Hippo signaling in cancer: regulatory mechanisms and therapeutic strategies
Zhao Huang

A Department of Biotherapy, Cancer Center and State Key Laboratory of Biotherapy, West China Hospital, Sichuan University, Chengdu, 610041, China.
B West China Hospital of Stomatology, Sichuan University, Chengdu, 610041, China.
C Mental Health Center and Psychiatric Laboratory, The State Key Laboratory of Biotherapy, West China Hospital, Sichuan University, Chengdu, 610041, China.
D West China Biomedical Big Data Center, West China Hospital, Sichuan University, Chengdu, 610041, China.
E Medical Big Data Center, Sichuan University, Chengdu, 610041, China.
F Sleep Medicine Center, Department of Respiratory and Critical Care Medicine, Mental Health Center, Translational Neuroscience Center, and State Key Laboratory of Biotherapy, West China Hospital, Sichuan University, Chengdu, China.
G Department of Biochemistry and Molecular Biology, Monash University, Clayton, Vic., Australia.
![]() Zhao Huang is currently postdoctor in Department of Biotherapy, Cancer Center and State Key Laboratory of Biotherapy, West China Hospital, Sichuan University. His research focuses on the tumor biology including the growth, metastasis and drug resistance of cancers. |
![]() Yunhan Tan is currently an undergraduate student in West China School of Stomatology, Sichuan University. Her research focuses on the application of nanotechnology and immune therapy for head and neck squamous cell carcinomas. |
![]() Prof. Wei Zhang graduated from the Clinical School of Medicine of West China University of Medical Sciences in 1985 and obtained a master’s degree in medicine in 1992. He has been working in West China Hospital since then. He is currently deputy secretary of the Party Committee of Sichuan University, the chief scientist of the Medical Big Data Center of Sichuan University and the director of the Biomedical Big Data Center of West China Hospital. He has successively undertaken over 20 projects including National 863 Program, National 973 Program, the 10th Five-Year Plan, the 11th Five-Year Plan, the 12th Five-Year Plan, the 13th Five-Year Plan, and National Natural Science Foundation projects. He has been awarded the First Prize of Natural Science by the Ministry of Education and the First Prize of Scientific and Technological Progress by Sichuan Province, among other awards. Under his leadership, the team has established a comprehensive biological database of depression and anxiety disorders in the Chinese population. His team is interested in providing a basis for early prevention, early diagnosis, and early personalized treatment of mental disorders. |
![]() Prof. Xiangdong Tang is the chief physician of Mental Health Center, West China Hospital, Sichuan University. He has been engaged in the basic and clinical research of sleep disorders for a long time, and is good at the diagnosis and treatment of common sleep problems such as insomnia, dreaminess and rhythm disorder, as well as sleep disorders related to breathing. He is the academic and technical leader in Sichuan Province, vice chairman of the Sleep Medicine Expert Committee of the Chinese Medical Doctor Association, deputy director of the Sleep and Mental Health Professional Committee of the Chinese Sleep Research Association, and deputy director of the Sleep Disorders Professional Committee. He has been responsible for and participated in 7 scientific research projects, and has published over 100 SCI papers. |
![]() Prof. Ed Nice obtained his Licentiate from the Royal Institute of Chemistry in London in Advanced Analytical Chemistry in 1972 and Fellowship of the Chemical Society London in 1973. He is currently Adjunct Professor at Monash University where he is Head of the Clinical Biomarker Discovery and Validation (Department of Biochemistry and Molecular Biology) and a scientific advisor to the Monash Antibody Technologies Facility (MATF), of which he was Director from 2009â2013. He holds a Visiting Professorship at Sichuan University s West China Hospital and an Adjunct position at Macquarie University. Ed s long-term research interests have been in biomarker discovery and validation, high throughput monoclonal antibody production and validation and clinical biomarker assay development, with a strong translational focus on colorectal cancer. |
![]() Prof. Canhua Huang returned to China in 2005 and was appointed as a Professor of the State Key Lab of Biotherapy, West China Hospital, Sichuan University. In 2012, he won the National Science Fund for Distinguished Young Scholars of China, and took up the post of the Chief Scientist for National 973 Program entitled âProteomics Profiling of the Redoxomes Associated with Virus-induced Carcinogenesisâ from 2013 to 2017. In 2014, he was hired as the Changjiang Scholars Program Endowed Professor. In 2018, he served as the director of an Innovative Research Group of the National Nature Science Foundation of China, entitled âRedox Signaling Regulation and Carcinogenesisâ. In 2020, he was a member of the 8th Discipline Evaluation Group of Academic Degrees Committee of The State Council (Basic Medicine Group). He was selected by Elsevier 2020 and 2021 China Highly Cited Scholar and won the First Prize of Natural Science Award of the Ministry of Education in 2020. |
Handling Editor: Mibel Aguilar
Australian Journal of Chemistry 76(8) 399-412 https://doi.org/10.1071/CH22241
Submitted: 21 November 2022 Accepted: 1 June 2023 Published: 7 July 2023
© 2023 The Author(s) (or their employer(s)). Published by CSIRO Publishing. This is an open access article distributed under the Creative Commons Attribution-NonCommercial-NoDerivatives 4.0 International License (CC BY-NC-ND)
Abstract
As an evolutionarily conserved pathway, Hippo signaling not only plays a key role in embryonic development, but also regulates the initiation and progression of cancer. The upstream factors regulating the Hippo pathway are complex, including cell–cell contact, cell–extracellular matrix contact, membrane receptor–ligand binding, and cytoskeletal tension. In response to these mechanical or soluble cues, the Hippo core kinases are activated or inactivated, regulating the activity of key transcription co-factor YAP/TAZ thus yielding biological consequences. In the context of neoplasm, dysregulation of Hippo signaling contributes to cancer hallmarks such as sustained proliferation, stem-like properties, and metastasis. Importantly, targeting Hippo signaling by chemicals is emerging as a promising anticancer strategy. This article briefly introduces the discovery process of the Hippo pathway, summarizes the upstream signals regulating the Hippo pathway, discusses the relationship between Hippo inactivation and cancer development, and highlights the potential use of chemicals targeting Hippo signaling in cancer treatment.
Keywords: cancer hallmarks, chemical inhibitors, embryonic development, Hippo signaling, oncogene, targeted therapy, tumor suppressor, YAP/TAZ.
Introduction
Tumor is a major threat worldwide, having a major impact on human health. However, further studies are urgently required to unravel the underlying biology. Scientists in different fields tend to interpret this problem from diverse perspectives, but fortunately these perspectives are highly interconnected. Thus, for example, geneticists usually explain the origin of tumors in terms of genetic mutations. However, in 1978, developmental biologist Beatrice Mintz pointed out that tumors arise from faulty developmental processes.[1] Similar to embryonic development, the tumorigenic ability of malignant cells is inhibited.[2] This evidence indicates that there is a tight correlation between embryonic development and tumorigenesis. Many hallmarks in cancer, such as cell proliferation, apoptosis, differentiation, and migration are also key features in embryonic or organ development, suggesting tumors are partly a problem in the field of developmental biology. Therefore, abnormal regulation of embryonic development-related pathways is an important cause of tumor initiation and progression. These signaling pathways include Wnt, Hedgehog, Notch, TGF-β, etc., which are highly conserved in evolution.[3–6] During embryonic development, these signaling pathways are activated and precisely regulated spatiotemporally. When the development process is completed, the corresponding pathways should revert to quiescence in order to keep the organs in their normal shape and size, which is the prerequisite for the organism to carry out its normal life function. In contrast, abnormal reactivation of these pathways in a well-developed organ often induces tumorigenesis, while underlying mechanisms are poorly understood.
Hippo is one of the key pathways in development, notably in organ size control.[7] Unlike other developmental pathways, activation of Hippo usually reflects the end of the developmental process, while inactivation of Hippo induces developmental and tumor-related phenotypes such as cell proliferation and migration. Therefore, Hippo is generally considered to be a tumor suppressive pathway.[8] The core of the Hippo pathway consists of a kinase cascade and downstream transcriptional/co-transcriptional factors. Briefly, when Hippo is activated, MST1/2 can be autophosphorylated or phosphorylated by TAO kinase; phosphorylated MST1/2 induce the phosphorylation of LATS1/2 by forming a complex with SAV1 and MOB1. Phosphorylated LATS1/2 further induce phosphorylation of the co-transcription factor YAP/TAZ, leading to the cytoplasmic retention or degradation of YAP/TAZ.[9] When the Hippo pathway is inhibited, the stability of the YAP/TAZ protein is enhanced, enabling YAP/TAZ translocation. YAP/TAZ is a well-known oncogene, which binds to transcription factor TEAD1–4 thus promoting tumorigenesis.[10] For example, clinicopathological analysis showed that inactivation of the Hippo signaling pathway was associated with poor prognosis in liver cancer patients.[11] Animal and cellular models also confirmed that the inactivation of the Hippo pathway can induce the initiation and progression of liver cancer both in vitro and in vivo.[12,13] In addition to liver cancer, the tumor suppressive role of Hippo signaling has been verified in a variety of tumors.[14]
The upstream signals controlling the Hippo pathway are extremely complex and can be roughly divided into mechanical factors and soluble signaling molecular factors. Specifically, it includes cell–cell contact, cell–extracellular matrix contact, membrane receptor–ligand binding, cytoskeleton tension, and liquid shear stress.[15–19] Under pathological conditions, these factors may be abnormally regulated, which inactivates the Hippo pathway, contributing to a series of tumor-related features such as aerobic glycolysis, persistent growth, metastasis, acquisition of tumor stem cell characteristics, and drug resistance.[20–23] In addition, the Hippo pathway can also interact with other signaling pathways (including the estrogen receptor, and IRS2/AKT and PI3K-TOR pathways) to form a complex signaling network, presenting a greater challenge for deciphering the cancer related mechanisms.[24–26]
Given the intrinsic link between the Hippo pathway and tumors, targeting the Hippo pathway is expected to be a promising anticancer strategy. Importantly, several chemical drugs have been shown to have potential therapeutic value by targeting components of the Hippo pathway. For example, verteporfin disrupts YAP–TEAD interactions and inhibits retinoblastoma.[27] This article will focus on the regulatory mechanisms of the Hippo pathway in the context of neoplasm, with a highlight on Hippo-targeting chemicals in order to provide new insights for cancer treatment.
A brief history of Hippo signaling
Organs maintain their normal size and shape in response to various stimuli, and this is an important prerequisite for their physiological functions. For example, it has long been found that in patients undergoing liver resection, the rest of the liver rapidly grows back to its original size and then automatically stops growing, indicating that organ size is finely regulated.[28] However, the molecular mechanisms continue to be unclear. In 1995, researchers found that homozygous deletion of the Warts gene in Drosophila resulted in tissue overgrowth and suggested that this event might contribute to tumor formation.[29] In 1999, researchers knocked out LATS1 (Warts homologous gene) in mice, and found that the knockout could form soft tissue sarcomas and ovarian cancer, confirming the tumor suppressive function of LATS1.[30] To date, as core components of the Hippo pathway, LATS1/2 have been extensively studied for their roles in tumor formation.[31] Other core components of the pathway, MST1/2, the mammalian ortholog of the Drosophila Hippo gene, were identified by multiple groups in 2003. These studies revealed that MST1/2 have similar functions to LATS1/2 in inhibiting tissue overgrowth.[32,33] In 2005, the downstream key effector YAP/TAZ (Yorkie in Drosophila) controlled by the Hippo pathway was discovered. Researchers have confirmed that LATS1/2 can phosphorylate YAP and induce the inactivation of YAP, thereby inhibiting cell proliferation, promoting apoptosis, and limiting tissue overgrowth, suggesting that YAP is an important oncogene.[34] However, YAP/TAZ lacks a DNA-binding domain, and thus, YAP/TAZ is more likely to function as a co-transcription factor rather than a transcription factor. In 2008, the YAP/TAZ interacting protein TEAD1–4 (Scalloped in Drosophila) was discovered, which binds directly to DNA and forms a transcriptional complex with YAP/TAZ, thus initiating transcriptional programs.[35,36] So far, the core of the Hippo pathway has only been partially discovered (Fig. 1).
A brief history of Hippo signaling. With increasing research, several key regulatory genes in the Hippo signaling pathway have been discovered (this figure does not include all components of the Hippo pathway). The corresponding genes between Drosophila and mammalian homologous are listed as follows: Wts-LATS1/2; Hpo-MST1/2; Yorkie-YAP/TAZ; Mats-MOB.
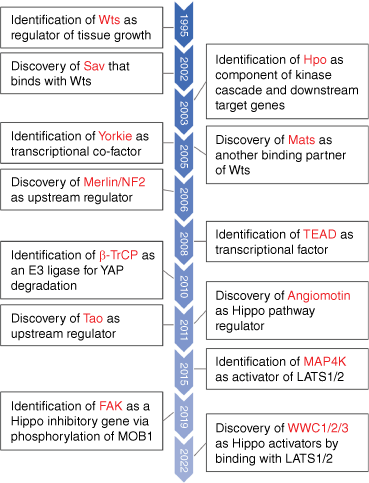
In addition, many other genes have also been identified as components of Hippo pathway, such as SAV and MOB, which can interact with LATS1 and mediate MST1/2-induced LATS1 phosphorylation.[37,38] Moreover, some factors regulating MST1/2 phosphorylation, such as RASSF1A and TAO-1, have also been found.[39,40] Additionally, MAP4K and STK25 have been shown to phosphorylate LATS1/2 independently of MST1/2.[41,42] Recently, focal adhesion kinase (FAK) was found to inhibit Hippo signaling by phosphorylating MOB1.[43] In addition, WWC1/2/3 were recently identified as novel Hippo regulators activating LATS1/2.[44] The discovery process behind these components has been comprehensively summarized elsewhere.[45] The regulatory factors of the Hippo pathway will continue to be established, allowing a more in-depth understanding of this pathway.
Upstream factors regulating Hippo signaling
To date, the Hippo pathway has been found to be regulated by a variety of upstream signals. These factors can be roughly divided into two categories, one is mechanical cues, such as the extrusion and stretching of cells, the stiffness of the extracellular matrix, and the liquid shear stress; the other is soluble cues, such as growth factor-receptor interactions (Fig. 2). Interestingly, it is worth noting that recent studies suggest Hippo signaling can also be regulated by heat, heavy metals, and glycogen accumulation.[46–48] Here, we summarize mechanical cues that can regulate Hippo signaling.
The upstream signals regulating Hippo signaling. Mechanical cues (including cell–cell contact, cell–extracellular matrix contact, cytoskeletal tension, and fluid shear stress) and soluble molecules (including certain hormones, growth factors, and chemokines) can regulate the activity of Hippo signaling.
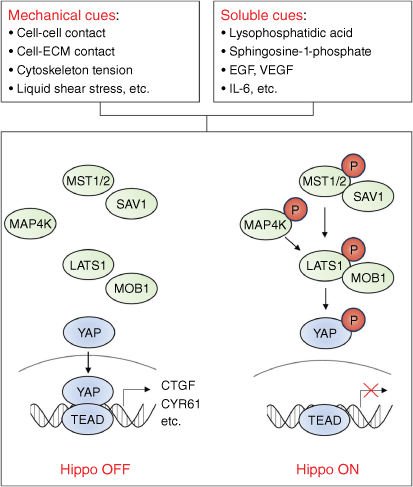
Cell–cell contact
Contact inhibition is a common phenomenon in a monolayer cell culture, whereby when the cultured cells are at high confluence, cell–cell contact will inhibit their proliferation. This contact inhibition effect underlies the regulation of embryogenesis and cancer. During embryonic development, outer cells exhibit more pronounced YAP nuclear translocation due to a relatively low cell confluence, which contrasts to inner cells that are at high density.[49] In the context of neoplasm, cultured tumor cells frequently break contact inhibition and achieve a multi-layer or agglomerate growth. This suggests that under normal physiological conditions, the Hippo pathway can restrict cell proliferation by sensing cell–cell contact. In contrast, during tumorigenesis, the Hippo pathway is often inactivated and cell proliferation is therefore no longer restricted by contact inhibition. Since the cell membrane is the first contact site between cells, it is reasonable to assume that there should be certain sensors located on the cell membrane that transmit the contact signal into the cell, thereby regulating the Hippo pathway. In fact, many studies have shown that this cellular contact inhibitory signal is largely sensed by adhesion junction molecules on the cell membrane. One such well-known adhesion junction molecule is E-cadherin. At high confluence, E-cadherin on neighboring cell membranes forms a dimer, which activates Hippo signaling and inhibits cell proliferation.[50]
Similar to adhesion junctions, tight junctions also mediate cell–cell contact inhibition by regulating Hippo signaling. For example, the tight junction component Angiomotin can interact with the Hippo signaling component NF2 (also known as Merlin), both of which have been demonstrated to have tumor suppressive roles.[51] The following studies confirm that NF2 interacts with LATS1/2 thus activating the Hippo pathway, and that this interaction is dependent on the binding of NF2 to Angiomotin. Mutations in NF2, which disrupt the interaction with tight junctions, is involved in cancer development such as neurofibromas.[52]
Cell–extracellular matrix contact
Cells are not only in contact with neighboring cells, but also with the extracellular matrix (ECM). The ECM provides a place for cells to attach, and the signaling substances in it are also crucial for cell-to-cell communication. When the adherent cells detach from the ECM, most of them cannot survive in the circulation, which is called apoptosis or, more specifically, anoikis.[53] This effect profoundly determines the fate of cells during embryogenesis and tumorigenesis. For example, most terrestrial animals have webs between their fingers in the early embryonic stage. However, the webbed structure disappears via apoptosis during development.[54] Likewise, the disappearance of frogs tails during development is associated with apoptosis.[55] In the context of cancer, anoikis resistance is the prerequisite for the metastasis of tumor cells. Given the vital roles of the Hippo pathway during development, many researchers have speculated that the Hippo pathway may sense cell–ECM contact to regulate apoptosis or anoikis. Indeed, in non-cancerous cells, LATS1/2 are activated to inhibit YAP and induce apoptosis in response to cell detachment. During the malignant transformation of cells, inactivation of the Hippo pathway prevents anoikis.[16] In addition, more and more evidence shows that anoikis is an important line of defense against tumor metastasis, and anoikis resistance caused by Hippo pathway inactivation largely contributes to tumor metastasis.[56,57]
Further mechanistic studies have shown that the integrin family of proteins play critical roles in the connection between ECM and Hippo pathway. Integrins are important molecules that anchor cells to the ECM and are responsible for sensing signals from it.[58] It has been reported that integrins bind to fibrin and activate the focal adhesion kinase FAK, which in turn activates PI3K-PDK1 signaling, thereby regulating the Hippo pathway.[59,60] In addition, integrins can also inhibit NF2, a Hippo pathway regulator, by activating RAC and PAK1.[61] These studies have confirmed that cell–ECM contact is one of the upstream regulators of the Hippo pathway.
Cytoskeleton and mechanotransduction
Another key factor regulating the Hippo pathway is the remodeling of the cytoskeleton. It has long been observed that the cytoskeleton can regulate the function of YAP, but the mechanism was not elucidated.[62] Following studies suggest that filamentous actin (F-actin) may serve as a key regulator of the Hippo pathway. On the one hand, F-actin can interact with components of the Hippo pathway, such as LATS1 and AMOT, thereby regulating the Hippo pathway.[63,64] On the other hand, F-actin regulates the Hippo pathway by mechanotransduction, because the organization of F-actin reflects the state of intracellular tension. As mentioned above, cell–cell contact and cell–ECM contact also fall into the category of mechanical cues. Therefore, researchers often discuss the influence of mechanical cues on the Hippo pathway from two aspects, namely stretching force and extrusion force.[65] In Drosophila cells, F-actin overgrowth caused by gene mutation can activate YAP, leading to tissue hyperplasia.[66,67] In mammalian cells, stretching and compression of F-actin can activate and inhibit YAP, respectively.[68,69] Importantly, YAP can affect the ECM through its target genes, which in turn regulates the surrounding mechanical environment. For example, two major YAP target genes, CTGF and CYR61, mediate cellular responses to extracellular mechanical cues.[70,71] Moreover, it has been shown that RAP2 plays a key role in the mechanotransduction of the Hippo pathway.[19] Briefly, under low stiffness conditions, RAP2 is activated to interact with MAP4K and ARHGAP29, which activates LATS1/2 thus inhibiting YAP.[19]
In addition to these mechanical cues, there are some soluble cues that also regulate the Hippo pathway, such as G protein-coupled receptor signaling activated by certain hormones.[17] Intracellular metabolic changes can also have significant effects on the Hippo pathway.[72,73] The regulatory mechanisms of these factors on the Hippo pathway have been well summarized,[74] so they will not be discussed herein.
YAP/TAZ is key oncogene downstream of Hippo signaling
In most cases, Hippo inactivation-induced tumorigenesis is largely dependent on the activation of YAP/TAZ, which is a co-transcription factor with nucleocytoplasmic shuttling properties. When the Hippo pathway is activated, YAP/TAZ is phosphorylated and then retained in the cytoplasm or degraded by ubiquitination. When the Hippo pathway is inactivated, YAP/TAZ enters the nucleus and binds to TEAD1–4 to activate the transcription of target genes.[75] So far, high expression, low phosphorylation, nuclear translocation, and copy number amplification of YAP/TAZ have been found in a variety of tumors, suggesting that YAP/TAZ is an important oncogene.[65] For example, the nuclear expression of YAP/TAZ in tumors was correlated with poor prognosis in lung cancer patients.[76] Furthermore, the expression level of TAZ in triple-negative breast cancer, the most malignant breast cancer type, is significantly higher than that in other types of breast cancer.[77] In addition, YAP activation is associated with cetuximab resistance in colorectal cancer patients, thus resulting in a poor prognosis.[78] Moreover, the expression of YAP is higher in poorly differentiated liver cancer than in well differentiated liver cancer.[79] Apart from high expression, YAP is also activated by phase separation. In response to anti‐PD‐1 therapy, interferon‐γ (IFN‐γ) promotes phase separation of YAP in nucleus, where YAP forms transcriptional hubs with many other transcription factors/co‐factors to activate gene transcription, leading to tumor immunotherapy resistance.[80] In addition to the above cancer types, activation of YAP/TAZ has also been found in many other types of tumors (including gastric cancer, pancreatic cancer, glioma, melanoma, and ovarian cancer).[81] These findings suggest that YAP/TAZ, as an oncogene in general, is widely involved in the initiation and progression of tumors.
It is well known that tumors are largely caused by genetic mutations in driver genes, such as the gain of function of oncogenes and the loss of function of tumor suppressors. Recent studies have shown that YAP/TAZ also mediates the oncogenic roles of driver mutations. For instance, KRAS gene mutation drives various tumors, including lung and pancreatic cancer. In KRAS-mutated mouse models, researchers found that YAP is required for tumor formation.[82,83] In addition to KRAS, mutations in other key oncogenes or tumor suppressor genes (including APC and LKB1) have also been found to regulate the Hippo pathway.[10] In summary, YAP/TAZ plays an important role in tumorigenesis induced by Hippo pathway inactivation.
Inactivation of Hippo signaling induces various cancer hallmarks
The Hippo pathway involves multi-functional signaling, which not only regulates cell proliferation, but also affects cell migration, invasion, metabolism, and differentiation. Therefore, inactivation of the Hippo pathway may lead to multiple cancer hallmarks, including tumor growth, stemness, metastasis, and drug resistance (Fig. 3). Moreover, these features do not exist independently, but interact with each other. For example, metabolic reprogramming supports tumor growth by activating aerobic glycolysis.[84] Dedifferentiation of tumor cells elevates their stem-like characteristics, acquiring the ability for radio- and chemoresistance, and eventually leads to tumor metastasis.[85]
Inactivation of the Hippo signaling induces various cancer hallmarks. Since a majority of YAP target genes are oncogenes, inactivation of the Hippo pathway can induce a variety of cancer hallmarks such as rapid growth, stemness-like phenotype, or distant metastasis by promoting cell proliferation, dedifferentiation, and invasion.
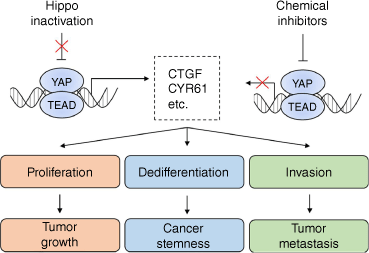
Tumor growth
As mentioned previously, the Hippo pathway senses cell density. At high confluence, cell–cell contact activates the Hippo pathway and inhibits cell proliferation. When the Hippo pathway is inactivated, the proliferation of cells is no longer restricted, which may promote tumor formation. Using Drosophila as a model, researchers found that inactivation of the Hippo pathway induces the nuclear translocation of YAP, which promotes cell proliferation.[86] Similarly, YAP was found to induce organ overgrowth by promoting cell proliferation in mouse models.[87] In cellular models, knockdown of MST1/2, and activation of YAP or TEAD, can promote cell proliferation.[35,88,89] In addition, phase separation of glycogen can sequester MST1/2 in liquid droplets, abrogating their inhibitory function to YAP. This event increases YAP activity, resulting in tumorigenesis.[48] These data demonstrate that the Hippo pathway can finely regulate cell proliferation, suggesting an important role for this pathway in tumor growth.
Mechanistically, cell proliferation is often regulated by the cell cycle. The cell cycle can generally be divided into four phases, namely G1 phase (cell size enlargement), S phase (DNA replication), G2 phase (ready to divide), and M phase (division). Regulation of the cell cycle relies on the control of checkpoints, such as the G1/S checkpoint and the S/G2 checkpoint, which check for DNA damage before and after DNA replication, respectively. Cells with DNA damage may enter the DNA repair pathway or directly undergo apoptosis to avoid entering the S phase. However, dysregulation of the cell cycle may allow cells containing abnormal DNA to bypass the checkpoint and enter division, inducing tumor growth.[90]
Recent studies have revealed how inactivation of the Hippo pathway induces tumor cell proliferation by regulating the cell cycle.[21,91] In fact, it has been shown that YAP can facilitate cells to pass the G1/S and G2/M checkpoints.[92] For instance, in the resting state, the tumor suppressor gene (i.e. cell cycle arrest protein) RB interacts with E2F, inhibiting the function of E2F, thereby inducing cell cycle arrest at the G1/S checkpoint. During the progression of the cell cycle, the cyclin kinases CDK4 and CDK6 phosphorylate RB, leading to the dissociation of RB and E2F. This effect activates E2F function, thereby enabling cells to enter the S phase.[93] Importantly, YAP and TEAD can at least partially compensate for the function of E2F, and the E2F target gene c-Myc can be also transcriptionally activated by YAP.[94,95] This evidence suggests that even if the checkpoint proteins provide cell cycle arrest signals, inactivation of the Hippo pathway can bypass these restricting signals and allow cells to continue dividing.[96] In addition to c-Myc, many cell cycle-related proteins (including MCM3 and MCM6, etc.) are also transcriptionally activated by YAP.[97,98] These findings suggest that the Hippo pathway can also directly regulate cell cycle-related proteins, thereby promoting cell proliferation and tumor growth.
Cancer stemness
It has long been speculated that tumors are derived from a small subset of cells with self-renewal capacity, called cancer stem cells.[99] These cells often show strong DNA repair ability and drug resistance capacity, which are important reasons underlying radio- and chemoresistance as well as tumor recurrence and metastasis.[100] Cancer stem cells and embryonic stem cells have many similar characteristics, and their stemness can be regulated by the Hippo pathway. In the context of embryogenesis, the developmental process of mouse embryos is stopped when YAP is knocked out.[101] In embryonic stem cells, YAP and TEAD2 can activate OCT4 and Nanog to maintain cell stemness, while inhibition of YAP and TEAD2 can induce differentiation of embryonic stem cells.[102] Besides, in embryonic stem cells that gradually lose their stemness and begin to differentiate, the expression of YAP also decreases.[103] More direct evidence suggests that YAP induces dedifferentiation of differentiated liver cells into pluripotent progenitor cells.[104] These findings suggest that activation of YAP confers the ability of cells to self-renew, indicating that inactivation of the Hippo pathway may promote stem-like properties in cells.
In the context of neoplasia, poorly differentiated tumors have stem-like characteristics and a more aggressive behavior, while benign tumors are overall well-differentiated. Inactivation of the Hippo pathway is closely related to the dedifferentiation and stem-like characteristics of tumors.[23,105,106] Breast cancer cells with low TAZ expression tend to form tumors with a low degree of malignancy (that is, well-differentiated) in mice, while breast cancer cells with high TAZ expression are prone to form poorly differentiated cancers, indicating that high TAZ expression promotes breast cancer stemness.[107] In addition, copy number amplification and high protein expression of YAP were observed in medulloblastoma stem cells.[108] Similarly, in osteosarcoma and glioma, the stemness marker SOX2 can induce inactivation of the Hippo pathway and the activation of YAP by inhibiting NF2 and WWC1, thereby maintaining the stemness of tumor stem cells.[109] These findings suggest that inactivation of the Hippo pathway may promote the self-renewal of cancer stem cells, leading to the formation of poorly differentiated cancers with a more progressive phenotype.
Tumor metastasis
Tumor metastasis refers to the process of tumor cells shedding from the primary tumor, invading blood vessels and surviving under anoikis conditions, and then colonizing to distant tissues forming metastases. Tumor metastasis means that the cancer has reached an advanced stage, with only little hope of cure and limited treatment options, and it is the ultimate cause of death for most cancer patients.[110] The mechanisms underlying tumor metastasis are very complex, involving the regulation of multiple signaling pathways, especially Notch, Wnt, Hedgehog, TGF-β, and other key developmental signaling pathways.[111–114] The Hippo signaling pathway is also closely related to tumor metastasis.[22,115] Clinical data show that low expression of MST1/2 or LATS1/2 is associated with the metastasis of gastric, breast, lung, and other tumors.[116,117] In addition, high expression of YAP/TAZ is also associated with the metastasis of many tumors including colon cancer, gastric cancer, and liver cancer.[118,119] Animal experiments have shown that mutation of a key phosphorylation site (Ser127) of YAP keeps YAP in a continuous activation state, which can induce the metastasis of breast cancer and melanoma in mice.[120] In contrast, knockdown of YAP can inhibit the metastasis of gastric cancer.[121] This suggests that inactivation of the Hippo pathway or activation of YAP largely promotes the metastasis of various tumors.
Tumor metastasis is a multi-step process, and a large number of studies have shown that the Hippo pathway is involved in almost all steps. Firstly, inactivation of the Hippo pathway promotes epithelial–mesenchymal transition (EMT) in tumor cells. Overexpression of YAP/TAZ has been found to induce EMT in various tumor cells,[122,123] while knockdown of YAP/TAZ can inhibit EMT.[124,125] Secondly, YAP promotes cell migration and invasion. For example, multiple studies have shown that knockdown or overexpression of LATS1/2 can promote or inhibit the migration and invasion of tumor cells, respectively.[126,127] Similarly, the effect of YAP/TAZ on the migration and invasion of tumor cells has also been widely reported.[128,129] Thirdly, YAP/TAZ can inhibit anoikis. Circulating tumor cells are in an anoikis state after invading blood vessels, and the ability to acquire anoikis resistance is a key step in tumor metastasis.
The use of chemical drugs to target Hippo signaling for cancer treatment
Most tumors are associated with dysregulated Hippo pathways and altered YAP/TAZ–TEAD activities.[130] Therefore, targeting this pathway using chemical compounds offers an opportunity for cancer treatment (Fig. 4). One potential strategy is to block the YAP/TAZ–TEAD interaction. According to recent in vivo tumor studies with preclinical compounds, inhibiting YAP/TAZ–TEAD interactions may be a feasible strategy. Alternatively, manipulation of upstream stimulators or downstream proteins of YAP/TAZ has also showed significant anticancer effects. The following sections discuss various therapeutic interventions, some of which have shown promise in preliminary studies.
Chemical compounds targeting the Hippo pathway in cancer. Several chemical compounds can target YAP/TAZ, or the upstream regulators of YAP/TAZ, or the downstream effectors of YAP/TAZ, thereby exhibiting antitumor activities. Lat A, Latrunculin A; CytoD, cytochalasin D; VP, verteporfin; PBP, palmitate-binding pocket; VGLL4, Vestigial-like protein 4.
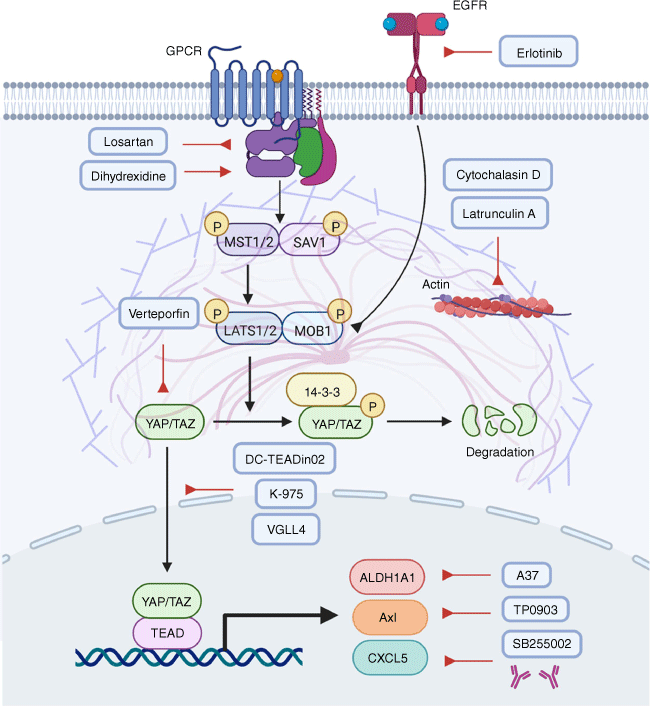
Targeting YAP/TAZ–TEAD activities
A variety of chemicals have been identified to target YAP/TAZ or TEAD. For example, verteporfin (VP) is the first inhibitor found to reduce the growth of tumors in mice by blocking YAP/TAZ–TEAD interactions.[131,132] However, VP has demonstrated proteotoxic properties, suggesting its antitumor effect may not be limited to the inhibition of the YAP–TEAD complex.[133] In addition, considering the short half-life and rapid elimination of VP in the bile, it may not be suitable for clinical use.[134,135] In spite of this, VP has been demonstrated as an effective antitumor agent in advanced pancreatic cancer.[136]
Similar to VP, vinyl sulfonamide derivatives are found to attach to the palmitate-binding pocket (PBP) of TEAD, affecting the interaction between YAP and TEAD. DC-TEADin02, an optimized vinyl sulfonamide derivative, has been shown to be a covalent inhibitor of TEAD auto-palmitoylation with an IC50 value of 197 ± 19 nM. It is noteworthy that DC-TEADin02 inhibits TEAD activity while having a minimal impact on YAP–TEAD interactions.[137] Another TEAD inhibitor, K-975, is capable of disrupting YAP/TAZ–TEAD interactions by covalently binding to a cysteine residue within TEAD’s PBP. Administration of K-975 has been shown to inhibit pleural mesothelioma, which mimics the antitumor effect of YAP deficiency.[138]
Aside from YAP/TAZ, vestigial-like protein 4 (VGLL4) binds to TEADs through their Tondu domains (TDU).[139] VGLL4 is inactivated in gastric cancer and has been recognized as a tumor suppressor.[140] Colorectal cancer (CRC) cells are more likely to proliferate when VGLL4 is inhibited.[141] Mechanistically, VGLL4 acts as an antagonist to YAP by competing with YAP for binding to TEADs through a TUD domain. In animal models, VGLL4 displays an impressive capability to penetrate cells and inhibit YAP-mediated tumorigenesis.[142]
Targeting the upstream stimulators of YAP/TAZ
Besides directly targeting YAP/TAZ, it is also tenable to inhibit YAP/TAZ indirectly by targeting their upstream stimulators. A variety of compounds have the capacity to block the upstream regulators of YAP/TAZ and suppress transcriptional activities of YAP/TAZ by promoting LATS-dependent inhibitory phosphorylation.[143,144] In addition, YAP/TAZ can activate EGFR to induce tumor formation.[145,146] In turn, persistent EGFR signaling leads to the disassembly of core complexes in the Hippo pathway, forming a signaling circuit.[147] Under such circumstances, erlotinib, which acts as an EGFR inhibitor, can disrupt YAP/TAZ activities and may therefore be useful in treating cancers driven by the EGFR-YAP/TAZ axis.[148] In addition, G-protein coupled receptor signaling also influences YAP/TAZ. Blocking Gαq/11 using losartan,[149] or activating Gαs with dihydrexidine,[17] appears to enhance the phosphorylation and consequent degradation of YAP.
Furthermore, actin polymerization facilitates the localization of YAP/TAZ to the nucleus.[66] Accordingly, polymerization modulators including latrunculin A (Lat A) and cytochalasin D (CytoD) can prevent the nuclear translocation of YAP/TAZ.[66,68] Lat A is a toxin derived from Latrunculia magnifica and is an important reagent inducing the depolymerization of actin filaments within living cells.[150] Mechanistically, Lat A attaches to actin monomers and prevents them from polymerization.[150] In gastric cancer, prostate cancer, and breast cancer, Lat A has demonstrated powerful antitumor properties.[151,152] Another actin polymerization inhibitor, CytoD, can diminish the interaction between actin monomers and cofilin.[153] Also, CytoD inhibits cofilin adhering to F-actin and slows down both actin polymerization and depolymerization in cancer cells. However, as discussed previously, YAP/TAZ can be regulated by multiple upstream mechanisms. Thus, targeting one upstream factor may not fully inhibit YAP/TAZ due to the activation of another upstream regulator.
Targeting the downstream proteins transcribed by YAP/TAZ
YAP/TAZ-driven cancer cell proliferation is largely attributed to several oncogenes transcribed by YAP/TAZ. Targeting these downstream proteins has been shown as a promising therapeutic approach. For instance, TAZ can activate the transcription of aldehyde dehydrogenase (ALDH1A1), which facilitates tumor growth and confers stemness in lung carcinoma. Using A37 as an ALDH1A1 inhibitor can reduce the stemness phenotype of lung cells in vitro and tumor formation in vivo.[154] In addition, Axl kinase is transcribed by YAP thus mediating the growth, metastasis, and drug resistance of hepatocellular carcinoma and lung cancer, which can be alleviated by the Axl inhibitor TP0903.[155,156] Of note, TP0903 exhibits significant therapeutic effects not only when used alone but also when combined with other chemotherapeutic agents, such as cisplatin and ATRA.[157]
The YAP–TEAD complex can also induce the transcription of chemokine ligand CXCL5, which contributes to the infiltration of myeloid-derived suppressor cell (MDSC) and the formation of prostate adenocarcinoma.[158] The use of CXCL5 neutralizing antibodies or the CXCL5 receptor antagonist SB255002 blocks MDSC intravasation and tumor growth. This evidence indicates that chemicals targeting Hippo-YAP signaling can be promising anticancer therapeutics.
Conclusion
This article summarizes current knowledge understanding the link between the Hippo pathway and tumor development, which may provide novel insights for the treatment of cancer. However, although significant progress has been made in this area, many questions remain to be further elucidated. For example, YAP may also function as a tumor suppressor gene by inducing cell apoptosis in response to tumor radio- or chemotherapy.[159] This finding implies a possibility that YAP inhibitor may accelerate tumor progression in some cases. In addition, the high toxicity of YAP inhibitor verteporfin limits its application for tumor therapy.[160,161] Although verteporfin theoretically functions by inhibiting the binding of YAP to TEAD, our results suggest that verteporfin also inhibits YAP expression, which is consistent with the reports of other groups.[63,132] These findings suggest that the role of verteporfin is not fully understood and there are potential off-target effects. Furthermore, as a key regulator of development, YAP is required for a variety of physiological processes in normal cells, such as the renewal of the intestinal epithelium.[162] Therefore, cancer therapeutic strategies that directly target the Hippo pathway inevitably affect normal tissues and organs, unless a specific drug delivery approach is available. Another potential strategy is to manipulate YAP target genes (such as ARHGAP29 and BMP4, etc.).[163,164] This method has higher specificity and safety, so it is more likely to become a future direction of cancer drug discovery based on the modulation of Hippo signaling.
References
1 Mintz B. Gene expression in neoplasia and differentiation. Harvey Lect 1978; 71: 193-246.
| Google Scholar |
2 Postovit LM, Margaryan NV, Seftor EA, Kirschmann DA, Lipavsky A, Wheaton WW, et al. Human embryonic stem cell microenvironment suppresses the tumorigenic phenotype of aggressive cancer cells. Proc Natl Acad Sci U S A 2008; 105: 4329-34.
| Crossref | Google Scholar |
3 Klaus A, Birchmeier W. Wnt signalling and its impact on development and cancer. Nat Rev Cancer 2008; 8: 387-98.
| Crossref | Google Scholar |
4 Briscoe J, Thérond PP. The mechanisms of Hedgehog signalling and its roles in development and disease. Nat Rev Mol Cell Biol 2013; 14: 416-29.
| Crossref | Google Scholar |
5 Bolo’s V, Grego-Bessa J, de la Pompa JL. Notch signaling in development and cancer. Endocr Rev 2007; 28: 339-63.
| Crossref | Google Scholar |
6 David CJ, Massagué J. Contextual determinants of TGFβ action in development, immunity and cancer. Nat Rev Mol Cell Biol 2018; 19: 419-35.
| Crossref | Google Scholar |
7 Yu FX, Zhao B, Guan KL. Hippo pathway in organ size control, tissue homeostasis, and cancer. Cell 2015; 163: 811-28.
| Crossref | Google Scholar |
8 Sebio A, Lenz HJ. Molecular pathways: Hippo signaling, a critical tumor suppressor. Clin Cancer Res 2015; 21: 5002-7.
| Crossref | Google Scholar |
9 Meng Z, Moroishi T, Guan KL. Mechanisms of Hippo pathway regulation. Genes Dev 2016; 30: 1-17.
| Crossref | Google Scholar |
10 Moroishi T, Hansen CG, Guan KL. The emerging roles of YAP and TAZ in cancer. Nat Rev Cancer 2015; 15: 73-9.
| Crossref | Google Scholar |
11 Sohn BH, Shim JJ, Kim SB, Jang KY, Kim SM, Kim JH, et al. Inactivation of Hippo pathway is significantly associated with poor prognosis in hepatocellular carcinoma. Clin Cancer Res 2016; 22: 1256-64.
| Crossref | Google Scholar |
12 Zhou D, Conrad C, Xia F, Park JS, Payer B, Yin Y, et al. Mst1 and Mst2 maintain hepatocyte quiescence and suppress hepatocellular carcinoma development through inactivation of the Yap1 oncogene. Cancer Cell 2009; 16: 425-38.
| Crossref | Google Scholar |
13 Kim W, Khan SK, Liu Y, Xu R, Park O, He Y, et al. Hepatic Hippo signaling inhibits protumoural microenvironment to suppress hepatocellular carcinoma. Gut 2018; 67: 1692-703.
| Crossref | Google Scholar |
14 Wang Y, Xu X, Maglic D, Dill MT, Mojumdar K, Ng PK-S, et al. Comprehensive molecular characterization of the Hippo signaling pathway in cancer. Cell Rep 2018; 25: 1304-17.e5.
| Crossref | Google Scholar |
15 Zhao B, Wei X, Li W, Udan RS, Yang Q, Kim J, et al. Inactivation of YAP oncoprotein by the Hippo pathway is involved in cell contact inhibition and tissue growth control. Genes Dev 2007; 21: 2747-61.
| Crossref | Google Scholar |
16 Zhao B, Li L, Wang L, Wang CY, Yu J, Guan KL. Cell detachment activates the Hippo pathway via cytoskeleton reorganization to induce anoikis. Genes Dev 2012; 26: 54-68.
| Crossref | Google Scholar |
17 Yu FX, Zhao B, Panupinthu N, Jewell JL, Lian I, Wang LH, et al. Regulation of the Hippo-YAP pathway by G-protein-coupled receptor signaling. Cell 2012; 150: 780-91.
| Crossref | Google Scholar |
18 Rauskolb C, Sun S, Sun G, Pan Y, Irvine KD. Cytoskeletal tension inhibits Hippo signaling through an Ajuba-Warts complex. Cell 2014; 158: 143-56.
| Crossref | Google Scholar |
19 Meng Z, Qiu Y, Lin KC, Kumar A, Placone JK, Fang C, et al. RAP2 mediates mechanoresponses of the Hippo pathway. Nature 2018; 560: 655-60.
| Crossref | Google Scholar |
20 Zheng X, Han H, Liu GP, Ma YX, Pan RL, Sang LJ, et al. LncRNA wires up Hippo and Hedgehog signaling to reprogramme glucose metabolism. EMBO J 2017; 36: 3325-35.
| Crossref | Google Scholar |
21 Ehmer U, Sage J. Control of proliferation and cancer growth by the Hippo signaling pathway. Mol Cancer Res 2016; 14: 127-40.
| Crossref | Google Scholar |
22 Janse van Rensburg HJ, Yang X. The roles of the Hippo pathway in cancer metastasis. Cell Signal 2016; 28: 1761-72.
| Crossref | Google Scholar |
23 Park JH, Shin JE, Park HW. The role of Hippo pathway in cancer stem cell biology. Mol Cells 2018; 41: 83-92.
| Crossref | Google Scholar |
24 Britschgi A, Duss S, Kim S, Couto JP, Brinkhaus H, Koren S, et al. The Hippo kinases LATS1 and 2 control human breast cell fate via crosstalk with ERα. Nature 2017; 541: 541-5.
| Crossref | Google Scholar |
25 Jeong S-H, Kim H-B, Kim M-C, Lee J-m, Lee JH, Kim J-H, et al. Hippo-mediated suppression of IRS2/AKT signaling prevents hepatic steatosis and liver cancer. J Clin Invest 2018; 128: 1010-25.
| Crossref | Google Scholar |
26 Tumaneng K, Schlegelmilch K, Russell RC, Yimlamai D, Basnet H, Mahadevan N, et al. YAP mediates crosstalk between the Hippo and PI(3)K-TOR pathways by suppressing PTEN via miR-29. Nat Cell Biol 2012; 14: 1322-9.
| Crossref | Google Scholar |
27 Brodowska K, Al-Moujahed A, Marmalidou A, Meyer Zu Horste M, Cichy J, Miller JW, et al. The clinically used photosensitizer Verteporfin (VP) inhibits YAP-TEAD and human retinoblastoma cell growth in vitro without light activation. Exp Eye Res 2014; 124: 67-73.
| Crossref | Google Scholar |
28 Michalopoulos GK, DeFrances MC. Liver regeneration. Science 1997; 276: 60-6.
| Crossref | Google Scholar |
29 Justice RW, Zilian O, Woods DF, Noll M, Bryant PJ. The Drosophila tumor suppressor gene warts encodes a homolog of human myotonic dystrophy kinase and is required for the control of cell shape and proliferation. Genes Dev 1995; 9: 534-46.
| Crossref | Google Scholar |
30 St John MAR, Tao W, Fei X, Fukumoto R, Carcangiu ML, Brownstein DG, et al. Mice deficient of Lats1 develop soft-tissue sarcomas, ovarian tumours and pituitary dysfunction. Nat Genet 1999; 21: 182-6.
| Crossref | Google Scholar |
31 Visser S, Yang X. LATS tumor suppressor: a new governor of cellular homeostasis. Cell Cycle 2010; 9: 3892-903.
| Crossref | Google Scholar |
32 Harvey KF, Pfleger CM, Hariharan IK. The Drosophila Mst ortholog, hippo, restricts growth and cell proliferation and promotes apoptosis. Cell 2003; 114: 457-67.
| Crossref | Google Scholar |
33 Jia J, Zhang W, Wang B, Trinko R, Jiang J. The Drosophila Ste20 family kinase dMST functions as a tumor suppressor by restricting cell proliferation and promoting apoptosis. Genes Dev 2003; 17: 2514-9.
| Crossref | Google Scholar |
34 Huang J, Wu S, Barrera J, Matthews K, Pan D. The Hippo signaling pathway coordinately regulates cell proliferation and apoptosis by inactivating Yorkie, the Drosophila Homolog of YAP. Cell 2005; 122: 421-34.
| Crossref | Google Scholar |
35 Ota M, Sasaki H. Mammalian Tead proteins regulate cell proliferation and contact inhibition as transcriptional mediators of Hippo signaling. Development 2008; 135: 4059-69.
| Crossref | Google Scholar |
36 Zhao B, Ye X, Yu J, Li L, Li W, Li S, et al. TEAD mediates YAP-dependent gene induction and growth control. Genes Dev 2008; 22: 1962-71.
| Crossref | Google Scholar |
37 Wu S, Huang J, Dong J, Pan D. hippo encodes a Ste-20 family protein kinase that restricts cell proliferation and promotes apoptosis in conjunction with salvador and warts. Cell 2003; 114: 445-56.
| Crossref | Google Scholar |
38 Wei X, Shimizu T, Lai ZC. Mob as tumor suppressor is activated by Hippo kinase for growth inhibition in Drosophila. EMBO J 2007; 26: 1772-81.
| Crossref | Google Scholar |
39 Guo C, Zhang X, Pfeifer GP. The tumor suppressor RASSF1A prevents dephosphorylation of the mammalian STE20-like kinases MST1 and MST2. J Biol Chem 2011; 286: 6253-61.
| Crossref | Google Scholar |
40 Boggiano JC, Vanderzalm PJ, Fehon RG. Tao-1 phosphorylates Hippo/MST kinases to regulate the Hippo–Salvador–Warts tumor suppressor pathway. Dev Cell 2011; 21: 888-95.
| Crossref | Google Scholar |
41 Meng Z, Moroishi T, Mottier-Pavie V, Plouffe SW, Hansen CG, Hong AW, et al. MAP4K family kinases act in parallel to MST1/2 to activate LATS1/2 in the Hippo pathway. Nat Commun 2015; 6: 8357.
| Crossref | Google Scholar |
42 Lim S, Hermance N, Mudianto T, Mustaly HM, Mauricio IPM, Vittoria MA, et al. Identification of the kinase STK25 as an upstream activator of LATS signaling. Nat Commun 2019; 10: 1547.
| Crossref | Google Scholar |
43 Feng X, Arang N, Rigiracciolo DC, Lee JS, Yeerna H, Wang Z, et al. A platform of synthetic lethal gene interaction networks reveals that the GNAQ uveal melanoma oncogene controls the Hippo pathway through FAK. Cancer Cell 2019; 35: 457-72.e5.
| Crossref | Google Scholar |
44 Qi S, Zhu Y, Liu X, Li P, Wang Y, Zeng Y, et al. WWC proteins mediate LATS1/2 activation by Hippo kinases and imply a tumor suppression strategy. Mol Cell 2022; 82: 1850-64.e7.
| Crossref | Google Scholar |
45 Kim W, Jho E-h. The history and regulatory mechanism of the Hippo pathway. BMB Rep 2018; 51: 106-18.
| Crossref | Google Scholar |
46 Luo M, Meng Z, Moroishi T, Lin KC, Shen G, Mo F, et al. Heat stress activates YAP/TAZ to induce the heat shock transcriptome. Nat Cell Biol 2020; 22: 1447-59.
| Crossref | Google Scholar |
47 Han H, Nakaoka HJ, Hofmann L, Zhou JJ, Yu C, Zeng L, et al. The Hippo pathway kinases LATS1 and LATS2 attenuate cellular responses to heavy metals through phosphorylating MTF1. Nat Cell Biol 2022; 24: 74-87.
| Crossref | Google Scholar |
48 Liu Q, Li J, Zhang W, Xiao C, Zhang S, Nian C, et al. Glycogen accumulation and phase separation drives liver tumor initiation. Cell 2021; 184: 5559-76.e19.
| Crossref | Google Scholar |
49 Nishioka N, Inoue K, Adachi K, Kiyonari H, Ota M, Ralston A, et al. The Hippo signaling pathway components Lats and Yap pattern Tead4 activity to distinguish mouse trophectoderm from inner cell mass. Dev Cell 2009; 16: 398-410.
| Crossref | Google Scholar |
50 Kim NG, Koh E, Chen X, Gumbiner BM. E-cadherin mediates contact inhibition of proliferation through Hippo signaling-pathway components. Proc Natl Acad Sci U S A 2011; 108: 11930-5.
| Crossref | Google Scholar |
51 Yi C, Troutman S, Fera D, Stemmer-Rachamimov A, Avila JL, Christian N, et al. A tight junction-associated Merlin-angiomotin complex mediates Merlin’s regulation of mitogenic signaling and tumor suppressive functions. Cancer Cell 2011; 19: 527-40.
| Crossref | Google Scholar |
52 Li Y, Zhou H, Li F, Chan SW, Lin Z, Wei Z, et al. Angiomotin binding-induced activation of Merlin/NF2 in the Hippo pathway. Cell Res 2015; 25: 801-17.
| Crossref | Google Scholar |
53 Chiarugi P, Giannoni E. Anoikis: a necessary death program for anchorage-dependent cells. Biochem Pharmacol 2008; 76: 1352-64.
| Crossref | Google Scholar |
54 Penaloza C, Lin L, Lockshin RA, Zakeri Z. Cell death in development: shaping the embryo. Histochem Cell Biol 2006; 126: 149-58.
| Crossref | Google Scholar |
55 Mathew S, Fu L, Fiorentino M, Matsuda H, Das B, Shi YB. Differential regulation of cell type-specific apoptosis by stromelysin-3: a potential mechanism via the cleavage of the laminin receptor during tail resorption in Xenopus laevis. J Biol Chem 2009; 284: 18545-56.
| Crossref | Google Scholar |
56 Buchheit CL, Weigel KJ, Schafer ZT. Cancer cell survival during detachment from the ECM: multiple barriers to tumour progression. Nat Rev Cancer 2014; 14: 632-41.
| Crossref | Google Scholar |
57 Cheng Y, Hou T, Ping J, Chen T, Yin B. LMO3 promotes hepatocellular carcinoma invasion, metastasis and anoikis inhibition by directly interacting with LATS1 and suppressing Hippo signaling. J Exp Clin Cancer Res 2018; 37: 228.
| Crossref | Google Scholar |
58 Larsen M, Artym VV, Green JA, Yamada KM. The matrix reorganized: extracellular matrix remodeling and integrin signaling. Curr Opin Cell Biol 2006; 18: 463-71.
| Crossref | Google Scholar |
59 Fan R, Kim NG, Gumbiner BM. Regulation of Hippo pathway by mitogenic growth factors via phosphoinositide 3-kinase and phosphoinositide-dependent kinase-1. Proc Natl Acad Sci U S A 2013; 110: 2569-74.
| Crossref | Google Scholar |
60 Kim NG, Gumbiner BM. Adhesion to fibronectin regulates Hippo signaling via the FAK–Src–PI3K pathway. J Cell Biol 2015; 210: 503-15.
| Crossref | Google Scholar |
61 Sabra H, Brunner M, Mandati V, Wehrle-Haller B, Lallemand D, Ribba AS, et al. β1 integrin–dependent Rac/group I PAK signaling mediates YAP activation of Yes-associated protein 1 (YAP1) via NF2/merlin. J Biol Chem 2017; 292: 19179-97.
| Crossref | Google Scholar |
62 Irvine KD. Integration of intercellular signaling through the Hippo pathway. Semin Cell Dev Biol 2012; 23: 812-7.
| Crossref | Google Scholar |
63 Huang Z, Zhou JK, Wang K, Chen H, Qin S, Liu J, et al. PDLIM1 inhibits tumor metastasis through activating Hippo signaling in hepatocellular carcinoma. Hepatology 2020; 71: 1643-59.
| Crossref | Google Scholar |
64 Mana-Capelli S, Paramasivam M, Dutta S, McCollum D. Angiomotins link F-actin architecture to Hippo pathway signaling. Mol Biol Cell 2014; 25: 1676-85.
| Crossref | Google Scholar |
65 Harvey KF, Zhang X, Thomas DM. The Hippo pathway and human cancer. Nat Rev Cancer 2013; 13: 246-57.
| Crossref | Google Scholar |
66 Sansores-Garcia L, Bossuyt W, Wada K-I, Yonemura S, Tao C, Sasaki H, et al. Modulating F-actin organization induces organ growth by affecting the Hippo pathway. EMBO J 2011; 30: 2325-35.
| Crossref | Google Scholar |
67 Fernández BG, Gaspar P, Brás-Pereira C, Jezowska B, Rebelo SR, Janody F. Actin-Capping Protein and the Hippo pathway regulate F-actin and tissue growth in Drosophila. Development 2011; 138: 2337-46.
| Crossref | Google Scholar |
68 Dupont S, Morsut L, Aragona M, Enzo E, Giulitti S, Cordenonsi M, et al. Role of YAP/TAZ in mechanotransduction. Nature 2011; 474: 179-83.
| Crossref | Google Scholar |
69 Wada K-I, Itoga K, Okano T, Yonemura S, Sasaki H. Hippo pathway regulation by cell morphology and stress fibers. Development 2011; 138: 3907-14.
| Crossref | Google Scholar |
70 Babic AM, Chen C-C, Lau LF. Fisp12/mouse connective tissue growth factor mediates endothelial cell adhesion and migration through integrin αvβ3, promotes endothelial cell survival, and induces angiogenesis in vivo. Mol Cell Biol 1999; 19: 2958-66.
| Crossref | Google Scholar |
71 Jedsadayanmata A, Chen CC, Kireeva ML, Lau LF, Lam SC-T. Activation-dependent adhesion of human platelets to Cyr61 and Fisp12/mouse connective tissue growth factor is mediated through integrin αIIbβ3. J Biol Chem 1999; 274: 24321-7.
| Crossref | Google Scholar |
72 Misra JR, Irvine KD. The Hippo signaling network and its biological functions. Annu Rev Genet 2018; 52: 65-87.
| Crossref | Google Scholar |
73 Koo JH, Guan KL. Interplay between YAP/TAZ and metabolism. Cell Metab 2018; 28: 196-206.
| Crossref | Google Scholar |
74 Ma S, Meng Z, Chen R, Guan KL. The Hippo pathway: biology and pathophysiology. Annu Rev Biochem 2019; 88: 577-604.
| Crossref | Google Scholar |
75 Piccolo S, Dupont S, Cordenonsi M. The biology of YAP/TAZ: Hippo signaling and beyond. Physiol Rev 2014; 94: 1287-312.
| Crossref | Google Scholar |
76 Noguchi S, Saito A, Horie M, Mikami Y, Suzuki HI, Morishita Y, et al. An integrative analysis of the tumorigenic role of TAZ in human non-small cell lung cancer. Clin Cancer Res 2014; 20: 4660-72.
| Crossref | Google Scholar |
77 Díaz-Martín J, López-García MÁ, Romero-Pérez L, Atienza-Amores MR, Pecero ML, Castilla MÁ, et al. Nuclear TAZ expression associates with the triple-negative phenotype in breast cancer. Endocr Relat Cancer 2015; 22: 443-54.
| Crossref | Google Scholar |
78 Lee KW, Lee SS, Kim SB, Sohn BH, Lee HS, Jang HJ, et al. Significant association of oncogene YAP1 with poor prognosis and cetuximab resistance in colorectal cancer patients. Clin Cancer Res 2015; 21: 357-64.
| Crossref | Google Scholar |
79 Han S-x, Bai E, Jin G-h, He C-c, Guo X-j, Wang L-j, et al. Expression and clinical significance of YAP, TAZ, and AREG in hepatocellular carcinoma. J Immunol Res 2014; 2014: 261365.
| Crossref | Google Scholar |
80 Yu M, Peng Z, Qin M, Liu Y, Wang J, Zhang C, et al. Interferon-γ induces tumor resistance to anti-PD-1 immunotherapy by promoting YAP phase separation. Mol Cell 2021; 81: 1216-30.e9.
| Crossref | Google Scholar |
81 Zanconato F, Cordenonsi M, Piccolo S. YAP/TAZ at the roots of cancer. Cancer Cell 2016; 29: 783-803.
| Crossref | Google Scholar |
82 Zhang W, Nandakumar N, Shi Y, Manzano M, Smith A, Graham G, et al. Downstream of mutant KRAS, the transcription regulator YAP is essential for neoplastic progression to pancreatic ductal adenocarcinoma. Sci Signal 2014; 7: ra42.
| Crossref | Google Scholar |
83 Shao DD, Xue W, Krall EB, Bhutkar A, Piccioni F, Wang X, et al. KRAS and YAP1 converge to regulate EMT and tumor survival. Cell 2014; 158: 171-84.
| Crossref | Google Scholar |
84 Ferreira LMR, Hebrant A, Dumont JE. Metabolic reprogramming of the tumor. Oncogene 2012; 31: 3999-4011.
| Crossref | Google Scholar |
85 Gupta PB, Pastushenko I, Skibinski A, Blanpain C, Kuperwasser C. Phenotypic plasticity: driver of cancer initiation, progression, and therapy resistance. Cell Stem Cell 2019; 24: 65-78.
| Crossref | Google Scholar |
86 Patel SH, Camargo FD, Yimlamai D. Hippo signaling in the liver regulates organ size, cell fate, and carcinogenesis. Gastroenterology 2017; 152: 533-45.
| Crossref | Google Scholar |
87 Zhao B, Li L, Lei Q, Guan KL. The Hippo-YAP pathway in organ size control and tumorigenesis: an updated version. Genes Dev 2010; 24: 862-74.
| Crossref | Google Scholar |
88 Li P, Chen Y, Mak KK, Wong CK, Wang CC, Yuan P. Functional role of Mst1/Mst2 in embryonic stem cell differentiation. PLoS One 2013; 8: e79867.
| Crossref | Google Scholar |
89 Overholtzer M, Zhang J, Smolen GA, Muir B, Li W, Sgroi DC, et al. Transforming properties of YAP, a candidate oncogene on the chromosome 11q22 amplicon. Proc Natl Acad Sci U S A 2006; 103: 12405-10.
| Crossref | Google Scholar |
90 Malumbres M, Barbacid M. Cell cycle, CDKs and cancer: a changing paradigm. Nat Rev Cancer 2009; 9: 153-66.
| Crossref | Google Scholar |
91 Barron DA, Kagey JD. The role of the Hippo pathway in human disease and tumorigenesis. Clin Transl Med 2014; 3: e25.
| Crossref | Google Scholar |
92 Shen Z, Stanger BZ. YAP regulates S-phase entry in endothelial cells. PLoS One 2015; 10: e0117522.
| Crossref | Google Scholar |
93 Hergovich A, Hemmings BA. Hippo signalling in the G2/M cell cycle phase: lessons learned from the yeast MEN and SIN pathways. Semin Cell Dev Biol 2012; 23: 794-802.
| Crossref | Google Scholar |
94 Dick FA, Rubin SM. Molecular mechanisms underlying RB protein function. Nat Rev Mol Cell Biol 2013; 14: 297-306.
| Crossref | Google Scholar |
95 Thalmeier K, Synovzik H, Mertz R, Winnacker EL, Lipp M. Nuclear factor E2F mediates basic transcription and trans-activation by E1a of the human MYC promoter. Genes Dev 1989; 3: 527-36.
| Crossref | Google Scholar |
96 Neto-Silva RM, de Beco S, Johnston LA. Evidence for a growth-stabilizing regulatory feedback mechanism between Myc and Yorkie, the Drosophila homolog of Yap. Dev Cell 2010; 19: 507-20.
| Crossref | Google Scholar |
97 Kapoor A, Yao W, Ying H, Hua S, Liewen A, Wang Q, et al. Yap1 activation enables bypass of oncogenic Kras addiction in pancreatic cancer. Cell 2014; 158: 185-97.
| Crossref | Google Scholar |
98 Ehmer U, Zmoos AF, Auerbach RK, Vaka D, Butte AJ, Kay MA, et al. Organ size control is dominant over Rb family inactivation to restrict proliferation in vivo. Cell Rep 2014; 8: 371-81.
| Crossref | Google Scholar |
99 Lytle NK, Barber AG, Reya T. Stem cell fate in cancer growth, progression and therapy resistance. Nat Rev Cancer 2018; 18: 669-80.
| Crossref | Google Scholar |
100 Shibue T, Weinberg RA. EMT, CSCs, and drug resistance: the mechanistic link and clinical implications. Nat Rev Clin Oncol 2017; 14: 611-29.
| Crossref | Google Scholar |
101 Morin-Kensicki EM, Boone BN, Howell M, Stonebraker JR, Teed J, Alb JG, et al. Defects in yolk sac vasculogenesis, chorioallantoic fusion, and embryonic axis elongation in mice with targeted disruption of Yap65. Mol Cell Biol 2006; 26: 77-87.
| Crossref | Google Scholar |
102 Tamm C, Böwer N, Annerén C. Regulation of mouse embryonic stem cell self-renewal by a Yes-YAP-TEAD2 signaling pathway downstream of LIF. J Cell Sci 2011; 124: 1136-44.
| Crossref | Google Scholar |
103 Lian I, Kim J, Okazawa H, Zhao J, Zhao B, Yu J, et al. The role of YAP transcription coactivator in regulating stem cell self-renewal and differentiation. Genes Dev 2010; 24: 1106-18.
| Crossref | Google Scholar |
104 Yimlamai D, Christodoulou C, Galli GG, Yanger K, Pepe-Mooney B, Gurung B, et al. Hippo pathway activity influences liver cell fate. Cell 2014; 157: 1324-38.
| Crossref | Google Scholar |
105 Hao J, Zhang Y, Jing D, Li Y, Li J, Zhao Z. Role of Hippo signaling in cancer stem cells. J Cell Physiol 2014; 229: 266-70.
| Crossref | Google Scholar |
106 Mo JS, Park HW, Guan KL. The Hippo signaling pathway in stem cell biology and cancer. EMBO Rep 2014; 15: 642-56.
| Crossref | Google Scholar |
107 Cordenonsi M, Zanconato F, Azzolin L, Forcato M, Rosato A, Frasson C, et al. The Hippo transducer TAZ confers cancer stem cell-related traits on breast cancer cells. Cell 2011; 147: 759-72.
| Crossref | Google Scholar |
108 Fernandez-L A, Northcott PA, Dalton J, Fraga C, Ellison D, Angers S, et al. YAP1 is amplified and up-regulated in hedgehog-associated medulloblastomas and mediates Sonic hedgehog-driven neural precursor proliferation. Genes Dev 2009; 23: 2729-41.
| Crossref | Google Scholar |
109 Basu-Roy U, Bayin NS, Rattanakorn K, Han E, Placantonakis DG, Mansukhani A, et al. Sox2 antagonizes the Hippo pathway to maintain stemness in cancer cells. Nat Commun 2015; 6: 6411.
| Crossref | Google Scholar |
110 Mehlen P, Puisieux A. Metastasis: a question of life or death. Nat Rev Cancer 2006; 6: 449-58.
| Crossref | Google Scholar |
111 Sethi N, Kang Y. Notch signalling in cancer progression and bone metastasis. Br J Cancer 2011; 105: 1805-10.
| Crossref | Google Scholar |
112 VanderVorst K, Dreyer CA, Konopelski SE, Lee H, Ho H-YH, Carraway III KL. Wnt/PCP signaling contribution to carcinoma collective cell migration and metastasis. Cancer Res 2019; 79: 1719-29.
| Crossref | Google Scholar |
113 Yao Z, Han L, Chen Y, He F, Sun B, Kamar S, et al. Hedgehog signalling in the tumourigenesis and metastasis of osteosarcoma, and its potential value in the clinical therapy of osteosarcoma. Cell Death Dis 2018; 9: 701.
| Crossref | Google Scholar |
114 Drabsch Y, ten Dijke P. TGF-β signalling and its role in cancer progression and metastasis. Cancer Metastasis Rev 2012; 31: 553-68.
| Crossref | Google Scholar |
115 Warren JSA, Xiao Y, Lamar JM. YAP/TAZ activation as a target for treating metastatic cancer. Cancers (Basel) 2018; 10: 115.
| Crossref | Google Scholar |
116 Lin XY, Zhang XP, Wu JH, Qiu XS, Wang EH. Expression of LATS1 contributes to good prognosis and can negatively regulate YAP oncoprotein in non-small-cell lung cancer. Tumour Biol 2014; 35: 6435-43.
| Crossref | Google Scholar |
117 Takahashi Y, Miyoshi Y, Takahata C, Irahara N, Taguchi T, Tamaki Y, et al. Down-regulation of LATS1 and LATS2 mRNA expression by promoter hypermethylation and its association with biologically aggressive phenotype in human breast cancers. Clin Cancer Res 2005; 11: 1380-5.
| Crossref | Google Scholar |
118 Liang K, Zhou G, Zhang Q, Li J, Zhang C. Expression of hippo pathway in colorectal cancer. Saudi J Gastroenterol 2014; 20: 188-94.
| Crossref | Google Scholar |
119 Zhou GX, Li XY, Zhang Q, Zhao K, Zhang CP, Xue CH, et al. Effects of the hippo signaling pathway in human gastric cancer. Asian Pac J Cancer Prev 2013; 14: 5199-205.
| Crossref | Google Scholar |
120 Lamar JM, Stern P, Liu H, Schindler JW, Jiang ZG, Hynes RO. The Hippo pathway target, YAP, promotes metastasis through its TEAD-interaction domain. Proc Natl Acad Sci U S A 2012; 109: E2441-50.
| Crossref | Google Scholar |
121 Zhou Z, Zhu JS, Gao CP, Li LP, Zhou C, Wang H, et al. siRNA targeting YAP gene inhibits gastric carcinoma growth and tumor metastasis in SCID mice. Oncol Lett 2016; 11: 2806-14.
| Crossref | Google Scholar |
122 Ling HH, Kuo CC, Lin BX, Huang YH, Lin CW. Elevation of YAP promotes the epithelial-mesenchymal transition and tumor aggressiveness in colorectal cancer. Exp Cell Res 2017; 350: 218-25.
| Crossref | Google Scholar |
123 Qu Y, Zhang L, Wang J, Chen P, Jia Y, Wang C, et al. Yes-associated protein (YAP) predicts poor prognosis and regulates progression of esophageal squamous cell cancer through epithelial-mesenchymal transition. Exp Ther Med 2019; 18: 2993-3001.
| Crossref | Google Scholar |
124 Zhou M, Zhang Y, Wei H, He J, Wang D, Chen B, et al. Furin inhibitor D6R suppresses epithelial-mesenchymal transition in SW1990 and PaTu8988 cells via the Hippo-YAP signaling pathway. Oncol Lett 2018; 15: 3192-6.
| Crossref | Google Scholar |
125 Tang X, Sun Y, Wan G, Sun J, Sun J, Pan C. Knockdown of YAP inhibits growth in Hep-2 laryngeal cancer cells via epithelial-mesenchymal transition and the Wnt/β-catenin pathway. BMC Cancer 2019; 19: 654.
| Crossref | Google Scholar |
126 Visser S, Yang X. Identification of LATS transcriptional targets in HeLa cells using whole human genome oligonucleotide microarray. Gene 2010; 449: 22-9.
| Crossref | Google Scholar |
127 Li Z, Wang Y, Zhu Y, Yuan C, Wang D, Zhang W, et al. The Hippo transducer TAZ promotes epithelial to mesenchymal transition and cancer stem cell maintenance in oral cancer. Mol Oncol 2015; 9: 1091-105.
| Crossref | Google Scholar |
128 Zhang Y, Xie P, Wang X, Pan P, Wang Y, Zhang H, et al. YAP promotes migration and invasion of human glioma cells. J Mol Neurosci 2018; 64: 262-72.
| Crossref | Google Scholar |
129 Chan SW, Lim CJ, Guo K, Ng CP, Lee I, Hunziker W, et al. A role for TAZ in migration, invasion, and tumorigenesis of breast cancer cells. Cancer Res 2008; 68: 2592-8.
| Crossref | Google Scholar |
130 Dey A, Varelas X, Guan KL. Targeting the Hippo pathway in cancer, fibrosis, wound healing and regenerative medicine. Nat Rev Drug Discov 2020; 19: 480-94.
| Crossref | Google Scholar |
131 Liu-Chittenden Y, Huang B, Shim JS, Chen Q, Lee SJ, Anders RA, et al. Genetic and pharmacological disruption of the TEAD-YAP complex suppresses the oncogenic activity of YAP. Genes Dev 2012; 26: 1300-5.
| Crossref | Google Scholar |
132 Wang C, Zhu X, Feng W, Yu Y, Jeong K, Guo W, et al. Verteporfin inhibits YAP function through up-regulating 14-3-3σ sequestering YAP in the cytoplasm. Am J Cancer Res 2016; 6: 27-37.
| Google Scholar |
133 Zhang H, Ramakrishnan SK, Triner D, Centofanti B, Maitra D, Győrffy B, et al. Tumor-selective proteotoxicity of verteporfin inhibits colon cancer progression independently of YAP1. Sci Signal 2015; 8: ra98.
| Crossref | Google Scholar |
134 Shamul JG, Shah SR, Kim J, Schiapparelli P, Vazquez-Ramos CA, Lee BJ, et al. Verteporfin-loaded anisotropic poly(beta-amino ester)-based micelles demonstrate brain cancer-selective cytotoxicity and enhanced pharmacokinetics. Int J Nanomedicine 2019; 14: 10047-60.
| Crossref | Google Scholar |
135 Houle JM, Strong A. Clinical pharmacokinetics of verteporfin. J Clin Pharmacol 2002; 42: 547-57.
| Crossref | Google Scholar |
136 Huggett MT, Jermyn M, Gillams A, Illing R, Mosse S, Novelli M, et al. Phase I/II study of verteporfin photodynamic therapy in locally advanced pancreatic cancer. Br J Cancer 2014; 110: 1698-704.
| Crossref | Google Scholar |
137 Lu W, Wang J, Li Y, Tao H, Xiong H, Lian F, et al. Discovery and biological evaluation of vinylsulfonamide derivatives as highly potent, covalent TEAD autopalmitoylation inhibitors. Eur J Med Chem 2019; 184: 111767.
| Crossref | Google Scholar |
138 Kaneda A, Seike T, Danjo T, Nakajima T, Otsubo N, Yamaguchi D, et al. The novel potent TEAD inhibitor, K-975, inhibits YAP1/TAZ-TEAD protein-protein interactions and exerts an anti-tumor effect on malignant pleural mesothelioma. Am J Cancer Res 2020; 10: 4399-415.
| Google Scholar |
139 Deng X, Fang L. VGLL4 is a transcriptional cofactor acting as a novel tumor suppressor via interacting with TEADs. Am J Cancer Res 2018; 8: 932-43.
| Google Scholar |
140 Jiao S, Wang H, Shi Z, Dong A, Zhang W, Song X, et al. A peptide mimicking VGLL4 function acts as a YAP antagonist therapy against gastric cancer. Cancer Cell 2014; 25: 166-80.
| Crossref | Google Scholar |
141 Jiao S, Li C, Hao Q, Miao H, Zhang L, Li L, et al. VGLL4 targets a TCF4–TEAD4 complex to coregulate Wnt and Hippo signalling in colorectal cancer. Nat Commun 2017; 8: 14058.
| Crossref | Google Scholar |
142 Zhang W, Gao Y, Li P, Shi Z, Guo T, Li F, et al. VGLL4 functions as a new tumor suppressor in lung cancer by negatively regulating the YAP-TEAD transcriptional complex. Cell Res 2014; 24: 331-43.
| Crossref | Google Scholar |
143 Calses PC, Crawford JJ, Lill JR, Dey A. Hippo pathway in cancer: aberrant regulation and therapeutic opportunities. Trends Cancer 2019; 5: 297-307.
| Crossref | Google Scholar |
144 Park HW, Guan KL. Regulation of the Hippo pathway and implications for anticancer drug development. Trends Pharmacol Sci 2013; 34: 581-9.
| Crossref | Google Scholar |
145 Yang N, Morrison CD, Liu P, Miecznikowski J, Bshara W, Han S, et al. TAZ induces growth factor-independent proliferation through activation of EGFR ligand amphiregulin. Cell Cycle 2012; 11: 2922-30.
| Crossref | Google Scholar |
146 He C, Lv X, Hua G, Lele SM, Remmenga S, Dong J, et al. YAP forms autocrine loops with the ERBB pathway to regulate ovarian cancer initiation and progression. Oncogene 2015; 34: 6040-54.
| Crossref | Google Scholar |
147 Ando T, Arang N, Wang Z, Costea DE, Feng X, Goto Y, et al. EGFR regulates the Hippo pathway by promoting the tyrosine phosphorylation of MOB1. Commun Biol 2021; 4: 1237.
| Crossref | Google Scholar |
148 Zhang J, Ji JY, Yu M, Overholtzer M, Smolen GA, Wang R, et al. YAP-dependent induction of amphiregulin identifies a non-cell-autonomous component of the Hippo pathway. Nat Cell Biol 2009; 11: 1444-50.
| Crossref | Google Scholar |
149 Wennmann DO, Vollenbröker B, Eckart AK, Bonse J, Erdmann F, Wolters DA, et al. The Hippo pathway is controlled by Angiotensin II signaling and its reactivation induces apoptosis in podocytes. Cell Death Dis 2014; 5: e1519.
| Crossref | Google Scholar |
150 Fujiwara I, Zweifel ME, Courtemanche N, Pollard TD. Latrunculin A accelerates actin filament depolymerization in addition to sequestering actin monomers. Curr Biol 2018; 28: 3183-92.e2.
| Crossref | Google Scholar |
151 Sayed KA, Khanfar MA, Shallal HM, Muralidharan A, Awate B, Youssef DT, et al. Latrunculin A and its C-17-O-carbamates inhibit prostate tumor cell invasion and HIF-1 activation in breast tumor cells. J Nat Prod 2008; 71: 396-402.
| Crossref | Google Scholar |
152 Konishi H, Kikuchi S, Ochiai T, Ikoma H, Kubota T, Ichikawa D, et al. Latrunculin A has a strong anticancer effect in a peritoneal dissemination model of human gastric cancer in mice. Anticancer Res 2009; 29: 2091-7.
| Google Scholar |
153 Kim M, Song K, Jin EJ, Sonn J. Staurosporine and cytochalasin D induce chondrogenesis by regulation of actin dynamics in different way. Exp Mol Med 2012; 44: 521-8.
| Crossref | Google Scholar |
154 Yu J, Alharbi A, Shan H, Hao Y, Snetsinger B, Rauh MJ, et al. TAZ induces lung cancer stem cell properties and tumorigenesis by up-regulating ALDH1A1. Oncotarget 2017; 8: 38426-43.
| Crossref | Google Scholar |
155 Xu MZ, Chan SW, Liu AM, Wong KF, Fan ST, Chen J, et al. AXL receptor kinase is a mediator of YAP-dependent oncogenic functions in hepatocellular carcinoma. Oncogene 2011; 30: 1229-40.
| Crossref | Google Scholar |
156 Ghiso E, Migliore C, Ciciriello V, Morando E, Petrelli A, Corso S, et al. YAP-Dependent AXL Overexpression Mediates Resistance to EGFR Inhibitors in NSCLC. Neoplasia 2017; 19: 1012-21.
| Crossref | Google Scholar |
157 Aveic S, Corallo D, Porcù E, Pantile M, Boso D, Zanon C, et al. TP-0903 inhibits neuroblastoma cell growth and enhances the sensitivity to conventional chemotherapy. Eur J Pharmacol 2018; 818: 435-48.
| Crossref | Google Scholar |
158 Wang G, Lu X, Dey P, Deng P, Wu CC, Jiang S, et al. Targeting YAP-dependent MDSC infiltration impairs tumor progression. Cancer Discov 2016; 6: 80-95.
| Crossref | Google Scholar |
159 Zhu N, Yang R, Wang X, Yuan L, Li X, Wei F, et al. The Hippo signaling pathway: from multiple signals to the hallmarks of cancers. Acta Biochim Biophys Sin (Shanghai) 2023; 55: 1-10.
| Crossref | Google Scholar |
160 Gibault F, Corvaisier M, Bailly F, Huet G, Melnyk P, Cotelle P. Non-photoinduced biological properties of Verteporfin. Curr Med Chem 2016; 23: 1171-84.
| Crossref | Google Scholar |
161 Konstantinou EK, Notomi S, Kosmidou C, Brodowska K, Al-Moujahed A, Nicolaou F, et al. Verteporfin-induced formation of protein cross-linked oligomers and high molecular weight complexes is mediated by light and leads to cell toxicity. Sci Rep 2017; 7: 46581.
| Crossref | Google Scholar |
162 Karpowicz P, Perez J, Perrimon N. The Hippo tumor suppressor pathway regulates intestinal stem cell regeneration. Development 2010; 137: 4135-45.
| Crossref | Google Scholar |
163 Qiao Y, Chen J, Lim YB, Finch-Edmondson ML, Seshachalam VP, Qin L, et al. YAP regulates actin dynamics through ARHGAP29 and promotes metastasis. Cell Rep 2017; 19: 1495-502.
| Crossref | Google Scholar |
164 Lai D, Yang X. BMP4 is a novel transcriptional target and mediator of mammary cell migration downstream of the Hippo pathway component TAZ. Cell Signal 2013; 25: 1720-8.
| Crossref | Google Scholar |