Location has a significant effect on body condition and blood parameters in the eastern longneck turtle (Chelodina longicollis)
Titus Franciscus Scheelings
A
Abstract
The aim of this investigation was to explore the effect point of capture has on relative weight (Wr), as well as haematology and biochemistry values, in wild eastern longneck turtles (Chelodina longicollis). This study group consisted of two sites of turtles residing in Duck Pond (DP) and Ivanhoe Wetland (IW) in the Darebin Parklands, in Alphington, Melbourne. From DP, 184 turtles were captured, and from IW, 37 turtles were captured. All turtles were weighed and measured, and a random subset of 20 turtles from each waterbody was selected for blood collection. Significant differences were found to exist for Wr, basophils, glucose, uric acid, triglycerides and bile acids between the two sites. Serum glucose levels tended to decrease as a turtle increased in mass, straight carapace length (SCL) and Wr. The results of this investigation highlight the need to take location into consideration when assessing blood parameters in reptiles.
Keywords: biochemistry, body condition, Chelodina longicollis, eastern longneck turtle, haematology, location, mass, serum glucose.
Introduction
Reptiles are frequently presented to veterinarians, and assessment of haematology and biochemistry parameters form a routine component of clinical examination in this taxon. Although details surrounding the methods and collection sites for venipuncture are relatively well described in a range of herptiles (de la Navarre 2006), analysis of results can be hampered by a lack of understanding of normal homeostasis in these non-domestic species. Blood parameters in herpetofauna are highly influenced by a range of extrinsic and intrinsic factors, including age (Eva et al. 2022), sex (Bielli et al. 2015; Johnson et al. 2018; Howard and Jaensch 2021), reproductive status (Rafferty et al. 2014; Howard and Jaensch 2021), season (Andrade et al. 2004; Bryant et al. 2012; Howard and Jaensch 2021), location (Bryant et al. 2012; Scheelings et al. 2020) and environment (Day et al. 2007). This variability makes the use of reference intervals for determining health or disease in reptiles problematic, especially when these data have been generated from a single population at a specific point in time. Reference ranges for Australian freshwater turtles are particularly depauperate, with only scant reports investigating the effects of physiological status on the blood values of a small number of species (Flint et al. 2011; Scheelings and Rafferty 2012; Rafferty et al. 2014). An improved understanding of Australian freshwater chelonian physiology is of considerable conservation importance, with nearly half (11 of 25, 44%) of the 25 taxa currently listed as threatened, and many populations experiencing significant declines (Van Dyke et al. 2018).
Clinical pathology is useful for evaluating baseline health status in wild animals and for assessing their response to disease. A range of haematological and biochemical parameters can be evaluated, but selection of analytes may depend on factors such as personal preference of the investigator, analysis costs or sensitivity to the time-lag from blood collection in the field and transport to the laboratory (Maceda-Veiga et al. 2015). Furthermore, the diagnostic value of specific tests depends on what question the investigator is attempting to answer. For example, analysis of red blood cell indices (colour, morphology, number, etc.) are important in formulating a clinical picture and determining whether an individual may be dehydrated, anaemic or suffering from a nutritional deficiency (Maceda-Veiga et al. 2015). Differential white blood cell counts can be used to assess response to stress, tissue damage or infectious disease (Maceda-Veiga et al. 2015). Interpretation of biochemical parameters is more problematic in reptiles, especially freshwater turtles, because tissue activities of blood enzymes have not been specifically evaluated in these species (Rosser 2022).
It is difficult to explore factors that influence morphology and physiology in wild animals due to the multitude of confounding variables that cannot be controlled. These may include food availability and local weather events. However, to some extent, the role that these variables may be exerting their effects on homeostasis can be minimised by sourcing populations of animals that are close in proximity and are sampled at the same time of year. Therefore, the purpose of this investigation was to explore the role capture site may have on morphology, and blood values, in wild eastern longneck turtles (Chelodina longicollis) during summer.
Materials and methods
Ethics statement
This study was approved by The University of Melbourne Office of Research Ethics and Integrity (Ethics ID: 2022-24808-32226-4), and all experiments were performed in accordance with relevant guidelines and regulations. Turtles were trapped and sampled under permit 10010480 from the Department of Environment, Land, Water and Planning and permit number RP1497 from the Victorian Fisheries Authority. All turtles were released alive at their point of capture immediately after sampling had been completed.
Study site
Turtles were trapped from two waterbodies, Duck Pond (DP, −37.7732825, 145.0348189) and Ivanhoe Wetland (IW, −37.7734116, 145.0354874), within the Darebin Parklands, in Alphington, Victoria, Australia (Fig. 1), in the Australian summer of 2022/23. These two waterbodies are separated by an approximate distance of 200 m in a straight line (Fig. 1), and there are differences in topography between the two sites. DP is in an area of high public visitation, is lined by scant vegetation along the edges of the bank and has a large population of waterbirds. IW is less accessible to the public, is well vegetated along the edge of the water and there are extensive reeds and grass beds within the waterbody.
Sample collection
Turtles were trapped using large, 6-hoop fyke nets with 28 mm black, knotless mesh and a 2.5 m wing with a 48 cm drop and baited with lamb liver. Six nets were placed into each waterbody and left overnight for a single night of trapping at each location. In the morning, traps were brought back to land and turtles removed for sample collection. Turtles were initially trapped in DP, and then 2 weeks later in IW.
After removal from the trap, turtles were weighed using standard kitchen scales, and a straight carapace length (SCL) was obtained using Haglof Mantax tree calipers. No attempt was made to determine sex, because it is difficult to accurately identify sex in this species based on morphometric data. After weighing, a subset (n = 20) of turtles was randomly selected for blood collection. Blood was not permitted to be collected from more than 20 turtles from each site due to Ethics Approval constraints. For blood collection, the turtle was placed into dorsal recumbency and an area of skin over the jugular vein was prepared using alcohol wipes. Blood was obtained from the jugular vein while the turtle’s head was retracted within the shell. A 25G needle attached to a 3 mL syringe was used, and a volume not exceeding 1% of body weight was obtained from each individual. Immediately after collection, 250 μL of blood was transferred into a lithium heparin container (Sarstedt AG & Co., Nümbrecht, Germany) and the remainder into gel clot activator tubes (Sarstedt AG & Co., Nümbrecht, Germany). Blood tubes were then placed into a portable ice pack and taken to the laboratory for processing. Blood smears were prepared by placing a drop of blood onto a microscope slide and smearing it with a cover slip. Slides were stained with Wright’s Giemsa (Siemens Australia, Bayswater, Australia) using an automatic stainer, Siemens Hematek (Siemens Australia, Bayswater, Australia). Packed cell volume (PCV) was determined using microhaematocrit tubes centrifuged in a Vet1 Mini Spin 12 centrifuge (Vet1, Coomera, Australia) spun at 3000g for 4 min. Leukocyte differential counts were performed manually on blood films, and white cells were classified as heterophils, lymphocytes, eosinophils, basophils or monocytes (Campbell and Grant 2022a). Heterophil/eosinophil counts were performed manually using a haemocytometer and by staining whole blood with phloxine B (made in-house). The total white cell count (TWCC) count was calculated by correcting the manual count for the percentage of heterophils and eosinophils present (Campbell and Grant 2022b). The heterophil:lymphocyte ratio was calculated as a measure of stress (Johnstone et al. 2012). Both PCV and heterophil/eosinophil counts were determined within 3 h of blood collection. Blood in the plain tube was centrifuged, and the resultant serum removed and stored at −80°C for a maximum of 2 weeks until analysis. Serum was analysed using a Cobas Integra 400 plus (Roche Diagnostics, Basel, Switzerland).
Determination of body condition
Body condition of turtles was assessed using a relative weight (Wr) measurement (Pope and Kruse 2007) with the formula:
where W = mass of the turtle and Ws = a length-specific standard weight predicted from existing data (K. Howard, unpubl. data), and can be calculated as follows:
The Wr value was then used as a measure of body condition with the following scoring system:
Statistics and data analysis
All statistical analysis was performed using the statistical software program R (R Development CoreTeam 2015). Data were assessed for normality with the Shapiro–Wilk test. For normally distributed data, Welch’s two sample t-test was conducted to identify whether there were significant differences in body condition or haematologic and biochemical values between populations, and for non-normally distributed data the Wilcoxon Rank sum test was used. To determine if blood results were correlated to morphology, linear regression analysis was used. To confirm the suitability of these data for linear model testing, the homogeneity of variances (residuals vs fitted plots and scale-location plots), the normality of residuals (Q-Q plots), and Cook’s Distance were explored. Although some relationships had the appearance of being non-monotonic when fitted with a loess smoothed line, an examination of the diagnostic plots still supported a best interpretation that the residuals of all models were suitable for linear fitting. Significance for all tests was accepted at P < 0.05.
Results
From DP, 184 turtles were trapped with a mean Wr of 104.79 (±7.35), and from IW, 37 turtles were captured with a mean Wr of 103.01 (±8.45) (Fig. 2, Table 1). The observed difference in body condition between trapping sites was significant (P = 0.04). Turtles from IW had a higher proportion of individuals in poor body condition (n = 2, 5.4%), compared with DP (n = 7, 3.8%) (Fig. 2).
Boxplot displaying differences in relative weight (Wr) of turtles in relation to capture site. Green lines indicate range for average body condition. Turtles from Duck Pond had higher mean Wr and fewer animals in poor body condition in comparison with turtles from Ivanhoe Wetland.
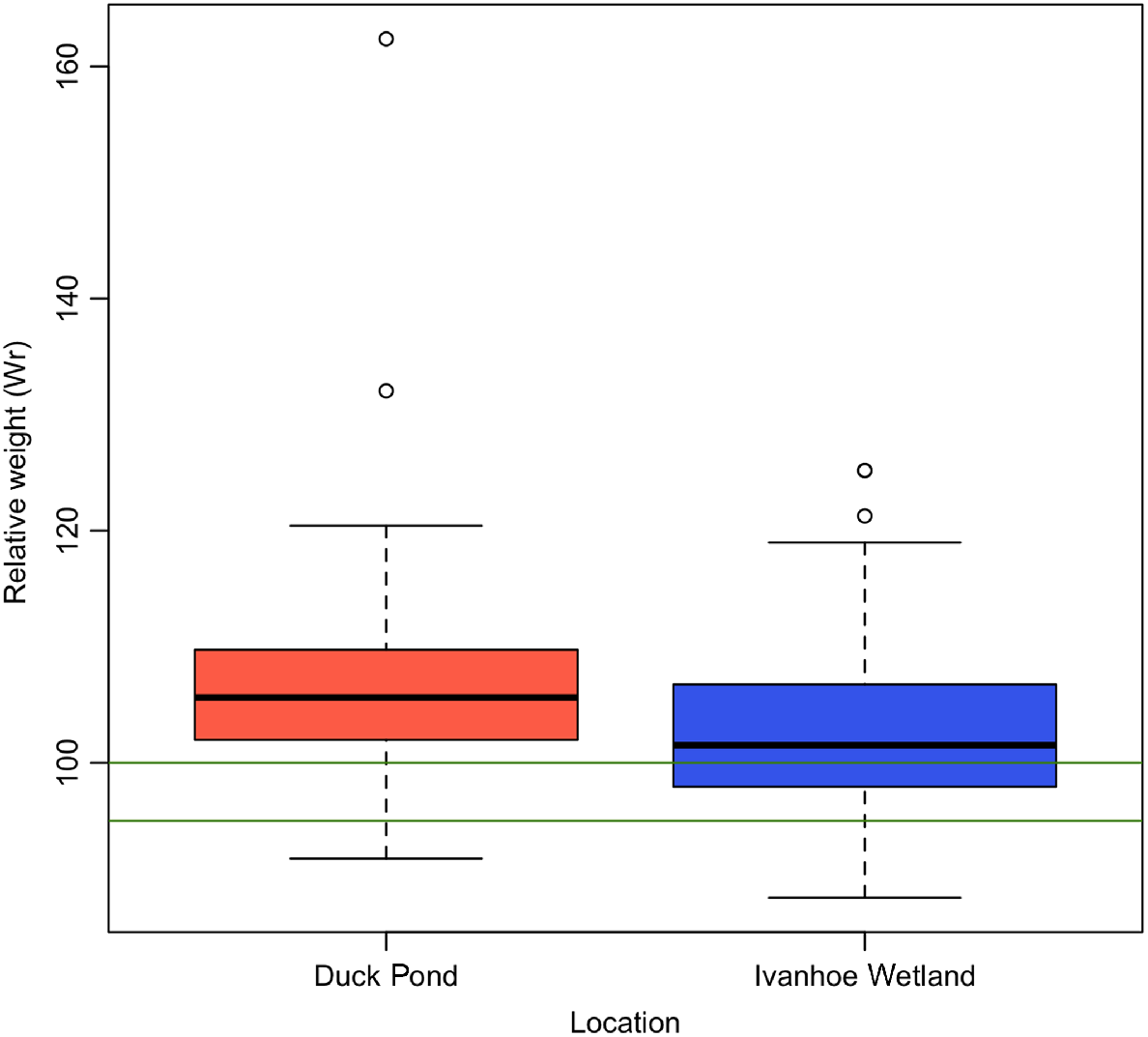
Parameter | Duck pond | Ivanhoe wetland | P | t | d.f. | W | |||
---|---|---|---|---|---|---|---|---|---|
Mean (±s.d.) | Min-max | Mean (±s.d.) | Min-max | ||||||
Relative weight (Wr) | 104.79 (±7.35) | 90.45–160.28 | 103.01 (±8.45) | 87.13–123.38 | 0.04 | – | – | 0.91 | |
PCV (%) | 30.60 (±4.99) | 23.00–42.00 | 30.65 (±4.52) | 22.00–38.00 | 1.00 | 0.00 | 37.64 | – | |
TWCC (109/μL) | 17.30 (±6.13) | 4.90–26.20 | 13.60 (±6.10) | 4.60–26.87 | 0.06 | 1.92 | 38.00 | – | |
Heterophils (109/μL) | 5.90 (±3.00) | 1.13–13.62 | 4.57 (±3.03) | 0.69–12.39 | 0.13 | – | – | 257.00 | |
Monocytes (109/μL) | 0.40 (±0.29) | 0.00–1.05 | 0.36 (±0.35) | 0.00–1.58 | 0.61 | – | – | 219.50 | |
Lymphocytes (109/μL) | 10.70 (±3.76) | 3.38–17.78 | 8.55 (±3.63) | 3.73–17.20 | 0.06 | 1.90 | 37.95 | – | |
Eosinophils (109/μL) | 0.06 (±0.13) | 0.00–0.53 | 0.01 (±0.03) | 0.00–0.12 | 0.07 | – | – | 241.50 | |
Basophils (109/μL) | 0.18 (±0.14) | 0.00–0.47 | 0.13 (±0.19) | 0.00–0.81 | 0.04 | – | – | 272.00 | |
H:L | 0.52 (±0.23) | 0.19–1.21 | 0.55 (±0.31) | 0.14–1.37 | 0.71 | – | – | 214.00 | |
Albumin g/L | 20.47 (±3.06) | 14.00–26.00 | 19.95 (±2.84) | 11.00–24.00 | 0.83 | – | – | 198.00 | |
Amylase U/L | 550.63 (±167.55) | 209.00–808.00 | 474.70 (±181.50) | 148.00–777.00 | 0.18 | 1.35 | 36.97 | – | |
AST U/L | 73.74 (±13.96) | 53.00–109.00 | 74.35 (±21.20) | 36.00–117.00 | 0.91 | −0.11 | 33.04 | – | |
Calcium mmol/L | 3.26 (±0.54) | 1.99–4.58 | 3.07 (±0.58) | 2.14–4.18 | 0.30 | 1.04 | 37.00 | – | |
Cholesterol mmol/L | 3.42 (±0.60) | 2.20–4.30 | 3.73 (±1.27) | 1.30–5.80 | 0.33 | −0.98 | 27.76 | – | |
CK U/L | 190.63 (±131.7) | 33.00–448.00 | 195.80 (±143.30) | 13.00–506.00 | 0.94 | – | – | 187.00 | |
GLDH U/L | 2.00 (±1.17) | 0.70–5.30 | 1.99 (±0.97) | 2.10–4.80 | 0.83 | – | – | 172.50 | |
Glucose mmol/L | 7.82 (±3.42) | 2.20–14.10 | 5.46 (±2.89) | 2.10–12.80 | 0.02 | – | – | 273.00 | |
Total protein g/L | 38.37 (±5.3) | 27.00–49.00 | 39.55 (±4.39) | 25.00–46.00 | 0.26 | – | – | 150.00 | |
Urea mmol/L | 4.97 (±1.79) | 1.80–9.20 | 9.02 (±20.45) | 1.80–95.00 | 0.37 | – | – | 222.00 | |
Uric acid μmol/L | 73.10 (±41.15) | 5.00–158.00 | 104.60 (±43.03) | 36.00–200.00 | 0.02 | −2.33 | 37.00 | – | |
Triglycerides mmol/L | 2.69 (±0.89) | 0.60–4.20 | 4.24 (±2.78) | 0.20–9.20 | 0.03 | −2.37 | 23.00 | – | |
Bile acids μmol/L | 5.77 (±4.80) | 0.51–20.38 | 2.35 (±3.77) | 0.00–14.77 | <0.01 | – | – | 300.00 |
Significant differences are indicated by bold text in the P-value column.
No differences in PCV existed between the two trap sites, with a range of 23–42% for DP and 22–38% for IW (P = 1). Immature erythrocytes were observed in all blood smears and comprised 1–2% of the entire erythrocyte population. Estimates of thrombocyte numbers were not possible due to clumping in most of the blood smears. The morphology of all cellular components of the blood was typical of that previously described for other chelonian species (Scheelings and Rafferty 2012; Campbell and Grant 2022a). No haemoparasites were observed in any of the blood smears examined.
The TWCC ranged from 4.9 to 26.2 × 109/μL in turtles from DP and 4.6 to 26.87 × 109/μL in turtles from IW (Table 1), but this was not statistically different. The only significant difference in leukocytes between the two groups was basophil numbers, which were significantly higher in animals from DP than IW (P = 0.04, W = 272) (Table 1, Fig. 3a).
Boxplots displaying differences in haematology and biochemistry values between turtles in relation to capture site, including (a) basophils, (b) glucose, (c) bile acids, (d) uric acid and (e) triglycerides.
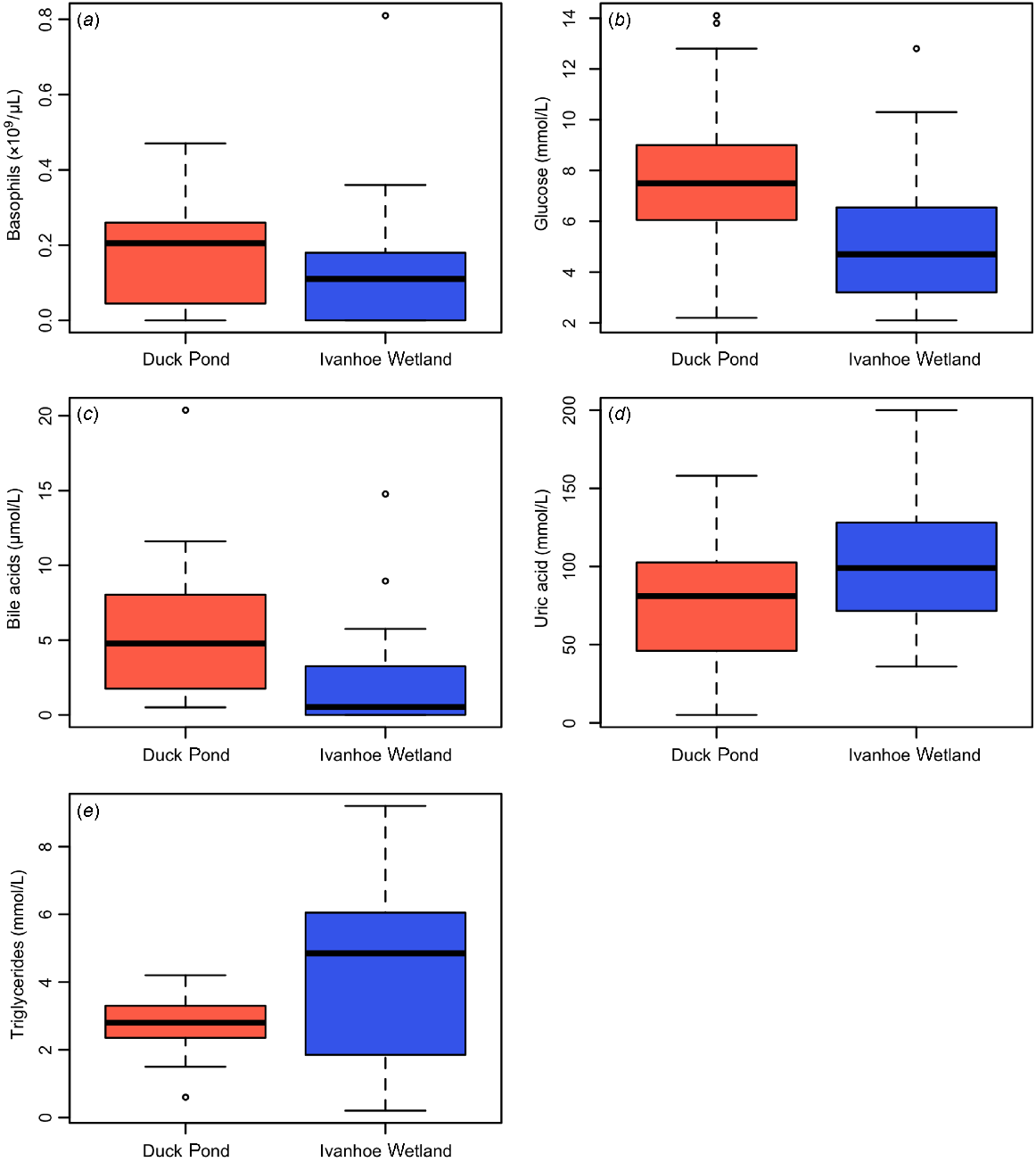
Glucose (P = 0.02, W = 273) and bile acids (P < 0.01, W = 300) were significantly higher in turtles from DP (Table 1, Figs 3b, c), and uric acid (P = 0.02, t = −2.33, d.f. = 37) and triglycerides (P = 0.03, t = −2.37, d.f. = 23) were significantly higher in turtles from IW (Table 1, Figs 3d, e). There were no differences observed in any other biochemistry parameters measured from either population.
Linear regression analysis revealed a negative correlation between glucose and mass (P < 0.01, r2 = 0.26, Adj r2 = 0.24, F = 12.84) (Table 2), with heavier turtles having a relatively lower blood glucose level (Fig. 4a). There was also a negative correlation between glucose and SCL (P < 0.01, r2 = 0.19, Adj r2 = 0.16, F = 8.53) (Table 2), with longer turtles having a relatively lower blood glucose level (Fig. 4b). Finally, there was a negative correlation between glucose and Wr (P < 0.01, r2 = 0.2, Adj r2 = 0.18, F = 9.36) (Table 2), with turtles in better body condition having lower blood glucose levels (Fig. 4c). No other relationships were discovered between any of the other morphometric data and the haematology or biochemistry values in these turtles (Table 2).
Analyte | Mass | SCL | Wr | ||||||||||
---|---|---|---|---|---|---|---|---|---|---|---|---|---|
P | r2 | Adj r2 | F | P | r2 | Adj r2 | F | P | r2 | Adj r2 | F | ||
PCV | 0.25 | 0.03 | <0.01 | 1.34 | 0.37 | 0.02 | <−0.01 | 0.82 | 0.52 | 0.01 | <−0.05 | 0.42 | |
TWCC | 0.44 | 0.01 | −0.01 | 0.60 | 0.31 | 0.03 | <0.01 | 1.06 | 0.71 | <0.01 | −0.02 | 0.14 | |
Heterophils | 0.21 | 0.04 | 0.01 | 1.62 | 0.13 | 0.06 | 0.03 | 2.38 | 0.58 | <0.01 | −0.02 | 0.31 | |
Monocytes | 0.35 | 0.02 | <−0.01 | 0.88 | 0.42 | 0.02 | <−0.01 | 0.66 | 0.57 | <0.01 | −0.02 | 0.33 | |
Lymphocytes | 0.88 | <0.01 | −0.02 | 0.02 | 0.73 | <0.01 | −0.02 | 0.12 | 0.88 | <0.01 | −0.02 | 0.02 | |
Eosinophils | 0.76 | <0.01 | −0.02 | 0.10 | 0.81 | <0.01 | −0.02 | 0.06 | 0.64 | <0.01 | −0.02 | 0.22 | |
Basophils | 0.46 | 0.01 | −0.01 | 0.56 | 0.24 | 0.03 | 0.01 | 1.41 | 0.09 | 0.07 | 0.05 | 2.90 | |
H:L | 0.30 | 0.03 | <0.01 | 1.10 | 0.22 | 0.04 | 0.01 | 1.57 | 0.40 | 0.02 | <−0.01 | 0.72 | |
Albumin | 0.47 | 0.01 | −0.01 | 0.53 | 0.49 | 0.01 | −0.01 | 0.48 | 0.98 | <0.01 | −0.02 | <0.01 | |
Amylase | 0.15 | 0.05 | 0.03 | 2.13 | 0.17 | 0.05 | 0.02 | 1.93 | 0.99 | <0.01 | −0.03 | <0.01 | |
AST | 0.74 | <0.01 | −0.02 | 0.11 | 0.70 | <0.01 | −0.02 | 0.15 | 0.73 | <0.01 | −0.02 | 0.12 | |
Calcium | 0.67 | <0.01 | −0.02 | 0.18 | 0.93 | <0.01 | −0.03 | <0.01 | 0.24 | 0.04 | 0.01 | 1.44 | |
Cholesterol | 0.90 | <0.01 | −0.02 | 0.01 | 0.75 | <0.01 | −0.02 | 0.10 | 0.34 | 0.02 | <−0.01 | 0.93 | |
CK | 0.20 | 0.04 | 0.02 | 1.68 | 0.11 | 0.06 | 0.04 | 2.61 | 0.18 | 0.05 | 0.02 | 1.90 | |
GLDH | 0.67 | <0.01 | −0.02 | 0.18 | 0.84 | <0.01 | −0.03 | 0.04 | 0.07 | 0.09 | 0.06 | 3.40 | |
Glucose | <0.01 | 0.26 | 0.24 | 12.84 | <0.01 | 0.19 | 0.16 | 8.53 | <0.01 | 0.20 | 0.18 | 9.36 | |
Total protein | 0.57 | <0.01 | −0.02 | 0.33 | 0.70 | <0.01 | −0.02 | 0.15 | 0.78 | <0.01 | −0.02 | 0.08 | |
Urea | 0.73 | <0.01 | −0.02 | 0.12 | 0.36 | 0.02 | <−0.01 | 0.87 | 0.12 | 0.06 | 0.04 | 2.45 | |
Uric acid | 0.90 | <0.01 | −0.03 | 0.01 | 0.73 | <0.01 | −0.02 | 0.12 | 0.86 | <0.01 | −0.32 | 0.03 | |
Triglycerides | 0.27 | 0.03 | <0.01 | 1.27 | 0.50 | 0.01 | −0.01 | 0.45 | 0.06 | 0.09 | 0.07 | 3.88 | |
Bile Acids | 0.42 | 0.02 | <−0.01 | 0.67 | 0.34 | 0.02 | <−0.01 | 0.94 | 0.45 | 0.01 | −0.01 | 0.57 |
Significant values are indicated by bold text.
Scatter plots displaying the relationships between (a) glucose and mass, (b) glucose and straight carapace length and (c) glucose and relative weight. The black line represents the regression line and demonstrates that as turtles get heavier, longer and increase in body condition, serum glucose levels decrease.
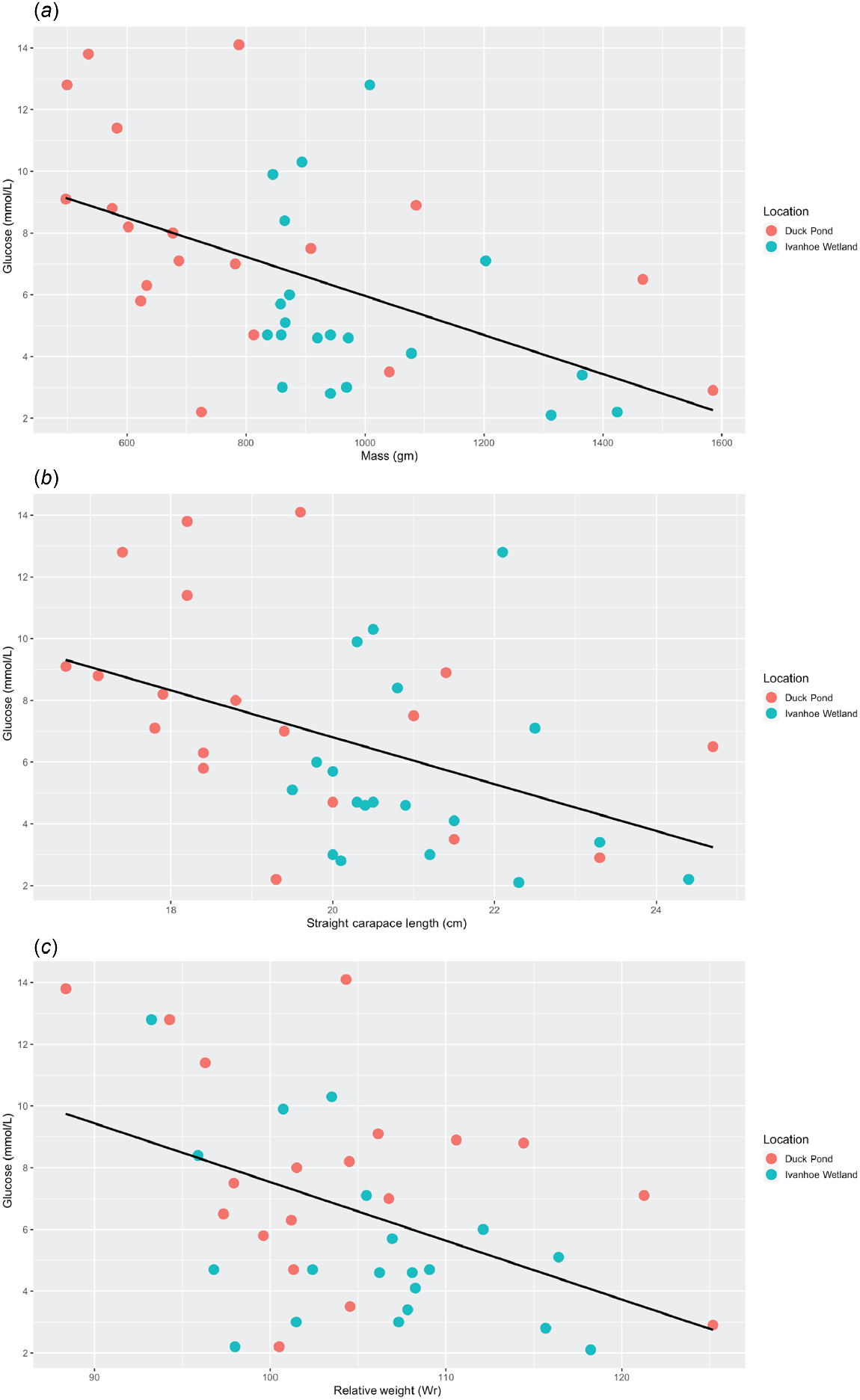
Discussion
In this investigation, the blood parameters of wild eastern longneck turtles captured from two different waterbodies are reported. The haematology results of this study are similar to a previous investigation in this species, in which lymphocytes are the predominate leukocyte, followed by heterophils (Scheelings and Rafferty 2012). This is not a consistent finding in chelonians, where in some species, lymphocytes and heterophils are equally abundant, whereas in others, either leukocyte type may predominate (Klaphake et al. 2018). In reptiles, the primary function of the heterophil is phagocytosis, but unlike mammalian neutrophils, they have limited oxidative ability in their bactericidal function (Campbell and Grant 2022a). Reptilian lymphocytes function in a similar manner to other vertebrates and may account for up to 80% of the normal leukogram in some species (Campbell and Grant 2022a). The only statistically significant haematological difference between the two sites was the higher number of basophils observed in animals from DP in comparison with those from IW. The physiological and clinical significance of this difference was not clear based on the preliminary nature of the examinations conducted on the trapped turtles. Cytochemical and ultrastructural studies have shown that reptilian basophils probably function similarly to mammalian basophils by processing surface immunoglobulins and releasing histamine upon degranulation (Campbell and Grant 2022a). In some reptiles, basophilia has been associated with parasitic and viral infections (Sypek and Borysenko 1988). If this investigation had incorporated methods to take this into account, differences in pathogen load may have been identified between the two populations, which may account for the observed variance in basophil numbers.
Biochemical variations between groups in this study included glucose, uric acid, triglycerides and bile acids. In this investigation, turtles from DP had a significantly higher serum glucose level than those from IW (Fig. 3a). Given the high trapping density within this pond, it is possible that this increase in glucose was a stress response due to the high volume of animals in each trap. Mean serum glucose levels in this cohort approximated values seen in other stressed freshwater turtles (Ray and Maiti 2001; Paramita Ray et al. 2008). Additionally, animals from DP also had significantly lower triglyceride levels, which has been identified as an indicator of stress in reptiles (Martínez Silvestre 2014). However, there were no cellular responses typically associated with stress in this taxon such as a change in the H:L ratio, increased TWCC or a relative heterophilia (Al-Johany and Haffor 2005; Johnstone et al. 2012; Stacy et al. 2017). Quantification of stress hormones was not performed, so no definitive statements regarding physiological stress can be made. A second plausible explanation for the higher serum glucose levels in turtles from DP is an increase in food availability in this waterbody. Given that DP is located on a popular walking track, it is possible that supplementary feeding by ‘Good Samaritan’ members of the public occurs at this site. However, if supplementary feeding did occur, it may be expected that triglyceride levels would also increase – as has been shown to occur in other species of chelonians with anthropogenic interference (Stewart et al. 2016). It is also possible that the large waterbird population that frequents the pond provides additional resources for the turtles to utilise. Further investigation into the ecology of the waterbodies in Darebin Parklands is warranted in order to elucidate the factors that influence resource availability at this location.
In this investigation, serum glucose levels decreased as mass, SCL and Wr of turtles increased. Conversely, in Galápagos marine iguanas (Amblyrhynchus cristatus), increased glucose levels are associated with an increase in body length, which is presumed to occur as a result of larger individuals being capable of longer foraging excursions (Lewbart et al. 2015). An elevation in blood glucose concentration has also been reported in captive mugger crocodiles (Crocodylus palustris) as animals mature (Stacy and Whitaker 2000). It may be that as turtles increase in size towards a maximal mass, SCL or Wr, their growth rate and caloric requirements decrease, and consequently their foraging requirements are lower, resulting in a relative decrease in serum glucose levels. Further study into the foraging behaviours of eastern longneck turtles is required in order better understand this observation.
Interpretation of the disparity in uric acid and bile acids between the two turtle populations is difficult due to the uncertainty of the clinical significance of these metabolites in reptiles. Although an increase in bile acids has been demonstrated in a variety of species with chronic liver disease (Girling and Fraser 2011; Knotek et al. 2011a, 2011b), this is not a consistent finding in all reptiles with substantial hepatic pathology (Giuseppe et al. 2017). Similarly, the validity of uric acid as a method for assessing renal function in reptiles is questionable due to the fact that it is neither sensitive nor specific for renal disease and may increase in association with severe dehydration, a recent carnivorous meal or decreased temperature (Heatley and Russell 2019). Therefore, the biological significance of these observed differences remains unknown at this time.
An important finding in this study was that location can have a significant effect on multiple parameters in freshwater chelonians, even over relatively short distances. In this investigation, the two waterbodies where turtles were trapped are separated by roughly 200 m. Eastern longneck turtles are a highly mobile species, capable of long terrestrial excursions (Kennett et al. 2009), meaning that movement between these ponds is likely to occur under favourable conditions. When turtles migrate it is unknown whether blood parameters change in response to their new surroundings, or the time frame in which this may occur. Although turtles were not permanently marked in this study, there is a very high degree of confidence that resampling of animals did not occur; the weather was hot and dry over the period in which turtles were trapped (turtle migration typically occurs during wet weather) (Kennett et al. 2009), turtle movement was not observed by parks staff and all morphometric data were unique for each individual. It is plausible that differences in habitat quality between the water bodies were responsible for the observed disparities in morphology and blood parameters from the two sets of turtles, but no attempt was made to assess this. In future investigations of this nature, a comprehensive assessment of environmental parameters such as water quality and food availability should be incorporated into the analysis. The results of this investigation may have implications on how clinicians utilise blood reference ranges to assess sick or injured turtles and brings into question the validity of using these data when they are so highly variable. This is especially pertinent in a widely disturbed species such as the eastern longneck turtle, where a vast array of climatic and environmental conditions is likely to exert a substantial physiological effect on individuals.
Conclusion
Body condition (Wr) and haematology and biochemistry values were determined for wild eastern longneck turtles from two different waterbodies within the same parklands. Significant differences were observed in Wr, basophils, glucose, uric acid, triglycerides and bile acids between the two populations. It was also discovered that serum glucose levels in this species decreases as the animal increases in length, mass and body condition. These results highlight the importance of considering locality when interpreting biological data from wild-sourced reptiles.
Data availability
The data that support this study will be shared upon reasonable request to the corresponding author.
Declaration of funding
Dr Scheelings is supported by a McKenzie Postdoctoral Fellowship at the University of Melbourne.
Acknowledgements
I thank the staff of Darebin Creek Management Committee Peter Wiltshire and Kim Davis for access to field sites and logistical help. I also thank Angela Simms, Tilli Beaumont, Dan Guinto and Jasper White for assistance in placing traps and processing turtles. Lastly, I thank Katie Howard (ARI, DEECA) for providing assistance in calculating condition scores from data that were collected as part of The Living Murray Program.
References
Al-Johany AM, Haffor AS (2005) Increased antioxidant and white blood cell counts and decreased free radical production during mild heat stress in Uromastyx egyptius. Journal of Medical Sciences 5, 311-315.
| Crossref | Google Scholar |
Andrade DV, Brito SP, Toledo LF, Abe AS (2004) Seasonal changes in blood oxygen transport and acid-base status in the tegu lizard, Tupinambis merianae. Respiratory Physiology & Neurobiology 140, 197-208.
| Crossref | Google Scholar | PubMed |
Bielli M, Nardini G, Di Girolamo N, Savarino P (2015) Hematological values for adult eastern Hermann’s tortoise (Testudo hermanni boettgeri) in semi-natural conditions. Journal of Veterinary Diagnostic Investigation 27, 68-73.
| Crossref | Google Scholar | PubMed |
Bryant GL, Fleming PA, Twomey L, Warren KA (2012) Factors affecting hematology and plasma biochemistry in the southwest carpet python (Morelia spilota imbricata). Journal of Wildlife Diseases 48, 282-294.
| Crossref | Google Scholar | PubMed |
Day RD, Segars AL, Arendt MD, Lee AM, Peden-Adams MM (2007) Relationship of blood mercury levels to health parameters in the loggerhead sea turtle (Caretta caretta). Environmental Health Perspectives 115, 1421-1428.
| Crossref | Google Scholar | PubMed |
de la Navarre BJS (2006) Common procedures in reptiles and amphibians. Veterinary Clinics of North America: Exotic Animal Practice 9, 237-267.
| Crossref | Google Scholar |
Eva C, Sandra R, Zora K, Vaclav C, Zdenek K (2022) Biochemical blood profile in 20 female veiled chameleons (Chamaeleo calyptratus) aged 7, 9 and 11 months. Journal of Exotic Pet Medicine 40, 72-75.
| Crossref | Google Scholar |
Flint M, Limpus DJ, Limpus CJ, Patterson-Kane JC, Eales JA, Mills PC (2011) Biochemical and hematological reference intervals for Krefft’s turtles Emydura macquarii krefftii from the Burnett River Catchment, Australia. Diseases of Aquatic Organisms 95, 43-48.
| Crossref | Google Scholar | PubMed |
Girling SJ, Fraser MA (2011) Cannabis intoxication in three green iguanas (Iguana iguana). Journal of Small Animal Practice 52, 113-116.
| Crossref | Google Scholar | PubMed |
Giuseppe MD, Oliveri M, Morici M, Rossi G, Spadola F (2017) Hepatic encephalopathy in a red-tailed boa (Boa constrictor imperator). Journal of Exotic Pet Medicine 26, 96-100.
| Crossref | Google Scholar |
Howard JG, Jaensch S (2021) Haematology and plasma biochemistry reference intervals in wild bearded dragons (Pogona vitticeps). Australian Veterinary Journal 99, 236-241.
| Crossref | Google Scholar | PubMed |
Johnson RSP, Harlow PS, Phillips CA, Hall EJS (2018) Baseline morphometric, haematological and plasma biochemical parameters in free-ranging eastern water dragons (Intellagama lesueurii lesueurii). Australian Veterinary Journal 96, 450-457.
| Crossref | Google Scholar | PubMed |
Johnstone CP, Reina RD, Lill A (2012) Interpreting indices of physiological stress in free-living vertebrates. Journal of Comparative Physiology B 182, 861-879.
| Crossref | Google Scholar |
Knotek Z, Dorrestein GM, Hrdá A, Tomek A, Proks P, Knotková Z, Jekl V, Lewis W (2011a) Hepatocellular carcinoma in a green iguana – a case study. Acta Veterinaria Brno 80, 243-247.
| Crossref | Google Scholar |
Knotek Z, Knotková Z, Trnková Š, Dorrestein GM, Lewis W (2011b) Chronic liver disease and subchronic nephritis in a male warty chameleon (Furcifer verrucosus) with transient hyperglycaemia – case report. Acta Veterinaria Brno 80, 397-400.
| Crossref | Google Scholar |
Lewbart GA, Hirschfeld M, Brothers JR, Munoz-Perez JP, Denkinger J, Vinueza L, Garcia J, Lohmann KJ (2015) Blood gases, biochemistry and haematology of Galápagos marine iguanas (Amblyrhynchus cristatus). Conservation Physiology 3, cov034.
| Crossref | Google Scholar |
Maceda-Veiga A, Figuerola J, Martinez-Silvestre A, Viscor G, Ferrari N, Pacheco M (2015) Inside the Redbox: applications of haematology in wildlife monitoring and ecosystem health assessment. Science of The Total Environment 514, 322-332.
| Crossref | Google Scholar | PubMed |
Martínez Silvestre A (2014) How to assess stress in reptiles. Journal of Exotic Pet Medicine 23, 240-243.
| Crossref | Google Scholar |
Paramita Ray P, Sarkar S, Chaudhuri-Sengupta S, Maiti BR (2008) Adrenomedullary and glycemic alterations following diverse stress in soft-shelled turtles Lissemys punctata punctata Bonnoterre. Endocrine Research 33, 119-127.
| Crossref | Google Scholar | PubMed |
Rafferty AR, Scheelings TF, Foley LJ, Johnstone CP, Reina RD (2014) Reproductive investment compromises maternal health in three species of freshwater turtle. Physiological and Biochemical Zoology 87, 411-419.
| Crossref | Google Scholar | PubMed |
Ray PP, Maiti BR (2001) Adrenomedullary hormonal and glycemic responses to high ambient temperature in the soft-shelled turtle, Lissemys punctata punctata. General and Comparative Endocrinology 122, 17-22.
| Crossref | Google Scholar | PubMed |
Rosser MF (2022) Clinical pathology of freshwater turtles. Veterinary Clinics of North America: Exotic Animal Practice 25, 785-804.
| Crossref | Google Scholar | PubMed |
Scheelings TF, Rafferty AR (2012) Hematologic and serum biochemical values of gravid freshwater Australian chelonians. Journal of Wildlife Diseases 48, 314-321.
| Crossref | Google Scholar | PubMed |
Scheelings TF, Moore RJ, Van TTH, Klaassen M, Reina RD (2020) No correlation between microbiota composition and blood parameters in nesting flatback turtles (Natator depressus). Scientific Reports 10, 8333.
| Crossref | Google Scholar | PubMed |
Stacy BA, Whitaker N (2000) Hematology and blood biochemistry of captive mugger crocodiles (Crocodylus palustris). Journal of Zoo and Wildlife Medicine 31, 339-347.
| Crossref | Google Scholar | PubMed |
Stacy NI, Field CL, Staggs L, MacLean RA, Stacy BA, Keene J, Cacela D, Pelton C, Cray C, Kelley M, Holmes S, Innis CJ (2017) Clinicopathological findings in sea turtles assessed during the Deepwater Horizon oil spill response. Endangered Species Research 33, 25-37.
| Crossref | Google Scholar |
Stewart K, Norton T, Mohammed H, Browne D, Clements K, Thomas K, Yaw T, Horrocks J (2016) Effects of “swim with the turtles” tourist attractions on green sea turtle (Chelonia mydas) health in Barbados, West Indies. Journal of Wildlife Diseases 52, S104-S117.
| Crossref | Google Scholar | PubMed |
Van Dyke JU, Ferronato BdO, Spencer R-J (2018) Current conservation status of Australian freshwater turtles. Australian Journal of Zoology 66, 1-3.
| Crossref | Google Scholar |