google Scholar |
43 Siewertsen R, Strübe F, Mattay J, Renth F, Temps F. Tuning of switching properties and excited-state dynamics of fulgides by structural modifications. Phys Chem Chem Phys 2011; 13: 3800-3808.
| Crossref | Google Scholar | PubMed |
44 Yokoyama Y, Tanaka T, Yamane T, Kurita Y. Synthesis and photochromic behavior of 5-substituted indolylfulgides. Chem Lett 1991; 20: 1125-1128.
| Crossref | Google Scholar |
45 Du Y, Huang C-R, Xu Z-K, Hu W, Li P-F, Xiong R-G, et al. Photochromic single-component organic fulgide ferroelectric with photo-triggered polarization response. JACS Au 2023; 3: 1464-1471.
| Crossref | Google Scholar | PubMed |
46 Thomas CJ, Wolak MA, Birge RR, Lees WJ. Improved synthesis of indolyl fulgides. J Org Chem 2001; 66: 1914-1918.
| Crossref | Google Scholar | PubMed |
47 Irie M. Advances in photochromic materials for optical data storage media. Jpn J Appl Phys 1989; 28: 215.
| Crossref | Google Scholar |
48 Irie M. Photochromism of diarylethene molecules and crystals. Proc Jpn Acad – B Phys Biol Sci 2010; 86: 472-483.
| Crossref | Google Scholar | PubMed |
49 Higashiguchi K, Matsuda K, Asano Y, Murakami A, Nakamura S, Irie M. Photochromism of dithienylethenes containing fluorinated thiophene rings. Eur J Org Chem 2005; 2005: 91-97.
| Crossref | Google Scholar |
50 Thomas Bens A, Frewert D, Kodatis K, Kryschi C, Martin H-D, Trommsdorff HP. Coupling of chromophores: carotenoids and photoactive diarylethenes – photoreactivity versus radiationless deactivation. Eur J Org Chem 1998; 1998: 2333-2338.
| Crossref | Google Scholar |
51 Irie M, Fukaminato T, Matsuda K, Kobatake S. Photochromism of diarylethene molecules and crystals: memories, switches, and actuators. Chem Rev 2014; 114: 12174-12277.
| Crossref | Google Scholar | PubMed |
52 Nakamura S, Irie M. Thermally irreversible photochromic systems. A theoretical study. J Org Chem 1988; 53: 6136-6138.
| Crossref | Google Scholar |
53 Kobatake S, Terakawa Y, Imagawa H. Solvent effect on photochromism of a dithienylperfluorocyclopentene having diethylamino group. Tetrahedron 2009; 65: 6104-6108.
| Crossref | Google Scholar |
54 Fredrich S, Göstl R, Herder M, Grubert L, Hecht S. Switching diarylethenes reliably in both directions with visible light. Angew Chem Int Ed Engl 2016; 55: 1208-1212.
| Crossref | Google Scholar | PubMed |
55 Herder M, Schmidt BM, Grubert L, Pätzel M, Schwarz J, Hecht S. Improving the Fatigue Resistance of Diarylethene Switches. J Am Chem Soc 2015; 137: 2738-2747.
| Crossref | Google Scholar | PubMed |
56 Irie M, Lifka T, Kobatake S, Kato N. Photochromism of 1,2-bis(2-methyl-5-phenyl-3-thienyl)perfluorocyclopentene in a single-crystalline phase. J Am Chem Soc 2000; 122: 4871.
| Crossref | Google Scholar |
57 Fitzpatrick TB. The Validity and practicality of sun-reactive skin types I through VI. Arch Dermatol 1988; 124: 869-871.
| Crossref | Google Scholar | PubMed |
58 Fukaminato T. Single-molecule fluorescence photoswitching: design and synthesis of photoswitchable fluorescent molecules. J Photochem Photobiol C 2011; 12: 177-208.
| Crossref | Google Scholar |
60 Pateman M, Harrison D, Marshall P, Cecchinato ME. The role of aesthetics and design: wearables in situ association for computing machinery. In: CHI EA ’18: Extended Abstracts of the 2018 CHI Conference on Human Factors in Computing Systems; Montreal, QC, Canada. New York, NY, USA: Association for Computing Machinery; 2018. Paper LBW518. 10.1145/3170427.3188556
61 Goulet-Hanssens A, Eisenreich F, Hecht S. Enlightening materials with photoswitches. Adv Mat 2020; 32: 1905966.
| Crossref | Google Scholar | PubMed |
62 Russew M-M, Hecht S. Photoswitches: from molecules to materials. Adv Mat 2010; 22: 3348-3360.
| Crossref | Google Scholar | PubMed |
63 Swann L, McKinnon H, Boase NRB, Mirzaei M, Wiedbrauk S, Wigman S. Beyond boundaries: 3D printing and functional materials as boundary objects to mediate interdisciplinary collaboration. In: Ferraris S, Rognoli V, Nimkulrat N, editors. Proceedings of the International Conference 2023 of the Design Research Society Special Interest Group on Experiential Knowledge (EKSIG): from abstractness to concreteness – experiential knowledge and the role of prototypes in design research; 19–20 June 2023; Milan, Italy. Milan, Italy: Politecnico di MIlano; 2023. pp. 587–604. Available at https://eprints.qut.edu.au/243827/1/147117344.pdf
64 Shi Y, Manco M, Moyal D, Huppert G, Araki H, Banks A, et al. Soft, stretchable, epidermal sensor with integrated electronics and photochemistry for measuring personal UV exposures. PLoS ONE 2018; 13: e0190233.
| Crossref | Google Scholar | PubMed |
![]() Lauren Blackwell completed undergraduate studies in chemistry and software engineering from the Queensland University of Technology in 2023. Since 2020, she has worked on a variety of organic synthesis and analysis projects under the supervision of Dr Nathan Boase. Specifically, she has an interest in organic small molecules for use in health and medical applications. |
![]() Sandra Wiedbrauk received her PhD in organic chemistry from Ludwig-Maximilians-Universität in Munich in 2018 under the supervision of Prof. Henry Dube. In 2018, she joined Queensland University of Technology as a postdoctoral fellow to work on advanced materials. Since 2021, she has been working in the research group of Kathryn E. Fairfull-Smith on anti-biofilm molecules. |
![]() Nathan Boase is a senior lecturer at the Queensland University of Technology, a member of the Centre for Materials Science, and a co-leader of the Medicinal Molecules and Materials Group. He completed his PhD at The University of Queensland in 2015. In 2019, he was recognised as a CAS Future Leader in chemistry and received an Australian Institute of Policy and Science (AIPS) Tall Poppy Science Award in 2023. His current research explores controlled synthetic strategies to design materials that can respond to their environment. These materials are applied to solve significant challenges in health care, such as antibiotic coatings, environmental sensing, nanomedicine and antiviral therapies. |