Behavioral plasticity in detection height of an invasive, arboreal snake based on size, condition, and prey
Melia G. Nafus

A
B
C
D Present address:
E Present address:
Abstract
Animals may adjust their behavior in predictable ways to balance tradeoffs between resource acquisition and survival or fecundity. Microhabitat selection based on individual traits or environmental conditions is one measure of risk–reward tradeoffs by individuals.
We used data from observational and manipulative studies to investigate whether an arboreal snake (brown treesnake, Boiga irregularis) had context-dependent behavior based on the relationship between estimated prey availability, body condition, size, and detection height (microhabitat use) in two Mariana Islands.
We used observational data collected in four study sites and data from a manipulative study that we collected over a 5-year period. The observational data focused on four sites with different counts of three prey types, including lizards, birds, and small mammals. During the manipulative study we removed snakes, which resulted in increased prey counts over time. Using these two approaches, we tested whether prey counts predicted body condition and then evaluated how prey counts, snake size, and snake condition interactively predicted the detection height of captured individuals.
We found that body condition was greater at sites or in years with greater prey counts across both the observational and manipulative studies. We also found that snakes displayed differential microhabitat use based on both their condition and size. Larger snakes tended to be detected lower than smaller snakes, but only at sites or during years with few bird or small mammal counts. Snakes at sites with greater mammal and bird counts had a positive relationship between size and detection height. Snakes with greater condition scores tended to be detected higher irrespective of size, but this was also dependent on prey counts. At sites with low bird counts, snakes that were in better condition tended to be closer to the ground.
Brown treesnakes modified microhabitat use based on their condition, size, and the number or type of prey available. Our findings were consistent with a hypothesis that they optimized habitat use to secure food resources and maximize survival.
Context-dependent behavioral plasticity may be an important consideration for management of reptiles for population control or growth.
Keywords: behavior, Boiga irregularis, brown treesnake, context dependent, invasive species, microhabitat use, prey availability, prey richness.
Introduction
Behavioral plasticity allows individuals to respond to changing environmental conditions in a context-dependent manner. In practice, organisms optimize behavior based on environmental conditions or their health (Tversky and Simonson 1993). Within the context of animal behavior, such decisions should inform habitat patch choice, and thus, occupancy and dispersal dynamics (Creel and Christianson 2008; Heithaus et al. 2009; Montgomery et al. 2013; Merems et al. 2020). The environment, therefore, is expected to affect both behaviors that determine resource accessibility (food, shelter, or reproduction) and antipredator behaviors (Heithaus et al. 2009; Montgomery et al. 2013). The context dependency of the relationship between foraging choices and mortality risk thus would be dependent on habitat, the type of risk, or trait-mediated interactions (Heithaus et al. 2009; Gordon 2011).
Risk can generally be categorized into factors that influence mortality, which can include exposure, starvation, or predation. When behaving optimally, individuals are expected to evaluate their conditional probability of mortality based on risks (Royama 1981). Individual or intrinsic traits, such as personality and environmental conditions, can affect risk and individual decisions in complex ways. For example, individuals that are at low risk of starvation may also be more risk averse when selecting foraging habitat (Montgomery et al. 2013). Internal state, such as hunger or fat reserves, can thus affect risk tolerance. Factors such as habitat complexity can also affect foraging decisions and flight responses, which influences predator–prey relationships (Hossie and Murray 2011; Klecka and Boukal 2014). For example, antipredator behaviors may be subject to the abundance of predators or how habitat structure influences probability of escape if attacked (Creel and Christianson 2008; Heithaus et al. 2009). Therefore, individual decision making has the potential to be influenced by individual variance (e.g. size, personality or condition), environmental effects (e.g. weather, resource availability, or habitat structure), or their interactive combination (van Oers et al. 2005; Domenici 2010; Citadini and Navas 2013). The choices made by individuals influence community dynamics, spatial use, and organismal response to anthropogenic actions and thus have important implications for management actions.
The context dependency of microhabitat use based on risk has been studied in a variety of terrestrial and aquatic systems (Anholt and Werner 1995; Creel and Christianson 2008; Domenici 2010; Gordon 2011; Montgomery et al. 2013). The decision-making process for the average reptile is, for example, expected to be a complex relationship between the competing biological requirements that include thermal regulation, forage acquisition, and predator avoidance (Downes and Shine 1998; Cooper 2000; Downes 2001; Webb and Whiting 2005). Many reptiles rely on crypsis as one of their primary antipredator strategies, including those that are predators, and must also balance the effect of environmental temperature on their physiology (Webb and Whiting 2005; Citadini and Navas 2013). Cryptic animals can select microhabitats with fewer resources if that improves their ability to avoid detection, which can occur in a size dependent manner (Skelhorn and Ruxton 2013; Taylor and Cox 2019). Therefore, a critical driver of foraging behavior in predatory reptiles that rely on crypsis may be locating prey while remaining hidden.
The brown treesnake (Boiga irregularis) is an arboreal, nocturnal forager that occupies virtually all habitat types in the island of Guam (known as Guåhan in CHamoru), United States of America. Their introduction to Guam and subsequent overexploitation of prey resulted in the decline or extirpation of several lizard species, birds, non-native rodents, and possibly a fruit bat (Savidge 1987; Wiles 1987; Rodda et al. 1992; Fritts and Rodda 1998; Wiles et al. 2003; Wiewel et al. 2009a). Brown treesnakes experience ontogenetic dietary shifts, with juveniles consuming primarily small lizards before adding endothermic prey and larger lizard species to their diet as they increase in body size (Savidge 1988; Lardner et al. 2009). In general, dietary studies indicate brown treesnakes consume nearly all available and size-appropriate species of mammals, birds, and lizards (Savidge 1988). However, the strength of the trophic relationship between brown treesnakes and their prey may be strongly driven by the ontogenetic structure of the population (Nafus and Gray 2024; Nafus et al. 2024a). Although abundant small lizards in Guam may be sufficient to support small- to medium-sized snakes (Savidge 1987), brown treesnakes caught with bird lures or around bird colonies tend to be larger and in better body condition than those caught with mouse lures or in most locations in Guam (Yackel Adams et al. 2019; Klug et al. 2022). Throughout prey-depleted landscapes in Guam, brown treesnakes in poor condition tend to be located on the ground more often (Nafus et al. 2020). Prior work also indicates that brown treesnakes modify their total, as well as three-dimensional, movement based on prey availability, and that individual condition may inform that choice (Nafus et al. 2020; Boback et al. 2022). Because brown treesnakes have specialized scale morphology and an elongated body and prehensile tail that enables the bridging of wide gaps between branches, their morphology is adapted towards arboreality (Jayne and Riley 2007; Jayne and Byrnes 2015). Thus, although prior work has found that brown treesnakes become more terrestrial with increased size in Guam, the relationship may be influenced by individual condition (Rodda and Reed 2007; Nafus et al. 2020). We, therefore, hypothesized that foraging decisions or microhabitat selection by brown treesnakes are affected by the total availability of size-specific prey, the location of prey (ground or arboreal), as well as the condition of the individual. Specifically, an arboreal snake should prefer to remain arboreal across all sizes when sufficient prey are available to sustain individuals but may become more terrestrial when arboreal prey are less available.
Based on the hypothesis that microhabitat use by brown treesnakes is affected by risk tradeoffs driven by prey availability, we sought to evaluate: (1) the relationship between size and arboreality in relation to prey availability; and (2) the relationship between snake condition and prey availability, as well as arboreality. To answer these questions, we used observational and manipulative studies of brown treesnakes in two Mariana Islands occupied by brown treesnakes. The observational study evaluated whether prey (lizards, birds, or small mammals) counted at different sites were predictive of snake body condition and likewise whether size or condition-based relationships with capture height of snakes supported prey-dependent microhabitat use. We re-examined these same questions in a manipulative study at a single site, in which snakes were removed over 5 years and a change in prey availability occurred. We propose that an arboreal snake should prefer to limit terrestrial behavior as a basic antipredator behavior and thus individuals with greater access to prey or in better condition are expected to remain more arboreal, irrespective of size, if snakes engage in optimized microhabitat selection.
Materials and methods
Site
We used three sites located in Guam, as well as Cocos Island (known as Islan Dåno’ in CHamoru; 13.240°N, 144.655°E), Guam, USA, approximately 2.5 km southwest of the coast of Guam as study sites (Fig. 1). In general, the islands have a wet, tropical environment with temperatures ranging from 24 to 32°C and annual rainfall of 2540 mm, with peak rainfall occurring from July to October (Yeo et al. 2023).
Three sites in Guam, USA and one site in Cocos Island, USA, used to measure the relationship among prey availability, snake size, and arboreality of brown treesnakes (Boiga irregularis). (a) North West Field North is a 5-ha research enclosure dominated by tangantangan (Leucaena leucocephala) forest. (b) The Habitat Management Unit is a 55-ha conservation site with a snake barrier surrounding degraded limestone forest dominated by Vitex parviflora. (c) Cocos Island is a 33-ha island off the southern shore of Guam vegetated primarily by strand scrub and forest. (d) Andersen Air Force Base is a periurban military residential compound.
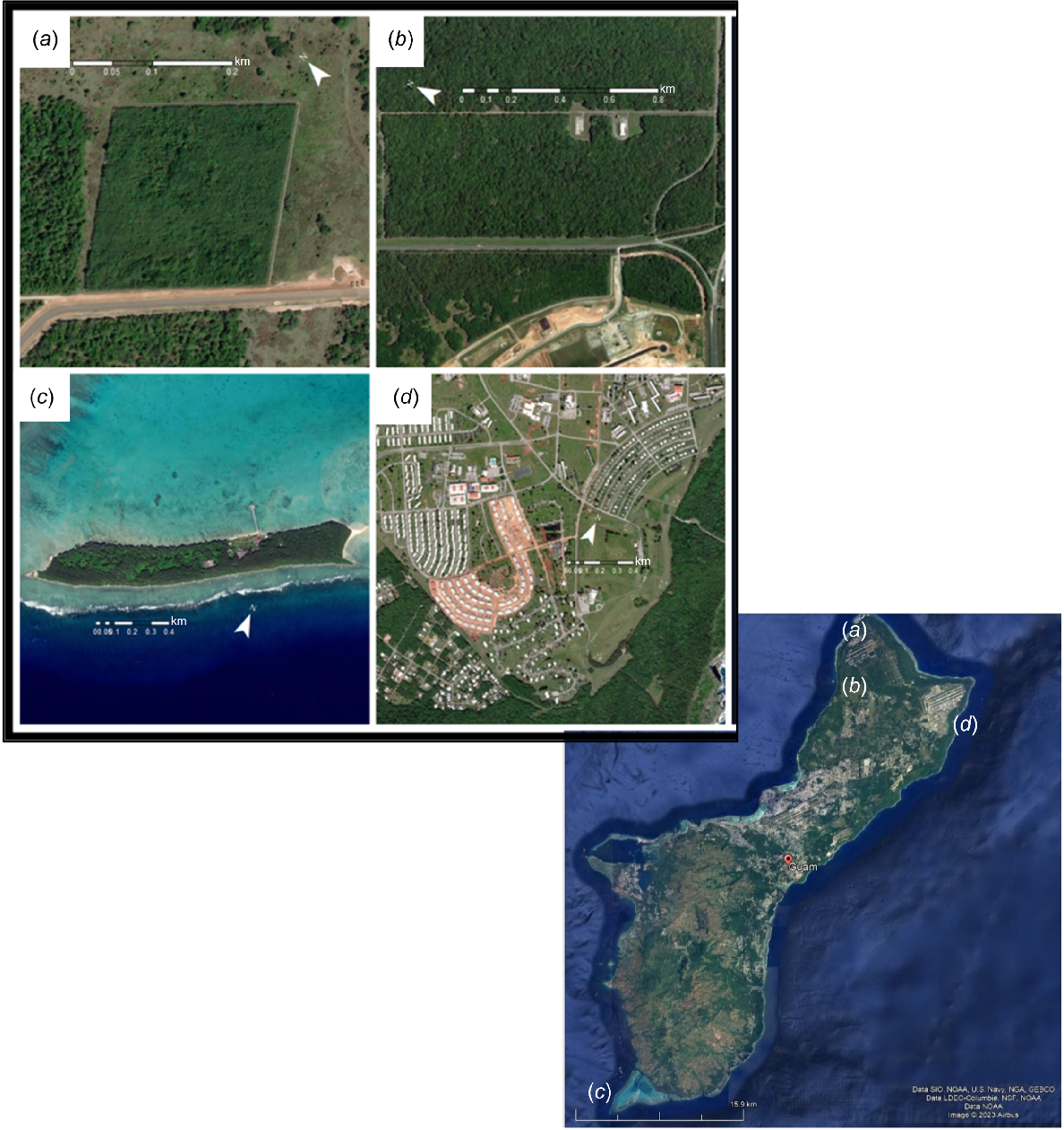
Andersen Air Force Base
Andersen Air Force Base housing (abbreviated as AAFB, Guam, USA; 13.561°N, 144.923°E) is ~105 ha in a periurban, residential neighborhood in northeast Guam (Fig. 1a). AAFB landcover included approximately 300 houses and cleared grass lawns intermixed with mature trees, primarily including Cocos nucifera, Casuarina equisetifolia, Vitex parviflora, Calophyllum inophyllum, and Delonix regia (Goetz and Nafus 2024). The site was not bound by any snake exclusion fencing and was bordered by limestone forest on a karst substrate.
Data collection on AAFB brown treesnakes occurred from December 2019 to August 2022, with 352 individual snakes captured. We visually searched for brown treesnakes, starting approximately 30 min after sundown using a high-lumen headlamp (Lupine Lighting Systems, Wilma R X14). A single observer collected all data on prey and snakes with surveys focused on individual trees. Each tree was allocated a 2-min search time. While surveying a given tree, we also counted each lizard, bird, or small mammal (fanihi or Mariana fruit bat (Pteropus mariannus mariannus), Old World rats (Rattus spp.), house mouse (Mus musculus), or Asian house shrew (Suncus murinus)) observed at the species level when possible. We stopped time when we observed a snake and recorded the approximate height based on visual estimation using 0.1–0.5 m intervals. Precision and accuracy of height estimates decreased as detection height increased. We attempted to capture all snakes by hand. We morphologically processed captured snakes (refer to section below) and then released them at the point of capture.
Cocos Island
Cocos Island (abbreviated as DANO hereafter) is a ~33 ha island (Fig. 1b). In October 2020, a population of brown treesnakes was discovered on the island (Barnhart et al. 2022). Mariana Island species present at this site include ko’ko’ or Guam rail (Gallirallus owstoni), Mariana skinks (Emoia slevini), Micronesian saw-tailed geckos (Perochirus ateles), oceanic geckos (Gehyra oceanica), and såli or Micronesian starling (Aplonis opaca) (Savidge 1987; Rodda et al. 1991; Rodda and Fritts 1992; Wiles et al. 2003). DANO is dominated by coastal strand forest on sand substrate. Limited limestone karst substrate exists in association with the southern shore.
Data collection on brown treesnakes in DANO occurred from October 2020 to September 2021, with 55 total snakes captured during this period. We visually searched for brown treesnakes and prey species along 31 transects that each were ~400 m long, starting approximately 30 min after sundown. We searched in teams of two using a high-lumen headlamp (Lupine Lighting Systems, Wilma R X14), with each observer focused exclusively on either the right or left side of a transect. Each transect survey required approximately 60 min to complete. On average individuals searched approximately three transects per night. Initially, we rotated nightly transect assignments to ensure approximately equal coverage across the island. In July 2021, after determining we were not finding snakes on six of the transects, we adjusted transect assignments to focus more intensively on areas with greater captures.
When a snake was observed, we recorded the approximate height based on visual estimation using 0.1–0.5 m intervals and then attempted to capture the snake. In addition to capturing snakes by hand, we also shot them with 0.39 g plastic pellets fired from electric gun air rifles to prevent escape (Knox et al. 2018). We placed captured snakes in a cloth snake bag and proceeded with surveys. Each snake was morphometrically processed at the laboratory the next day (described below) and then euthanized. During each transect, we also counted each lizard, bird, or small mammal observed to the level of species when identifiable. The full dataset from this effort is available (USGS 2023).
Habitat Management Unit
The Habitat Management Unit (abbreviated as HMU, Guam, USA; 13.596°N, 144.865°E) is a 55 ha snake exclosure built in 2010 for conservation purposes (Fig. 1c). The area is enclosed by a 1.5 m tall fence intended to prevent entry and allow escape of brown treesnakes (Siers et al. 2017). The site is primarily composed of degraded limestone forest on a karst substrate and the dominant tree was Vitex parviflora (Siers et al. 2017).
Data collection on brown treesnakes at the HMU occurred from March to August 2013, with 199 snakes detected. Visual surveys followed similar protocols as described for DANO, with 17 transects of ~470 m in length. We completed each transect survey in approximately 30 min. When a snake was observed, as with other projects, we recorded the height at which a snake was seen (detection height) and attempted hand capture. We also recorded all lizards, birds, or small mammals observed during surveys.
North West Field North
North West Field North (abbreviated as NWFN, Guam, USA; 13.640°N, 144.865°E) is a 5-ha fenced area also located in northern Guam (Fig. 1d), used to study brown treesnakes. The area was enclosed by a two-way 1.5 m tall barrier fence, with a wire mesh overlay, that prevents entry or escape of snakes (Rodda et al. 2007). The dominant vegetation is tangantangan (Leucaena leucocephala) forest intermixed with degraded limestone forest on a karst substrate (Nafus et al. 2018a). The forest was continuously maintained with 29 transects that are 220 m long and separated by ~8 m.
This site has been the focus of long-term research on brown treesnakes; thus, data collection occurred on and off from 2006 to 2023. The full process of visual surveys and field efforts at this site for these data are well described in prior publications (Rodda et al. 2007; Nafus et al. 2022, 2024a). Briefly, from October 2006 to December 2007 and from October 2016 to March 2017, we censused the population by conducting surveys one to four nights per week. Pacing for 2006–2007 surveys was 30 min per 220 m transect. In 2016, we increased observer pace to complete each 220 m transect in 10 min. Visual survey and snake capture protocols followed those already described for DANO and HMU.
On 31 March 2017, we began an intensive snake removal effort, which is described in previously published work (Nafus et al. 2022, 2023, 2024a). Briefly, we removed snakes using toxic mouse carrion from 31 March 2017 to 20 September 2021. Between 14 September 2020 and 25 October 2021, we added chick and rat carrion as baits. Between 1 March 2021 and 23 December 2021, we started euthanizing snakes captured in live traps that had mouse or bird lures. From 24 October 2016 to 31 March 2023, we also euthanized any snakes captured by hand. Throughout this period, the snake population was in continuous decline other than a brief birth pulse (Nafus et al. 2024a). The full data from 2016 to 2023 with detailed descriptions of snake removal methods are available (Nafus et al. 2023).
Snake morphological data
Following capture, we weighed snakes using a Pesola® spring scale and measured snout-vent length (SVL) by stretching the snake along a cloth measuring tape. We calculated the condition of each snake as a ratio calculated by dividing the log mass of the individual by the expected mass. The expected mass was the averages of the study population for each site. We uniquely marked snakes captured at NWFN, HMU, and AAFB by implanting a passive integrated transponder tag (PIT tag; Biomark Inc, Boise, Idaho) and clipped four of the ventral scales in a unique pattern. Unless otherwise described in a preceding section, we immediately released snakes following capture. Because DANO and in some NWFN years had only one capture point for individuals, we used only data from an individual’s first capture within a site or year. All snake handling was conducted in accordance with Animal Care and Use Committee protocols approved through U.S. Geological Survey Fort Collins Science Center (FORT IACUC 2017-04, FORT IACUC 2018-06 and -07, FORT IACUC 2021-02).
Statistical methods
We created two datasets for analyses according to whether the study design was observational (AAFB, DANO, HMU, and NWFN: 2006–2007) or we were manipulating the snake population by removal (NWFN: >2016). For the dataset in which we removed snakes, we broke the data into four discrete intervals: October 2016–March 2017 (pre-treatment or 2016), September 2019–March 2020 (year 2 of treatment or 2019), September 2020–May 2021 (year 4 of treatment or 2021), and September 2022–March 2023 (year 5 of treatment or 2022). For each dataset, we calculated cumulative prey counts per cumulative hour searched (prey count rates) for lizards, small mammals, or birds as an index of prey availability. We then assigned prey counts as a relative, but continuous value for each snake based on the snake’s SVL and the site or year of capture. We distinguished prey by size class based on prior work suggesting size-specific predator–prey dynamics or trophic effects (Nafus and Gray 2024). For snakes <850 mm SVL, we assigned a prey count value using only counts of lizards during the sampled year or site (Lardner et al. 2009; Nafus and Gray 2024). For snakes 850–1199 mm SVL, we assigned the prey count value per snake as the sum of all lizards, birds, and small mammals counted at that site or during the specified year (Savidge 1988). For snakes >1199 mm SVL, we used counts of birds and small mammals and excluded lizards (Savidge 1988). For descriptive purposes, we report the proportional size structure of snake SVL by site or year and mean body conditions based on the identified size categories (<850, 850–1199, ≥1200 mm).
To evaluate whether there were site (observational study) or annual (manipulative study) effects on snake condition, we used two linear regressions with SVL and either site or treatment year as fixed effects, with an interactive term between them. We also included prey counts as an additive, continuous fixed effect to evaluate whether prey counts predicted snake condition. We square root transformed condition to normalize the distribution and evaluated model fit by comparing the predicted and observed residuals. We used the emmeans package in R to conduct pairwise comparisons by site or treatment year on condition, after accounting for site- or year-specific differences in SVL, using a Tukey Test (Lenth 2024).
To evaluate whether SVL or condition predicted detection height for snakes, we completed two generalized linear mixed effect regressions. We square root transformed detection height to normalize the distribution. We included centered and scaled data for SVL and condition as fixed effects, with an interactive term between them. We also included size-specific prey counts as an additive, continuous fixed effect. We treated site (AAFB, DANO, HMU, NWFN) or treatment year (NWFN: 2016, 2019, 2021, 2022) as random effects in the observational and manipulative models, respectively. We tested for multicollinearity between SVL and condition using variance inflation factors (VIF) and found their VIF < 5 (Akinwande et al. 2015). We verified that the detection height data were normally distributed and not over- or under-dispersed by comparing residual deviances between the observed and predicted values, as well as testing for outliers. For all statistics we used R v. 4.3.3 (R Core Team 2020, Vienna, Austria) and accepted significance when P ≤ 0.05. The dataset used in these analyses is available for download (Nafus et al. 2024b).
Results
Prey availability
Prey count rates were variable by site (Fig. 2a). At AAFB, we documented eight endothermic species including three native birds (white tern or chunge’ (Gygis alba), kakkak yellow bittern or kakkak (Ixobrychus sinensis), and A. opaca), two non-native small mammals (S. murinus and Rattus spp.), and four lizard species. Across all four sites, AAFB had the second greatest number of birds observed, and we counted more birds than lizards or small mammals (Fig. 2a). At DANO, we documented 11 endothermic species including one native small mammal (P. mariannus mariannus) and seven native bird species (black noddy (Anous minutus), brown noddy (A. stolidus), G. alba, I. sinensis, G. owstoni, Pacific reef heron (Egretta sacra), and A. opaca), as well as 12 lizard species. Of our sites, DANO was the most species rich and had the greatest numbers of birds and lizards counted per hour surveyed (Fig. 2a). At the HMU, we documented five endothermic species including two non-native birds (Philippine collared dove (Streptopelia bitorquata) and red junglefowl (Gallus gallus)) and three non-native small mammals (S. murinus, M. musculus, and Rattus spp.), as well as seven lizard species. Prey encounter rates were relatively low compared to other sites, although we counted 60 times more birds at the HMU (0.25/h) than at NWFN (0.004/h) during 2006–2007. At NWFN (2006–2007), we documented two endothermic species including one native bird (I. sinensis) and one non-native small mammal (Rattus spp.), as well as four lizard species. NWFN had the lowest counts of endotherms across all four sites, with total counts for birds and small mammals of 0.01/h.
Prey count rates by site (a) or year within site (b). During nocturnal visual surveys we counted the number of lizards, birds, or small mammals seen per hour of surveys at four study sites: Andersen Air Force Base (AAFB), Cocos Island (DANO), Habitat Management Unit (HMU), and North West Field North (NWFN) in Guam as part of an observational study on how prey availability may affect snake condition and behavior (a). We also conducted the same work within NWFN across sequential years starting in 2016 when no snake removal occurred and then again in 2019, 2021, and 2022 when snake removal resulted in a continuous decline of the snake population over time (b).
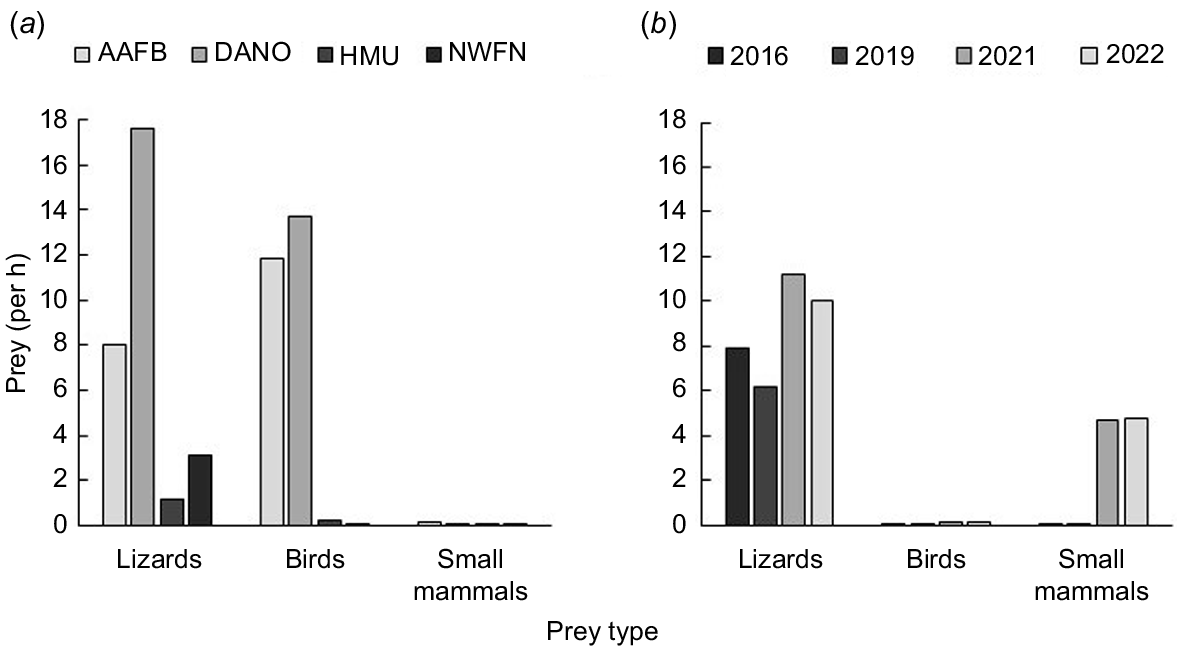
Prey count rates also varied across treatment years in NWFN (Fig. 2b), with hourly counts tending to increase as snake removal continued. Lizard counts were relatively stable in 2016 and 2019 and nearly doubled in 2021 and 2022 (Fig. 2b). Birds were virtually undetectable in 2016 (0.006/h) and 2019 (0.004/h) and increased by 32 times from 2019 to 2021 (0.11/h) and another 1.3 times from 2021 to 2022 (0.16/h). Small mammals had similar trends, and increased from 0.03/h to 0.06/h from 2016 to 2019 and then to 4.71/h and 4.73/h in 2021 and 2022, respectively. Overall, endothermic prey was counted at a rate that was 144 times greater in 2022 as compared to 2016.
Size and condition of the sampled snake populations
Snake size (Fig. 3a) and condition (Fig. 3b) varied by site. At AAFB, captured snakes were distributed relatively evenly across the three size categories (Fig. 3a), with an average SVL of 930 ± 228 mm SVL (±1 s.d.) for captured snakes. The majority of snakes captured at DANO were ≥1200 mm SVL (Fig. 3a), with the average SVL being 1108 ± 314 mm. Snakes captured at the HMU were smaller on average, with few captures of individuals in the ≥1200 mm SVL size category (Fig. 3a) and an average SVL of 849 ± 209 mm. NWFN snakes captured in 2006–2007 were on average the smallest captured, at 569 ± 171 mm SVL, with most individuals <850 mm and no individuals ≥1200 mm (Fig. 3a). Snake condition had a positive relationship with size (β = 0.00039, 95% confidence interval [CI] = 0.00037–0.00040, t = 61.5, P < 0.001) and prey counts (β = 0.002, 95% CI = 0.002–0.0003, t = 10.8, P < 0.001). After controlling for site-specific size differences, condition also varied by site. AAFB snakes were in significantly better condition than HMU (β = 0.00005, 95% CI = 0.00003–0.00007, t = 4.6, P < 0.001) or NWFN (β = 0.00009, 95% CI = 0.00006–0.00012, t = 6.2, P < 0.001) snakes and had similar conditions to DANO (β = −0.00003, 95% CI = 0.00001–0.00006, t = −2.4, P = 0.08) snakes. DANO snakes were also in significantly better condition than those captured in the HMU (β = 0.00008, 95% CI = 0.00005–0.00010, t = 5.7, P < 0.001) or NWFN (β = 0.00012, 95% CI = 0.00010–0.00016, t = 7.1, P < 0.001). HMU snakes were in better condition than NWFN snakes (β = 0.00004, 95% CI = 0.00001–0.00008, t = 2.8, P = 0.02). Trends in condition by site appeared variable by size category, although DANO snakes were in the best condition across all size categories (Fig. 3b).
Size (a) and body condition (b) trends of brown treesnakes across four sites with variable prey counts. The proportion of the total captured number of brown treesnakes (Boiga irregularis) in four sites (Andersen Air Force Base (AAFB), Cocos Island (DANO), Habitat Management Unit (HMU), and North West Field North (NWFN)) in each of three snout-vent length (SVL) size categories related to dietary preference towards lizards (<850 mm), transitioning between lizards and endotherms (850–1199 mm), and preferring endotherms (≥1200 mm). Average body condition reflected site differences that were additive to size dependent relationships with snakes in sites that had greater prey counts (AAFB, DANO) tending to be in better condition than snakes with lower prey counts (HMU, NWFN). Error bars represent +1 standard deviation.
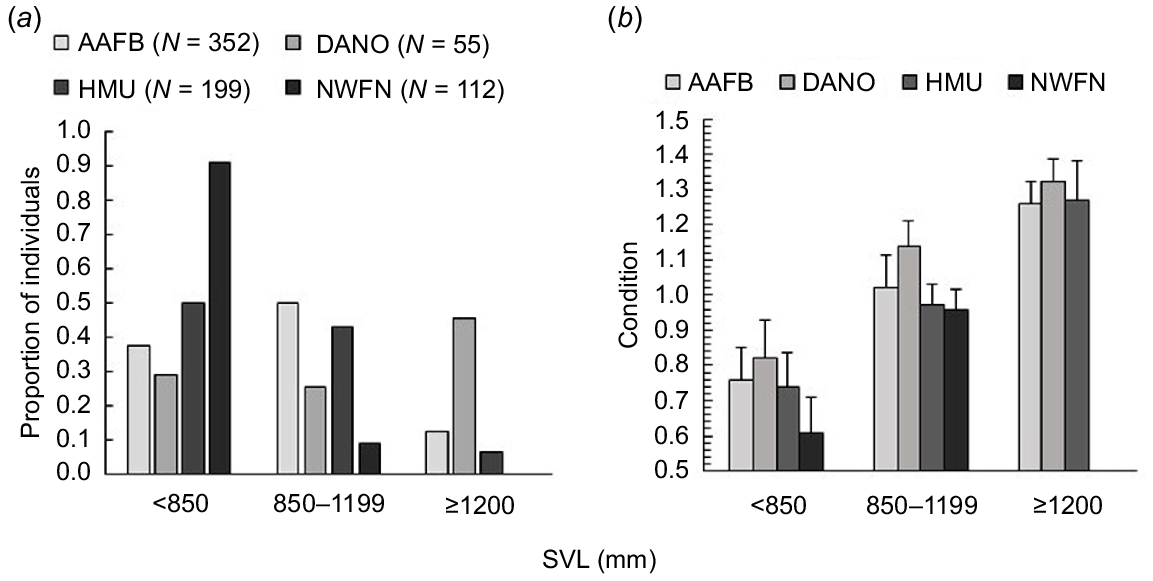
Snake size (Fig. 4a) and condition (Fig. 4b) within NWFN varied by year. Prior to any snake removal, in 2016, the mean size of snakes captured was 1019 ± 68 mm SVL, with no individuals <850 mm SVL (Fig. 4a). In 2019, after 2 years of removal via toxic mouse baits, the mean size of snakes captured decreased to 901 ± 177 mm SVL, and individuals were more evenly spread between the two smaller size categories (Fig. 4a). After 4 years of removal (2021), the mean size of snakes captured had decreased to 729 ± 217 mm SVL. This mean decrease in the size of captured snakes remained consistent in the fifth year of removals (2022), with a mean SVL of 741 ± 206 mm (Fig. 4a). Snake condition continued to have a positive relationship with size (β = 0.00035, 95% CI = 0.00028–0.00042, t = 10.4, P < 0.001) and prey counts (β = 0.003, 95% CI = 0.001–0.005, t = 3.3, P = 0.001). After controlling for size differences across the years, condition also varied by year. Snakes captured in 2016 were in worse condition than those captured in 2021 (β = −0.00014, 95% CI = −0.00019 to −0.00004, t = −2.9, P = 0.02) and 2022 (β = −0.00013, 95% CI = −0.00021 to −0.00005, t = −3.2, P = 0.008), but equivalent condition to snakes captured in 2019 (β = −0.00008, 95% CI = −0.00014 to 0.00000, t = −2.1, P = 0.14). Snakes captured in 2019 were not, however, significantly different in their condition from snakes captured in 2021 (β = −0.00038, 95% CI = −0.00008 to −0.00000, t = −1.7, P = 0.34) and 2022 (β = −0.00005, 95% CI = −0.00009 to 0.00000, t = −2.2, P = 0.12), nor were snakes captured in 2021 in different condition from those captured in 2022 (β = −0.00001, 95% CI = −0.00006 to 0.00004, t = −0.4, P = 0.97). Overall, differences in condition across years were most strongly driven by snakes that were ≥1200 mm SVL (Fig. 4b).
Size (a) and body condition (b) trends of brown treesnakes in a single site as prey varied across time following snake removal. The proportion of the total captured number of brown treesnakes (Boiga irregularis) in North West Field North over 4 years (2016, 2019, 2021, and 2022) divided into three snout-vent length (SVL) size categories related to dietary preference towards lizards (<850 mm), transitioning between lizards and endotherms (850–1199 mm), and preferring endotherms (≥1200 mm). Average body condition reflected site differences that were additive to size dependent relationships with snakes in years with greater prey counts (2021, 2022) tending to be in better condition than snakes captured in the year with very low prey counts, before snake removal began (2016). Error bars represent +1 standard deviation.
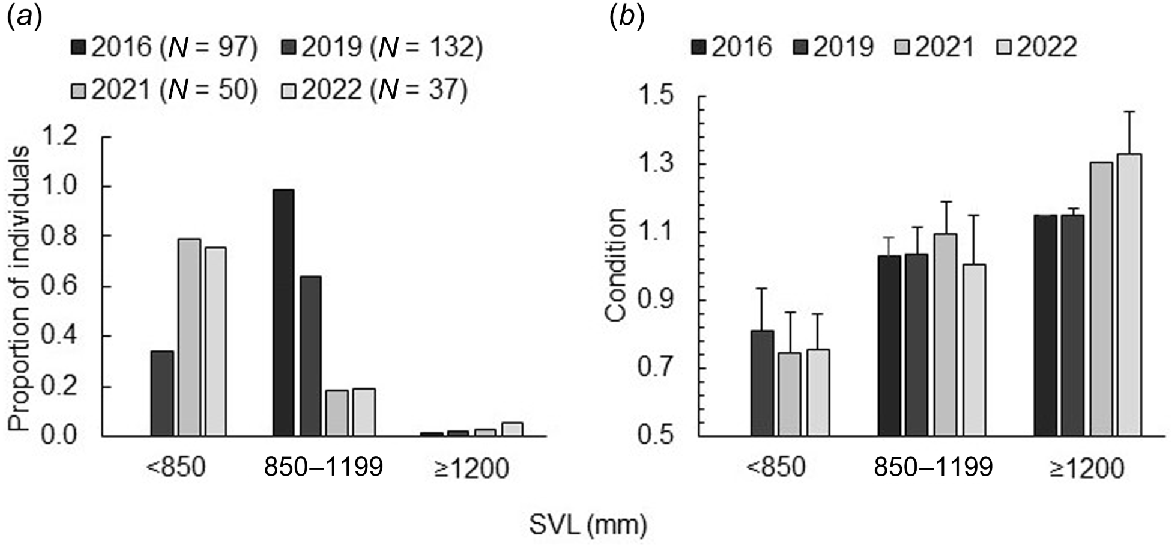
Size, condition, and prey relationships with detection height
The average estimated detection height of captured snakes varied across all four of the study sites, such that snakes were captured at the greatest average height in DANO, followed by AAFB, then HMU and last NWFN (Fig. 5a). When averaged across all four study sites, detection height had an inverse relationship with size (Table 1). However, detection height relationships with size were site-specific. In sites with greater relative bird counts (DANO, AAFB), larger snakes were more likely to be found higher in the tree, whereas in low bird count sites (HMU, NWFN) larger snakes were more likely to be found closer to the ground (Fig. 5b). In contrast to SVL, detection height increased with condition (Table 1). This effect was also site-specific: at sites with high bird counts (AAFB, DANO), snakes in good condition tended to be higher in trees; whereas, at sites with low bird counts (HMU, NWFN) snakes in good condition tended to be found closer to the ground (Fig. 5c). Condition had a positive interactive effect with SVL on detection height (Table 1). Prey counts were positively correlated with snake heights at capture (Table 1).
Detection height (m) of arboreal brown treesnakes captured in four sites (Andersen Air Force Base (AAFB), Cocos Island (DANO), Habitat Management Unit (HMU), and North West Field North (NWFN)) with different prey counts. Mean estimated height (±1 s.e.) of detection was greatest at the site with the most prey counts (DANO) and least at the site with the lowest prey counts (NWFN) (a). Linear regression of detection height based on size (snout-vent length (SVL, mm)) indicated snakes in sites with high prey counts had no or a positive size relationship with detection height and a negative one in prey-depleted sites (b). Body condition had an overall positive relationship with detection height but was negative for sites with low and dominantly rodent endothermic prey counts (HMU, NWFN) (c).
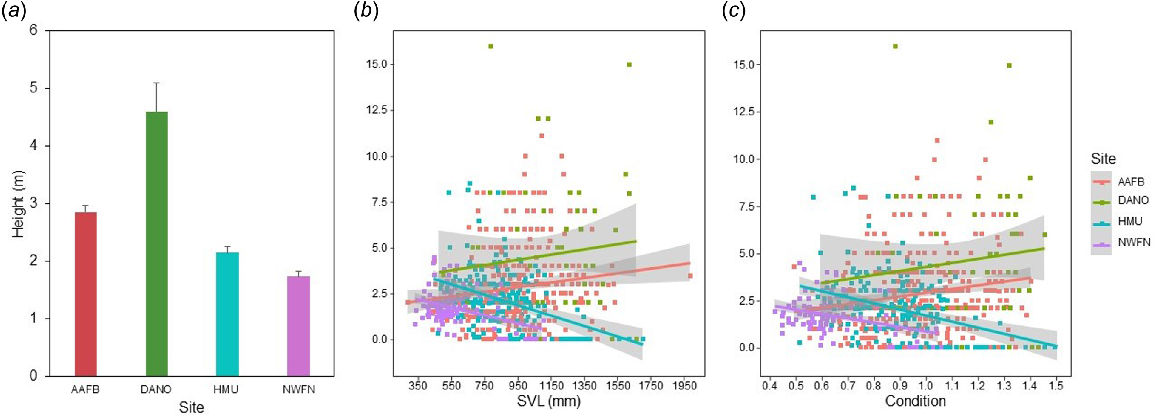
β | 95% CI | t | P | |||
---|---|---|---|---|---|---|
Lower | Upper | |||||
β0 | 1.12 | 0.91 | 1.34 | 10.2 | ||
SVL | −0.43 | −0.68 | −0.18 | −3.3 | <0.001 | |
Condition | 0.30 | 0.05 | 0.56 | 2.3 | 0.02 | |
SVL × Condition | 0.05 | 0.01 | 0.10 | 2.2 | 0.03 | |
Prey | 0.03 | 0.02 | 0.04 | 5.4 | <0.001 |
Each of the four sites varied in prey availability and site-specific effects were controlled as a random effect in a mixed effect linear model. All predictors were scaled and centered so that coefficients can be compared and interpreted as relative effect sizes.
Detection height of captured snakes was less variable within NWFN across years as compared to cross-site contrasts, although there was a slight tendency for increased capture heights in 2021 and 2022 as compared to prior years (Fig. 6a). Across all 4 years, size had an inverse relationship with detection height (Table 2). Within the years with greater prey counts (2021, 2022), larger snakes were more likely to be found higher in the tree as compared to when prey counts were lower (2016 and 2019) (Fig. 6b). Detection height increased with condition, such that snakes in better condition were captured higher in the tree (Table 2). The effect on condition on detection height had slight annual variance but was generally positive (Fig. 6c). There was no interactive effect between SVL and condition (Table 2). Changes in prey counts across treatment years did not correlate with an increase in capture height at NWFN (Table 2).
Detection height (m) of arboreal brown treesnakes captured in 4 years at North West Field North (NWFN). Snake removal from 2016 to 2023 resulted in increasing prey counts across time. Mean estimated height (±1 s.e.) of detection did not change across time (a). Linear regression of detection height based on size (snout-vent length [SVL, mm]) indicated snakes in years with high prey counts had no or a positive size relationship with detection height and a negative one in prey-depleted years (b). Body condition had an overall positive relationship with detection height but was neutral in 2019 (c).
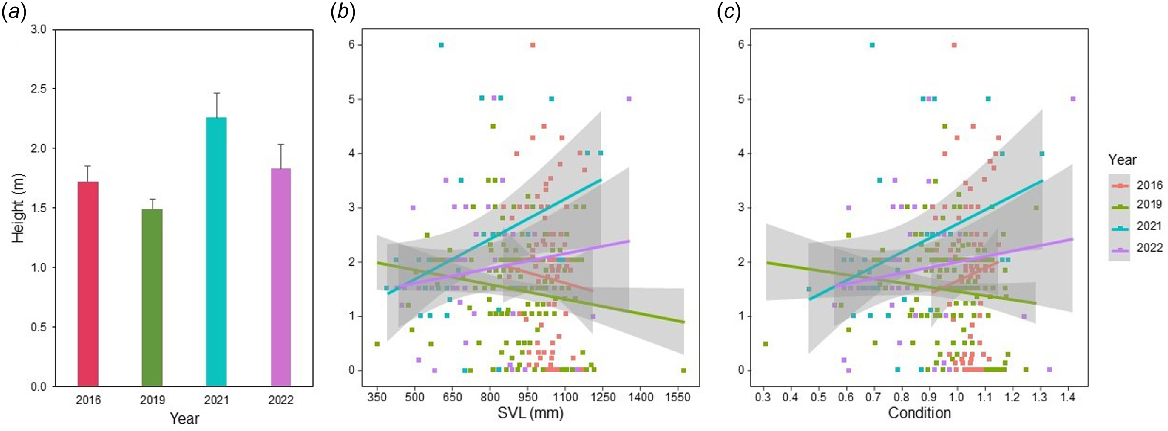
β | 95% CI | t | P | |||
---|---|---|---|---|---|---|
Lower | Upper | |||||
β0 | 1.07 | 0.79 | 1.35 | 7.6 | ||
SVL | −0.42 | −0.66 | −0.17 | −3.3 | 0.001 | |
Condition | 0.36 | 0.11 | 0.60 | 2.8 | 0.004 | |
SVL × Condition | −0.01 | −0.07 | 0.05 | −0.6 | 0.55 | |
Prey | 0.01 | −0.02 | 0.05 | 0.9 | 0.41 |
The site experienced prey count changes in response to a snake removal that began after the first year. We controlled for annual differences as a random effect in a mixed effect linear model and scaled and centered predictors so that coefficients can be compared and interpreted as relative effect sizes.
Discussion
We found evidence to support that rates of prey counts by human observers were a positive predictor of snake body condition scores. We also found that size was inversely related to estimated height at detection in Guam, but the effect was site-specific when prey counts were considered. Across sites, snakes in locations with greater prey counts were found higher in trees, but this was not true for inter-annual comparisons of snakes within a single site. Thus, sites with greater prey counts may have had taller trees that led to the overall increase in detection height by snakes, or the increase in detection height may have required a much greater abundance of birds than we observed in NWFN. In general, however, snake size at sites or during times with greater prey counts had a positive relationship with detection height instead of the negative relationship more broadly measured within Guam. Body condition, in contrast, was generally positively correlated with detection height. This was less true, however, at forested sites in which rodents were the primary endothermic prey item counted and birds were rarely recorded. In these locations, the top condition snakes tended to be located closer to the ground. Thus, prey availability and type as a function of snake size and body condition appear to affect height at which brown treesnakes are detected.
We used hourly prey counts as a proxy for prey availability, which relies on the same conceptual principles of capture per unit effort (CPUE). In general, CPUE is often a problematic index of density. There is a known poor relationship between abundance and CPUE for lizards in the Mariana Islands (Rodda et al. 2015). Counts may be a function of detectability, activity, or abundance and do not control for resampling of individuals. We found, however, that prey counts, when classified based on predicted ontogenetic relationships between snakes and prey, were positively correlated with snake condition based on both observational and manipulative datasets. Moreover, additional studies in brown treesnakes have supported measurable responses of prey counts following the extensive removal of snakes (Campbell et al. 2012; Nafus et al. 2024a). The measurable and strong relationship between prey counts and snake condition thus supports that prey counts are at least a potentially reliable measure of food availability for brown treesnakes, even if they are not a reliable index of total population sizes of prey species.
The site-specific relationships between snake size and detection height may, in part, be explained by habitat use by prey. For instance, the sites with abundant endothermic prey also had more avian than small mammal prey. Small mammals that commonly occur in Guam forests include Old World rats, such as the black rat (Rattus rattus diardii), as well as R. exulans and R. norvegicus, Asian house shrews, and mice (Wiewel et al. 2009b). Shrews are primarily terrestrial, while R. r. diardii is semiarboreal with the authors primarily sighting rats in the trees. Few native ground-dwelling birds are in the Mariana Islands and none currently occur on Guam (Savidge 1987), although three ground-dwelling non-native birds (blue-breasted quail (Coturnix chinensis), black francolin (Francolinus francolinus), and red junglefowl) occur in various locations in Guam and Guam rails occur in Cocos Island. Sites such as DANO and AAFB likely, therefore, had more arboreal than terrestrial endothermic prey, which may partly explain why large snakes were more arboreal in those sites even at larger sizes. The venom specificity of brown treesnakes and experimental trials on brown treesnake behavior indicate both an evolutionary history with avian prey and a potential behavioral preference for them as prey over mammals (Mackessy et al. 2006; Nafus et al. 2021). Therefore, in habitats in which birds are present and available as a prey source for larger individuals, brown treesnakes may preferentially forage in areas in which they are more likely to encounter birds (Klug et al. 2021, 2022), which may cause increased arboreality.
The relationship between detection height and size was, however, also observed in the manipulative experiment, and the primary change in prey counts during this study was a large increase in rat counts. Given the relationships between prey counts and condition, it may be reasonable to assume that individuals in better condition were feeding more frequently. For reptiles, basking or other forms of thermoregulation are likely important for digestion of meals (Hammond et al. 1988; Blouin-Demers and Weatherhead 2001). In dense forests, arboreal snakes may therefore prefer higher canopy refugia following consumption of meals to aid in digestion (Siers et al. 2018). In this case, brown treesnakes in higher prey habitats may have been located higher in the tree as a byproduct of spending a greater proportion of their time digesting recent meals.
A context-dependent relationship between size or condition and arboreality could also be explained by plasticity in foraging technique by snakes based on the type of prey available. Snakes forage using sit-and-wait, in which individuals ambush mobile prey from stationary positions, and active, in which individuals seek prey by moving across the landscape, tactics. In general, the two foraging modes balance energy expenditure: sit-and-wait has low energy expense and low energy intake, whereas active foraging has high energy expense and greater energy consumption (Secor and Nagy 1994). Brown treesnakes have been observed switching between foraging mode, even within the same night (Rodda 1992). Foraging mode may also vary ontogenetically (Secor and Nagy 1994), while overall activity can be influenced by food availability (Glaudas and Alexander 2017; Siers et al. 2018). Within individuals, prey type can also influence hunting strategy. For example, in venomous species large prey may be struck and released, presumably to avoid injury, whereas small prey struck and held (Glaudas et al. 2017). Thus, within individuals and across species, snakes can adopt differential foraging modes based on prey type (Rodda 1992; Perry and Pianka 1997). Because most birds in Guam are stationary at night, a diet heavily reliant on birds would require active foraging. Rats, shrews, and geckos, in contrast, are active at night and could reasonably be ambushed by a snake engaged in a sit-and-wait tactic. Foraging mode or behavior determined by the type of prey being hunted could, therefore, also influence microhabitat selection.
Context-dependent changes in arboreality may also be caused by differences in movement frequency. Overall movement seemingly declines with greater prey counts, although dispersal-type movement behaviors may have been encouraged when the original invasion was spreading across Guam to allow the exploitation of prey-rich areas not yet colonized by snakes (Rodda et al. 2008; Boback et al. 2022). Even snakes engaged in sit-and-wait foraging can make large movements when selecting new ambush locations (Glaudas and Alexander 2017). Imperfect connectivity between trees may predict that individuals traveling greater distances between foraging sites spend more time on the ground. However, such a hypothesis would anticipate that urban snakes would use the ground more frequently than forest snakes, which was not supported by our data. We found periurban snakes were more arboreal at larger sizes compared to Guam forest snakes.
Microhabitat selection by snakes in Guam may also be influenced by their evolutionary history with predators as well. Although predators of brown treesnakes in their native range are poorly documented, the evolution of specific morphological attributes indicate brown treesnakes may be better equipped to escape enemies in trees. For example, brown treesnakes have specialized scale morphology that increases climbing speed and an elongate body and prehensile tail enabling the bridging of wide gaps between branches (Jayne and Riley 2007; Jayne and Byrnes 2015). Moreover, locomotor choices and style are influenced by musculature and preference for moving upward relative to thicker-bodied snakes, supporting high adaptations for arboreality (Hoefer and Jayne 2013). The interaction between prey escape tactics, which can be species specific, and landscape features may be one factor that is underappreciated in the understanding of the context dependency of risk in individual decision making (Heithaus et al. 2009). While there are few predators for brown treesnakes in Guam, there are documented instances in which they have been consumed by domestic cats (Felis catus), as well as Mariana monitor or hilitai, (Varanus tsukamotoi) (Nafus et al. 2020). Both predators are semiarboreal but probably pose the greatest risk to snakes on the ground. Brown treesnakes in the canopy may be harder to detect, as their bodies resemble the branches of trees and related arboreal structures such as vines. Moreover, brown treesnake arboreality may reflect an evolutionary response to predators present in their native range and have little to do with predators in Guam.
Independently of the mechanism, however, the data strongly support that sampled snake populations with greater access to prey were healthier and generally more arboreal. Although our data supported prior findings that juvenile brown treesnakes are more arboreal than adults in Guam (Rodda and Reed 2007), there was also evidence for a counter relationship between arboreality and size in more prey-rich sites. The two sites with the greatest prevalence of prey overall demonstrated an opposing trend in which arboreality increased with size. Given that we would expect distance to affect size dependent detection rates, the data generally indicate that brown treesnakes of all sizes may prefer to be arboreal when environmental conditions support such habitat selection. However, although behavioral plasticity may inform microhabitat selection strategies, some studies indicate that microhabitat choice in arboreal snakes is driven more by extrinsic than intrinsic traits (Thaler et al. 2022). Our results support that microhabitat selection in reptiles is complex and important to consider when assessing predator-prey interactions that can scale up to landscape distributions.
Implications for management
Prior work examining the relationship between trap captures and trap height indicated that smaller snakes were captured more often in traps placed farther from the ground (Nafus et al. 2018b). This may be because larger brown treesnakes are more terrestrial in a prey-limited environment; arguably prey-limited conditions characterize most historical studies of brown treesnakes in Guam. Thus, as prey richness or avian availability increases, low placement of traps may become less effective and lure types may also disproportionally influence captures (Nafus et al. 2021, 2024a). The three-dimensional placement of detection or removal tools can influence population response (Nafus et al. 2022). As a consequence, particularly when detection tools are dependent on foraging behavior, the factors influencing foraging behavior have management implications. These data indicate that placing traps or baits closer to the ground when targeting arboreal snakes may increase accessibility in prey-depleted landscapes. In contrast, elevating them towards heights over 2.5 m may improve contact rates in more prey-rich locations.
Declaration of funding
This research was supported by Office of Insular Affairs, U.S. Department of Navy (IAA #N61128-20140529-0001), including Joint Region Marianas and Marine Corps Forces, Pacific, and U.S. Geological Survey Ecosystems Mission Area. No funders were involved as authors in the preparation of this manuscript.
Acknowledgements
We would like to thank P. Barnhart, A. Collins, C. Hopkins, L. Huse, M. Viernes, and many others for their assistance in coordinating various projects. We acknowledge the contributions of B. Lardner in generating a subset of the data used. We thank M. Hall and H. McCoy for administration of funding. We thank N. Claunch for providing comments that improved an earlier version of this manuscript. We thank the multitude of biologists that contributed to the collection of these data and members of the Research Corporation of the University of Guam, including C. Moore-Linn, for their assistance with field efforts. Any use of trade, firm, or product names is for descriptive purposes only and does not imply endorsement by the U.S. Government.
References
Akinwande MO, Dikko HG, Samson A (2015) Variance inflation factor: as a condition for the inclusion of suppressor variable(s) in regression analysis. Open Journal of Statistics 5, 754-767.
| Crossref | Google Scholar |
Anholt BR, Werner EE (1995) Interaction between food availability and predation mortality mediated by adaptive behavior. Ecology 76, 2230-2234.
| Crossref | Google Scholar |
Barnhart PD, Quiogue ZC, Frasch ER, Vice DL, Hopkins CB, Yackel Adams AA, Reed RN, Nafus MG (2022) Boiga irregularis (Brown Treesnake). Herpetological Review 53, 444-445.
| Google Scholar |
Blouin-Demers G, Weatherhead PJ (2001) An experimental test of the link between foraging, habitat selection and thermoregulation in black rat snakes Elaphe obsoleta obsoleta. Journal of Animal Ecology 70, 1006-1013.
| Crossref | Google Scholar |
Boback SM, Nafus MG, Yackel Adams AA, Reed RN (2022) Invasive brown treesnakes (Boiga irregularis) move short distances and have small activity areas in a high prey environment. Scientific Reports 12, 12705.
| Crossref | Google Scholar |
Campbell EW, III, Adams AAY, Converse SJ, Fritts TH, Rodda GH (2012) Do predators control prey species abundance? An experimental test with brown treesnakes on Guam. Ecology 93, 1194-1203.
| Crossref | Google Scholar |
Citadini JM, Navas CA (2013) Inter-individual variation and temperature-dependent antipredator behavior in the snake Tomodon dorsatus (Dipsadidae). Behavioural Processes 97, 11-17.
| Crossref | Google Scholar |
Cooper W (2000) Tradeoffs between predation risk and feeding in a lizard, the broad-headed skink (Eumeces laticeps). Behaviour 137, 1175-1189.
| Crossref | Google Scholar |
Creel S, Christianson D (2008) Relationships between direct predation and risk effects. Trends in Ecology & Evolution 23, 194-201.
| Crossref | Google Scholar | PubMed |
Domenici P (2010) Context-dependent variability in the components of fish escape response: integrating locomotor performance and behavior. Journal of Experimental Zoology Part A: Ecological Genetics and Physiology 313A, 59-79.
| Crossref | Google Scholar |
Downes S (2001) Trading heat and food for safety: costs of predator avoidance in a lizard. Ecology 82, 2870-2881.
| Crossref | Google Scholar |
Downes S, Shine R (1998) Heat, safety or solitude? Using habitat selection experiments to identify a lizard’s priorities. Animal Behaviour 55, 1387-1396.
| Crossref | Google Scholar |
Fritts TH, Rodda GH (1998) The role of introduced species in the degradation of island ecosystems: a case history of Guam. Annual Review of Ecology and Systematics 29, 113-140.
| Crossref | Google Scholar |
Glaudas X, Alexander GJ (2017) Food supplementation affects the foraging ecology of a low-energy, ambush-foraging snake. Behavioral Ecology and Sociobiology 71, 5.
| Crossref | Google Scholar |
Glaudas X, Kearney TC, Alexander GJ (2017) To hold or not to hold? The effects of prey type and size on the predatory strategy of a venomous snake. Journal of Zoology 302, 211-218.
| Crossref | Google Scholar |
Goetz SM, Nafus MG (2024) Guam, Andersen Air Force Base housing area visual surveys for brown treesnakes (Boiga irregularis) and associated tree data, December 2019–November 2022. U.S. Geological Survey data release. Available at https://doi.org/10.5066/P14Q2JCZ
Gordon DM (2011) The fusion of behavioral ecology and ecology. Behavioral Ecology 22, 225-230.
| Crossref | Google Scholar |
Hammond KA, Spotila JR, Standora EA (1988) Basking behavior of the turtle Pseudemys scripta: effects of digestive state, acclimation temperature, sex, and season. Physiological Zoology 61, 69-77.
| Crossref | Google Scholar |
Heithaus MR, Wirsing AJ, Burkholder D, Thomson J, Dill LM (2009) Towards a predictive framework for predator risk effects: the interaction of landscape features and prey escape tactics. Journal of Animal Ecology 78, 556-562.
| Crossref | Google Scholar |
Hoefer KM, Jayne BC (2013) Three-dimensional locations of destinations have species-dependent effects on the choice of paths and the gap-bridging performance of arboreal snakes. Journal of Experimental Zoology Part A: Ecological Genetics and Physiology 319, 124-137.
| Crossref | Google Scholar |
Hossie TJ, Murray DL (2011) Effects of structural refuge and density on foraging behaviour and mortality of hungry tadpoles subject to predation risk. Ethology 117, 777-785.
| Crossref | Google Scholar |
Jayne BC, Byrnes G (2015) The effects of slope and branch structure on the locomotion of a specialized arboreal colubrid snake (Boiga irregularis). Journal of Experimental Zoology Part A: Ecological Genetics and Physiology 323, 309-321.
| Crossref | Google Scholar |
Jayne BC, Riley MA (2007) Scaling of the axial morphology and gap-bridging ability of the brown tree snake, Boiga irregularis. Journal of Experimental Biology 210, 1148-1160.
| Crossref | Google Scholar |
Klecka J, Boukal DS (2014) The effect of habitat structure on prey mortality depends on predator and prey microhabitat use. Oecologia 176, 183-191.
| Crossref | Google Scholar |
Klug PE, Yackel Adams AA, Siers SR, Brindock KM, Mosher SM, Mazurek MJ, Pitt WC, Reed RN (2021) Locally abundant, endangered Mariana swiftlets impact the abundance, behavior, and body condition of an invasive predator. Oecologia 195, 1083-1097.
| Crossref | Google Scholar |
Klug PE, Yackel Adams AA, Reed RN (2022) Olfactory lures in predator control do not increase predation risk to birds in areas of conservation concern. Wildlife Research 49, 183-192.
| Crossref | Google Scholar |
Knox AJ, Lardner B, Yackel Adams A, Reed RN (2018) Evaluating airsoft electric guns for control of invasive brown treesnakes. Wildlife Society Bulletin 42, 534-539.
| Crossref | Google Scholar |
Lardner B, Savidge JA, Rodda GH, Reed RN (2009) Prey preferences and prey acceptance in juvenile brown treesnakes (Boiga irregularis). Herpetological Conservation and Biology 4, 313-323.
| Google Scholar |
Lenth R (2024) emmeans: estimated marginal means, aka least-squares means. R package version 1.10.0. Available at https://CRAN.R-project.org/package=emmeans
Mackessy SP, Sixberry NM, Heyborne WH, Fritts T (2006) Venom of the brown treesnake, Boiga irregularis: ontogenetic shifts and taxa-specific toxicity. Toxicon 47, 537-548.
| Crossref | Google Scholar |
Merems JL, Shipley LA, Levi T, Ruprecht J, Clark DA, Wisdom MJ, Jackson NJ, Stewart KM, Long RA (2020) Nutritional-landscape models link habitat use to condition of mule deer (Odocoileus hemionus). Frontiers in Ecology and Evolution 8, 98.
| Crossref | Google Scholar |
Montgomery RA, Vucetich JA, Peterson RO, Roloff GJ, Millenbah KF (2013) The influence of winter severity, predation and senescence on moose habitat use. Journal of Animal Ecology 82, 301-309.
| Crossref | Google Scholar |
Nafus MG, Gray LN (2024) Quantifying the importance of ontogeny and prey type in modeling top-down and bottom-up effects of an ectothermic predator. Scientific Reports 14, 21601.
| Crossref | Google Scholar |
Nafus MG, Savidge JA, Yackel Adams AA, Christy MT, Reed RN (2018a) Passive restoration following ungulate removal in a highly disturbed tropical wet forest devoid of native seed dispersers. Restoration Ecology 26, 331-337.
| Crossref | Google Scholar |
Nafus MG, Yackel Adams AA, Klug PE, Rodda GH (2018b) Habitat type and structure affect trap capture success of an invasive snake across variable densities. Ecosphere 9, e02339.
| Crossref | Google Scholar |
Nafus MG, Yackel Adams AA, Boback SM, Siers SR, Reed RN (2020) Behavior, size, and body condition predict susceptibility to management and reflect post-treatment frequency shifts in an invasive snake. Global Ecology and Conservation 21, e00834.
| Crossref | Google Scholar |
Nafus MG, Xiong PX, Paxton EH, Yackel Adams AA, Goetz SM (2021) Foraging behavior in a generalist snake (brown treesnake, Boiga irregularis) with implications for avian reintroduction and recovery. Applied Animal Behaviour Science 243, 105450.
| Crossref | Google Scholar |
Nafus MG, Siers SR, Levine BA, Quiogue ZC, Yackel Adams AA (2022) Demographic response of brown treesnakes to extended population suppression. The Journal of Wildlife Management 86, e22136.
| Crossref | Google Scholar |
Nafus MG, Collins AF, Viernes MC, Hopkins CB, Nacpil A (2023) Guam, USGS Closed Population (NWFN), an experimental eradication of brown treesnakes in a 5-ha study site, 2016–2023. U.S. Geological Survey data release. Available at https://doi.org/10.5066/P9QRWKQB
Nafus MG, Reyes A, Fies T, Goetz SM (2024a) Adaptive resource management: achieving functional eradication of invasive snakes to benefit avian conservation. Journal of Applied Ecology 61, 733-745.
| Crossref | Google Scholar |
Nafus MG, Barnhart PD, Collins AF, Goetz SM, Hopkins CB, Klug PE, Rodda GH, Viernes MC, Yackel Adams AA (2024b) Guam, data on the height at which brown treesnakes were detected at four sites on Guam, 2007–2023. U.S. Geological Survey data release. Available at https://doi.org/10.5066/P93K6LG5
Perry G, Pianka ER (1997) Animal foraging: past, present and future. Trends in Ecology & Evolution 12, 360-364.
| Crossref | Google Scholar |
R Core Team (2020) R – the R project for statistical computing. R Foundation for Statistical Computing, Vienna, Austria. Available at https://www.r-project.org/ [accessed February 2020]
Rodda GH (1992) Foraging behaviour of the brown tree snake, Boiga irregularis. Herpetological Journal 2, 110-114.
| Google Scholar |
Rodda GH, Fritts TH (1992) The impact of the introduction of the Colubrid Snake Boiga irregularis on Guam’s lizards. Journal of Herpetology 26, 166-174.
| Crossref | Google Scholar |
Rodda GH, Reed RN (2007) Size-based trends and management implications of microhabitat utilization by brown treesnakes, with an emphasis on juvenile snakes. In ‘Managing vertebrate invasive species: proceedings of an international symposium’. pp. 257–267. (US Department of Agriculture National Wildlife Research Center, National Wildlife Research Center)
Rodda GH, Fritts TH, Reichel J (1991) The distributional patterns of reptiles and amphibians in the Mariana Islands. Micronesica 24, 195-210.
| Google Scholar |
Rodda GH, Fritts TH, Conry PJ (1992) Origin and population growth of the brown tree snake, Boiga irregularis, on Guam. Pacific Science 46, 46-57.
| Google Scholar |
Rodda GH, Savidge JA, Tyrrell CL, Christy MT, Ellingson AR (2007) Size bias in visual searches and trapping of brown treesnakes on Guam. The Journal of Wildlife Management 71, 656-661.
| Crossref | Google Scholar |
Rodda GH, Dean-Bradley K, Savidge JA, Christy MT, Tyrrell CL (2008) Post-colonization reversal of selection pressure on dispersal behavior of the brown treesnake, Boiga irregularis, on Guam. South American Journal of Herpetology 3, 123-134.
| Crossref | Google Scholar |
Rodda GH, Dean-Bradley K, Campbell EW, Fritts TH, Lardner B, Yackel Adams AA, Reed RN (2015) Stability of detectability over 17 years at a single site and other lizard detection comparisons from Guam. Journal of Herpetology 49, 513-521.
| Crossref | Google Scholar |
Royama T (1981) Evaluation of mortality factors in insect life table analysis. Ecological Monographs 51, 495-505.
| Crossref | Google Scholar |
Savidge JA (1987) Extinction of an island forest avifauna by an introduced snake. Ecology 68, 660-668.
| Crossref | Google Scholar |
Savidge JA (1988) Food habits of Boiga irregularis, an introduced predator on Guam. Journal of Herpetology 22, 275-282.
| Crossref | Google Scholar |
Secor SM, Nagy KA (1994) Bioenergetic correlates of foraging mode for the snakes Crotalus Cerastes and Masticophis Flagellum. Ecology 75, 1600-1614.
| Crossref | Google Scholar |
Siers SR, Yackel Adams AA, Reed RN (2018) Behavioral differences following ingestion of large meals and consequences for management of a harmful invasive snake: a field experiment. Ecology and Evolution 8, 10075-10093.
| Crossref | Google Scholar |
Skelhorn J, Ruxton GD (2013) Size-dependent microhabitat selection by masquerading prey. Behavioral Ecology 24, 89-97.
| Crossref | Google Scholar |
Taylor Q, Cox CL (2019) Evidence of predation risk increases with body size in a diminutive snake. Journal of Zoology 307, 141-148.
| Crossref | Google Scholar |
Thaler R, Ortega Z, Ferreira VL (2022) Extrinsic traits consistently drive microhabitat decisions of an arboreal snake, independently of sex and personality. Behavioural Processes 199, 104649.
| Crossref | Google Scholar |
Tversky A, Simonson I (1993) Context-dependent preferences. Management Science 39, 1179-1189.
| Crossref | Google Scholar |
USGS (2023) Cocos Island, Guam Brown Treesnake Rapid Response visual survey and capture data, 10/2020-05/2023. U.S. Geological Survey data release. Available at https://doi.org/10.5066/P9MT1JNO
van Oers K, Klunder M, Drent PJ (2005) Context dependence of personalities: risk-taking behavior in a social and a nonsocial situation. Behavioral Ecology 16, 716-723.
| Crossref | Google Scholar |
Webb JK, Whiting MJ (2005) Why don’t small snakes bask? Juvenile broad-headed snakes trade thermal benefits for safety. Oikos 110, 515-522.
| Crossref | Google Scholar |
Wiewel AS, Yackel Adams AA, Rodda GH (2009a) Distribution, density, and biomass of introduced small mammals in the southern Mariana Islands. Pacific Science 63, 205-222.
| Crossref | Google Scholar |
Wiewel AS, Adams AAY, Rodda GH (2009b) Evaluating abundance estimate precision and the assumptions of a count-based index for small mammals. The Journal of Wildlife Management 73, 761-771.
| Crossref | Google Scholar |
Wiles GJ (1987) The status of fruit bats on Guam. Pacific Science 41, 148-157.
| Google Scholar |
Wiles GJ, Bart J, Beck RE, Jr., Aguon CF (2003) Impacts of the brown tree snake: patterns of decline and species persistence in Guam’s avifauna. Conservation Biology 17, 1350-1360.
| Crossref | Google Scholar |
Yackel Adams AA, Nafus MG, Klug PE, Lardner B, Mazurek MJ, Savidge JA, Reed RN (2019) Contact rates with nesting birds before and after invasive snake removal: estimating the effects of trap-based control. NeoBiota 49, 1-17.
| Crossref | Google Scholar |
Yeo M-H, Patil UD, Chang A, King R (2023) Changing trends in temperatures and rainfalls in the western Pacific: Guam. Climate 11, 81.
| Crossref | Google Scholar |