Foraging habitat locations of flatback (Natator depressus) and olive ridley turtles (Lepidochelys olivacea) in northern Australia
Justin S. Smith A , Colin J. Limpus B , Takahiro Shimada A B , Laurie Booth C , Eve C. Hinchliffe

A
B
C
D
Abstract
Understanding the spatial ecology of endangered species is important for their management. With flatback turtles (Natator depressus) Vulnerable and the olive ridley turtle (Lepidochelys olivacea) Endangered under Australia’s EPBC Act 1999, it is important to understand their movement activity to assess exposure to threats better and appropriately manage population demographics.
Describe, quantify, and compare the foraging patterns of flatback turtles from the Arafura Sea genetic stock and olive ridley turtles from the eastern Gulf of Carpentaria (GoC) genetic stock.
Satellite telemetry was used to track post-nesting flatbacks (n = 16) and olive ridleys (n = 8) to identify foraging-activity behaviour and locations between 2013 and 2017.
Overall, core foraging home ranges showed considerable variability from strong site fidelity to extensive spatial movement (flatback: 1–1065 km2, olive ridley: 2–113 km2). Foraging areas for both species were identified to cross over state and international boundaries (Torres Strait, eastern GoC, western GoC, Kakadu coast, Joseph Bonaparte Gulf and the Arafura Sea within Australia and Indonesia), with nine individuals foraging in Indonesian waters and five foraging in both Indonesian and Australian waters.
Flatback and olive ridley turtles in northern Australia have a large, widespread spatial distribution with varying use levels across the space. Foraging hot spots identified in this study can improve and guide the designation of biologically important areas. Inter- and intra-specific overlaps between foraging grounds were consistent with previous research, strengthening the understanding of foraging hot spots for flatbacks and olive ridleys in Indonesia and the Joseph Bonaparte Gulf.
This study thus emphasises the importance of area-based management to benefit highly mobile species from multiple populations and taxa, potentially from all life-cycle phases.
Keywords: competition, distribution, flatback, foraging, management, movement, olive ridley, satellite-tracking, spatial ecology.
Introduction
Understanding the spatial ecology of threatened migratory species is a priority for conservation and management (Hyrenbach et al. 2000; Shuter et al. 2011). The spatial distribution of migratory species varies extensively, within and among species, particularly throughout foraging periods (Hussey et al. 2015). Foraging areas can support several genetic stocks of multiple taxa, occurring across administrative and legislative boundaries (Blumenthal et al. 2006; Whiting et al. 2007; Whittock et al. 2016) or representing strong fidelity with highly localised, well-defined feeding locations (Hawkes et al. 2006; Schofield et al. 2010).
It is challenging to manage threats to species or populations that undertake large-scale movements or use large home ranges, such as marine turtles, because they can occur across jurisdictional boundaries and often overlap with multiple anthropogenic stressors (Hays et al. 2019; Miller et al. 2019). Further, travel between various habitats, such as those used for breeding and foraging, could mean that turtles move between areas with different levels of threat exposure, such as protected breeding areas and foraging areas with high shipping traffic or fishery pressures (Blumenthal et al. 2006; Pendoley et al. 2014; Thums et al. 2017). As such, understanding spatial movements of individuals or species can provide robust information for conservation by detailing specific habitats and migration corridors, while highlighting potential threats and population implications (Lucchetti et al. 2016; Fuentes et al. 2020).
Satellite telemetry provides an advanced understanding of spatial distribution and movement behaviours of marine species (Hays and Hawkes 2018). Individuals can be tracked over extended periods, but briefly within the overall life span of the turtles, and their fine-scale movements can be monitored with high position accuracy (Godley et al. 2008). Satellite telemetry, such as fastloc GPS, coupled with data-filtering measures and application techniques, allows finer-scale detail of movement characteristics, indicating specific spatial and temporal attributes of movement behaviour, such as home ranges and movement within them (Godley et al. 2002; Polovina et al. 2004). Consequently, satellite-tracking is increasingly able to provide the information necessary to inform management and conservation policies (Hays et al. 2019; Armstrong et al. 2020; Santos et al. 2021). Increases in available data can aid implementation and inform management initiatives at local and regional scales. They may indicate specific threat implications and species interactions at remote localities within complex environments such as the broad expanse of neritic waters of northern Australia–New Guinea continental shelf (Shimada et al. 2017).
Olive ridley (Lepidochelys olivacea) and flatback (Natator depressus) marine turtles are generally less understood than are other marine turtle species breeding within Australia, with knowledge of their foraging ecology in northern Australia particularly lacking (Limpus 2009; Department of Environment and Science 2021). The paucity of information is likely to be due to remote nesting locations, foraging areas and non-specific migratory paths (Limpus 2009). In general, both species typically inhabit the broad expanse of subtidal neritic waters of the continental shelf of remote, tropical, northern Australia and southern New Guinea (Robins et al. 2002; Whittock et al. 2016), restricting available survey methods (Whiting et al. 2007), except via fisheries bycatch data (Poiner et al. 1990). Efforts to determine spatial coverage and abundance in northern Australia have been made for both flatback and olive ridley turtles, and, although limited, these data have provided a generic understanding of species distribution, foraging areas and areas that are exposed to threats (Robins 1995; Dryden et al. 2008; Wilcox et al. 2013; Riskas et al. 2016). However, ecological information at a smaller habitat-use scale and migration routes are lacking, hindering targeted protection/management efforts or designation of critical habitat.
Consequently, this study aims to determine and describe the foraging locations of flatback turtles from the Arafura Sea (AS) genetic stock and olive ridley turtles of the eastern Gulf of Carpentaria (GoC) genetic stock (FitzSimmons and Limpus 2014) by using satellite telemetry. To achieve these aims, we attached satellite-tracking tags to post-nesting female turtles to discover the spatial and temporal characteristics of their post-nesting foraging activity. This will provide insight into the spatial ecology of these species that can be used to develop and implement management strategies that mitigate adverse anthropogenic pressures on marine turtle populations in northern Australia.
Materials and methods
Study species and study sites
Between 2008 and 2017 (predominantly 2013–2017), satellite transmitters were attached to 16 flatback turtles (Waral Kawa [=Deliverance Island] n = 11, Greenhill Island n = 2, Jardine River n = 2 and Crab Island n = 1) and olive ridley turtles (Western Cape York Peninsula/eastern GoC (n = 8) (Table 1)). All turtles were tagged and tracked during the peak of their nesting season.
Species | Turtle ID | Location | Transmitter type | Release date | Time tracked (days) | |
---|---|---|---|---|---|---|
N. depressus | 108468 | WK | WC Splash10 – GPS | 26/2/2013 | 413 | |
N. depressus | 54530 | WK | WC Splash10 – GPS | 26/2/2013 | 133 | |
N. depressus | 64208 | WK | WC Splash10 – GPS | 26/2/2013 | 223 | |
N. depressus | 95894 | WK | WC Splash10 – GPS | 26/2/2013 | 586 | |
N. depressus | 95896 | WK | WC Splash10 – GPS | 26/2/2013 | 593 | |
N. depressus | 133406 | WK | WC Splash10 – GPS | 4/3/2014 | 69 | |
N. depressus | 133407 | WK | WC Splash10 – GPS | 4/3/2014 | 95 | |
N. depressus | 133410 | WK | WC Splash10 – GPS | 4/3/2014 | 95 | |
N. depressus | 140117 | JR | WC Splash10 – GPS | 1/4/2014 | 344 | |
N. depressus | 140119 | JR | WC Splash10 – GPS | 12/9/2014 | 61 | |
N. depressus | 111064 | GI | WC SPOT5 – Argos | 11/7/2013 | 332 | |
N. depressus | 129555 | GI | WC SPOT5 – Argos | 10/7/2013 | 266 | |
N. depressus | 88239 | CI | Sirtrack – PTT | 5/11/2008 | 871 | |
N.depressus | 133409 | WK | WC Splash10 – GPS | 4/03/2014 | 43 | |
N. depressus | 133405 | WK | WC Splash10 – GPS | 4/03/2014 | 50 | |
N. depressus | 133408 | WK | WC Splash10 – GPS | 4/03/2014 | 76 | |
L. olivacea | K65252_48845 | FB | Sirtrack – GPS | 5/08/2013 | 43 | |
L. olivacea | K90229_133765 | FB | WC Splash10 – GPS | 3/08/2017 | 62 | |
L. olivacea | QA39899_140118 | SWB | WC Splash10 – GPS | 24/7/2014 | 82 | |
L. olivacea | K90108_48850 | FB | Sirtrack – GPS | 7/08/2015 | 75 | |
L. olivacea | QA14005_48850 | FB | Sirtrack – GPS | 16/8/2013 | 80 | |
L. olivacea | QA14046_48845 | FB | Sirtrack – GPS | 1/08/2014 | 61 | |
L. olivacea | QA23520_48850 | FB | Sirtrack – GPS | 15/8/2014 | 74 | |
L. olivacea | QA57043_48845 | FB | Sirtrack – GPS | 5/8/2015 | 92 |
Tagging location of the turtle (WK, Waral Kawa; JR, Jardine River; GI, Greenhill Island; CI, Crab Island; FB, Flinders Beach; SWB, South Wik Beach), transmitter type (WC, Wildlife Computers), release date and time tracked (days) are specified.
Transmitter application
After a successful nesting attempt, satellite tags were attached using one of two methods. Units were attached to flatback turtles by using a specialised harness with a pre-attached transmitter (see Sperling and Guinea 2004). The harness was adjusted to have the transmitter positioned above the top vertebral scute for best transmission performance (Whittock et al. 2014). Turtles were retained on the beach for ~30 min after they had laid eggs and released after transmitter deployment. Transmitters were directly attached to olive ridley turtles on the top vertebral scute by using fibreglass tape and Sika Anchorfix 3+ resin covering five surrounding scutes to maximise adhesion and unit retention (Gredzens et al. 2014; Gredzens and Shaver 2020). Before attachment, the carapace scutes of the olive ridleys were cleaned of algae and barnacles. Anti-fouling paint was applied to the transmitters to reduce biofouling and premature loss of tag function. For both species, only turtles bearing no flipper or carapace damage were selected for tag attachment. Each turtle was measured for curved carapace length and fitted with titanium flipper tags to provide identification to each turtle. PIT tags were also injected into the upper left shoulder of the olive ridley turtles.
Tags and transmission
The following three different models of transmitter were used in the study: SPOT5 (Argos) and Splash10 (Argos-Fastloc GPS) from Wildlife Computers and Argos only and Argos-fastloc-GPS units from Sirtrack Ltd (Table 1). Each GPS tracking unit was programmed to run for 24 h every day for the first 4 months and then every second day for the remainder of its deployment. The tags were programmed to record two positions every hour, with up to 10 repeated attempts following a failed record.
Data processing
Data were collected via Argos and/or Wildlife Computer Internet systems. To remove erroneous data implausible for marine turtle movement, locations were filtered and screened by spatial and temporal duplicates and a data-driven filter as described in Shimada et al. (2012, 2016) by using the R package SDLfilter (Shimada et al. 2012, 2016). Filtering criteria included the following: (1) only using location data for Argos (LC 1, 2 and 3) and only using more than five satellites for GPS; (2) travel between two successive points could not exceed 5 km/h; and (3) locations with turning angles between two successive locations of <25° were dismissed. Data are presented as means ± s.d.
Foraging behaviour was identified when the turtle was deemed to have finished migrating from her nesting site. This was determined using daily movement data, and the end of migration was designated when movement ceased to be in a uniform direction and when the daily speed of movement (using distance and time between successive points) decreased, and daily locations became aggregated in a particular location (as per Barr et al. 2021).
Foraging home ranges
Location data of specific foraging periods were filtered (processed as above) for each turtle to generate fixed kernel density (FKD) estimates for individual foraging areas. This non-parametric analysis highlights areas of use within a home-range boundary. Utilisation distributions (UD) of 50% and 95% were calculated to represent the core areas of foraging (50%), while providing a representation of total home-range movements (95%). UD estimates were calculated for each turtle by using movement-based kernel-density estimation with biased random bridges, by using the adehabitatHR package (version 0.4.21) in R software (version 4.2.1)(Calenge 2006; Benhamou 2011), and all polygons were mapped using ArcGIS Pro (ver. 3.0.0).
Seasonal foraging
The data from two individual flatback turtles (ID: 95894 and 95896) that were tracked using GPS tags for more than a year were examined to determine whether there was a difference in spatial use patterns over longer time scales. Data were filtered and processed (as above), and then home ranges were constructed to achieve 50% and 95% UD for every 3 months of data for each turtle, aligning with the seasons in the southern hemisphere (summer: December–February; autumn: March–May; winter: June–August; and spring: September–November). Overlap in the foraging area across successive seasons was quantified in ArcGIS Pro (ver. 3.0.0) by overlaying 50% and 95% UDs of the foraging areas from successive seasons and calculating the area of the new foraging areas for each change in season.
Bathymetry
The bathymetry layers (100 m horizontal resolution) of the study region were sourced and downloaded from General Bathymetric Charts of the Ocean (available at gebco.net, accessed 21 April 2023). However, foraging area and depth use were quantified by combining turtles’ positions with associated depth data from bathymetry information in ArcGIS Pro to calculate a depth corresponding to each location and then an average water depths (m) for individual home ranges.
Results
Foraging home range
Flatback turtles were tracked for a mean of 264 ± 61.39 days (range: 43–871), and olive ridley turtles were tracked for a mean of 71 ± 5.40 days (range: 43–92) (Table 1). Most individuals of both species showed comprehensive movement (i.e. 95% UD) around a core (50% UD) foraging areas, which ranged from 1 to 1065 km2 (mean value 173 km2, n = 16) (Fig. 1) and from 2 to11 km2 (mean value 33 km2, n = 8) (Fig. 2) for flatback and olive ridley turtles respectively (Table 2).
Distribution of flatback turtle migration and foraging areas (50% and 95% utilisation distributions) for individuals tracked from northern Australian rookeries. Tagging sites L–R; Greenhill Island, Waral Kawa, Jardine River.
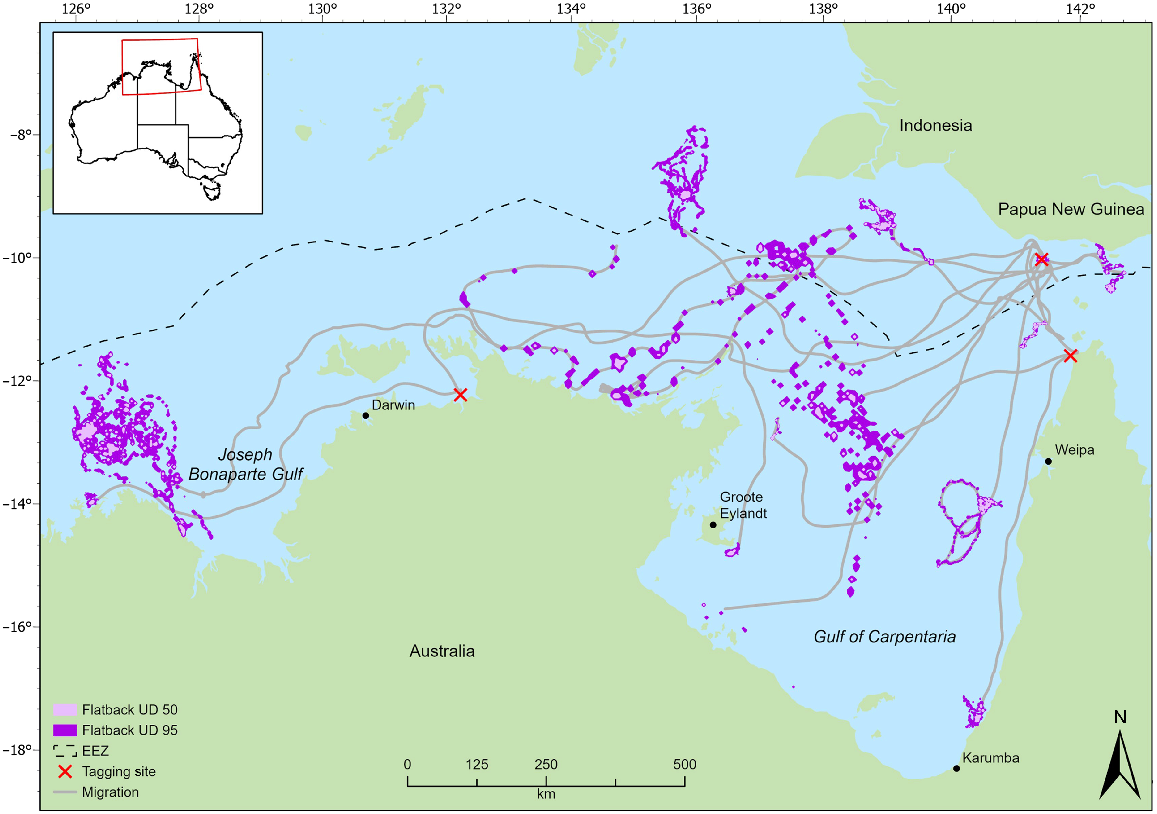
Distribution of olive ridley turtle migration and foraging areas (50% and 95% utilisation distributions) for individuals tracked from north-eastern Gulf of Carpentaria (western Cape York Peninsula) rookeries. Inserted boxes represent foraging areas identified by prior studies: (a) McMahon et al. (2007) and Whiting et al. (2007); (b) Whiting et al. (2007); (c) Whiting et al. (2007); Fukuoka et al. (2022); (d) McMahon et al. (2007); Fukuoka et al. (2022); and (e) McMahon et al. (2007). Tagging site is Mapoon.
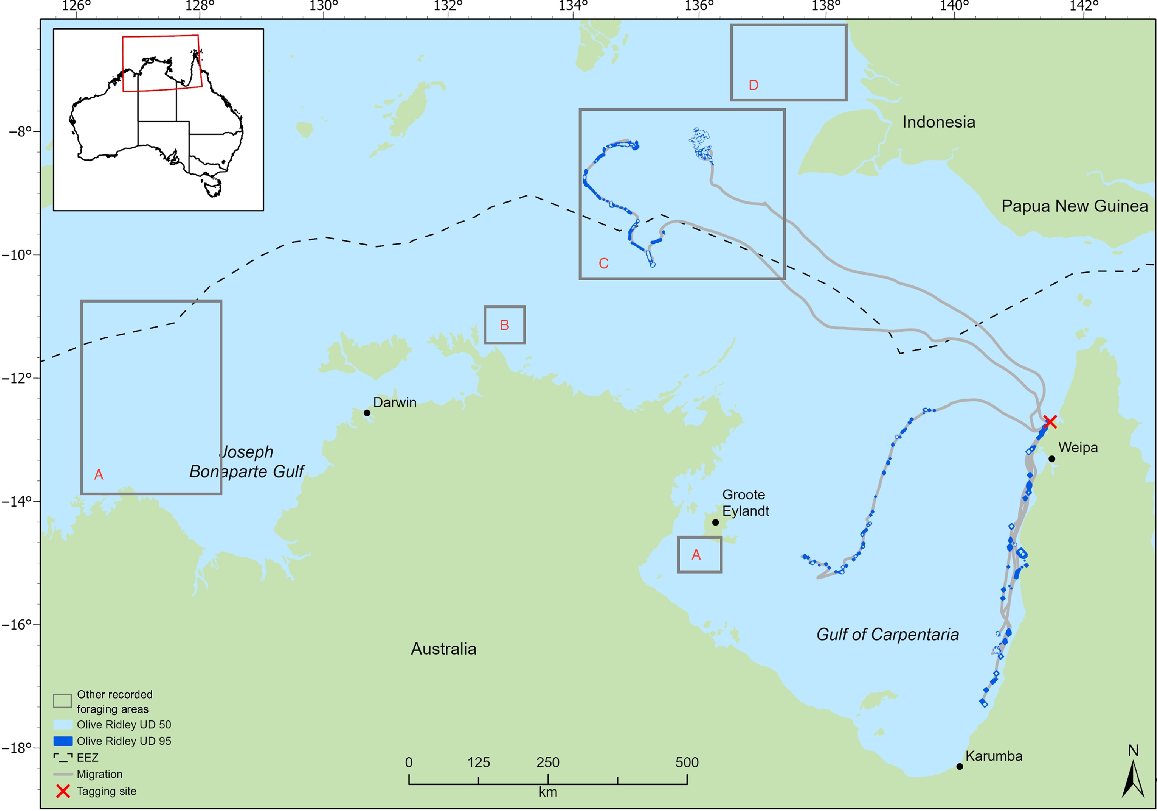
Species | Turtle ID | Foraging time (days) | Core foraging area 50% UD (km2) | Foraging area 95% UD (km2) | Average depth used (m) | |
---|---|---|---|---|---|---|
N. depressus | 108468 | 215 | 257 | 5811 | −27.73 | |
N. depressus | 54530 | 58 | 212 | 1375 | −28.75 | |
N. depressus | 64208 | 162 | 257 | 2795 | −62.39 | |
N. depressus | 95894 | 521 | 1065 | 12410 | −69.97 | |
N. depressus | 95896 | 567 | 532 | 11369 | −56.73 | |
N. depressus | 133406 | 32 | 52 | 253 | −14.24 | |
N. depressus | 133407 | 63 | 31 | 162 | −57.87 | |
N. depressus | 133410 | 80 | 125 | 1103 | −49.31 | |
N. depressus | 140117 | 103 | 67 | 530 | −3.66 | |
N. depressus | 140119 | 47 | 32 | 306 | −6.18 | |
N. depressus | 111064 | 269 | 47 | 250 | −34.26 | |
N. depressus | 129555 | 232 | 31 | 232 | −15.17 | |
N. depressus | 88239 | 808 | 1 | 74 | −20.7 | |
N. depressus | 133409 | 17 | 30 | 218 | −17.91 | |
N. depressus | 133405 | 50 | 25 | 114 | −2.28 | |
N. depressus | 133408 | 8 | 10 | 77 | −53.71 | |
L. olivacea | K65252_48845 | 13 | 2 | 11 | −14.54 | |
L. olivacea | K90229_133765 | 10 | 3 | 20 | −16.61 | |
L. olivacea | QA39899_140118 | 77 | 12 | 328 | −2.81 | |
L. olivacea | K90108_48850 | 52 | 19 | 600 | −60.61 | |
L. olivacea | QA14005_48850 | 30 | 113 | 623 | −51.74 | |
L. olivacea | QA14046_48845 | 61 | 28 | 1134 | −12.94 | |
L. olivacea | QA23520_48850 | 22 | 16 | 71 | −14.25 | |
L. olivacea | QA57043_48845 | 32 | 76 | 1099 | −110.90 |
The tracking data identified foraging areas for flatbacks in regions of the northern Torres Strait, south-eastern GoC, central GoC, Kakadu coast, Northern Territory, Joseph Bonaparte Gulf, Western Australia, and Indonesia (Fig. 1). Home-range analyses for the olive ridleys identified four separate foraging areas, namely, two in Indonesian waters, one in eastern GoC and one in the central GoC (Fig. 2). Of the four olive ridley foraging areas identified in this study, two were within a previously identified foraging area (Fig. 2).
All individuals demonstrated multiple interconnected core foraging areas (Figs 1, 2). Seven individuals had foraging areas in Indonesian waters of the Arafura Sea (flatback: 64208, 95896, 108468, 54530, 133406; olive ridley: QA14005_48850, QA57043_48845) and two had foraging areas in the Papua New Guinean region of the coral sea (133405, 140119); five of these individuals also recorded foraging areas in Australian waters. Overall, we identified foraging areas in Indonesian waters for flatback (n = 7) and olive ridley (n = 2) turtles from breeding sites in northern Australia.
There was an overlap in the overall home range between the two species (95% UD) at three separate locations. First, there is an area of 54.8 km2 in Indonesian waters approximately 180 km west of Indonesia and Papua New Guinea, shared by a flatback individual from Waral Kawa (64208) and an olive ridley individual from Flinders Beach (QA14005_48850) (Figs 1, 2). Second, there is an area in the central GoC, 320 km north of the Northern Territory coastline and 250 km off the Queensland coastline, which supports foraging by both species. Flatback 95896 and olive ridley (K90108_48850) overlapped 21.7 km2 in this area. The third area utilised by both species is located in the eastern GoC, approximately 10 km from the Queensland coast, covering 56.7 km2. Here, there was an overlap between a flatback tracked from Crab Island (140117) and an olive ridley tracked from Mapoon (QA14046_48845). The area of overlap specified in Indonesian waters has also been used by turtles tracked in previous studies (Fig. 2; Whiting et al. 2007; Fukuoka et al. 2022).
Seasonal foraging
Two flatback individuals (95894 and 95896) used large foraging areas over the study duration (Table 3). Analysis of seasonal changes in foraging activity indicated that turtles 95894 (Fig. 3) and 95896 (Fig. 4) show distinct seasonal shifts in the location of the 50% UD area, with most consecutive seasons showing more than 90% of season-to-season shifts of core foraging to have changed (Table 4). However, the overlap of 95% UD is more prevalent for both individuals between seasons and a lower percentage, but still >60%, the percentage of area occupied has changed (Table 5). For both individuals, there was little consistency in the use of space across corresponding seasons when examining core foraging (50%UD) activity (Figs 3, 4).
Season | 95894 UD 95 (UD 50) (km2) | 95896 UD 95 (UD 50) (km2) | |
---|---|---|---|
Autumn 2013 | 2168 (211) | 1757 (88) | |
Winter 2013 | 3184 (191) | 2906 (125) | |
Spring 2013 | 3296 (321) | 1988 (207) | |
Summer 2013/2014 | 1724 (258) | 1372 (66) | |
Autumn 2014 | 2927 (473) | 2964 (150) | |
Winter 2014 | 1711 (191) | 1199 (211) | |
Spring 2014 | 1587 (222) | 1333 (211) |
Individual foraging core area (50% UD) for flatback individual 95894 in the Joseph Bonaparte Gulf, highlighting space use among and within seasons.
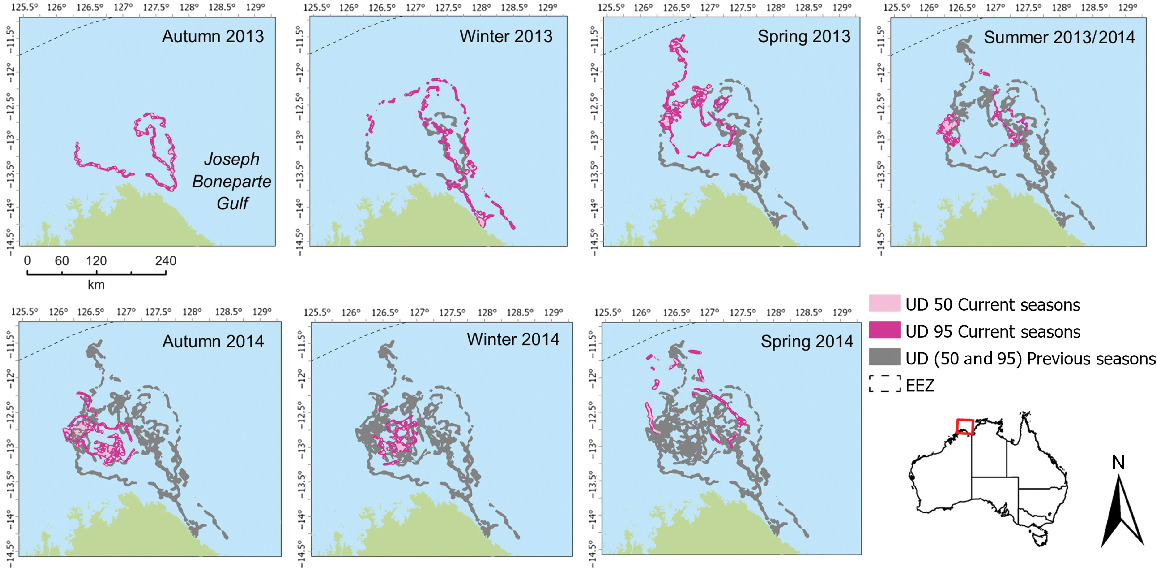
Individual foraging core area (50% UD) for individual 95896 in the Gulf of Carpentaria among seasons, highlighting space use among and within seasons.
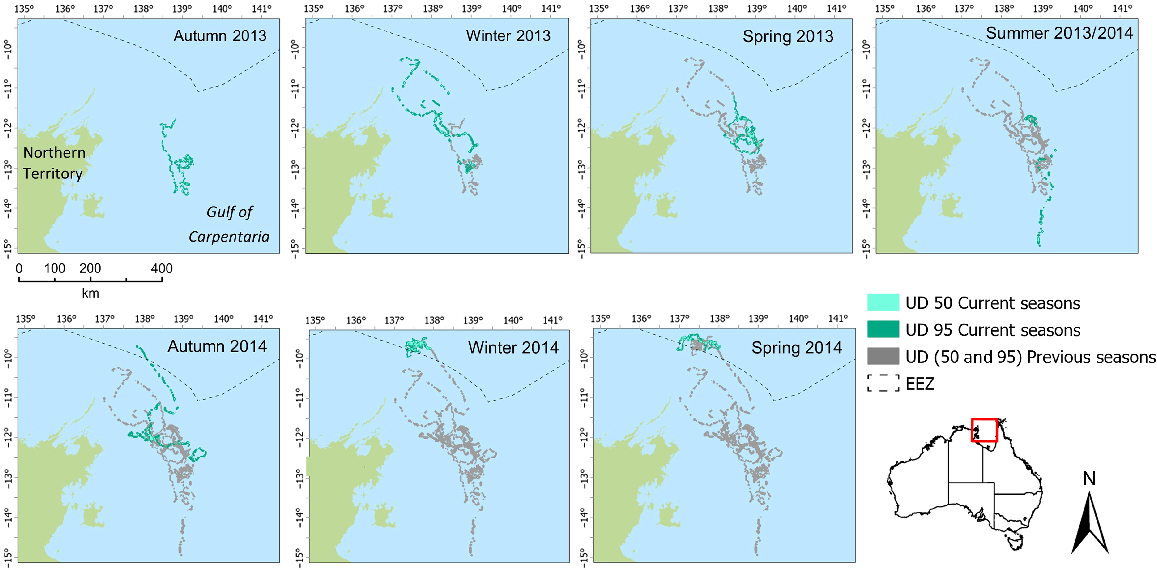
Consecutive seasons | 95894 UD 50 (%) | 95894 UD 50 (km2) | 95896 UD 50 (%) | 95896 UD 50 (km2) | |
---|---|---|---|---|---|
Autumn 2013 – winter 2013 | 99 | 189 | 100 | 125 | |
Winter 2013 – spring 2013 | 99 | 318 | 98 | 203 | |
Spring 2013 – summer 2013/2014 | 90 | 233 | 96 | 63 | |
Summer 2013/2014 – autumn 2014 | 89 | 422 | 100 | 105 | |
Autumn 2014 – winter 2014 | 70 | 136 | 100 | 211 | |
Winter 2014 – spring 2014 | 100 | 222 | 91 | 190 |
Consecutive seasons | 95894 UD 95 (%) | 95894 UD 95 (km2) | 95896 UD 95 (%) | 95896 UD 95 (km2) | |
---|---|---|---|---|---|
Autumn 2013 – winter 2013 | 89 | 2822 | 96 | 2776 | |
Winter 2013 – spring 2013 | 90 | 2959 | 88 | 1758 | |
Spring 2013 – summer 2013/2014 | 81 | 1391 | 89 | 1220 | |
Summer 2013/2014 – autumn 2014 | 79 | 2341 | 99 | 2946 | |
Autumn 2014 – winter 2014 | 61 | 1037 | 98 | 1169 | |
Winter 2014 – spring 2014 | 99 | 1578 | 75 | 996 |
Bathymetry
The foraging grounds of each flatback and olive ridley differed in the average bathymetry profile, ranging from 2 m to 69 m and 2 to 110 m of depth respectively (Table 2). There were two flatbacks and one olive ridley that had foraging home ranges close to the coastline, and thus, the average depth uses of these turtles was <10 m. In contrast, four flatbacks and two olive ridleys used mean water depth >50 m. The average seabed depth was similar across foraging habitats used by both species, with flatback foraging areas averaging 32 ± 5 m deep and olive ridleys foraging areas averaging 35 ± 13 m deep.
Discussion
Post-nesting flatback and olive ridley turtles in northern Australia have large spatial distributions and widely dispersed foraging grounds across different jurisdictions. In the longest flatback dispersal, one turtle moved 1660 km over 55 days from Waral Kawa in Torres Strait to a foraging area in north-western Western Australia. The longest olive ridley migration was 844 km over 50 days from Mapoon, Queensland, into Indonesian waters. Our data are similar to other data from the species elsewhere in Australia, which collectively demonstrates that both species move long distances between nesting and foraging grounds and variable-sized foraging areas are distributed exclusively within neritic continental shelf waters of northern Australia–southern New Guinea (McMahon et al. 2007).
Home-range analysis for flatbacks showed high inter-individual variation in the size of the core (50% UD) foraging area, ranging from 1 to 1065 km2 (mean = 173 km2). It consequently demonstrated that flatback turtles in this region can move extensively during foraging across large areas, and, in general, more than any other marine turtle species tracked for similar durations in Australia (Block et al. 2011; Gredzens et al. 2014; Shimada et al. 2016, 2020; Barr et al. 2021). Similarly, analysis of olive ridley home ranges showed a large variation in core (50% UD) areas of 2 to 113 km2 (mean = 34 km2) for four individuals throughout the GoC (n = 2) and Indonesian waters (n = 2). The upper limit of the home-range area is similar to that found for individuals tracked from the Northern Territory, where Whiting et al. (2007) found olive ridley home ranges to vary between 138 and 1260 km2. Together, these results demonstrate that olive ridley turtles can forage over large areas. Still, the results of the present study also indicated that individual olive ridley and flatback turtles can constrict their foraging activity to a small specific region, presumably if resources allow.
When comparing the foraging areas identified in this study to results from other studies, the partial overlap of foraging areas between females from genetically distinct populations is evident (Whittock et al. 2016). In particular, the collective research conducted on the spatial ecology of flatback and olive ridley turtles in northern Australia has indicated that the central and northern waters of the Arafura Sea, including those in Indonesian waters, are important foraging sites for both species, with nine individuals using multiple foraging core areas (50% UD). In addition, it has given additional strength to justify a regionally significant foraging area in the Joseph Bonaparte Gulf region of Northern Territory, Western Australia, and the adjacent Commonwealth waters. This strengthens the need for cooperation in the management or development of regional-focused strategies for managing pressures on the marine environment across the northern Australian and southern Indonesia region (Miller et al. 2020; Pilcher and Welly 2021).
Two flatback individuals, tracked for over a year, displayed seasonal-based variations in their foraging home ranges. In both cases, turtles used separate 50% UD core foraging areas across seasons, highlighting the potential for temporal variation in foraging areas. Previous evidence of seasonal influence on adult marine turtle foraging habitat use was recorded for green and loggerhead turtles in southern Queensland and loggerhead turtles in the Mediterranean (Shimada et al. 2016; Dujon et al. 2018). Although the spatial scales are different, and the green turtles moved only short distances, in this study, the general pattern of our results is similar in that the tracked turtles used different foraging areas across seasons. Flatback turtles seem to require large areas relative to some other hard-shelled turtles (e.g. greens, loggerheads, hawksbills), presumably because their primary diet is sparsely distributed or less predictable benthic and planktonic soft-bodied invertebrates (Limpus 2009). That said, while there are no data from any study to indicate whether flatback turtles have the capacity to react to changes in food accessibility, if they are like other hard-shelled species, they will tend to remain in their familiar home habitats, possibly because shifting locations to unknown areas could be too risky, for example, because of poor knowledge of food locations or shelter from predators in the new habitat. Therefore, despite the occupancy of large areas, flatback turtles are likely to be vulnerable to aperiodic changes in habitat quality or availability of food.
From bathymetry projections, we can infer that flatback and olive ridleys typically reside in habitats deeper than 10 m, which supports knowledge from previous research and studies of bycatch (Poiner et al. 1990; Robins 1995; Whittock et al. 2016; Wildermann et al. 2017). All home ranges indicated a foraging depth range that extended from 2 to 70 m for flatbacks and from 3 to 111 m for olive ridley turtles. However, correlating spatial-use data with spatial bathymetric data does not provide information on an individual’s diving behaviour and depth use. Future studies could combine telemetry with depth recorders to obtain specific data on dive behaviour and foraging (e.g. Hounslow et al. 2023). However, our data concur with those of studies by Whiting et al. (2007), Whittock et al. (2016) and Thums et al. (2017), which indicated that flatbacks from the Pilbara populations use mean water depths of 36.5 ± 22.5 m, whereas olive ridley populations from Northern Territory rookeries are reported to forage in water depths between 20 and 150 m, also on the basis of bathymetry information rather than diving data.
Foraging data show that flatbacks and olive ridleys use coastal and open-water habitats from nearshore areas and up to 225 km offshore. From previous tracking overlap, we can confirm that the foraging areas identified within the Joseph Bonaparte Gulf are remote, difficult to access and comprise various habitat types (Whiting et al. 2007; Whittock et al. 2016). Given the remoteness of the area, little information is available on bathymetry and biophysical features of this region. Inference of resource quality and availability can be made only in certain areas concerning fisheries’ productivity or satellite-derived chlorophyll layers, and this will not illuminate the trophic structure and complete available food source. Flatbacks feed primarily on benthic and planktonic soft-bodied invertebrates (Limpus 2009), reacting to specific environmental predictors influencing prey distribution (Wildermann et al. 2017). Benthic-community variability may significantly affect species distribution and foraging behaviours, because individuals must constantly move to follow mobile or unreliable food sources or seek specific habitats. Information on the triggers of community variability in particular regions may be used to indicate potential population movement and behaviour. Further dietary-analysis details will help highlight and determine the significant resources of both species, providing possible insight into population distribution patterns and may aid in management initiatives of specific populations and habitat areas.
Further research into the links between space use and foraging ecology may explain and determine resource use and availability for marine turtles in the northern Australian region. Identifying the predictors that drive responses can help determine species and population distribution patterns. However, telemetry-tracking of a small number of turtles from restricted breeding sites within a stock will almost certainly underestimate the actual foraging range of marine turtles (Shimada et al. 2021; Perez et al. 2022). Flipper-tag recoveries (n = 3) from flatback turtles tagged while nesting at eastern Gulf of Carpentaria nesting beaches for the AS genetic stock have been recorded within Papua New Guinea coastal waters up to 500 km east of Torres Strait (C. J. Limpus, unpubl. data). Overall, this study has shown that flatbacks and olive ridleys of northern Australia are capable of large-scale movement patterns, reside in large foraging habitats and exhibit intra-annual shifts in their core habitats. Future research should concentrate on elucidating the factors influencing these behaviours. Focusing on spatial population ecology, resource type, and availability will help develop a management protocol to aid species recovery by outlining potential threats from known spatial and temporal movement characteristics, identifying the resources that may influence specific distribution and setting boundaries of areas such as biologically important areas, or as a basis for other spatial-based threat mitigation.
Declaration of funding
The work conducted at Waral Kawa was part of a project funded by NERP to understand marine turtles in northern Torres Strait by the Torres Strait Regional Authority and Rangers of Boigu Island. The olive ridley tracking was funded by the Queensland Department of Environment and Science.
Acknowledgements
We acknowledge the Traditional Owners of the land and sea country in which the study took place. We thank Janine Ferguson for technical support and the Mapoon Land and Sea Rangers and the Torres Strait Regional Authority Land and Sea Rangers for facilitating approvals, transport and participation in fieldwork. The work conducted at Waral Kawa was part of a larger Land and Sea Management project of the Torres Strait Regional Authority and Rangers of Boigu Island.
References
Armstrong AJ, Armstrong AO, McGregor F, Richardson AJ, Bennett MB, Townsend KA, Hays GC, van Keulen M, Smith J, Dudgeon CL (2020) Satellite tagging and photographic identification reveal connectivity between two UNESCO world heritage areas for reef manta rays. Frontiers in Marine Science 7, 725.
| Crossref | Google Scholar |
Barr CE, Hamann M, Shimada T, Bell I, Limpus CJ, Ferguson J (2021) Post-nesting movements and feeding ground distribution by the hawksbill turtle (Eretmochelys imbricata) from rookeries in the Torres Strait. Wildlife Research 48(7), 598-608.
| Crossref | Google Scholar |
Benhamou S (2011) Dynamic approach to space and habitat use based on biased random bridges. PLoS ONE 6(1), e14592.
| Crossref | Google Scholar | PubMed |
Block BA, Jonsen ID, Jorgensen SJ, Winship AJ, Shaffer SA, Bograd SJ, Hazen EL, Foley DG, Breed GA, Harrison A-L, Ganong JE, Swithenbank A, Castleton M, Dewar H, Mate BR, Shillinger GL, Schaefer KM, Benson SR, Weise MJ, Henry RW, Costa DP (2011) Tracking apex marine predator movements in a dynamic ocean. Nature 475, 86-90.
| Crossref | Google Scholar | PubMed |
Blumenthal JM, Solomon JL, Bell CD, Austin TJ, Ebanks-Petrie G, Coyne MS, Broderick AC, Godley BJ (2006) Satellite tracking highlights the need for international cooperation in marine turtle management. Endangered Species Research 2, 51-61.
| Crossref | Google Scholar |
Calenge C (2006) The package ‘adehabitat’ for the R software: a tool for the analysis of space and habitat use by animals. Ecological Modelling 197(3–4), 516-519.
| Crossref | Google Scholar |
Dryden J, Grech A, Moloney J, Hamann M (2008) Rezoning of the Great Barrier Reef World Heritage Area: does it afford greater protection for marine turtles? Wildlife Research 35(5), 477-485.
| Crossref | Google Scholar |
Dujon AM, Schofield G, Lester RE, Papafitsoros K, Hays GC (2018) Complex movement patterns by foraging loggerhead sea turtles outside the breeding season identified using Argos-linked Fastloc-Global Positioning System. Marine Ecology 39(1), e12489.
| Crossref | Google Scholar |
FitzSimmons NN, Limpus CJ (2014) Marine turtle genetic stocks of the Indo-Pacific: identifying boundaries and knowledge gaps. Indian Ocean Turtle Newsletter 20, 2-18.
| Google Scholar |
Fuentes MMPB, Wildermann N, Gandra TBR, Domit C (2020) Cumulative threats to juvenile green turtles in the coastal waters of southern and southeastern Brazil. Biodiversity and Conservation 29, 1783-1803.
| Crossref | Google Scholar |
Fukuoka T, Suganuma H, Kondo S, Sato K (2022) Long dive capacity of olive ridley turtles (Lepidochelys olivacea) at high water temperature during the post-nesting foraging period in the Arafura Sea. Journal of Experimental Marine Biology and Ecology 546, 151649.
| Crossref | Google Scholar |
Godley BJ, Richardson S, Broderick AC, Coyne MS, Glen F, Hays GC (2002) Long-term satellite telemetry of the movements and habitat utilisation by green turtles in the Mediterranean. Ecography 25, 352-362.
| Crossref | Google Scholar |
Godley BJ, Blumenthal JM, Broderick AC, Coyne MS, Godfrey MH, Hawkes LA, Witt MJ (2008) Satellite tracking of sea turtles: where have we been and where do we go next? Endangered Species Research 4, 3-22.
| Crossref | Google Scholar |
Gredzens C, Shaver DJ (2020) Satellite tracking can inform population-level dispersal to foraging grounds of post-nesting kemp’s ridley sea turtles. Frontiers in Marine Science 7, 559.
| Crossref | Google Scholar |
Gredzens C, Marsh H, Fuentes MMPB, Limpus CJ, Shimada T, Hamann M (2014) Satellite tracking of sympatric marine megafauna can inform the biological basis for species co-management. PLoS ONE 9, e98944.
| Crossref | Google Scholar | PubMed |
Hawkes LA, Broderick AC, Coyne MS, Godfrey MH, Lopez-Jurado L-F, Lopez-Suarez P, Merino SE, Varo-Cruz N, Godley BJ (2006) Phenotypically linked dichotomy in sea turtle foraging requires multiple conservation approaches. Current Biology 16(10), 990-995.
| Crossref | Google Scholar | PubMed |
Hays GC, Hawkes LA (2018) Satellite tracking sea turtles: opportunities and challenges to address key questions. Frontiers in Marine Science 5, 432.
| Crossref | Google Scholar |
Hays GC, Bailey H, Bograd SJ, Bowen WD, Campagna C, Carmichael RH, Casale P, Chiaradia A, Costa DP, Cuevas E, Nico de Bruyn PJ, Dias MP, Duarte CM, Dunn DC, Dutton PH, Esteban N, Friedlaender A, Goetz KT, Godley BJ, Halpin PN, Hamann M, Hammerschlag N, Harcourt R, Harrison A-L, Hazen EL, Heupel MR, Hoyt E, Humphries NE, Kot CY, Lea JSE, Marsh H, Maxwell SM, McMahon CR, Notarbartolo di Sciara G, Palacios DM, Phillips RA, Righton D, Schofield G, Seminoff JA, Simpfendorfer CA, Sims DW, Takahashi A, Tetley MJ, Thums M, Trathan PN, Villegas-Amtmann S, Wells RS, Whiting SD, Wildermann NE, Sequeira AMM (2019) Translating marine animal tracking data into conservation policy and management. Trends in Ecology & Evolution 34(5), 459-473.
| Crossref | Google Scholar | PubMed |
Hounslow JL, Fossette S, Chong W, Bali R, Tucker AD, Whiting SD, Gleiss AC (2023) Behaviour-specific spatiotemporal patterns of habitat use by sea turtles revealed using biologging and supervised machine learning. Journal of Applied Ecology 60(9), 1828-1840.
| Crossref | Google Scholar |
Hussey NE, Kessel ST, Aarestrup K, Cooke SJ, Cowley PD, Fisk AT, Harcourt RG, Holland KN, Iverson SJ, Kocik JF, Mills Flemming JE, Whoriskey FG (2015) Aquatic animal telemetry: a panoramic window into the underwater world. Science 348(6240), 1255642.
| Crossref | Google Scholar | PubMed |
Hyrenbach KD, Forney KA, Dayton PK (2000) Marine protected areas and ocean basin management. Aquatic Conservation: Marine and Freshwater Ecosystems 10(6), 437-458.
| Crossref | Google Scholar |
Lucchetti A, Pulcinella J, Angelini V, Pari S, Russo T, Cataudella S (2016) An interaction index to predict turtle bycatch in a Mediterranean bottom trawl fishery. Ecological Indicators 60, 557-564.
| Crossref | Google Scholar |
McMahon CR, Bradshaw CJA, Hays GC (2007) Satellite tracking reveals unusual diving characteristics for a marine reptile, the olive ridley turtle Lepidochelys olivacea. Marine Ecology Progress Series 329, 239-252.
| Crossref | Google Scholar |
Miller RL, Marsh H, Benham C, Hamann M (2019) A framework for improving the cross-jurisdictional governance of a marine migratory species. Conservation Science and Practice 1(8), e58.
| Crossref | Google Scholar |
Miller RL, Marsh H, Benham C, Hamann M (2020) Stakeholder engagement in the governance of marine migratory species: barriers and building blocks. Endangered Species Research 43, 1-19.
| Crossref | Google Scholar |
Pendoley KL, Schofield G, Whittock PA, Ierodiaconou D, Hays GC (2014) Protected species use of a coastal marine migratory corridor connecting marine protected areas. Marine Biology 161, 1455-1466.
| Crossref | Google Scholar |
Perez MA, Limpus CJ, Hofmeister K, Shimada T, Strydom A, Webster E, Hamann M (2022) Satellite tagging and flipper tag recoveries reveal migration patterns and foraging distribution of loggerhead sea turtles (Caretta caretta) from eastern Australia. Marine Biology 169, 80.
| Crossref | Google Scholar |
Poiner IR, Buckworth RC, Harris ANM (1990) Incidental capture and mortality of Sea Turtles in Australia’s northern Prawn Fishery. Australian Journal of Marine and Freshwater Research 41(1), 97-110.
| Crossref | Google Scholar |
Polovina JJ, Balazs GH, Howell EA, Parker DM, Seki MP, Dutton PH (2004) Forage and migration habitat of loggerhead (Caretta caretta) and olive ridley (Lepidochelys olivacea) sea turtles in the central North Pacific Ocean. Fisheries Oceanography 13(1), 36-51.
| Crossref | Google Scholar |
Riskas KA, Fuentes MMPB, Hamann M (2016) Justifying the need for collaborative management of fisheries bycatch: a lesson from marine turtles in Australia. Biological Conservation 196, 40-47.
| Crossref | Google Scholar |
Robins JB (1995) Estimated catch and mortality of sea turtles from the east coast otter trawl fishery of Queensland, Australia. Biological Conservation 74(3), 157-167.
| Crossref | Google Scholar |
Robins CM, Bache SJ, Kalish SR (2002) Bycatch of Sea Turtles in Pelagic Longline Fisheries – Australia. Agriculture, Fisheries and Forestry Australia. Available at https://www.bmis-bycatch.org/system/files/zotero_attachments/library_1/8E38W9FW%20-%20Robins_etal_2002_Turtle_bycatch_LL_Aus.pdf
Santos AJB, Bellini C, Santos EAP, Sales G, Ramos R, Vieira DHG, Marcovaldi MA, Gillis A, Wildermann N, Mills M, Gandra T, Fuentes MMPB (2021) Effectiveness and design of marine protected areas for migratory species of conservation concern: a case study of post-nesting hawksbill turtles in Brazil. Biological Conservation 261, 109229.
| Crossref | Google Scholar |
Schofield G, Hobson VJ, Fossette S, Lilley MKS, Katselidis KA, Hays GC (2010) Biodiversity research: fidelity to foraging sites, consistency of migration routes and habitat modulation of home range by sea turtles. Diversity and Distributions 16(5), 840-853.
| Crossref | Google Scholar |
Shimada T, Jones R, Limpus C, Hamann M (2012) Improving data retention and home range estimates by data-driven screening. Marine Ecology Progress Series 457, 171-180.
| Crossref | Google Scholar |
Shimada T, Jones R, Limpus C, Groom R, Hamann M (2016) Long-term and seasonal patterns of sea turtle home ranges in warm coastal foraging habitats: implications for conservation. Marine Ecology Progress Series 562, 163-179.
| Crossref | Google Scholar |
Shimada T, Limpus C, Jones R, Hamann M (2017) Aligning habitat use with management zoning to reduce vessel strike of sea turtles. Ocean & Coastal Management 142, 163-172.
| Crossref | Google Scholar |
Shimada T, Limpus CJ, Hamann M, Bell I, Esteban N, Groom R, Hays GC (2020) Fidelity to foraging sites after long migrations. Journal of Animal Ecology 89(4), 1008-1016.
| Crossref | Google Scholar | PubMed |
Shimada T, Thums M, Hamann M, Limpus CJ, Hy G, FitzSimmons NN, Wildermann NE, Duarte CM, Meekan MG (2021) Optimising sample sizes for animal distribution analysis using tracking data. Methods in Ecology and Evolution 12(2), 288-297.
| Crossref | Google Scholar |
Shuter JL, Broderick AC, Agnew DJ, Jonzén N, Godley BJ, Milner-Gulland EJ, Thirgood S (2011) Conservation and management of migratory species. In ‘Animal migration: a synthesis’. (Eds EJ Milner-Gulland, JM Fryxell, ARE Sinclair) pp. 172–206. (Oxford University Press) doi:10.1093/acprof:oso/9780199568994.003.0011
Sperling JB, Guinea ML (2004) A harness for attachment of satellite transmitters on flatback turtles. Marine Turtle Newsletter 103, 11-13.
| Google Scholar |
Thums M, Waayers D, Huang Z, Pattiaratchi C, Bernus J, Meekan M (2017) Environmental predictors of foraging and transit behaviour in flatback turtles Natator depressus. Endangered Species Research 32, 333-349.
| Crossref | Google Scholar |
Whiting SD, Long JL, Coyne M (2007) Migration routes and foraging behaviour of olive ridley turtles Lepidochelys olivacea in northern Australia. Endangered Species Research 3, 1-9.
| Crossref | Google Scholar |
Whittock PA, Pendoley KL, Hamann M (2014) Inter-nesting distribution of flatback turtles Natator depressus and industrial development in Western Australia. Endangered Species Research 26, 25-38.
| Crossref | Google Scholar |
Whittock PA, Pendoley KL, Hamann M (2016) Flexible foraging: post-nesting flatback turtles on the Australian continental shelf. Journal of Experimental Marine Biology and Ecology 477, 112-119.
| Crossref | Google Scholar |
Wilcox C, Hardesty BD, Sharples R, Griffin DA, Lawson TJ, Gunn R (2013) Ghostnet impacts on globally threatened turtles, a spatial risk analysis for northern Australia. Conservation Letters 6(4), 247-254.
| Crossref | Google Scholar |
Wildermann N, Critchell K, Fuentes MMPB, Limpus CJ, Wolanski E, Hamann M (2017) Does behaviour affect the dispersal of flatback post-hatchlings in the Great Barrier Reef? Royal Society Open Science 4(5), 170164.
| Crossref | Google Scholar | PubMed |