Intraspecific variation in behaviour and ecology in a territorial agamid, Ctenophorus fionni
Benjamin Carl Wilson A , José Antonio Ramos
A Animal Behaviour Group, Department of Ecology, Environment and Evolution, La Trobe University, Bundoora, Vic. 3086, Australia.
B Corresponding author. Email: j.ramos@latrobe.edu.au
Australian Journal of Zoology 68(2) 85-97 https://doi.org/10.1071/ZO20091
Submitted: 9 November 2020 Accepted: 6 May 2021 Published: 3 June 2021
Journal Compilation © CSIRO 2020 Open Access CC BY-NC-ND
Abstract
Intraspecific variation as a way to explore factors affecting the evolution of species traits in natural environments is well documented, and also important in the context of preserving biodiversity. In this study, we investigated the extent of behavioural, morphological and ecological variation in the peninsula dragon (Ctenophorus fionni), an endemic Australian agamid that displays extensive variation in colour across three allopatric populations. The aims of the study were to quantify variation across the different populations in terms of the environment, morphometric characteristics and behaviour. We found population level differences in habitat structure and encounter rates. Adult body size of C. fionni, as well as a range of morphometric traits, differed between populations, as well as the frequency of social interactions, which appears to be related to population density and abundance. Analysis of communicative signals showed differences between the southern and central populations, which appear consistent with variations in response to environmental differences between study sites. The findings of the present study, coupled with previous work examining colour variation in this species, show that the three populations of C. fionni have likely undergone substantial differentiation, and would make an interesting study system to explore trait variation in more detail.
Keywords: Agamidae, allopatry, animal communication, behavioural ecology, Ctenophorus fionni, intraspecific variation, lizard, signalling, social interactions, territoriality.
Introduction
Spatial variation in environmental factors can lead to changes in the most optimal phenotype within the same species (Calsbeek et al. 2007; Kaliontzopoulou et al. 2010; Bolnick et al. 2011). Such intraspecific variation acts on many phenotypic traits, including behaviour (Herczeg and Välimäki 2011) and morphology (Calsbeek and Smith 2007). Intraspecific variation can be driven by both sexual and natural selection (Biro et al. 2005), and has been used to explore environmental factors driving the function of traits, as well as the evolutionary processes shaping them (Kaliontzopoulou et al. 2010; Ramos and Peters 2017a). Understanding intraspecific variation has recently gained attention as a potential tool to manage species against anthropogenic threatening processes by considering population level differences in tolerance to different threats (Kelly and Phillips 2016; Herrando-Pérez et al. 2019). This indicates the need for continued investigation in this field, particularly with respect to taxa that are poorly understood.
Behaviour can be highly plastic and may be shaped by a wide range of biotic and abiotic factors, such as predation pressure (Herczeg and Välimäki 2011), food availability (Biro et al. 2005), climate (Dell et al. 2011), resource competition (Herczeg et al. 2012), and habitat (Ramos and Peters 2017a). Understanding which environmental factors are driving behavioural change in a species is complicated by the fact that one environmental factor may result in numerous behavioural shifts (Magurran and Seghers 1991); conversely, many environmental factors may manifest themselves in only one behavioural adaptation (Herczeg and Välimäki 2011). Manipulating the environment (e.g. modifying the surrounding vegetation or environmental conditions) to determine the effect of different levels of variation is impractical given the number of relevant variables and the extent of their influence (although see Bian et al. 2018), and changes in phenotypic traits may take a long time to become detectible. Intraspecific variation represents a natural experiment in this regard, as one can determine how behaviour might change between populations due to selection and plasticity, and then place them in the context of the differing environment.
Intraspecific resource competition is an important behavioural driver in a range of taxa (reviewed by Svanbäck and Bolnick 2007). This often leads to an increase in intraspecific aggression, which manifests as male–male competition for resources and/or mates (Hews 1993; Peters et al. 2016). Such competition has also been observed in females, particularly in more resource limited environments (Wu et al. 2018). Increased body size is a common morphological adaptation to increased aggression and territoriality (Calsbeek and Smith 2007), with larger individuals out-competing smaller conspecifics (Persson 1985), which can also result in demographic changes (Howard 1981; Johnston 2011). Physiological and morphological adaptations represent a clear selective advantage to maintaining a territory. However, violent interactions are costly to all combatants, leading many taxa to develop non-violent behavioural displays to mediate physical aggression (Lailvaux and Irschick 2007). Such signals may seek to either advertise ownership of a territory or project a message designed to showcase fitness in order to avoid violent exchange (Lailvaux and Irschick 2007).
Territorial signalling is constrained by physiology, environmental factors and the sensory systems of receivers. Physiological and morphological factors, such as vocal amplitude (Podos 2001) or dewlap length (Ord et al. 2015), pose a physical limit to signal projection, while factors such as environmental background noise may mask or distort the signal (Peters et al. 2008; Steinberg et al. 2014; Számadó and Hemmi 2015; Ramos and Peters 2017a). Effective signalling requires detectable signals. However, increasing conspicuousness against the environmental background may incur an increased risk of predation, which may constrain signal contrast (Gibbons and Lillywhite 1981; Stuart-Fox et al. 2003). These factors can lead to rapid and high diversification amongst signal strategies (Tinghitella 2008).
Lizards are excellent models for understanding the evolution of both colour- and movement-based visual signalling strategies (Fleishman 1988; Nicholson et al. 2007; Fleishman et al. 2011, 2017; Peters et al. 2016; Ramos and Peters 2016). Movement from wind-blown plants has been documented to be an important source of environmental noise that may impact lizard movement-based communication (Peters et al. 2008), leading to alterations in speeds, durations and amplitudes of motion signals (Peters et al. 2007; Ord and Stamps 2008; Ramos and Peters 2017a). Different vegetation densities, as well as different plant structures, respond differently to wind (Peters et al. 2008), which in turn alters the environment through which signals must be projected (Peters et al. 2007; Ramos and Peters 2017a). This has the potential to drive significant intraspecific variation in signal structure between species widely distributed across a varying landscape.
Species that range over a wide area or that display allopatric distribution patterns often exhibit interpopulation morphological variation (Stock et al. 2000; Calsbeek et al. 2007). This can be a result of genetic drift or of local adaptation to novel environments (Calsbeek et al. 2007; Calderón-Espinosa et al. 2013). Such species are informative as morphological variation between populations suggests the possibility of further phenotypic or ecological differentiation. One such species is the peninsula dragon (Ctenophorus fionni) (Gibbons and Lillywhite 1981; Stuart‐Fox et al. 2004). This small agamid lizard is endemic to the Eyre Peninsula in South Australia (Fig. 1), and is well known for its territoriality and sexual dimorphism (Stuart‐Fox et al. 2004). Males are usually bigger and much more conspicuously coloured than females, which have a more cryptic colouration that tends to match their local substrate well (Fig. 1) (Stuart‐Fox et al. 2004). This species also displays a high degree of geographic variation in colour (Fig. 1a–c) (Stuart‐Fox et al. 2004), body size (Houston 1974) and territorial display behaviour (Gibbons 1979). Variation in predation rate has been suggested as the main driver of this polymorphism (Gibbons and Lillywhite 1981; Stuart‐Fox et al. 2004). Furthermore, the chemical compositions of the pheromones that are used in social communication by this species vary between populations (La Nafie et al. 1995). Thus, evidence suggests that this species is undergoing substantial divergence between its three populations. Interestingly, we know very little about interpopulation variation in ecology, physiology and behaviour in general. As with other members of the Ctenophorus decresii complex, this species is known to engage in intricate territorial interactions, including some of the most elaborate movement-based signalling performed by any Australian reptile (Gibbons 1979; Ord et al. 2001; Ramos and Peters 2016). The territorial displays performed by this species are composed of three main sequential motor patterns beginning with limb waving, followed by hind leg push ups coupled with tail coiling, and ending with head bobbing (Gibbons 1979). Signal diversification has been shown to generally occur rapidly under selection (Tinghitella 2008), so it is probable that the communicative behaviour of C. fionni has also diverged between the different populations.
![]() |
The objective of this study was to gather further data on the extent to which the three populations of C. fionni differ in aspects of behaviour and morphology (other than colouration), and to place these differences in the context of the environments they inhabit. We addressed four specific aims:
-
to quantify variation in the environment across the different populations through analysis of vegetation and substrate composition;
-
to compare relative encounter rates of C. fionni at different sites as an index of relative population density;
-
to determine whether morphological features of C. fionni differ between sampled populations;
-
to explore variation in the behaviour of C. fionni between populations.
We expected to find substantial variation in substrate and vegetation between the three sampled populations due to known differences in geology and climatic factors. It was unclear how population densities of C. fionni may differ between sites; however, studies have shown that variation in density and abundance of lizard species are often correlated with habitat variation (Monasterio et al. 2010). Intraspecific variation in morphometric traits has been observed to correlate with differences in habitat and microhabitat use in a range of lizard species (e.g. many species of Caribbean anoles: Calsbeek et al. 2007), so we also expected population level variation in the morphology of C. fionni. In view of anticipated climate and habitat differences between the three populations, we also expected variation in the proportion of time lizards engaged in different behaviours. Social interactions are also likely to be influenced by the population density of C. fionni (Stamps 1977). Communication strategies are constrained by the environment in which they are used, with wind-blown vegetation known to cause changes to the structure of signal displays in a range of social lizards (Peters et al. 2008; Ramos and Peters 2017a). We hypothesised that signal structure would vary between populations in a manner consistent with differences in vegetation composition. More specifically, we expected populations exposed to noisier environments to produce faster display movements. Although we did not measure motion noise, we expected the coastal habitat of Lincoln National Park (NP) would provide a noisier environment and lizards therein would produce faster displays (Ord et al. 2007).
Materials and methods
Study sites
Ctenophorus fionni is distributed widely on the Eyre Peninsula in South Australia, and shows considerable geographic variation in colour, body size and behaviour (Fig. 1) (Houston 1974; Gibbons and Lillywhite 1981). Study site selection was based on records from the Atlas of Living Australia (2020), and advice from experienced herpetologists. The Lincoln NP site is near the southern extreme of C. fionni’s geographic range, located on the sea cliffs south of Wanna Lookout on the south-west coast of Lincoln NP (34°53′38.0″S, 135°51′21.1″E). The Gawler Ranges NP site is near the centre of C. fionni’s geographic range and is located in the ephemeral stream known as Policemen’s Point in Gawler Ranges NP (32°35′09.7″S, 135°26′26.3″E). The most northerly site was in an ephemeral stream south-east of the town of Pimba (31°22′23.3″S, 136°51′51.4″E). This species is known to be strongly associated with rock outcrops (Houston 1974), so search effort was concentrated on rocky areas within these sites. All sites were visited twice between November 2018 and January 2019, after mating had occurred (Johnston 1999).
Data collection
Environmental variation was examined by quantifying habitat structure and substrate composition. Relative abundances of C. fionni at the northern, central and southern populations were estimated from encounter rates following standardised search protocols. To quantify behaviour, we undertook focal sampling of multiple individuals, behavioural sampling to document rare but functionally important events, and tethered introductions of ‘intruder’ males to resident males to film communicative displays. Morphometric data were also collected from all three populations.
Habitat structure
For each of the three study populations, 10 quadrats were selected using ArcGIS global imaging software. The coordinates of the quadrats were selected by overlaying a grid onto the study site and selecting 10 evenly spaced 10 m2 quadrats to ensure even coverage across the site (selected coordinates indicate the north-east corner of the quadrat). Vegetation structure was assessed by subdividing each quadrat into 1 m2 cells and using a touch pole placed at the north-west corner of every cell. At each point we recorded the substrate composition as solid rock, loose rock, plant matter or bare ground. Vegetation data were recorded at 0.5 m height increments to a height of 2 m, with vegetation higher than 2 m categorised as a tree. Plant species were grouped into five broad categories based on life form: herb and forb, grass, spinifex (Triodia sp.), shrubs and woody plants. Substrate and vegetation data were analysed separately but using the same analytical approach. An analysis of similarity (ANOSIM) was performed to determine whether these sites varied statistically from each other. A multidimension scaling (MDS) plot using Bray–Curtis similarities was then used to identify discriminating factors between sites. All statistical analyses were performed using PRIMER ver. 7. Solid rock cover and presence of small shrubs (<0.5 m) are considered important ecological factors for this species so an analysis of variance (ANOVA) was performed to specifically look at site variation in these factors.
Encounter rates
We adapted a standard method for sampling birds formulated by Loyn (1985) to sample lizards. The method entailed two people actively searching the study site for the target species for 1 h. As the study sites varied in climate, it was decided that instead of standardising by time of day or temperature, the survey would begin once two lizards had been found within 20 min of each other, as this would indicate that conditions were suitable for lizard activity. Once these two lizards were seen, the two surveyors walked haphazardly across the site as a pair and recorded the sex (male, female or juvenile) and GPS coordinates of every lizard observed. Two surveys were conducted at each study site over consecutive days under similar environmental conditions, with the highest count for males and the highest count for females from either day used in subsequent analyses. We calculated the distance between lizards across the landscape for each site and compared these data between sites using the permutational dispersion (PERMDISP) routine in the permutational analysis of variance (PERMANOVA) add-on, in PRIMER ver. 7 (Clarke and Gorely 2015; Anderson 2017). We examined overall significance as well as pairwise comparisons between sites.
Morphometric measurements
Morphometric measurements were taken for adult lizards of both sexes (sample sizes are provided in the results). Lizards were caught using a noose, made from fishing line and attached to a pole, and measured after being observed or filmed as part of the behavioural and display analyses (see below). An effort was made to catch even numbers of both sexes, but it was not always possible. Weight was measured using Pesola scales (to the nearest 0.5 g). Digital callipers were used to measure snout–vent length (SVL), head length, head height, head width, interlimb, femur, tibia and metacarpal of the rear limb, humerus and radius of the forelimb (to the nearest 0.01 mm). All measurements, except head width, were taken on the left side of the lizard. To determine how the morphology varied between populations, we regressed all morphometric measurements by SVL to remove the effect of body size and performed a multivariate analysis of variance (MANOVA) on the residuals, followed by pairwise comparisons to determine which populations showed divergence. In addition, SVL was analysed separately using an ANOVA. All morphological data analyses were performed in the R statistical environment (R Core Team 2018). Male and female morphometric data were considered separately.
Behavioural analysis
A partial ethogram of the behavioural repertoire of C. fionni was developed based on the literature (Gibbons 1979), pilot observations and discussions with herpetologists (Table S1, Supplementary Material). Focal sampling (focus on one individual per session: Martin and Bateson 2007) was undertaken for a period of 2 h, or until the lizard was lost from view. Continuous recording of activities for this period can be difficult so we used instantaneous time-sampling with a 2 min interval (Martin and Bateson 2007) to record the frequency of occurrence of each behaviour across the 2 h session. We observed three females at each site, while six males were observed at Gawler Ranges NP and four each at Pimba and Lincoln NP. At the start of each session, we recorded the date and time along with estimates for percentage cloud cover and distance to the nearest conspecific. Temperature was recorded every 10 min using a Kestrel 4000 anemometer (Kestrel Meters), which was also used to record the wind speed at the start of the session. Focal sampling data (frequencies) were converted to proportions and analysed separately for each behaviour using the BETAREG function from the BETAREG package (Cribari-Neto and Zeileis 2010) in the R statistical environment. Individuals that did not express a given behaviour were assigned a value of zero and the median temperature for the session. We fitted the models with predictor variables of population, sex and temperature, and transformed the dependent variable to account for absolute zero values, using the formula
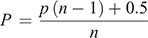
where n is the sample size, p is the raw proportion and P is the transformed proportion (Smithson and Verkuilen 2006). We examined the coefficients and corresponding z-scores for sex and temperature, and for pairwise comparisons for population. Estimated marginal means were generated from the model and plotted for behaviours that yielded a significant effect. The occurrence of rare behaviours is often missed, or misrepresented when using time intervals, therefore behaviour sampling (frequency) was undertaken concurrently with focal sampling. A separate observer recorded any rare behaviours seen in the general population (including the focal lizard), including territorial push up displays, non-aggressive social interactions and predator avoidance. We noted the time of occurrence as well as ambient temperature. Behaviour sampling data were considered separately for males and females and analysed as count data in the R statistical environment (R Core Team 2018) using GLMER from the lme4 package (Bates et al. 2015) and fitting a Poisson error distribution. We used population as a fixed effect and session as a random effect to account for multiple observation sessions with the same population.
Display data collection
Films of male territorial displays were collected in the field at Gawler Ranges NP and Lincoln NP. While effort was made to record displays from Pimba, extreme temperatures coupled with a scarcity of male lizards thwarted this attempt. Displays were elicited by introducing a tethered male via a fishing pole to a free-living male. This lizard was caught from the corresponding populations before commencing filming. To record displays, we followed the protocols of Peters et al. (2016). Briefly, two Sony RX10 iii cameras were used to simultaneously record the displaying individual at 100 frames per second. After the lizard left the scene, a calibration object consisting of 25 non-coplanar points of known distance in relation to each other was placed at the location of the display and without changing any camera settings. This object was later used as reference to calibrate the cameras and combine their footage in order to reconstruct the motion from the displays in three dimensions, using specialised software (see section below).
Display structure
Territorial displays performed by C. fionni consist of three key sequential motor patterns: limb waving, hind-leg push ups coupled with a tail coil and head bobbing (see Ramos and Peters 2016; Movie S1, Supplementary Material). To investigate whether the territorial displays performed by C. fionni varied between populations, we first calculated the duration of each motor pattern within the display (limb wave, push up and head bob) and used an independent samples t-test to compare between populations. We then used 3D reconstruction of displays in Matlab (MathWorks Inc.) to precisely compare movement speeds between populations, using the method outlined by Hedrick (2008) and previously applied to quantifying lizard displays by Peters et al. (2016). Three points were selected on the lizard: the eye, wrist and base of the tail dorsal to the cloacal vent. These points were selected as they represent key components of the motor patterns in the display, and correspond to head bobs, limb waves and hind leg push ups, respectively. All points were manually tracked frame by frame for the course of the full display. The data were then combined along with the calibration coefficient derived from a calibration object to generate a 3D reconstruction of these motor patterns using direct linear transformation to generate x–y–z coordinates of each point in each frame (see Peters et al. 2016 for full details of this approach). Speed was computed separately for each point as the change in position over time. We divided the duration of display sequences into quartiles and obtained an average speed within each quartile for each point. The resultant data were then statistically analysed using a mixed effects model with quartile, population and point as factors and lizards as a random effect. We used the lme function from the nlme package (Pinheiro et al. 2018), where the significance of factors is provided by an F-test.
Results
Environmental variation
Habitat variation
The MDS (Fig. 2a) and ANOSIM (Table 1) for substrate composition showed that Pimba differed from the other two sites, while Gawler Ranges NP and Lincoln NP were not significantly different from each other. A vector overlay used to explore specific environmental factors driving dissimilarities showed solid rock cover as a key factor driving site divergence (Fig. 2a). We explored this further using an ANOVA and found a significant difference across populations (F2,27 = 9.194, P < 0.001) (Fig. 2a inset), with pairwise comparisons suggesting that Gawler Ranges NP had a significantly higher proportion of rock cover than both other sites (P = 0.025 and P < 0.001 for Lincoln NP and Pimba, respectively) but Pimba and Lincoln NP did not differ from each other (P = 0.344).
![]() |
The MDS (Fig. 2b) and ANOSIM (Table 1) for vegetation showed that sites differed in vegetation composition and structure, with Gawler Ranges NP most dissimilar to the other two sites. Much of this dissimilarity appears to be explained by Gawler Ranges NP being associated with branching plants and negatively associated with the lower vegetation categories (e.g. shrubs, herbs, dead wood). The clustering of points for Lincoln NP (Fig. 2b) suggests that it had a more homogeneous vegetation covering than Gawler Ranges NP or Pimba. Taken together, our results indicated a less diverse and closed understorey at Pimba and Lincoln NP, in contrast to more open area at Gawler Ranges NP. Further analysis on the presence of small shrubs confirmed differences across sites (F2,27 = 9.208, P < 0.001) (Fig. 2b inset), with Gawler Ranges NP indeed having less coverage of small shrubs than either of the other sites (P < 0.001 and P = 0.010 for Lincoln NP and Pimba, respectively), but Lincoln NP and Pimba were not significantly different from each other (P = 0.626).
Encounter rates
The maximum number of lizards observed during the timed surveys was substantially higher at Gawler Ranges NP (n = 17: 11 males, 6 females), whereas the number at Lincoln NP (n = 10: 4 males, 4 females, 2 juveniles) and Pimba (n = 8: 0 males, 6 females, 2 juveniles) were more similar. An analysis using the PERMDISP routine in the PERMANOVA add-on for PRIMER revealed significant differences in dispersal (F1,2 = 16.002, P < 0.001), with lizards at Pimba significantly more dispersed in relation to each other than those from Gawler Ranges NP (t = 4.828, d.f. = 23, P < 0.001) or Lincoln NP (t = 3.370, d.f. = 16, P = 0.004), which were not statistically different from each other (t = 1.039, d.f. = 25, P = 0.309).
Morphological and behavioural variation
Morphometric variation
Overall, significant variation in most morphological traits was detected between populations for both male (Table 2) and female (Table 3) C. fionni. Significant variation in SVL was observed between all populations for both male and female lizards, with individuals from Pimba being the largest and those from Lincoln NP the smallest. Males had significantly larger SVL measurements than females at Gawler Ranges NP and Pimba, but not at Lincoln NP (Lincoln NP: t = 1.372, d.f. = 4.654, P = 0.311; Gawler Ranges NP: t = –3.89, d.f. = 14.825, P = 0.002; Pimba: t = –6.419, d.f. = 2.851, P = 0.009). In regressed morphometric traits (e.g. tail length, head height), the Gawler Ranges NP population was the most divergent from the other two groups, differing in most traits from Lincoln NP lizards for both males and females. Differences between Gawler Ranges NP and Pimba lizards were less consistent (Tables 2 and 3 for males and females, respectively). Gawler Ranges NP males generally had larger morphometric features in relation to body size than the other two populations, whereas Lincoln NP males generally had the smallest morphometric traits in relation to body size. Small sample size made any trends difficult to observe in Pimba, but lizards from this population appear to have longer tails in relation to size. Although most trait variations were found to be consistent between males and females, size trends in females were less clear due to a greater degree of within-population variation.
![]() |
![]() |
Focal and behaviour sampling
From the 16 behaviours defined in the ethogram (Table S1, Supplementary Material), only 10 were exhibited by lizards during focal sampling. We focussed our analysis on six of these, selecting those that reflect social interactions (i.e. push up display, head bobbing not part of a display, and limb waving not part of a display) as well as one passive (basking) and active (feeding) behaviour, and scanning (see Table S2 in the Supplementary Material for summary data on all behaviours). The β regression analysis (Table 4) revealed significant differences between sexes with respect to feeding, whereby females engaged in the activity more frequently than males across all populations (Fig. 3a). We also found a significant interaction between head bobbing and population, with individuals from Gawler Ranges NP performing head bobs more frequently than Lincoln NP or Pimba (Fig. 3a). Of the behaviours recorded during behavioural sampling, only push up displays occurred frequently enough to warrant further analysis (Fig. 3b). While females were also observed to perform push up displays, they did not display this behaviour frequently enough to analyse statistically. A generalised linear mixed-effects model for male data showed only that Gawler Ranges NP males performed push up displays significantly more frequently than males from Lincoln NP or Pimba (Z = –2.571, P = 0.010 and Z = –2.723, P = 0.006, respectively).
![]() |
![]() |
Signalling displays
The displays filmed at Gawler Ranges NP (n = 5) and Lincoln NP (n = 4) both matched the broad stereotyped signal movements outlined by Gibbons (1979). Mean durations for components of the territorial displays (i.e. limb waves, push ups and head bobs) at the two populations are shown in Fig. 4a. Lizards from Gawler Ranges NP spent significantly longer performing limb waves (t = 3.110, d.f. = 11.74, P = 0.009), whereas Lincoln NP males spent significantly longer performing the push up component of the displays (t = 2.687, d.f. = 10.18, P = 0.022). Head bobbing did not differ in duration across the two populations. Three-dimensional reconstruction of displays permitted computation of motion speeds (Fig. 4b) between Lincoln NP and Gawler Ranges NP lizards. Lincoln NP males performed their displays at greater speed than Gawler Ranges NP males for all digitised points across the total duration of the display except during limb waving (Fig. 4b). A GLM model was used to compare speeds between populations, quartiles (time) and points. Table 5 summarises the outcome of the model and indicates that all factors and two-way interactions were significant. Visual inspection of the data revealed that a significant population × point interaction is likely attributable to forelimb movements being equivalent in speed for all populations, whereas Lincoln NP speeds are faster for all other points. With respect to variation across time (population × quartiles), Lincoln NP lizards exhibit a steady decline in speed as the display progresses, while Gawler Ranges NP lizards are more consistent in speed throughout the middle part of the display.
![]() |
![]() |
Discussion
Intraspecific variation across populations of C. fionni was observed to be related to the environment, behaviour and morphology. Notwithstanding the preliminary nature of the study, we recorded environmental variation between habitats, both in terms of substrate and vegetation structure, and encounter rates of C. fionni. We also found significant differences in lizard size between populations, as well as several other morphometric traits in both sexes. Through focal sampling, we were able to identify significant differences in behavioural activities, most notably in the proportion of time spent head bobbing at Gawler Ranges NP, whereas behavioural sampling revealed a significantly higher frequency of push up displays by Gawler Ranges NP lizards compared with the Lincoln NP and Pimba populations. Furthermore, detailed aspects of signalling behaviour were observed to differ between populations in terms of signal component duration and speed. Overall, these data are consistent with previous studies of variation in the ecology, morphology and behaviour of this species. However, our results explore how this variation is influenced by characteristics of the habitat and population density.
We observed substantial variation in encounter rates of C. fionni between the different sites, with more lizards detected at Gawler Ranges NP. More precise measurements of population size and density are warranted than were possible in the present study, but our preliminary findings are consistent with environmental factors (Monasterio et al. 2010) and competition (Buckley et al. 2008) influencing lizard population size. Previous work on the species reported a population density estimate of 27 lizards per 10 000 m2 (Johnston 2000), which appears consistent with our own observations at the Gawler Ranges NP population during the encounter rate surveys. A meta-analysis of population densities of lizards from several different environments found lizard body size to be negatively correlated with population density (Buckley et al. 2008). In our study, the lowest encounter rates were in Pimba, where the lizards are larger. Another explanation for differing population densities is the difference in availability of suitable habitat between sites. The site with the greatest proportion of rock substrate, with which these lizards are typically associated (Gibbons 1979), was also where encounter rates were highest (Gawler Ranges NP). We also recorded higher male abundance at Gawler Ranges NP, which is surprising given that sex ratios are generally predicted to be tightly constrained by sexual competition (Greenwood 1980). In at least one other lizard species exhibiting male-biased sex ratios (Lacerta vivipara), males showed increased aggression towards females leading to increased mortality, which in turn increases the male sex ratio bias (Le Galliard et al. 2005). However, males in the present study were not found to display overt intersexual aggression but it is probably because the observations were made after the mating season (i.e. August–October: Johnston 1999). Other factors that could be influencing the sex bias include population density, which in turn is influenced by crevice availability in this species (Johnston 1997), and also environmental temperature, given that C. fionni exhibits temperature-dependent sex determination (Harlow and Price 2000). Additionally, the more conspicuous colouration that males usually display compared with females may play a role in increasing their detectability at this site. Further consideration of the factors affecting sex ratios is important for wildlife conservation and management (Ginsberg and Milner-Gulland 1994; Wedekind 2002; Robertson et al. 2006), and our observations herein suggest that C. fionni may make a good case study for exploring these factors.
This study found variation in morphology between the three populations of C. fionni. Herpetologists and enthusiasts have long been aware of these morphometric differences; however, this information has previously remained mostly unpublished (Greg Johnston, pers. comm. 2018). Intraspecific morphological variation with respect to differences in habitat use has been documented in several lizard species (Aerts et al. 2000; Herrel et al. 2006; Calsbeek et al. 2007; Brecko et al. 2008; Dollion et al. 2017). Arboreal species are particularly well documented as following consistent morphological variation patterns with respect to preferred microhabitat use, such as limb length increasing with branch diameter (Irschick et al. 2005; Calsbeek et al. 2007). Morphological traits may also be linked to social signalling behaviour, as increased size may generate a greater visual contrast and project a more aggressive or clearer signal (Kotiaho et al. 1999; Bertram 2000). Increased body size has been postulated as a product of territoriality in several species (Persson 1985; Calsbeek et al. 2007). We observed significant differences in overall size between the three populations of C. fionni, but to what extent this is a product of aggression and the need to maintain a territory is unclear. Agonistic interactions were most common at Gawler Ranges NP, and while they were found to have proportionally larger morphometric features in proportion to body size, their overall size was smaller than that of Pimba lizards. Further investigation into the factors driving this observed morphometric divergence is warranted, as our preliminary results clearly show interpopulation morphological variation in C. fionni. Alternative explanations of the observed patterns in morphometric variability in this species are genetic drift, the founder effect or population bottlenecks (Nei et al. 1975; England et al. 2003).
The results of the focal and behaviour sampling found a significant increase in two territorial behaviours, head bobs and push up displays, among the Gawler Ranges NP lizards compared with Lincoln NP and Pimba. We postulate that this might reflect the higher abundance observed at this site, despite population density not being statistically different between all sites. This observation is corroborated by several published accounts of increased population density and abundance leading to an increase in the rate of agonistic interactions (Fletcher 2007; Knell 2009; Cooper et al. 2015). In addition, males were more common at Gawler Ranges NP than at the other two sites, which also may have played a role in this increase in agonistic interactions, as it suggests an increased competition for mates and resources (Le Galliard et al. 2005). We observed feeding rates to be significantly higher in females than males across all populations. This could reflect the need to sustain egg development (Rose 1982), although most females would have laid their eggs by this stage (Johnston 1999). Alternatively, it may be reflective of the males’ need to spend more time actively guarding a territory, and therefore less time feeding (Whitham 1986).
Analysis of territorial displays revealed that males from Lincoln NP and Gawler Ranges NP signalled for approximately the same total duration, but prioritised different components within their display and differed in speed characteristics. Males from Gawler Ranges NP performed the opening limb wave component for significantly longer than their Lincoln NP counterparts, and males from Lincoln NP performed the hindleg push up component for significantly longer than those from Gawler Ranges NP. Both species performed the head bob component for a comparable time. Limb waves generated the fastest movements of the sequence, but slowed down rapidly over time and were equivalent across populations. However, lizards from Lincoln NP produced faster hind leg push ups and head bobs, which was consistent with our hypothesis. Could this variation reflect differences in the signalling environment? If so, it adds to a growing body of scientific work suggesting a strong interaction between the communicative behaviour of an animal and the environmental context in which it is situated (Fleishman 1988; Brumm 2004; Wood and Yezerinac 2006; Peters et al. 2007; Ramos and Peters 2017a). Our analysis of the vegetation data has shown that Lincoln NP is strongly associated with low shrubs and strong winds, which generate considerable background noise at the lizard’s plane of view and so may make detection by a receiver more difficult. With this in mind, male lizards from Lincoln NP may be altering their display to improve signal efficacy. Faster movements have been reported previously in this context (Ord et al. 2007), while adjusting relative use of different motor patterns would be a novel finding.
Divergence in communication behaviour has the potential to lead to speciation if populations can no longer interact effectively with each other (Morton 1975; Leal and Fleishman 2004). Results from this study suggest that diversification in signal structure has occurred between two populations of C. fionni. Within-species variation in display structure can be a plastic response to the signalling conditions (West-Eberhard 1989; Peters et al. 2007; Ramos and Peters 2017b). However, some genetic and colour variation has been recorded between these populations, and our results have provided evidence supporting substantial morphological variation. The genetic basis for movement-based signalling is not known for any lizard species, but it is intriguing to consider the possibility of a genetic basis for display variation. Even if signal variation does have a genetic determinant, it may not preclude effective communication as the core stereotyped display (Gibbons 1979) is retained between populations. Barquero et al. (2015) observed that population variation in signal structure, genetics and morphology did not alter rival recognition or aggression in jacky dragons (Amphibolurus muricatus). Barquero et al. (2015) suggest further that the underlying core signalling behaviour of A. muricatus has a genetic basis, with the observed intraspecific variation being the product of behavioural plasticity to local environments. This may also be true for C. fionni, although A. muricatus does not show the same geographic variation in colour between populations that C. fionni does, which may affect recognition between peninsula dragon populations. Chemical cues are important recognition traits in several lizard species (López et al. 2003, 2006; Baeckens et al. 2015), and chemical analysis of femoral gland secretions of C. fionni demonstrated variability between populations (La Nafie et al. 1995). Additional studies to test whether species recognition has been maintained in the peninsula dragon may give further insight into the extent to which this species is undergoing speciation.
In conclusion, we report substantial variation in habitat, encounter rates, morphology and behaviour between the three populations of C. fionni, as well as in the structure of territorial signalling between Lincoln NP and Gawler Ranges NP. We add to an existing body of evidence suggesting that the three populations have undergone divergence, and habitat differences may be a key driving force. Previous studies have found geographic variation in colour, size and body shape (Houston 1974; Stuart‐Fox et al. 2004) in C. fionni populations, and the current study has recorded significant variation in morphology and behaviour and related it to aspects of their environment and relative abundance. Environmental factors are known drivers of intraspecific phenotypic changes, including behaviour (Magurran and Seghers 1991; Dell et al. 2011; Ramos and Peters 2017a). This is because particular phenotypes are better suited for specific habitats or habitat components. The differences we detected in both substrate type and vegetation between habitats could be driving the development of morphological, behavioural and social structure differences between populations, with the vegetation potentially promoting further behavioural and morphological differentiation as a consequence of population density. Clearly, this avenue of research has implications for conservation and population management, but it is also an important tool for understanding how species are responding to changes in their environment, including anthropogenic processes.
Ethics approval
All work was conducted in accordance with the ethical guidelines of La Trobe University under permit AEC18-022, as well as a scientific research permit issued by the South Australian Government Department of Environment and Water (E26771-2).
Conflicts of interest
The authors declare no conflicts of interest.
Declaration of funding
This project was funded by the Australian Research Council Discovery Project scheme (DP170102370).
Acknowledgements
We thank Greg Johnston for sharing his knowledge of the general ecology of our study species. We also thank our field volunteers, Lexi Carroll, Maddy Walsh, and Lachie Burgess, for their assistance during the data collection stage of the project.
References
Aerts, P., Van Damme, R., Vanhooydonck, B., Zaaf, A., and Herrel, A. (2000). Lizard locomotion: how morphology meets ecology. Netherlands Journal of Zoology 50, 261–277.| Lizard locomotion: how morphology meets ecology.Crossref | GoogleScholarGoogle Scholar |
Anderson, M. J. (2017). Permutational multivariate analysis of variance (PERMANOVA). In ‘Wiley StatsRef: Statistics Reference Online’. (Eds N. Balakrishnan, T. Colton, B. Everitt, W. Piegorsch, F. Ruggeri, and J. L. Teugels.) pp. 1–15. (John Wiley.)
Atlas of Living Australia (2020). Species page. Available at http://www.ala.org.au/
Baeckens, S., Edwards, S., Huyghe, K., and Van Damme, R. (2015). Chemical signalling in lizards: an interspecific comparison of femoral pore numbers in Lacertidae. Biological Journal of the Linnean Society 114, 44–57.
| Chemical signalling in lizards: an interspecific comparison of femoral pore numbers in Lacertidae.Crossref | GoogleScholarGoogle Scholar |
Barquero, M. D., Peters, R. A., and Whiting, M. J. (2015). Geographic variation in aggressive signalling behaviour of the jacky dragon. Behavioral Ecology and Sociobiology 69, 1501–1510.
| Geographic variation in aggressive signalling behaviour of the jacky dragon.Crossref | GoogleScholarGoogle Scholar |
Bates, D., Maechler, M., Bolker, B., and Walker, S. (2015). Fitting linear mixed-effects models using lme4. Journal of Statistical Software 67, 1–48.
| Fitting linear mixed-effects models using lme4.Crossref | GoogleScholarGoogle Scholar |
Bertram, S. (2000). The influence of age and size on temporal mate signalling behaviour. Animal Behaviour 60, 333–339.
| The influence of age and size on temporal mate signalling behaviour.Crossref | GoogleScholarGoogle Scholar | 11007642PubMed |
Bian, X., Chandler, T., Laird, W., Pinilla, A., and Peters, R. A. (2018). Integrating evolutionary biology with digital arts to quantify ecological constraints on vision-based behaviour. Methods in Ecology and Evolution 9, 544–559.
| Integrating evolutionary biology with digital arts to quantify ecological constraints on vision-based behaviour.Crossref | GoogleScholarGoogle Scholar |
Biro, P. A., Post, J. R., and Abrahams, M. V. (2005). Ontogeny of energy allocation reveals selective pressure promoting risk-taking behaviour in young fish cohorts. Proceedings of the Royal Society of London. Series B, Biological Sciences 272, 1443–1448.
| Ontogeny of energy allocation reveals selective pressure promoting risk-taking behaviour in young fish cohorts.Crossref | GoogleScholarGoogle Scholar |
Bolnick, D. I., Amarasekare, P., Araújo, M. S., Bürger, R., Levine, J. M., Novak, M., and Vasseur, D. A. (2011). Why intraspecific trait variation matters in community ecology. Trends in Ecology & Evolution 26, 183–192.
| Why intraspecific trait variation matters in community ecology.Crossref | GoogleScholarGoogle Scholar |
Brecko, J., Huyghe, K., Vanhooydonck, B., Herrel, A., Grbac, I., and Van Damme, R. (2008). Functional and ecological relevance of intraspecific variation in body size and shape in the lizard Podarcis melisellensis (Lacertidae). Biological Journal of the Linnean Society 94, 251–264.
| Functional and ecological relevance of intraspecific variation in body size and shape in the lizard Podarcis melisellensis (Lacertidae).Crossref | GoogleScholarGoogle Scholar |
Brumm, H. (2004). The impact of environmental noise on song amplitude in a territorial bird. Journal of Animal Ecology 73, 434–440.
| The impact of environmental noise on song amplitude in a territorial bird.Crossref | GoogleScholarGoogle Scholar |
Buckley, L. B., Rodda, G. H., and Jetz, W. (2008). Thermal and energetic constraints on ectotherm abundance: a global test using lizards. Ecology 89, 48–55.
| Thermal and energetic constraints on ectotherm abundance: a global test using lizards.Crossref | GoogleScholarGoogle Scholar | 18376546PubMed |
Calderón-Espinosa, M. L., Ortega-León, A. M., and Zamora-Abrego, J. G. (2013). Intraspecific variation in body size and shape in an Andean highland anole species, Anolis ventrimaculatus (Squamata: Dactyloidae). Revista de Biología Tropical 61, 255–262.
| Intraspecific variation in body size and shape in an Andean highland anole species, Anolis ventrimaculatus (Squamata: Dactyloidae).Crossref | GoogleScholarGoogle Scholar | 23894978PubMed |
Calsbeek, R., and Smith, T. B. (2007). Probing the adaptive landscape using experimental islands: density-dependent natural selection on lizard body size. Evolution 61, 1052–1061.
| Probing the adaptive landscape using experimental islands: density-dependent natural selection on lizard body size.Crossref | GoogleScholarGoogle Scholar | 17492960PubMed |
Calsbeek, R., Smith, T. B., and Bardeleben, C. (2007). Intraspecific variation in Anolis sagrei mirrors the adaptive radiation of Greater Antillean anoles. Biological Journal of the Linnean Society 90, 189–199.
| Intraspecific variation in Anolis sagrei mirrors the adaptive radiation of Greater Antillean anoles.Crossref | GoogleScholarGoogle Scholar |
Clarke, K., and Gorely, R. (2015). ‘Primer v7 User Manual/Tutorial.’ (PRIMER-E: Auckland, New Zealand.)
Cooper, W. E., Dimopoulos, I., and Pafilis, P. (2015). Sex, age, and population density affect aggressive behaviors in island lizards promoting cannibalism. Ethology 121, 260–269.
| Sex, age, and population density affect aggressive behaviors in island lizards promoting cannibalism.Crossref | GoogleScholarGoogle Scholar |
Cribari-Neto, F., and Zeileis, A. (2010). Beta regression in R. Journal of Statistical Software 34, 1–24.
| Beta regression in R.Crossref | GoogleScholarGoogle Scholar |
Dell, A. I., Pawar, S., and Savage, V. (2011). Systematic variation in the temperature dependence of physiological and ecological traits. Proceedings of the National Academy of Sciences of the United States of America 108, 10591–10596.
| Systematic variation in the temperature dependence of physiological and ecological traits.Crossref | GoogleScholarGoogle Scholar | 21606358PubMed |
Dollion, A. Y., Measey, G. J., Cornette, R., Carne, L., Tolley, K. A., da Silva, J. M., and Herrel, A. (2017). Does diet drive the evolution of head shape and bite force in chameleons of the genus Bradypodion? Functional Ecology 31, 671–684.
| Does diet drive the evolution of head shape and bite force in chameleons of the genus Bradypodion?Crossref | GoogleScholarGoogle Scholar |
England, P., Osler, G., Woodworth, L., Montgomery, M., Briscoe, D., and Frankham, R. (2003). Effects of intense versus diffuse population bottlenecks on microsatellite genetic diversity and evolutionary potential. Conservation Genetics 4, 595–604.
| Effects of intense versus diffuse population bottlenecks on microsatellite genetic diversity and evolutionary potential.Crossref | GoogleScholarGoogle Scholar |
Fleishman, L. J. (1988). Sensory and environmental influences on display form in Anolis auratus, a grass anole from Panama. Behavioral Ecology and Sociobiology 22, 309–316.
Fleishman, L. J., Loew, E. R., and Whiting, M. J. (2011). High sensitivity to short wavelengths in a lizard and implications for understanding the evolution of visual systems in lizards. Proceedings of the Royal Society of London. Series B, Biological Sciences 278, 2891–2899.
| High sensitivity to short wavelengths in a lizard and implications for understanding the evolution of visual systems in lizards.Crossref | GoogleScholarGoogle Scholar |
Fleishman, L. J., Yeo, A. I., and Perez, C. W. (2017). Visual acuity and signal color pattern in an Anolis lizard. The Journal of Experimental Biology 220, 2154–2158.
| Visual acuity and signal color pattern in an Anolis lizard.Crossref | GoogleScholarGoogle Scholar | 28385798PubMed |
Fletcher, R. J. (2007). Species interactions and population density mediate the use of social cues for habitat selection. Journal of Animal Ecology 76, 598–606.
| Species interactions and population density mediate the use of social cues for habitat selection.Crossref | GoogleScholarGoogle Scholar |
Gibbons, J. R. (1979). The hind leg pushup display of the Amphibolurus decresii species complex (Lacertilia: Agamidae). Copeia 1979, 29–40.
| The hind leg pushup display of the Amphibolurus decresii species complex (Lacertilia: Agamidae).Crossref | GoogleScholarGoogle Scholar |
Gibbons, J. R., and Lillywhite, H. B. (1981). Ecological segregation, color matching, and speciation in lizards of the Amphibolurus decresii species complex (Lacertilia: Agamidae). Ecology 62, 1573–1584.
| Ecological segregation, color matching, and speciation in lizards of the Amphibolurus decresii species complex (Lacertilia: Agamidae).Crossref | GoogleScholarGoogle Scholar |
Ginsberg, J. R., and Milner-Gulland, E. J. (1994). Sex-biased harvesting and population dynamics in ungulates: implications for conservation and sustainable use. Conservation Biology 8, 157–166.
| Sex-biased harvesting and population dynamics in ungulates: implications for conservation and sustainable use.Crossref | GoogleScholarGoogle Scholar |
Greenwood, P. J. (1980). Mating systems, philopatry and dispersal in birds and mammals. Animal Behaviour 28, 1140–1162.
| Mating systems, philopatry and dispersal in birds and mammals.Crossref | GoogleScholarGoogle Scholar |
Harlow, P. S., and Price, A. H. (2000). Incubation temperature determines hatchling sex in Australian rock dragons (Agamidae: genus Ctenophorus). Copeia 2000, 958–964.
| Incubation temperature determines hatchling sex in Australian rock dragons (Agamidae: genus Ctenophorus).Crossref | GoogleScholarGoogle Scholar |
Hedrick, T. L. (2008). Software techniques for two- and three-dimensional kinematic measurements of biological and biomimetic systems. Bioinspiration & Biomimetics 3, 034001.
| Software techniques for two- and three-dimensional kinematic measurements of biological and biomimetic systems.Crossref | GoogleScholarGoogle Scholar |
Herczeg, G., and Välimäki, K. (2011). Intraspecific variation in behaviour: effects of evolutionary history, ontogenetic experience and sex. Journal of Evolutionary Biology 24, 2434–2444.
| Intraspecific variation in behaviour: effects of evolutionary history, ontogenetic experience and sex.Crossref | GoogleScholarGoogle Scholar | 21883614PubMed |
Herczeg, G., Gonda, A., Kuparinen, A., and Merilä, J. (2012). Contrasting growth strategies of pond versus marine populations of nine-spined stickleback (Pungitius pungitius): a combined effect of predation and competition? Evolutionary Ecology 26, 109–122.
| Contrasting growth strategies of pond versus marine populations of nine-spined stickleback (Pungitius pungitius): a combined effect of predation and competition?Crossref | GoogleScholarGoogle Scholar |
Herrando-Pérez, S., Ferri-Yáñez, F., Monasterio, C., Beukema, W., Gomes, V., Belliure, J., and Araújo, M. B. (2019). Intraspecific variation in lizard heat tolerance alters estimates of climate impact. Journal of Animal Ecology 88, 247–257.
| Intraspecific variation in lizard heat tolerance alters estimates of climate impact.Crossref | GoogleScholarGoogle Scholar |
Herrel, A., Joachim, R., Vanhooydonck, B., and Irschick, D. J. (2006). Ecological consequences of ontogenetic changes in head shape and bite performance in the Jamaican lizard Anolis lineatopus. Biological Journal of the Linnean Society 89, 443–454.
| Ecological consequences of ontogenetic changes in head shape and bite performance in the Jamaican lizard Anolis lineatopus.Crossref | GoogleScholarGoogle Scholar |
Hews, D. K. (1993). Food resources affect female distribution and male mating opportunities in the iguanian lizard Uta palmeri. Animal Behaviour 46, 279–291.
| Food resources affect female distribution and male mating opportunities in the iguanian lizard Uta palmeri.Crossref | GoogleScholarGoogle Scholar |
Houston, T. F. (1974). Revision of the Amphibolurus decresii complex (Lacertilia: Agamidae) of South Australia. Transactions of the Royal Society of South Australia 98, 49–60.
Howard, R. D. (1981). Sexual dimorphism in bullfrogs. Ecology 62, 303–310.
| Sexual dimorphism in bullfrogs.Crossref | GoogleScholarGoogle Scholar |
Irschick, D. J., Vanhooydonck, B., Herrel, A., and Meyers, J. (2005). Intraspecific correlations among morphology, performance and habitat use within a green anole lizard (Anolis carolinensis) population. Biological Journal of the Linnean Society 85, 211–221.
| Intraspecific correlations among morphology, performance and habitat use within a green anole lizard (Anolis carolinensis) population.Crossref | GoogleScholarGoogle Scholar |
Johnston, G. R. (1997). Behavioural Ecology of the Peninsula Dragon Lizard, Ctenophorus fionni. Ph.D. Thesis, Flinders University, South Australia.
Johnston, G. R. (1999). Reproductive biology of the Peninsula dragon lizard, Ctenophorus fionni. Journal of Herpetology 33, 694–698.
| Reproductive biology of the Peninsula dragon lizard, Ctenophorus fionni.Crossref | GoogleScholarGoogle Scholar |
Johnston, G. R. (2000). Population density and habitat use in the Peninsula dragon lizard (Ctenophorus fionni). Herpetofauna 30, 34–36.
Johnston, G. R. (2011). Growth and survivorship as proximate causes of sexual size dimorphism in peninsula dragon lizards Ctenophorus fionni. Austral Ecology 36, 117–125.
| Growth and survivorship as proximate causes of sexual size dimorphism in peninsula dragon lizards Ctenophorus fionni.Crossref | GoogleScholarGoogle Scholar |
Kaliontzopoulou, A., Carretero, M. A., and Llorente, G. A. (2010). Intraspecific ecomorphological variation: linear and geometric morphometrics reveal habitat-related patterns within Podarcis bocagei wall lizards. Journal of Evolutionary Biology 23, 1234–1244.
| Intraspecific ecomorphological variation: linear and geometric morphometrics reveal habitat-related patterns within Podarcis bocagei wall lizards.Crossref | GoogleScholarGoogle Scholar | 20406345PubMed |
Kelly, E., and Phillips, B. L. (2016). Targeted gene flow for conservation. Conservation Biology 30, 259–267.
| Targeted gene flow for conservation.Crossref | GoogleScholarGoogle Scholar | 26332195PubMed |
Knell, R. J. (2009). Population density and the evolution of male aggression. Journal of Zoology 278, 83–90.
| Population density and the evolution of male aggression.Crossref | GoogleScholarGoogle Scholar |
Kotiaho, J. S., Alatalo, R. V., Mappes, J., and Parri, S. (1999). Honesty of agonistic signalling and effects of size and motivation asymmetry in contests. Acta Ethologica 2, 13–21.
| Honesty of agonistic signalling and effects of size and motivation asymmetry in contests.Crossref | GoogleScholarGoogle Scholar |
La Nafie, N., Prager, R. H., and Johnston, G. R. (1995). Chemical characterisation of races of the peninsula dragon (Ctenophorus fionni). ACGC Chemical Research Communications 3, 40–44.
Lailvaux, S., and Irschick, D. (2007). The evolution of performance based male fighting ability in Caribbean Anolis lizards. American Naturalist 170, 573–586.
| The evolution of performance based male fighting ability in Caribbean Anolis lizards.Crossref | GoogleScholarGoogle Scholar |
Le Galliard, J., Fitze, P. S., Ferriere, R., and Clobert, J. (2005). Sex ratio bias, male aggression, and population collapse in lizards. Proceedings of the National Academy of Sciences of the United States of America 102, 18231–18236.
| Sex ratio bias, male aggression, and population collapse in lizards.Crossref | GoogleScholarGoogle Scholar | 16322105PubMed |
Leal, M., and Fleishman, L. J. (2004). Differences in visual signal design and detectability between allopatric populations of Anolis lizards. American Naturalist 163, 26–39.
| Differences in visual signal design and detectability between allopatric populations of Anolis lizards.Crossref | GoogleScholarGoogle Scholar |
López, P., Aragón, P., and Martín, J. (2003). Responses of female lizards, Lacerta monticola, to males’ chemical cues reflect their mating preference for older males. Behavioral Ecology and Sociobiology 55, 73–79.
| Responses of female lizards, Lacerta monticola, to males’ chemical cues reflect their mating preference for older males.Crossref | GoogleScholarGoogle Scholar |
López, P., Amo, L., and Martín, J. (2006). Reliable signalling by chemical cues of male traits and health state in male lizards, Lacerta monticola. Journal of Chemical Ecology 32, 473–488.
| Reliable signalling by chemical cues of male traits and health state in male lizards, Lacerta monticola.Crossref | GoogleScholarGoogle Scholar | 16555130PubMed |
Loyn, R. H. (1985). Bird populations in successional forest of mountain ash Eucalyptus regnans in central Victoria. Emu 85, 213–230.
| Bird populations in successional forest of mountain ash Eucalyptus regnans in central Victoria.Crossref | GoogleScholarGoogle Scholar |
Magurran, A. E., and Seghers, B. (1991). Variation in schooling and aggression amongst guppy (Poecilia reticulata) populations in Trinidad. Behaviour 118, 214–234.
| Variation in schooling and aggression amongst guppy (Poecilia reticulata) populations in Trinidad.Crossref | GoogleScholarGoogle Scholar |
Martin, P., and Bateson, P. (2007). ‘Measuring Behaviour: An Introductory Guide.’ (Cambridge University Press: New York.)
Monasterio, C., Salvador, A., and Díaz, J. A. (2010). Altitude and rock cover explain the distribution and abundance of a mediterranean alpine lizard. Journal of Herpetology 44, 158–163.
| Altitude and rock cover explain the distribution and abundance of a mediterranean alpine lizard.Crossref | GoogleScholarGoogle Scholar |
Morton, E. S. (1975). Ecological sources of selection on avian sounds. American Naturalist 109, 17–34.
| Ecological sources of selection on avian sounds.Crossref | GoogleScholarGoogle Scholar |
Nei, M., Maruyama, T., and Chakraborty, R. (1975). The bottleneck effect and genetic variability in populations. Evolution 29, 1–10.
| The bottleneck effect and genetic variability in populations.Crossref | GoogleScholarGoogle Scholar | 28563291PubMed |
Nicholson, K. E., Harmon, L. J., and Losos, J. B. (2007). Evolution of Anolis lizard dewlap diversity (dewlap evolution). PLoS One 2, e274.
| Evolution of Anolis lizard dewlap diversity (dewlap evolution).Crossref | GoogleScholarGoogle Scholar | 17342208PubMed |
Ord, T. J., and Stamps, J. A. (2008). Alert signals enhance animal communication in ‘noisy’ environments. Proceedings of the National Academy of Sciences of the United States of America 105, 18830–18835.
| Alert signals enhance animal communication in ‘noisy’ environments.Crossref | GoogleScholarGoogle Scholar | 19033197PubMed |
Ord, T. J., Blumstein, D. T., and Evans, C. S. (2001). Intrasexual selection predicts the evolution of signal complexity in lizards. Proceedings of the Royal Society of London. Series B, Biological Sciences 268, 737–744.
| Intrasexual selection predicts the evolution of signal complexity in lizards.Crossref | GoogleScholarGoogle Scholar |
Ord, T. J., Peters, R. A., Clucas, B., and Stamps, J. A. (2007). Lizards speed up visual displays in noisy motion habitats. Proceedings of the Royal Society of London. Series B, Biological Sciences 274, 1057–1062.
| Lizards speed up visual displays in noisy motion habitats.Crossref | GoogleScholarGoogle Scholar |
Ord, T. J., Klomp, D. A., Garcia-Porta, J., and Hagman, M. (2015). Repeated evolution of exaggerated dewlaps and other throat morphology in lizards. Journal of Evolutionary Biology 28, 1948–1964.
| Repeated evolution of exaggerated dewlaps and other throat morphology in lizards.Crossref | GoogleScholarGoogle Scholar | 26237179PubMed |
Persson, L. (1985). Asymmetrical competition: are larger animals competitively superior? American Naturalist 126, 261–266.
| Asymmetrical competition: are larger animals competitively superior?Crossref | GoogleScholarGoogle Scholar |
Peters, R. A., Hemmi, J. M., and Zeil, J. (2007). Signaling against the wind: modifying motion-signal structure in response to increased noise. Current Biology 17, 1231–1234.
| Signaling against the wind: modifying motion-signal structure in response to increased noise.Crossref | GoogleScholarGoogle Scholar | 17614279PubMed |
Peters, R. A., Hemmi, J. M., and Zeil, J. (2008). Image motion environments: background noise for movement-based animal signals. Journal of Comparative Physiology. A, Neuroethology, Sensory, Neural, and Behavioral Physiology 194, 441–456.
| Image motion environments: background noise for movement-based animal signals.Crossref | GoogleScholarGoogle Scholar |
Peters, R. A., Ramos, J. A., Hernandez, J., Wu, Y., and Qi, Y. (2016). Social context affects tail displays by Phrynocephalus vlangalii lizards from China. Scientific Reports 6, 31573.
| Social context affects tail displays by Phrynocephalus vlangalii lizards from China.Crossref | GoogleScholarGoogle Scholar | 27526625PubMed |
Pinheiro, J., Bates, D., Debroy, S., and Sarkar, D. (2018). nlme: linear and nonlinear mixed effects models. R package version 3.1-137. Available at https://CRAN.R-project.org/package=nlme
Podos, J. (2001). Correlated evolution of morphology and vocal signal structure in Darwin’s finches. Nature 409, 185–188.
| Correlated evolution of morphology and vocal signal structure in Darwin’s finches.Crossref | GoogleScholarGoogle Scholar | 11196640PubMed |
R Core Team (2018). R: a language and environment for statistical computing. R Foundation for Statistical Computing, Vienna, Austria. Available at https://www.R-project.org/
Ramos, J. A., and Peters, R. A. (2016). Dragon wars: movement‐based signalling by Australian agamid lizards in relation to species ecology. Austral Ecology 41, 302–315.
| Dragon wars: movement‐based signalling by Australian agamid lizards in relation to species ecology.Crossref | GoogleScholarGoogle Scholar |
Ramos, J. A., and Peters, R. A. (2017a). Habitat-dependent variation in motion signal structure between allopatric populations of lizards. Animal Behaviour 126, 69–78.
| Habitat-dependent variation in motion signal structure between allopatric populations of lizards.Crossref | GoogleScholarGoogle Scholar |
Ramos, J. A., and Peters, R. A. (2017b). Motion-based signaling in sympatric species of Australian agamid lizards. Journal of Comparative Physiology. A, Neuroethology, Sensory, Neural, and Behavioral Physiology 203, 661–671.
| Motion-based signaling in sympatric species of Australian agamid lizards.Crossref | GoogleScholarGoogle Scholar | 28573349PubMed |
Robertson, B., Elliott, P., Eason, K., Clout, N., and Gemmell, J. (2006). Sex allocation theory aids species conservation. Biology Letters 2, 229–231.
| Sex allocation theory aids species conservation.Crossref | GoogleScholarGoogle Scholar | 17148369PubMed |
Rose, B. (1982). Food intake and reproduction in Anolis acutus. Copeia 1982, 322–330.
| Food intake and reproduction in Anolis acutus.Crossref | GoogleScholarGoogle Scholar |
Smithson, M. J., and Verkuilen, J. (2006). A better lemon squeezer? Maximum likelihood regression with beta-distributed dependent variables. Psychological Methods 11, 54–71.
| A better lemon squeezer? Maximum likelihood regression with beta-distributed dependent variables.Crossref | GoogleScholarGoogle Scholar |
Stamps, J. A. (1977). The relationship between resource competition, risk, and aggression in a tropical territorial lizard. Ecology 58, 349–358.
| The relationship between resource competition, risk, and aggression in a tropical territorial lizard.Crossref | GoogleScholarGoogle Scholar |
Steinberg, D., Losos, J. B., Schoener, W., Spiller, A., Kolbe, J., and Leal, M. (2014). Predation-associated modulation of movement-based signals by a Bahamian lizard. Proceedings of the National Academy of Sciences of the United States of America 111, 9187–9192.
| Predation-associated modulation of movement-based signals by a Bahamian lizard.Crossref | GoogleScholarGoogle Scholar | 24843163PubMed |
Stock, S., Mrácek, Z., and Webster, J. (2000). Morphological variation between allopatric populations of Steinernema kraussei (Steiner, 1923) (Rhabditida: Steinernematidae). Nematology 2, 143–152.
| Morphological variation between allopatric populations of Steinernema kraussei (Steiner, 1923) (Rhabditida: Steinernematidae).Crossref | GoogleScholarGoogle Scholar |
Stuart‐Fox, D. M., Moussalli, A., Marshall, J. N., and Owens, I. P. (2003). Conspicuous males suffer higher predation risk: visual modelling and experimental evidence from lizards. Animal Behaviour 66, 541–550.
| Conspicuous males suffer higher predation risk: visual modelling and experimental evidence from lizards.Crossref | GoogleScholarGoogle Scholar |
Stuart‐Fox, D. M., Moussalli, A., Johnston, G. R., and Owens, I. P. (2004). Evolution of color variation in dragon lizards: quantitative tests of the role of crypsis and local adaptation. Evolution 58, 1549–1559.
| Evolution of color variation in dragon lizards: quantitative tests of the role of crypsis and local adaptation.Crossref | GoogleScholarGoogle Scholar | 15341157PubMed |
Svanbäck, R., and Bolnick, I. (2007). Intraspecific competition drives increased resource use diversity within a natural population. Proceedings of the Royal Society of London. Series B, Biological Sciences 274, 839–844.
| Intraspecific competition drives increased resource use diversity within a natural population.Crossref | GoogleScholarGoogle Scholar |
Számadó, S., and Hemmi, J. M. (2015). Attention-seeking displays. PLoS One 10, e0135379.
| Attention-seeking displays.Crossref | GoogleScholarGoogle Scholar | 26287489PubMed |
Tinghitella, R. M. (2008). Rapid evolutionary change in a sexual signal: genetic control of the mutation ‘flatwing’ that renders male field crickets (Teleogryllus oceanicus) mute. Heredity 100, 261–267.
| Rapid evolutionary change in a sexual signal: genetic control of the mutation ‘flatwing’ that renders male field crickets (Teleogryllus oceanicus) mute.Crossref | GoogleScholarGoogle Scholar | 18000520PubMed |
Wedekind, C. (2002). Manipulating sex ratios for conservation: short-term risks and long-term benefits. Animal Conservation 5, 13–20.
| Manipulating sex ratios for conservation: short-term risks and long-term benefits.Crossref | GoogleScholarGoogle Scholar |
West-Eberhard, M. J. (1989). Phenotypic plasticity and the origins of diversity. Annual Review of Ecology and Systematics 20, 249–278.
| Phenotypic plasticity and the origins of diversity.Crossref | GoogleScholarGoogle Scholar |
Whitham, T. G. (1986). Costs and benefits of territoriality: behavioral and reproductive release by competing aphids. Ecology 67, 139–147.
| Costs and benefits of territoriality: behavioral and reproductive release by competing aphids.Crossref | GoogleScholarGoogle Scholar |
Wood, W. E., and Yezerinac, S. M. (2006). Song sparrow (Melospiza melodia) song varies with urban noise (Le chant de Melospiza melodia varie avec le bruit urbain). The Auk 123, 650–659.
| Song sparrow (Melospiza melodia) song varies with urban noise (Le chant de Melospiza melodia varie avec le bruit urbain).Crossref | GoogleScholarGoogle Scholar |
Wu, Y., Ramos, J. A., Qiu, X., Peters, R. A., and Qi, Y. (2018). Female–female aggression functions in mate defence in an Asian agamid lizard. Animal Behaviour 135, 215–222.
| Female–female aggression functions in mate defence in an Asian agamid lizard.Crossref | GoogleScholarGoogle Scholar |